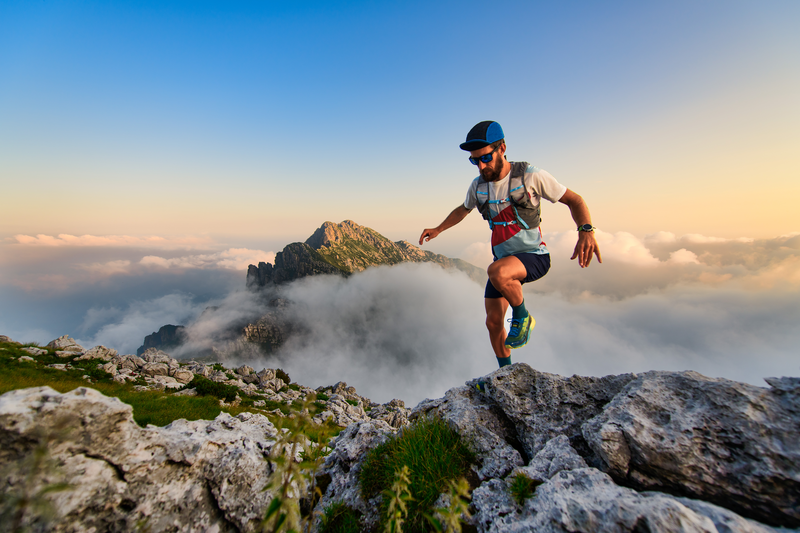
94% of researchers rate our articles as excellent or good
Learn more about the work of our research integrity team to safeguard the quality of each article we publish.
Find out more
ORIGINAL RESEARCH article
Front. Psychiatry
Sec. Addictive Disorders
Volume 15 - 2024 | doi: 10.3389/fpsyt.2024.1441815
The final, formatted version of the article will be published soon.
You have multiple emails registered with Frontiers:
Please enter your email address:
If you already have an account, please login
You don't have a Frontiers account ? You can register here
Background and Aims: Repetitive transcranial magnetic stimulation (rTMS) can be a potential therapeutic tool for the treatment of addiction, thanks to its ability to non-invasively modulate cortical excitability. In the present study, we investigated the short-and the long-term rTMS effects on craving behaviour and psychopathological symptoms in a sample of individuals suffering from gambling and cocaine use disorder.Methods: 42 individuals (age: 40.7±9.5 years; 40 M) underwent an initial screening testing craving behaviour, by means of visual analogue scales, and psychopathological symptoms, by means of Symptom Checklist-90-R. Participants were subsequently assigned to a real or sham (placebo) rTMS treatment of 2 weeks delivered over the left dorso-lateral prefrontal cortex. To assess the short-and long-term effects of rTMS, participants were evaluated again after 1, 2, 4, 8, 12, 16, 20 and 24 weeks.Results: After an initial similar trend in the craving behaviour of the two groups, our trend analysis showed a long-lasting decrease (until 24 weeks) in the real-rTMS group, following a linear trend (p<0.001); whereas the sham-rTMS group progressively returned to the initial level after about 12 weeks, following a quadratic trend (p<0.001). In addition, we observed moderate-to-strong correlations (0.4
Keywords: Addiction, craving, Cocaine, Gambling, rTMS, DLPFC, trend analysis, mixed models
Received: 31 May 2024; Accepted: 24 Oct 2024.
Copyright: © 2024 Casula, Chieco, Papaioannou, Frizzarin, Rocchi and Camporese. This is an open-access article distributed under the terms of the Creative Commons Attribution License (CC BY). The use, distribution or reproduction in other forums is permitted, provided the original author(s) or licensor are credited and that the original publication in this journal is cited, in accordance with accepted academic practice. No use, distribution or reproduction is permitted which does not comply with these terms.
* Correspondence:
Elias Paolo Casula, University of Rome Tor Vergata, Roma, Italy
Disclaimer: All claims expressed in this article are solely those of the authors and do not necessarily represent those of their affiliated organizations, or those of the publisher, the editors and the reviewers. Any product that may be evaluated in this article or claim that may be made by its manufacturer is not guaranteed or endorsed by the publisher.
Research integrity at Frontiers
Learn more about the work of our research integrity team to safeguard the quality of each article we publish.