- 1Department of Psychiatry, University of Colorado School of Medicine, Aurora, CO, United States
- 2Department of Radiology, University of Colorado School of Medicine, Aurora, CO, United States
- 3Department of Neurology, University of Colorado School of Medicine, Aurora, CO, United States
- 4Department of Neurosurgery, University of Colorado School of Medicine, Aurora, CO, United States
- 5National Institute on Drug Abuse, Bethesda, MD, United States
- 6Department of Neurosurgery, University of Nebraska Medical Center, Omaha, NE, United States
Substance use disorders are prevalent, causing extensive morbidity and mortality worldwide. Evidence-based treatments are of low to moderate effect size. Growth in the neurobiological understanding of addiction (e.g., craving) along with technological advancements in neuromodulation have enabled an evaluation of neurosurgical treatments for substance use disorders. Deep brain stimulation (DBS) involves surgical implantation of leads into brain targets and subcutaneous tunneling to connect the leads to a programmable implanted pulse generator (IPG) under the skin of the chest. DBS allows direct testing of neurobiologically-guided hypotheses regarding the etiology of substance use disorders in service of developing more effective treatments. Early studies, although with multiple limitations, have been promising. Still the authors express caution regarding implementation of DBS studies in this population and emphasize the importance of safeguards to ensure patient safety and meaningful study results. In this perspectives article, we review lessons learned through the years of planning an ongoing trial of DBS for methamphetamine use disorder.
Introduction
Substance use disorders (SUD) are common [e.g., 5-20% prevalence for alcohol use disorder alone (1)], and when severe, are often chronic, life-threatening disorders (2). Several evidence-based treatments for SUD are available but many patients do not respond to these interventions and new approaches are needed. To date most work has focused on psychosocial and pharmacological treatments. In recent decades, our understanding of the neurobiology of SUD has grown (3–6) and neuromodulation methods have been developed and tested for other disorders, paving the way for testing neuromodulation for SUD.
DBS is a neurosurgical procedure, which was first FDA-approved in the United States in 1997 for the treatment of tremor (7), and is well-established for treating movement disorders. Approximately 208,000 individuals have undergone DBS surgery worldwide (8). DBS involves stereotactic placement of an electrode (lead) into specific brain targets, which is connected via an extension cable to an implanted pulse generator (IPG). The IPG is positioned underneath the skin of the chest and is programmed by a treating clinician via a wirelessly-connected tablet to deliver electrical stimulation to one or more of the electrode contacts (Figure 1). DBS is an expensive procedure that carries surgical risk, and commonly requires at least an overnight hospital admission. Compared to transcranial magnetic stimulation (TMS), DBS provides targeted, constant, precise and highly titratable stimulation to deep brain structures.
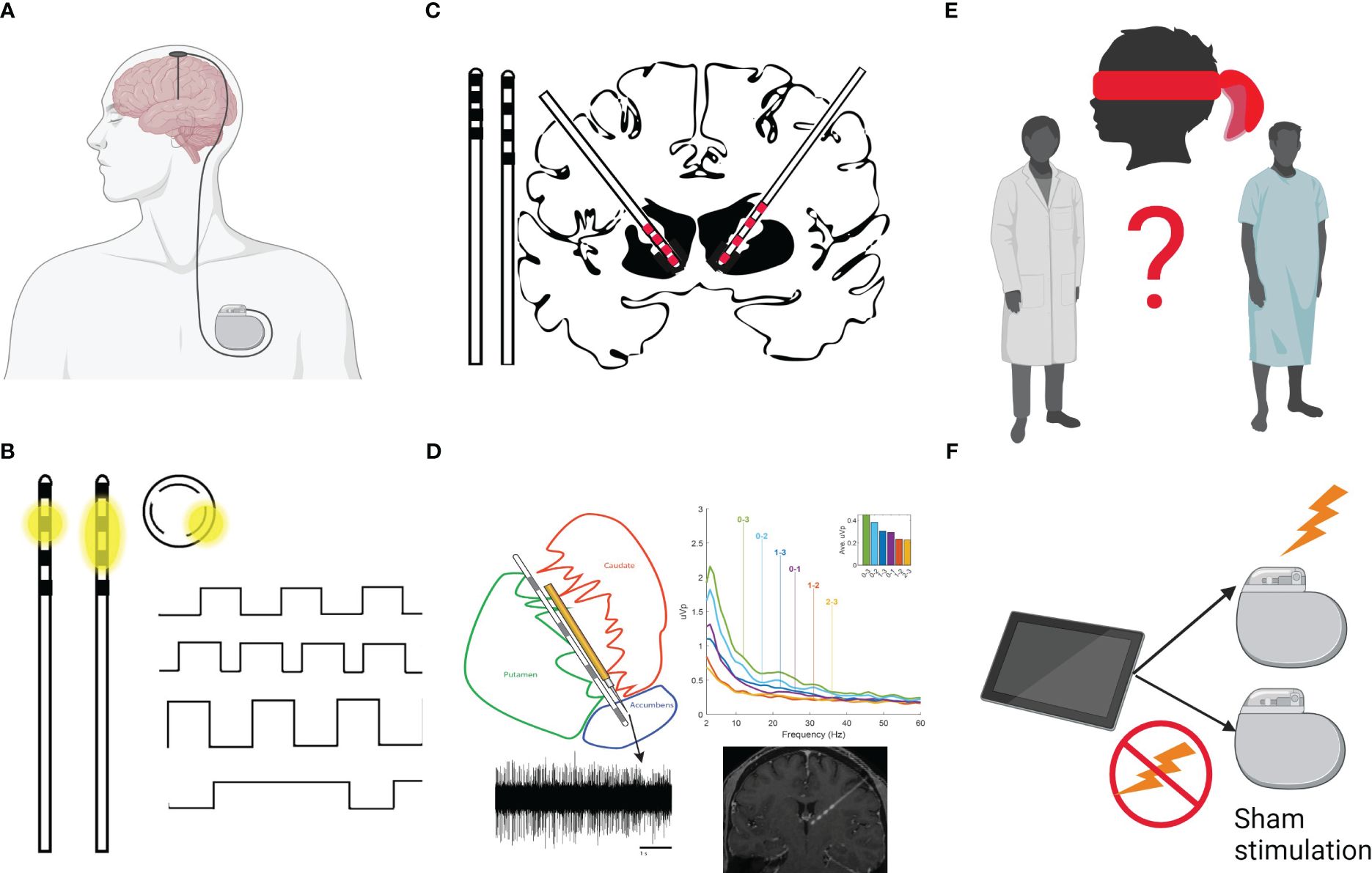
Figure 1 (A) The DBS system is composed of the lead (with electrodes at the tip), extension wires connecting the lead and Implantable Pulse Generator (IPG, battery). The neurosurgical procedure involves stereotactic placement of an electrode (lead) into specific brain targets. The lead is connected via an extension cable tunneled under the scalp, underneath the skin of the neck, and connected to a programmable battery (IPG) that is positioned underneath the skin of the chest and delivers electrical stimulation to one or more of the electrode contacts. (B) The tip of the lead generally has several electrodes (4 black squares here) spaced evenly apart. The battery can be programmed to send the stimulation through one contact (monopolar setting; e.g., with the case as the anode/+ and the electrode as the cathode/-) and the current will flow in a donut shape (dissipating with distance from the contact; see first inset image of a lead). Alternatively, the programmer can set one contact to serve as anode and one other as the cathode (bipolar setting, sending the current between contacts in an oval shape; see second inset lead image). Some leads allow “directional” stimulation (see circular inset image, which represents a cross-section of the lead). These leads allow sending the stimulation in one of 3 directions (e.g., anterior in a direction perpendicular to the lead). The shape of stimulation can help target specific structures based on patient imaging and offers alternative stimulation setting to mitigate reported side effects. The programmer can also control characteristics of the stimulation delivered. Programming includes designation of active contact(s), amplitude (voltage/mA), pulse width (µs), and frequency (Hz) of stimulation. The top image shows a stimulation setting with a rectangular waveform. The second wave form shows an increased frequency stimulation setting (more pulses/rectangles per second). The third wave form shows an increased amplitude (higher mA as shown by taller rectangles) and the final wave form shows a very long pulse width. (C) Leads vary in their spacing of the electrical contacts. To the left of the brain, two leads are shown side-by-side. The Medtronic leads B33005 (left; with 0.5mm spacing between contacts, which themselves are 1.5mm in length) and B33015 (right; with 1.5mm spacing between contacts). The brain image shows a typical approach to the Nucleus Accumbens (NAc) with the B33005 on the left and the B33015 on the right. Given an average height of the NAc here is estimated to be ~7mm (Neto et al., 2008), the B33015 lead placement (right part of the brain image) would allow the two most distal contacts to be placed in the NAc and electrode coverage superiorly into the anterior limb of the internal capsule. B33005 lead placement (left side of the brain image) in the NAc allows coverage similar to the first 3 contacts of the B33015 (e.g., bullet or rounded tip of the lead plus three 1.5mm contacts and two 1.5mm inter-contact spaces = bullet plus four 1.5mm contacts and three 0.5mm intercontact spaces = bullet + 7.5mm). Thus, the B33005 may allow tighter control to stimulate different portions of the NAc, but does not allow full coverage of the Anterior Limb of the Internal Capsule. (D) The Medtronic Sensight™ leads used in the authors’ trial, allow “sensing” or recording of local field potentials (LFP) or electrical potentials in the extracellular space (e.g., from a cluster of neurons in the nearby region). Unlike EEG, which has excellent temporal resolution but poor spatial resolution and MRI, which has excellent spatial resolution but limited temporal resolution, LFP measurement allows recording activity directly from a brain structure (in the authors’ study the NAc/BNST/ALIC; local field potential and MRI data represent modified versions of data published in Duffy et al., 2023) in real time (e.g., while presenting a cue craving paradigm). Sensing cannot be conducted while using bipolar stimulation and stimulation must be set using only the middle contacts in a monopolar fashion while recording. (E) Blinding of the patient and of the study team represents a major methodological challenge in the design of DBS studies. Because of the nuances in programming for substance use treatment, the Addiction Psychiatrist is appropriately the de facto programmer. Although the patient is blinded to the treatment arm, the psychiatrist not only knows the assigned arm but also adjusts program settings based on subjective clinical responses. There are ways to mitigate this confound, for example, by standardizing rather than individualizing programming or having an unblinded study member reset programming according to treatment arm after the psychiatrists’ evaluation. Each approach will have different limitations. Unfortunately, we are unaware of programming tablets which would allow blinding of the individual conducting programming. (F) Placebo response rates are relatively high for psychiatric conditions. As is the case for any new intervention, a randomized controlled trial of DBS should be the gold standard. Open-label DBS trials provide information on safety, tolerability, time to response and optimization of stimulation parameter settings. Many initially promising open-label DBS studies for psychiatric disorders, however, have been followed by placebo-controlled trials, with disappointing results. Figure created with BioRender.com.
The exact mechanism of DBS’ therapeutic effect is unknown, likely multifactorial, and may involve axonal stimulation, inhibition, and disruption of pathologic oscillations. Current research is aimed at understanding the effects of DBS at the molecular, cellular, and systems levels (9), determining optimal targets within neural networks and optimal stimulation parameters. Early animal work has suggested that DBS of the ventral tegmental area may attenuate drug-induced dopamine increases in the nucleus accumbens (NAc) following intravenous drug administration (10), and DBS of the NAc shell may reduce the extinction period for conditioned place preference and prevent drug-primed reinstatement (11). Early work in humans focusing on DBS and multiple SUDs has been promising as reviewed previously (12), suggesting high remission rates. In the only double-blind randomized controlled trial (RCT) published to date, a reduction in alcohol use was observed, but the trial failed to meet its primary endpoint of abstinence (13). Unlike DBS for the treatment of movement disorders, which has a well-characterized and acceptable risk-benefit profile, DBS for SUD is off-label, and trials require careful planning to address multiple population-specific concerns.
Safety and patient selection
DBS surgery is generally considered to be low risk, with a permanent surgical morbidity rate of ~1% (14). The most serious risks include intracranial hemorrhage, stroke, and infection sometimes requiring explantation of some or all DBS components. It is currently unclear whether associated morbidity and mortality differs in persons with SUD compared with other populations but available data to date do not suggest substantive differences (Table 1). Still, as more patients with SUD undergo surgery and different neurosurgical targets are tested/adopted, these results may change.

Table 1 Adverse events (AEs) and serious adverse events (SAEs) reported for deep brain stimulation for substance use disorder.
Which patients? Unlike medication trials that often recruit broadly, studies of DBS generally have been reserved for patients with severe, long-term, treatment-refractory SUD (12). Patient selection criteria have been well described for neurological disorders. Patients undergo determination of candidacy, then surgery if appropriate and patient is willing. Both patients and families generally participate in the programming process (38). In DBS candidacy determinations for movement disorders, all active psychiatric comorbidities must be identified and managed prior to surgery (38, 39). Presurgical evaluation often involves assessment of family and/or other social support (40). Severe SUD are associated with numerous psychiatric conditions, including personality disorders (41), and many individuals with severe SUD have disrupted family connections, socioeconomic challenges, and criminal justice involvement (42). Thus, while many patients with Parkinson’s disease recover post-operatively at home with supportive family members (e.g., providing a stable recovery environment), individuals with SUD may have limited support. DBS programming typically begins several weeks after surgery to allow for resolution of peri-lead post-operative edema which can temporarily suppress symptoms and confound determination of stimulation effect. Patients with severe, treatment-refractory SUD are at risk for relapse during this post-operative period. Thus, trials of DBS for individuals with SUD generally provide residential care following surgery, which adds logistical challenges and costs. These patients may also benefit from additional support (e.g., case management) upon discharge from residential care. In addition, SUDs can be viewed in part as subverting motivational systems and prioritizing drug reinforcement (e.g., pursuit of the drug to the detriment of roles/positive relationships), leading to fluctuations in level of treatment motivation across time. Thus, studies of DBS for SUD are faced with a conundrum: recruit individuals with severe, treatment-refractory SUD, but prioritize those who have more treatment motivation and family support and hence may be more likely to comply with long-term study participation requirements. Such individuals, however, represent a small subsample of those with SUD.
The potential for harm from DBS must be considered in patient selection. Prior work has reported suicidal ideation and completed suicides during DBS treatment for other conditions (43), leading some to conclude that those at high risk for suicide should be excluded from DBS consideration (44), though this has been debated in depression trials (45). Those with severe SUD may also have co-morbid serious medical concerns which must be carefully assessed in relation to DBS risks. Another concern is the risk of infection due to bacterial colonization of the DBS hardware (46). Should people who inject drugs be excluded from enrollment in DBS studies given potential for increased infection risk? Excluding these individuals may exclude those with the greatest need. SUDs in the US are commonly associated with criminal justice involvement/incarceration (47). Given that studies of DBS involve a small number of individuals enrolled over long periods, what happens if a patient enrolled in a DBS trial becomes incarcerated?
Trial design issues
Although early open label studies have suggested long-term improvement in SUD phenotypes (i.e., long-term remission), there are multiple design choices which may impact confidence in trial results, including target selection, surgical approach, approach to programming, expected time courses, and blinding.
Which target? The neurobiological understanding of SUD has grown dramatically from a focus mainly on reward-related processes (the dopamine hypothesis) to including network based models, and including a broad number of brain regions and circuits (48, 49). Thus, target selection for the treatment of a specific SUD is complex. Animal models have been used to test a broad range of DBS targets including the subthalamic nucleus, substantia nigra, amygdala, anterior cingulate, insula, hippocampus, infralimbic and prelimbic cortices, along with NAc (50–55). Cortical regions (e.g., dorsolateral prefrontal cortex, ventromedial prefrontal cortex, and anterior cingulate) are generally accessible to TMS, and studies of DBS generally focus on subcortical regions. To our knowledge, DBS in SUD, including early case reports showing substance use outcomes in patients treated for other conditions (25, 26, 30), has generally focused on NAc, anterior limb of the internal capsule, bed nucleus of the stria terminalis, and ventral capsule-ventral striatum region (a broader region which includes NAc) (12). However, a trial focusing on the limbic pallidum for alcohol use disorder was funded in 2022 (AA030505). Current DBS lead technology is not ideally suited to differentially stimulate intimately adjacent structures (e.g., shell vs. core of NAc). In such instances, stimulation might inadvertently result in opposing effects—e.g., lateral versus medial NAc shell (56) or core versus shell (50), or vary depending on white matter fiber connections to cortical regions (57). The large number of candidate targets speaks to limitations in our current understanding of the fundamental pathophysiology and neural circuitry of SUD, but also highlights the possibility that thoughtful target selection informed by our current neurobiological models, may further inform our understanding of these disorders.
Surgical planning
The use of 3T MRI-guidance (or CT-guided with MRI fusion) for stereotactic procedures allows accurate targeting of many brain structures. Individual anatomy may require adjustments to trajectory—for instance, the need to avoid cortical veins, which are highly variable between individuals, or the targeting of white matter tracts, if indicated, which also vary between individuals. Significant brain-shift from opening the dura and subsequent air entry is a potential targeting confound when using preoperative image guidance. Standard surgical techniques can be used to reduce the likelihood of significant brain shift—such as positioning the patient with minimal head-of-bed elevation, and the use of intraoperative MRI to account for any brain shift during the surgical procedure is also an option.
Programming plan
In Parkinson’s disease, tremor, rigidity, and bradykinesia provide quantifiable symptoms against which to set stimulation parameters and select active contacts. Although a half-smile may be observed during DBS programming for obsessive compulsive disorder (58), stimulation settings for psychiatric conditions generally rely on subjective self-report (e.g., patient self-rating of mood, anxiety, energy, and side effects) to identify the optimal stimulation settings. It remains unclear whether self-report represents a reliable and valid measure for guiding stimulation parameter settings in SUD. The subjectivity of response and complexity of the DBS programming parameter space (Figure 1) presents significant challenges (59). Thus, a pressing goal in DBS for the treatment of psychiatric conditions is to identify objective measures for optimizing stimulation parameters (60), such as using symptom-relevant features of the local field potential (LFP) spectrum (61) and imaging NAc during cue craving, as has been done for food craving (62). Many of the published case reports on DBS for SUD include the final stimulation parameter settings (12), but do not describe the approach to optimizing these parameters. Post-operative brain imaging can be used to inform contact selection for programming by examining location of individual contacts and through estimation of volume of tissue activation [i.e., shaping the stimulation field based on local anatomy (63)]. Bach and colleagues (13) describe a proscribed stimulation protocol for parameter selection in their recent RCT, which would be easily reproducible in clinical practice, analogous to the careful dosing guidelines used in medication trials.
Time course to expected response
A challenge in DBS trial design for SUD is predicting time to clinical response. For depression, treatment response may take a minimum of 6 months or as long as 1-2 years, with increasing response rates generally observed with longer follow-up (43, 64). Post-hoc assessment of the BROADEN Trial (subgenual cingulate white matter stimulation for treatment-refractory depression) data has suggested that prolonged time to response may have played a role in the interim futility analyses that halted the trial. This experience has underscored the importance of designing trials of DBS for SUD to capture long-term effects (65). Furthermore, the time necessary for washout of the effects of stimulation is unclear, which creates difficulties in designing cross-over studies (i.e., it is unclear how long is needed for a subject’s symptoms to return to baseline after discontinuation of stimulation).
For SUD, response rates have been reported to occur both rapidly/immediately following onset of stimulation (29) and as late as 10 months after beginning stimulation (30). The only double-blind RCT of DBS for SUD published to date, used a 6-month follow up period, with one group off and one on stimulation, followed by open-label stimulation for 12 months. Symptom amelioration, on average, was seen within the first two weeks of stimulation (13). As noted above, residential treatment may be used during the post-surgical recovery period, and may impact relapse rates at 3 months, and even 1 year, after discharge (66); effects may be confounded by adjunctive treatments in open-label designs. Finally, microlesion effects (i.e., post-operative edema in the targeted brain structure) have been previously reported up to 2-3 months following surgery (67).
Trial design-placebo and blinding
As with pharmaceutical interventional studies for psychiatric conditions, blinding provides protection against bias in neuromodulation studies. DBS case reports and case series can be criticized for lacking both blinding and sham stimulation. There are, however, challenges to blinding and design of sham-control arms in DBS studies (Figure 1). Because DBS surgery and residential substance treatment is expensive, and the follow-up period is long, studies will likely only enroll a small number of subjects. Crossover designs using the same participant on- and off-stimulation may enhance power and account for ordering effects.
Ethical issues
DBS for treatment of patients with SUD raises several ethical considerations including, but not limited to, considerations around informed consent and trial completion.
Informed consent
Patients with SUD are not de facto challenged from a decision-making vantage and are capable of providing informed consent for study participation, though such consent cannot be given in the context of acute intoxication or withdrawal. When selecting patients who are severely affected and treatment-refractory for a novel intervention, there is always the potential for misunderstanding the likelihood of treatment effect and the experimental nature of the study. A patient advocate can provide an additional and important voice during these meetings. Managing patient expectations is an important part of the informed consent process and utilization of visual aids to help potential participants understand the surgical procedures is paramount. Our study employs a Multi-disciplinary Ethics Board, chaired by a bioethicist, and includes our patient advocate, and outside representatives with expertise in Addiction Psychiatry, Neurology, and Neurosurgery. This board reviews all potential participants recommended by the study team and prevents surgery with a single “No” vote.
Battery life, concluding the trial, costs
DBS leads and extension cables are permanently implanted devices while the IPG has a finite lifespan, the duration of which is dependent on stimulation parameters and whether or not a rechargeable IPG is used. Currently available IPGs—including rechargeable IPGs— require periodic and repeated replacement over the life of the patient. The life of the IPG is related to the therapeutic stimulation parameters selected, with certain configurations (e.g., higher mA) causing faster battery depletion. Battery life can range from 7 months to several years, requiring plans for possible IPG replacement surgery over the study duration. Rechargeable batteries can increase time until battery replacement, but recharging may pose compliance issues for some patients with SUD. Sensing and storage of LFP signals will also shorten battery life. At the conclusion of study participation for a medication trial, the subject no longer uses the study medication. At the conclusion of a DBS trial, if a participant is doing well, who will cover the cost of IPG replacement surgery or ongoing care? Should enrollment in a longer-term open-label follow-up study be offered? Who will cover costs if explantation is required? Such questions have been considered previously (68), but we are unaware of any clear guidance and each research team must carefully consider such questions at the outset of the trial and include relevant information in the informed consent process for study participation.
Discussion
Studies of DBS for treatment-refractory SUD are currently underway in the US, Europe, and Asia, and offer hope for more effective treatments and enhanced understanding of the neurobiological underpinnings of SUD. Early results of single case reports and open-label trials have been promising, with some individuals experiencing sustained remission (12). However, such trial results come with many important considerations and concerns. From our experience in planning and implementing one such study, we present five recommendations to guide future DBS trials for SUD.
(1) Case reports and small, open-label studies have now been published suggesting that DBS of the NAc may provide benefit to individuals with different SUDs. Future studies must implement more rigorous trial design to control for placebo effects, which may be nontrivial with surgical intervention (69) and in addiction treatment (70). Because of costs and the usually extensive requirements for participant selection, sample sizes will generally remain small, but additional open-label studies will be inadequate to convincingly demonstrate efficacy. Multi-site studies may be required, along with utilization of designs which maximize power with modest sample sizes (e.g., cross-over design), to push the field forward. The field should also focus on development of standard approaches to sham programming that maintains the blind.
(2) Table 1 reviews the available literature on adverse events in DBS for SUD trials. It is important to note that the available data do not raise new safety concerns for DBS in this population. However, many prior papers do not provide details on how adverse events were monitored and because of an open trial design, cannot comment on rates of adverse events in sham vs. active stimulation. Adverse events should be systematically assessed and reported in all trials to better understand risks in this population. Careful assessment of adverse events will also help inform future potential participants and investigators regarding the risk-to-benefit ratio.
(3) Prior studies often do not carefully explain approaches to stimulation parameter setting or subsequent optimization. Reproducibility requires careful protocolization of stimulation parameters and documentation of those approaches. Ideally, future studies will collect objective markers (e.g., MRI, LFP) which may aid in the development of objective measures to guide programming.
(4) Unlike medication trials, DBS trials involve surgical implantation of hardware. Because of this, research teams should work to carefully anticipate long-term patient needs and provide information to potential participants during the informed consent process about what can, and cannot, be provided throughout the study (e.g., explantation, battery replacement, etc.).
(5) Ethical issues in DBS for SUD (from patient selection and informed consent to conduct and conclusion of the trial) must be delineated and managed in protocol development.
Future Directions: DBS, in relation to other neuromodulation techniques, may offer some advantages which should be pursued. For example, DBS with its ability to provide long-term stimulation to deep structures likely enhances durability and precision of treatment response (e.g., relative to some noninvasive stimulation approaches) and this should be investigated. Advances in the measurement of symptom-relevant LFP-derived biomarker may also inform our understanding of the neurobiology of addiction and may open pathways to develop closed loop approaches (e.g., IPG senses LFP changes signifying craving and provides stimulation). Thus, while increasing rigor is needed to define treatment effects, these technologies may offer ways to advance our fundamental understanding of addiction-related process.
Data availability statement
The original contributions presented in the study are included in the article/supplementary material. Further inquiries can be directed to the corresponding author.
Author contributions
JS: Conceptualization, Funding acquisition, Writing – original draft, Writing – review & editing. JT: Conceptualization, Funding acquisition, Writing – review & editing. SB: Writing – review & editing. MZ: Writing – review & editing. SM-G: Writing – review & editing. DK: Writing – review & editing. JAT: Writing – review & editing. KR: Writing – review & editing. PG: Writing – review & editing. KF: Writing – review & editing. AA: Conceptualization, Funding acquisition, Writing – review & editing.
Funding
The author(s) declare financial support was received for the research, authorship, and/or publication of this article. This work was supported by the National Institute on Drug Abuse grant UG3DA054746 and funding from the Kane Family and Hewit Family Foundations. MZ is part of the CU Pathways Resident Research Track (R25MH125758). JS is also supported by K24DA058882.
Conflict of interest
DK has served as an advisor for Colorado Clinical and Translational Sciences Institute CCTSI Data Safety Monitoring Board, and Medical Boards for Boston Scientific, Medtronic, and AbbVie Pharmaceutics; received honorarium from AbbVie Pharmaceutics, Abbott, and Boston Scientific, received grants from the Boston Scientific, Medtronic, University of Colorado Department of Neurology, and the Parkinson’s Foundation. AA receives investigator-initiated research funding and received implantable research devices from, and serves on a post approval clinical events committee (epilepsy therapy) for Medtronic. JT has received consulting fees from Icometrix. JAT received grants and honorarium from Medtronic.
The remaining authors declare that the research was conducted in the absence of any commercial or financial relationships that could be construed as a potential conflict of interest.
Publisher’s note
All claims expressed in this article are solely those of the authors and do not necessarily represent those of their affiliated organizations, or those of the publisher, the editors and the reviewers. Any product that may be evaluated in this article, or claim that may be made by its manufacturer, is not guaranteed or endorsed by the publisher.
Author disclaimer
The views and opinions expressed in this manuscript are those of the authors only and do not necessarily represent the views, official policy, or position of the United States Department of Health and Human Services or any of its affiliated institutions or agencies.
References
1. Mojtabai R. Estimating the prevalence of substance use disorders in the US using the benchmark multiplier method. JAMA Psychiatry. (2022) 79:1074. doi: 10.1001/jamapsychiatry.2022.2756
2. Available online at: https://nida.nih.gov/research-topics/trends-statistics/overdose-death-rates#:~:text=There%20were%20106%2C699%20drug%2Dinvolved,to%202021%20(Figure%202).
3. Adinoff B. Neurobiologic processes in drug reward and addiction. Harvard Rev Psychiatry. (2004) 12:305–20. doi: 10.1080/10673220490910844
4. Cooper S, Robison A, Mazei-Robison MS. Reward circuitry in addiction. Neurotherapeutics. (2017) 14:687–97. doi: 10.1007/s13311-017-0525-z
5. Hyman SE, Malenka RC. Addiction and the brain: the neurobiology of compulsion and its persistence. Nat Rev Neurosci. (2001) 2:695–703. doi: 10.1038/35094560
6. Lüscher C. The emergence of a circuit model for addiction. Annu Rev Neurosci. (2016) 39:257–76. doi: 10.1146/annurev-neuro-070815-013920
7. Deng H, Yue JK, Wang DD. Trends in safety and cost of deep brain stimulation for treatment of movement disorders in the United States: 2002–2014. Br J Neurosurg. (2021) 35:57–64. doi: 10.1080/02688697.2020.1759776
8. Vedam-Mai V, Deisseroth K, Giordano J, Lazaro-Munoz G, Chiong W, Suthana N, et al. Proceedings of the eighth annual deep brain stimulation think tank: Advances in optogenetics, ethical issues affecting DBS research, neuromodulatory approaches for depression, adaptive neurostimulation, and emerging DBS technologies. Front Hum Neurosci. (2021) 15:644593. doi: 10.3389/fnhum.2021.644593
9. Lozano AM, Lipsman N, Bergman H, Brown P, Chabardes S, Chang JW, et al. Deep brain stimulation: current challenges and future directions. Nat Rev Neurol. (2019) 15:148–60. doi: 10.1038/s41582-018-0128-2
10. Yuen J, Goyal A, Rusheen AE, Kouzani AZ, Berk M, Kim JH, et al. Oxycodone-induced dopaminergic and respiratory effects are modulated by deep brain stimulation. Front Pharmacol. (2023) 14:1199655. doi: 10.3389/fphar.2023.1199655
11. Eskandari K, Fattahi M, Riahi E, Khosrowabadi R, Haghparast A. A wide range of Deep Brain Stimulation of the nucleus accumbens shell time independently reduces the extinction period and prevents the reinstatement of methamphetamine-seeking behavior in rats. Life Sci. (2023) 319:121503. doi: 10.1016/j.lfs.2023.121503
12. Mahoney JJ III, Koch-Gallup N, Scarisbrick DM, Berry JH, Rezai AR. Deep brain stimulation for psychiatric disorders and behavioral/cognitive-related indications: Review of the literature and implications for treatment. J Neurological Sci. (2022) 437:120253. doi: 10.1016/j.jns.2022.120253
13. Bach P, Luderer M, Müller UJ, Jakobs M, Baldermann JC, Voges J, et al. Deep brain stimulation of the nucleus accumbens in treatment-resistant alcohol use disorder: a double-blind randomized controlled multi-center trial. Trans Psychiatry. (2023) 13:49. doi: 10.1038/s41398-023-02337-1
14. Voges J, Hilker R, Bötzel K, Kiening KL, Kloss M, Kupsch A, et al. Thirty days complication rate following surgery performed for deep-brain-stimulation. Movement disorders: Off J Movement Disord Soc. (2007) 22:1486–9. doi: 10.1002/mds.21481
15. Chen L, Li N, Ge S, Lozano AM, Lee DJ, Yang C, et al. Long-term results after deep brain stimulation of nucleus accumbens and the anterior limb of the internal capsule for preventing heroin relapse: an open-label pilot study. Brain stimulation. (2019) 12:175–83. doi: 10.1016/j.brs.2018.09.006
16. Ge S, Geng X, Wang X, Li N, Chen L, Zhang X, et al. Oscillatory local field potentials of the nucleus accumbens and the anterior limb of the internal capsule in heroin addicts. Clin Neurophysiol. (2018) 129:1242–53. doi: 10.1016/j.clinph.2018.03.008
17. Davidson B, Giacobbe P, George TP, Nestor SM, Rabin JS, Goubran M, et al. Deep brain stimulation of the nucleus accumbens in the treatment of severe alcohol use disorder: a phase I pilot trial. Mol Psychiatry. (2022) 27:3992–4000. doi: 10.1038/s41380-022-01677-6
18. Ge S, Chen Y, Li N, Qu L, Li Y, Jing J, et al. Deep brain stimulation of nucleus accumbens for methamphetamine addiction: two case reports. World Neurosurg. (2019) 122:512–7. doi: 10.1016/j.wneu.2018.11.056
19. Gonçalves-Ferreira A, do Couto FS, Campos AR, Neto LPL, Gonçalves-Ferreira D, Teixeira J. Deep brain stimulation for refractory cocaine dependence. Biol Psychiatry. (2016) 79:e87–9. doi: 10.1016/j.biopsych.2015.06.023
20. Heinze H-J, Heldmann M, Voges J, Hinrichs H, Marco-Pallares J, Hopf J-M, et al. Counteracting incentive sensitization in severe alcohol dependence using deep brain stimulation of the nucleus accumbens: clinical and basic science aspects. Front Hum Neurosci. (2009) 22. doi: 10.3389/neuro.09.022.2009
21. Müller U, Sturm V, Voges J, Heinze H-J, Galazky I, Heldmann M, et al. Successful treatment of chronic resistant alcoholism by deep brain stimulation of nucleus accumbens: first experience with three cases. Pharmacopsychiatry. (2009) 42:288–91. doi: 10.1055/s-0029-1233489
22. Müller U, Sturm V, Voges J, Heinze H-J, Galazky I, Büntjen L, et al. Nucleus accumbens deep brain stimulation for alcohol addiction–safety and clinical long-term results of a pilot trial. Pharmacopsychiatry. (2016) 49:170–3. doi: doi: 10.1055/s-0042-104507
23. Voges J, Müller U, Bogerts B, Münte T, Heinze H-J. Deep brain stimulation surgery for alcohol addiction. World Neurosurg. (2013) 80:S28. e21–S28. e31. doi: 10.1016/j.wneu.2012.07.011
24. Heldmann M, Berding G, Voges J, Bogerts B, Galazky I, Müller U, et al. Deep brain stimulation of nucleus accumbens region in alcoholism affects reward processing. PloS One. (2012) 7:e36572. doi: 10.1371/journal.pone.0036572
25. Kuhn J, Lenartz D, Huff W, Lee S, Koulousakis A, Klosterkoetter J, et al. Remission of alcohol dependency following deep brain stimulation of the nucleus accumbens: valuable therapeutic implications? J Neurology Neurosurg Psychiatry. (2007) 78:1152–3. doi: 10.1136/jnnp.2006.113092
26. Kuhn J, Bauer R, Pohl S, Lenartz D, Huff W, Kim EH, et al. Observations on unaided smoking cessation after deep brain stimulation of the nucleus accumbens. Eur Addict Res. (2009) 15:196–201. doi: 10.1159/000228930
27. Kuhn J, Gründler TO, Bauer R, Huff W, Fischer AG, Lenartz D, et al. Successful deep brain stimulation of the nucleus accumbens in severe alcohol dependence is associated with changed performance monitoring. Addict Biol. (2011) 16:620–3. doi: 10.1111/j.1369-1600.2011.00337.x
28. Kuhn J, Möller M, Treppmann J, Bartsch C, Lenartz D, Gründler TO, et al. Deep brain stimulation of the nucleus accumbens and its usefulness in severe opioid addiction. Mol Psychiatry. (2014) 19:145–6. doi: 10.1038/mp.2012.196
29. Mahoney JJ III, Haut MW, Hodder SL, Zheng W, Lander LR, Berry JH, et al. Deep brain stimulation of the nucleus accumbens/ventral capsule for severe and intractable opioid and benzodiazepine use disorder. Exp Clin Psychopharmacol. (2021) 29:210. doi: 10.1037/pha0000453
30. Mantione M, van de Brink W, Schuurman PR, Denys D. Smoking cessation and weight loss after chronic deep brain stimulation of the nucleus accumbens: therapeutic and research implications: case report. Neurosurgery. (2010) 66:E218–8. doi: 10.1227/01.NEU.0000360570.40339.64
31. Rezai AR, Mahoney JJ, Ranjan M, Haut MW, Zheng W, Lander LR, et al. Safety and feasibility clinical trial of nucleus accumbens deep brain stimulation for treatment-refractory opioid use disorder. J Neurosurg. (2023) 140:231–9. doi: 10.3171/2023.4.JNS23114
32. Valencia-Alfonso C-E, Luigjes J, Smolders R, Cohen MX, Levar N, Mazaheri A, et al. Effective deep brain stimulation in heroin addiction: a case report with complementary intracranial electroencephalogram. Biol Psychiatry. (2012) 71:e35–7. doi: 10.1016/j.biopsych.2011.12.013
33. Zhang C, Wei H, Zhang Y, Du J, Liu W, Zhan S, et al. Increased dopamine transporter levels following nucleus accumbens deep brain stimulation in methamphetamine use disorder: a case report. Brain Stimulation: Basic Translational Clin Res Neuromodulation. (2019) 12:1055–7. doi: 10.1016/j.brs.2019.02.023
34. Zhou H, Xu J, Jiang J. Deep brain stimulation of nucleus accumbens on heroin-seeking behaviors: a case report. Biol Psychiatry. (2011) 69:e41–2. doi: 10.1016/j.biopsych.2011.02.012
35. Zhu R, Zhang Y, Wang T, Wei H, Zhang C, Li D, et al. Deep brain stimulation of nucleus accumbens with anterior capsulotomy for drug addiction: a case report. Stereotactic Funct Neurosurg. (2020) 98:345–9. doi: 10.1159/000509313
36. Patel DM, Walker HC, Brooks R, Omar N, Ditty B, Guthrie BL. Adverse events associated with deep brain stimulation for movement disorders: analysis of 510 consecutive cases. Operative Neurosurg. (2015) 11:190–9. doi: 10.1227/NEU.0000000000000659
37. Kisely S, Hall K, Siskind D, Frater J, Olson S, Crompton D. Deep brain stimulation for obsessive–compulsive disorder: a systematic review and meta-analysis. psychol Med. (2014) 44:3533–42. doi: 10.1017/S0033291714000981
38. Rodriguez RL, Fernandez HH, Haq I, Okun MS. Pearls in patient selection for deep brain stimulation. neurologist. (2007) 13:253–60. doi: 10.1097/NRL.0b013e318095a4d5
39. Pollak P. Deep brain stimulation for Parkinson’s disease–patient selection. Handb Clin Neurol. (2013) 116:97–105. doi: 10.1016/B978-0-444-53497-2.00009-7
40. Moro E, Lang AE. Criteria for deep-brain stimulation in Parkinson’s disease: review and analysis. Expert Rev Neurother. (2006) 6:1695–705. doi: 10.1586/14737175.6.11.1695
41. Compton WM, Thomas YF, Stinson FS, Grant BF. Prevalence, correlates, disability, and comorbidity of DSM-IV drug abuse and dependence in the United States: results from the national epidemiologic survey on alcohol and related conditions. Arch Gen Psychiatry. (2007) 64:566–76. doi: 10.1001/archpsyc.64.5.566
42. Lander L, Howsare J, Byrne M. The impact of substance use disorders on families and children: from theory to practice. Soc work Public Health. (2013) 28:194–205. doi: 10.1080/19371918.2013.759005
43. Mosley PE, Marsh R. The psychiatric and neuropsychiatric symptoms after subthalamic stimulation for Parkinson’s disease. J Neuropsychiatry Clin Neurosci. (2015) 27:19–26. doi: 10.1176/appi.neuropsych.14040069
44. Burkhard PR, Vingerhoets F, Berney A, Bogousslavsky J, Villemure J-G, Ghika J. Suicide after successful deep brain stimulation for movement disorders. Neurology. (2004) 63:2170–2. doi: 10.1212/01.WNL.0000145603.48221.B5
45. Coulter A. Deep brain stimulation research protocols and depressive patients with previous suicidality: We can’t afford to lose focus. AJOB Neurosci. (2013) 4:41–2. doi: 10.1080/21507740.2012.748707
46. Doshi PK. Long-term surgical and hardware-related complications of deep brain stimulation. Stereotactic Funct Neurosurg. (2011) 89:89–95. doi: 10.1159/000323372
47. Slade EP, Stuart EA, Salkever DS, Karakus M, Green KM, Ialongo N. Impacts of age of onset of substance use disorders on risk of adult incarceration among disadvantaged urban youth: A propensity score matching approach. Drug Alcohol Depend. (2008) 95:1–13. doi: 10.1016/j.drugalcdep.2007.11.019
48. Koob G, Volkow ND. Neurocircuit van verslaving. Neuropsychopharmacology. (2010) 35:217–38. doi: 10.1038/npp.2009.110
49. Koob GF, Volkow ND. Neurobiology of addiction: a neurocircuitry analysis. Lancet Psychiatry. (2016) 3:760–73. doi: 10.1016/S2215-0366(16)00104-8
50. Vassoler FM, White SL, Hopkins TJ, Guercio LA, Espallergues J, Berton O, et al. Deep brain stimulation of the nucleus accumbens shell attenuates cocaine reinstatement through local and antidromic activation. J Neurosci. (2013) 33:14446–54. doi: 10.1523/JNEUROSCI.4804-12.2013
51. Zhang L, Meng S, Chen W, Chen Y, Huang E, Zhang G, et al. High-frequency deep brain stimulation of the substantia nigra pars reticulata facilitates extinction and prevents reinstatement of methamphetamine-induced conditioned place preference. Front Pharmacol. (2021) 12:705813. doi: 10.3389/fphar.2021.705813
52. Degoulet M, Tiran-Cappello A, Combrisson E, Baunez C, Pelloux Y. Subthalamic low-frequency oscillations predict vulnerability to cocaine addiction. Proc Natl Acad Sci. (2021) 118:e2024121118. doi: 10.1073/pnas.2024121118
53. Liu HY, Jin J, Tang JS, Sun WX, Jia H, Yang XP, et al. Preclinical study: chronic deep brain stimulation in the rat nucleus accumbens and its effect on morphine reinforcement. Addict Biol. (2008) 13:40–6. doi: 10.1111/j.1369-1600.2007.00088.x
54. Hamilton J, Lee J, Canales J. Chronic unilateral stimulation of the nucleus accumbens at high or low frequencies attenuates relapse to cocaine seeking in an animal model. Brain stimulation. (2015) 8:57–63. doi: 10.1016/j.brs.2014.09.018
55. Chang H, Gao C, Sun K, Xiao L, Li X, Jiang S, et al. Continuous high frequency deep brain stimulation of the rat anterior insula attenuates the relapse post withdrawal and strengthens the extinction of morphine seeking. Front Psychiatry. (2020) 11:577155. doi: 10.3389/fpsyt.2020.577155
56. van der Plasse G, Schrama R, van Seters SP, Vanderschuren LJ, Westenberg HG. Deep brain stimulation reveals a dissociation of consummatory and motivated behaviour in the medial and lateral nucleus accumbens shell of the rat. PloS One. (2012) 7:e33455. doi: 10.1371/journal.pone.0033455
57. Haber SN, Yendiki A, Jbabdi S. Four deep brain stimulation targets for obsessive-compulsive disorder: are they different? Biol Psychiatry. (2021) 90:667–77. doi: 10.1016/j.biopsych.2020.06.031
58. Okun MS, Bowers D, Springer U, Shapira NA, Malone D, Rezai AR, et al. What’s in a “smile?” Intra-operative observations of contralateral smiles induced by deep brain stimulation. Neurocase. (2004) 10:271–9. doi: 10.1080/13554790490507632
59. Widge AS, Dougherty DD. Managing patients with psychiatric conditions treated with deep brain stimulation. In: Deep brain stimulation management. Cambridge: Cambridge University Press (2015). p. 124–37.
60. Wendt K, Denison T, Foster G, Krinke L, Thomson A, Wilson S, et al. Physiologically informed neuromodulation. J Neurological Sci. (2022) 434:120121. doi: 10.1016/j.jns.2021.120121
61. Duffy KA, Fenstermacher EA, Thompson JA, Tanabe J, Patel MS, Ojemann S, et al. Case report: Clinical efficacy of deep brain stimulation contacts corresponds to local field potential signals in a patient with obsessive-compulsive disorder. Front Psychiatry. (2023) 14:1279972. doi: 10.3389/fpsyt.2023.1279972
62. Shivacharan RS, Rolle CE, Barbosa DAN, Cunningham TN, Feng A, Johnson ND, et al. Pilot study of responsive nucleus accumbens deep brain stimulation for loss-of-control eating. Nat Med. (2022) 28:1791–6. doi: 10.1038/s41591-022-01941-w
63. Butson CR, Cooper SE, Henderson JM, McIntyre CC. Patient-specific analysis of the volume of tissue activated during deep brain stimulation. Neuroimage. (2007) 34:661–70. doi: 10.1016/j.neuroimage.2006.09.034
64. Holtzheimer PE, Husain MM, Lisanby SH, Taylor SF, Whitworth LA, McClintock S, et al. Subcallosal cingulate deep brain stimulation for treatment-resistant depression: a multisite, randomised, sham-controlled trial. Lancet Psychiatry. (2017) 4:839–49. doi: 10.1016/S2215-0366(17)30371-1
65. Bergfeld IO, Mantione M, Hoogendoorn MLC, Ruhé HG, Notten P, Van Laarhoven J, et al. Deep brain stimulation of the ventral anterior limb of the internal capsule for treatment-resistant depression. JAMA Psychiatry. (2016) 73:456. doi: 10.1001/jamapsychiatry.2016.0152
66. McKetin R, Najman JM, Baker AL, Lubman DI, Dawe S, Ali R, et al. Evaluating the impact of community-based treatment options on methamphetamine use: findings from the M ethamphetamine T reatment E valuation S tudy (MATES). Addiction. (2012) 107:1998–2008. doi: 10.1111/j.1360-0443.2012.03933.x
67. Granziera C, Pollo C, Russmann H, Staedler C, Ghika J, Villemure J-G, et al. Sub-acute delayed failure of subthalamic DBS in Parkinson’s disease: the role of micro-lesion effect. Parkinsonism Related Disord. (2008) 14:109–13. doi: 10.1016/j.parkreldis.2007.06.013
68. Lázaro-Muñoz G, Pham MT, Muñoz KA, Kostick-Quenet K, Sanchez CE, Torgerson L, et al. Post-trial access in implanted neural device research: Device maintenance, abandonment, and cost. Brain Stimul. (2022) 15:1029–36. doi: 10.1016/j.brs.2022.07.051
69. Kaptchuk TJ, Goldman P, Stone DA, Stason WB. Do medical devices have enhanced placebo effects? J Clin Epidemiol. (2000) 53:786–92. doi: 10.1016/s0895-4356(00)00206-7
Keywords: substance use disorder, addiction, brain stimulation, neuromodulation, invasive, craving
Citation: Sakai JT, Tanabe J, Battula S, Zipperly M, Mikulich-Gilbertson SK, Kern DS, Thompson JA, Raymond K, Gerecht PD, Foster K and Abosch A (2024) Deep brain stimulation for the treatment of substance use disorders: a promising approach requiring caution. Front. Psychiatry 15:1435109. doi: 10.3389/fpsyt.2024.1435109
Received: 19 May 2024; Accepted: 25 June 2024;
Published: 12 July 2024.
Edited by:
James Mahoney, West Virginia University, United StatesReviewed by:
Pierre-François D’Haese, Vanderbilt University, United StatesCopyright © 2024 Sakai, Tanabe, Battula, Zipperly, Mikulich-Gilbertson, Kern, Thompson, Raymond, Gerecht, Foster and Abosch. This is an open-access article distributed under the terms of the Creative Commons Attribution License (CC BY). The use, distribution or reproduction in other forums is permitted, provided the original author(s) and the copyright owner(s) are credited and that the original publication in this journal is cited, in accordance with accepted academic practice. No use, distribution or reproduction is permitted which does not comply with these terms.
*Correspondence: Joseph T. Sakai, Joseph.sakai@cuanschutz.edu
†These authors share first authorship
‡ORCID: Joseph T. Sakai, orcid.org/0000-0003-1600-6100
Jody Tanabe, orcid.org/0000-0002-7110-8444