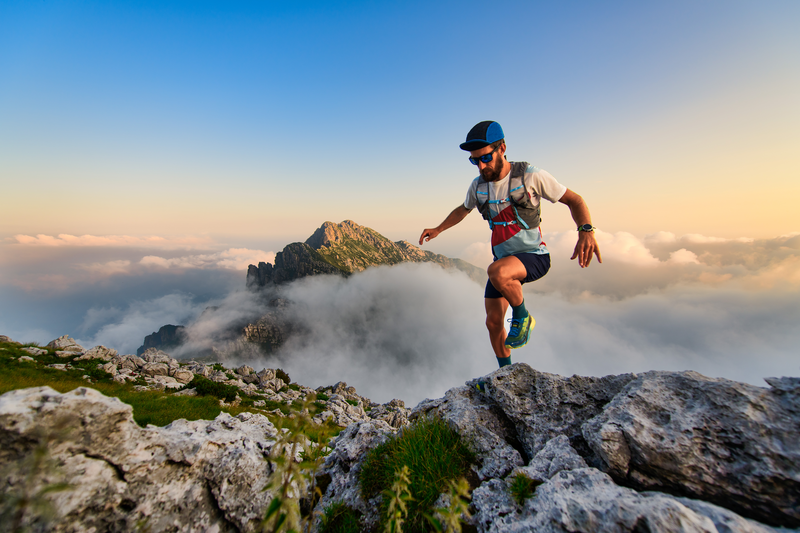
94% of researchers rate our articles as excellent or good
Learn more about the work of our research integrity team to safeguard the quality of each article we publish.
Find out more
MINI REVIEW article
Front. Psychiatry , 05 November 2024
Sec. Mood Disorders
Volume 15 - 2024 | https://doi.org/10.3389/fpsyt.2024.1421490
Major depressive disorder (MDD) and bipolar disorder (BD) are two of the most prevalent mood disorders that seriously jeopardize both physical and mental health. The current diagnosis of MDD and BD relies primarily on clinical symptoms. However, correctly differentiating between MDD and BD during depressive episode states remains a substantial clinical challenge. The human gut hosts a large and diverse microbiota, which plays a pivotal role in various physiological processes. Emerging evidence suggests that the gut microbiota (GM) exerts beneficial effects on mental health disorders, including MDD, BD, and schizophrenia, through the microbe-gut-brain axis (MGBA). In recent years, the relationship between GM and mood disorders has garnered considerable attention, leading to intensive research in this area. The MGBA is a bidirectional communication system between the gut and the brain. Growing evidence indicates that the brain can influence the GM, which in turn may modulate the brain through this axis. This review aims to explore the changes in the GM of patients with MDD and BD and evaluate the effects of different treatments on their GM, including medication, probiotic, prebiotic and synbiotic interventions, and fecal microbiota transplantation (FMT). By doing so, we seek to identify potential disease-specific biomarkers, improve differential diagnosis, and offer novel therapeutic avenues for these disorders.
Major depressive disorder (MDD) and bipolar disorder (BD) are two important subtypes of severe mood disorders that pose a significant global disease burden and economic stress. The 2010 Global Burden of Disease report on mental disorders revealed that MDD contributes 40.5% to the global burden of mental disorders, while BD accounts for 7.0% (1). MDD is characterized by distinct episodes lasting at least 2 weeks, involving disruptions across various domains of emotional, cognitive, and neurotrophic functioning (2). The lifetime prevalence of MDD is estimated at 10.8% (3). BD is characterized by recurrent episodes of elevated mood and depression accompanied by fluctuations in activity and energy, along with distinct cognitive, physical, and behavioral symptoms (4). The lifetime risk of BD is approximately 1% (5). Current treatment options for mood disorders include pharmacological interventions, such as antidepressants, and non-pharmacological interventions, including psychotherapy (6). However, each approach has several limitations, for example, drug therapy often proves ineffective and is associated with a high risk of relapse (7). Therefore, there is an urgent need to develop new therapeutic strategies to enhance treatment outcomes.
The gut microbiota (GM) is a dynamic and complex microbial ecosystem that includes bacteria, viruses, archaea, protozoa, and fungi (8). It communicates with the host and plays an important role in maintaining human health (9). The microbe-gut-brain axis (MGBA) represents the bidirectional communication pathway between the gut and the brain, primarily mediated through the immune system, the vagus nerve, and the endocrine system (8, 10). The gut microbiota influences brain function by modulating neurotransmitters and their precursors, as well as by secreting and upregulating key proteins and metabolites involved in the release of neuropeptides and gastrointestinal hormones, including short-chain fatty acids (SCFAs) and brain-derived neurotrophic factor (BDNF) (11).
Over the past decade, MGBA has garnered increasing attention and has been implicated in various disorders, including MDD and BD (12). Evidence suggests that patients with mood disorders exhibit alterations in GM composition (9, 13, 14), and these changes correlate with the severity of mood disorders (15).Conversely, psychobiotics, prebiotics, and fecal microbiota transplantation (FMT) have shown potential in the treatment of mood disorders by modulating the GM and enhancing MGBA function (16). In patients with MDD, dysregulation of the GM can disrupt bile acid metabolism, potentially triggering depressive symptoms through systemic inflammation (17). Thus, MGBA dysfunction may play an important role in the pathogenesis of mood disorders. Recently, there has been a growing interest in GM regulation as a therapeutic strategy for mood disorders. However, these treatments remain in the developmental stage, and pharmacological interventions remain the primary therapeutic approach. In addition, the etiological overlap and symptomatic similarities between MDD and BD complicate differential diagnosis, thereby delaying treatment and negatively affecting patient prognosis (18, 19).Despite emerging insights, little is known about the shared and distinct microbiological characteristics of MDD and BD. Therefore, this review aims to describe the specific GM alterations in patients with MDD and BD, providing insights into potential biomarkers for diagnosis and treatment. Moreover, the interactions between various treatment modalities and GM are explored to elucidate underlying mechanisms.
Alpha and beta diversity are widely utilized in sequencing-based microbiota studies to offer comprehensive insights into the structure and composition of microbial communities (20). Alpha diversity measures the diversity within a single sample’s GM and is commonly used to assess both the richness (abundance) and evenness (distribution) of unique taxa. Low alpha diversity is frequently associated with potential health risks for the host organism (20, 21). Beta diversity is calculated from pairwise measures of similarity or dissimilarity between GM in different groups and is used to evaluate compositional differences between samples, such as between patients and healthy controls (HCs) (20, 21). We then compared gut microbial diversity between patients with MDD and BD using both alpha and beta diversity metrics.
Findings on alpha and beta diversity in patients with MDD have been mixed. Overall, several studies have reported no significant differences in alpha diversity between people with MDD and HCs (2, 21–27). However, some studies have reported decreased alpha diversity in patients with MDD (28–30). In addition, using the Shannon index, Jiang et al. and Ye et al. observed increased alpha diversity in patients with MDD (31, 32). Unlike alpha diversity, most studies have shown that beta diversity in patients with MDD differs significantly from that of HCs (2, 21, 24, 28, 33). However, similar to alpha diversity, some studies have reported conflicting results regarding beta diversity in individuals with MDD. In a study of 160 adolescent participants, no significant differences in beta diversity were observed among MDD patients, suggesting that age may influence beta diversity in MDD (34).
In patients with BD, alpha diversity is reduced compared to healthy individuals, and the extent of this reduction is positively associated with the duration of the disease (35). This reduction may be attributed to the inflammatory processes associated with BD, leading to progressive neurobiological and functional deterioration, or to the chronic use of psychotropic medications and potential malnutrition (36, 37). In contrast to MDD, no significant differences in beta diversity were observed in patients with BD compared to HCs (38).
In summary, compared to HCs, patients with MDD exhibited increased beta diversity, while those with BD showed decreased alpha diversity. A comparative study of MDD and BD yielded results consistent with those of studies comparing both groups to HCs (38, 39). These findings suggest a potential avenue for distinguishing MDD from BD during episodes of depressive symptoms. However, given the inconsistency of results across studies, this conclusion remains a preliminary diagnostic indicator. More reliable biomarkers are needed for a more accurate and precise diagnosis.
Most studies have shown that the GM of patients with MDD differs from that of healthy individuals at the phylum, class, order, family, and genus levels, with particular emphasis on differences at the phylum, family, and genus levels (Table 1).
In the 14 included studies, we found that the changes at the phylum level in patients with MDD primarily involved Actinobacteria, Bacteroidetes, and Firmicutes. Although some studies reported opposite findings, the overall trend indicated an increase in Actinobacteria and Bacteroidetes levels, along with a decrease in Firmicutes levels (25, 31, 41). These variations may be influenced by the age of patients with MDD. Compared to age-matched HCs, younger patients showed elevated Bacteroidetes and reduced Firmicutes, while middle-aged patients exhibited the opposite pattern. Notably, Actinobacteria levels consistently increased, independent of age (47).
At the family level, patients with MDD exhibited decreased levels of Lachnospiraceae and Ruminococcaceae, along with increased levels of Bifidobacteriaceae (24, 27, 31, 39, 41). It has been hypothesized that since most of the patients in the study were in the early stages of MDD, the elevation of Bifidobacteria may be a protective response strategy of the GM, which enhances intestinal barrier function, anti-inflammatory, and immunomodulatory effects, as well as promotes gamma-aminobutyric acid (GABA) production (41, 48).
At the genus level, we observed trends consistent with those at the phylum and family levels: a consistent decrease in the levels of Firmicutes-Ruminococcaceae–Ruminococcus and Firmicutes-Lachnospiraceae-Blautia, along with a consistent increase in Actinobacteria-Bifidobacteriaceae-Bifidobacterium (25, 28, 43). Notably, Actinobacteria-Bifidobacteriaceae-Bifidobacterium demonstrated highly concordant elevated levels across studies, which is rare among other genera. Furthermore, a study examining the GM of subgroups with varying severities of MDD revealed distinct GM phenotypes in patients with moderate and severe MDD. The phylum Actinobacteria emerged as a covariant marker, with the genera Collinsella, Eggerthella, Alistipe, Faecaliba, and Framonifractor suggested as potential diagnostic indicators for MDD (15). However, it is important to note that Bifidobacterium counts can increase with higher plant-based protein intake (49). The elevation of the phylum Bacteroidetes is primarily attributed to the combined effects of increased levels of Bacteroidetes-Bacteroidaceae-Bacteroides and decreased levels of Bacteroidetes-Prevotellaceae-Prevotella (25, 31, 46). Elevated levels of Bacteroides are associated with cytokine production, aligning with findings of upregulation of pro-inflammatory bacteria and downregulation of anti-inflammatory bacteria in MDD patients, thus providing support for the inflammatory hypothesis of depression (44, 50). Reduced levels of Prevotella are associated with the development of autism in young patients (46). Klebsiella, a gram-negative bacterium, may play a significant role in the pathophysiology of MDD through its translocation and the immune responses to lipopolysaccharide (LPS) (51). In the study by Lin et al., the changes in the relative proportions of Prevotella and Klebsiella in the fecal flora were expected to be a valuable indicator for laboratory diagnosis and prognostic assessment of patients with MDD (46). Another study showed that individuals with MDD had elevated levels of Bilophila and Alistipes compared to HCs. The LPS present on the membranes of Bilophila and Alistipes can induce depressive symptoms through the activation of toll-like receptor 4. Additionally, Alistipes may influence the production of indole, which affects tryptophan metabolism and disrupts the homeostasis of the 5-hydroxytryptaminergic system (52). Indole derivatives are known neuroinhibitory molecules, and indoles along with their derivatives participate in “MGBA” mediated metabolic, immune, and neural communication processes by binding to the aryl hydrocarbon receptor, contributing to the onset of MDD (53). However, due to the limited literature included in this study, it is not yet possible to draw definitive conclusions about the specific changes in Alistipes. Studies have shown that an increase in Blautia abundance is accompanied by an increase in serum C-reactiveprotein levels. Therefore, Blautia may cause MDD by promoting inflammation (54).
Studies have shown that the relative abundance of the Actinobacteria phylum and its associated families is increased in patients with BD. Both Actinobacteria and Coriolobacteriaceae are involved in lipid metabolism and correlate with cholesterol levels, which may contribute to obesity in patients with BD (36). Research has found that the microbiota of patients with BD and higher Body Mass Index (BMI) harbored a significantly greater quantity of Lactobacilli than the group with lower BMI. Additionally, the family Lactobacillaceae and the genus Lactobacillus were more abundant in BD patients with metabolic syndrome, suggesting that Lactobacilli may also play a role in obesity among these patients (8). In addition, BMI is positively correlated with Roseburia abundance in patients with BD (14). Ruminococcaceae have been reported to be associated with energy metabolism pathways, including gluconeogenesis, glycolysis, and pentose phosphate pathways. Therefore, lower levels of Ruminococcaceae in patients with BD may be linked to abnormal glucose metabolism (14).
Untreated patients with BD have been found to exhibit downregulated levels of various butyrate-producing bacteria compared to HCs, including Roseburia, Faecalibacterium, and Coprococcus. These bacteria are responsible for producing SCFAs, such as butyrate, which can influence CNS function. In particular, butyrate has been shown to affect hippocampal function and promote the expression of BDNF, a protein associated with antidepressant-like effects in animal models. Therefore, a deficiency in butyrate-producing bacteria may be linked to the development of BD (14).
Faecalibacterium is a prevalent intestinal gram-positive microorganism known for its anti-inflammatory properties (55). In patients with BD, a decrease in Faecalibacterium levels appears to be associated with disease severity, psychotic symptoms, and altered sleep quality. Furthermore, Faecalibacterium shows a correlation with self-reported symptoms and disease severity (56); therefore, it may be possible to differentiate patients with BD from HCs based on Faecalibacterium levels (47). Enterobacter spp. have also been found to positively correlate with serum interleukin-6 (IL-6) levels, and BD is strongly associated with immune dysfunction. Consequently, Enterobacter spp. may contribute to the pathogenesis of BD through mechanisms related to immune dysfunction (57) (Table 2).
Enterobacteriaceae levels have been found to be elevated in both MDD and BD (60), with an inflammatory state in the GM facilitating the proliferation of Enterobacteriaceae (61). Some studies have reported that the family Lactobacillaceae and members of the genus Lactobacillus are enriched in MDD and BD. In the authors’ exploratory analysis, Lactobacillaceae levels were significantly increased only in the medication group, suggesting that the use of psychotropic drugs may contribute to the elevation of this family and its member genus in patients (38).
At the genus level, both MDD and BD exhibited relatively increased levels of Flavonifractor, a genus of bacteria involved in the breakdown of quercetin, a flavonoid known for its antioxidant and anti-inflammatory properties, which has been shown to have depression-relieving effects (8, 62). However, Flavonifractor may also induce oxidative stress and inflammation in its host (59), suggesting that increased levels could contribute to the inflammation associated with depression. Additionally, Clostridium levels were found to be elevated in both conditions. Adults with depression and those on specific antidepressants are at a higher risk of developing Clostridium difficile infections (63).
Depletion of anti-inflammatory butyrate-producing bacteria and enrichment of pro-inflammatory bacteria, lower levels of SCFA-producing bacterial genera, higher levels of lactate-producing bacteria, and higher levels of bacteria associated with glutamate and GABA metabolism were found in both diseases (21, 60). SCFAs exert anti-inflammatory effects by interfering with the NF-κB pathway, and a reduction in SCFA-producing bacteria may cause MDD and BD via an inflammatory response (54). They may be considered biomarkers to improve diagnostic accuracy, guide treatment, and help monitor the response to therapy. However, further studies are needed to determine its feasibility.
Interestingly, some taxa are differentiated between MDD and BD. Bacteroidaceae, Veillonellaceae, and Roseburia are higher in MDD than in BD, while Enterobacteriaceae, Pseudomonadaceae, and Megasphaera are higher in BD than in MDD (21, 39). In a study exploring GM differences among MDD, bipolar disorder with the current major depressive episode (BPD) and HCs, the genera Escherichia and Klebsiella showed changes in abundance only between the BPD and HC groups. At the species level, compared with BPD patients, MDD patients had a higher abundance of Prevotellaceae including Prevotella denticola F0289, Prevotella intermedia 17, Prevotella ruminicola, and Prevotella intermedia. Furthermore, the abundance of Fusobacteriaceae, Escherichia blattae DSM 4481 and Klebsiella oxytoca were significantly increased, whereas the Bifidobacterium longum subsp. infantis ATCC 15697 = JCM 1222 was significantly reduced in the BPD group compared with MDD group (64). MDD is usually characterized by higher Alistipes and Parabacteroides and lower Prevotella; BD is usually characterized by higher Bifidobacterium and Oscillibacter (21).
Pharmacological interventions remain the cornerstone of depression treatment. Antidepressants are broadly categorized into selective serotonin reuptake inhibitors (SSRIs), serotonin-norepinephrine reuptake inhibitors (SNRIs), tricyclic and tetracyclic antidepressants, atypical antidepressants, monoamine oxidase inhibitors, N-methyl-D-aspartate (NMDA) antagonists, and neuroactive steroids, such as GABA-A receptor positive modulators. Among these, second-generation antidepressants, particularly SNRIs, are the most frequently prescribed (65, 66). These antidepressants not only alleviate depression through their respective mechanisms of action but also significantly impact the GM (50, 67, 68). Antidepressants reduce the gut bacterial abundance and increase beta diversity, with significant reductions in the abundance of Ruminococcus, Adlercreutzia, and the unclassified genus Alphaproteobacteria in particular (69). In patients with MDD treated with escitalopram, alpha diversity of the gut microbiota decreased to levels comparable to healthy controls. In addition, the abundance of Christensenellaceae_R-7_group, [Eubacterium]_ruminantium_group, and Fusobacterium was significantly elevated, while Lactobacillus abundance, as well as the Firmicutes/Bacteroidetes ratio, were significantly reduced compared to pre-treatment levels and healthy controls. Interestingly, higher pre-treatment levels of Firmicutes were positively associated with treatment response (70, 71). Duloxetine, an SNRI, exerts its antidepressant effects primarily through modulation of gene expression in the cortex. Specifically, it inhibits the upregulation of mitochondrial oxidative phosphorylation genes and downregulates genes related to neuronal plasticity. This action is closely associated with the downregulation of Ruminococcus abundance (69). In addition, the relative abundance of Blautia, Bifidobacterium, and Coprococcus has been positively correlated with the antidepressant efficacy of SSRIs (72).
Current pharmacological treatments for BD include lithium, atypical antipsychotics (AAP), and antiepileptic drugs. Lithium, considered the first-line treatment for BD, has been shown to increase the GM species richness and diversity, with significant increases in the relative abundance of Clostridium spp., Clostridium perfringens, Enterobacter spp., and Christenellaceae spp. Atypical antipsychotics, such as aripiprazole, quetiapine, and olanzapine, are widely used in the management of acute manic and depressive episodes, as well as for the maintenance therapy in BD. In a study involving aripiprazole administration in rats, an increase in the relative abundance of Clostridium spp., Clostridium tumefaciens, Enterobacter spp., and Eubacterium faecalis was observed in the gut following treatment (8). In addition, another study demonstrated that olanzapine reduced the Mycobacterium avium/Hypobacterium chauvinum ratio, contributing to increased appetite, visceral fat accumulation, and peripheral inflammation, all of which are risk factors for obesity (8). Similarly, reduced gut biodiversity in BD patients following quetiapine treatment, especially the diminished relative abundance of Ackermannia and Suturella associated with normal metabolism—has been linked to APP-induced obesity and metabolic complications (8, 55, 73). Antiepileptic drugs, including valproic acid, lamotrigine, and carbamazepine, are frequently used to stabilize mood in BD. In one trial, valproic acid was associated with increased abundance of Clostridium spp., Clostridium perfringens, Enterobacter spp., and Christenellaceae spp. in the rat cecum (74). Additionally, a vitro study found that lamotrigine significantly inhibited the growth of Gram-positive bacteria, such as Bacillus subtilis and Staphylococcus aureus (55). However, the relationship between microbiological alterations induced by antiepileptic drugs and therapeutic efficacy in BD remains to be further elucidated.
Ketamine, an NMDA receptor antagonist, has shown potent efficacy in the treatment of BD and in antidepressant-naïve patients with MDD (75). It has been shown that ketamine modulates the dysbiotic composition of the GM by elevating the abundance of Actinobacteriaceae and Coriolobacteriaceae Piliobacteriaceae, Lactobacillus, Turicibacter, and Sarcina, while decreasing levels of Fusobacterium, Clostridium and Ruminalococcaceae, Clostridium and Butyric acidomonas spp. These changes in the GM are associated with improvements in depressive symptoms (75–78).
Collectively, these studies suggest that pharmacological treatments can significantly alter the abundance and composition of the GM. Moreover, the composition and abundance of gut microbes appear to affect the efficacy of pharmacological treatments and are closely related to certain adverse effects, such as metabolic complications (79).
Although pharmacotherapy is effective in treating MDD and BD, it’s often accompanied by withdrawal symptoms, gastrointestinal adverse reactions, and even life-threatening side effects (80). In addition, between 1/3 to 1/2 of MDD patients do not respond to multiple antidepressants (81). In contrast, non-pharmacological interventions with significantly fewer side effects, such as electroconvulsive therapy, psychosocial interventions, cognitive-behavioral therapy, and dietary-dietary fiber or probiotic therapies, are receiving increasing attention and research.
Probiotics, prebiotics, synbiotics, and postbiotics exert a potent regulatory effect on the GM (82). Studies have demonstrated that administration of probiotic powder containing Lacticaseibacillus paracasei strain Shirota (LcS) to patients with MDD or BD improves depressive symptoms. This improvement is positively correlated with an increased abundance of Actinobacteriophage and Bifidobacterium in the GM (83). Probiotic supplementation with strains such as Lactobacillus and Bifidobacterium bifidum has been shown to elevate serotonin levels and reduce both depression and rumination scores in patients with BD, as well as decrease rehospitalization rates, duration of hospital, and mania scores in BD patients with manic episodes (84). Moreover, Lactobacilli or bifidobacteria produce significant amounts of lactate and/or acetate, which are subsequently metabolized to butyrate by butyrate-producing bacteria such as Clostridium butyricum and E.faecalis przewalskii. This process enhances brain-derived neurotrophic factor (BDNF) levels, promotes neurogenesis, and ultimately alleviates depressive symptoms in MDD (83). In addition, Lactobacillus can convert sugar-derived carbon sources into tryptophan, producing indole and its derivatives, which promote hippocampal neurogenesis and reduce depressive symptoms (85). Probiotic intake has also been shown to downregulate systemic pro-inflammatory cytokines such as interleukin-1beta, tumor necrosis factor-alpha, IL-6, and interferon-gamma (86). An 8-week intervention with Lactobacillus plantarum PS128 in MDD patients found that improvements in depressive symptoms were accompanied by an increase in the relative abundance of Akkermansia, a bacterium closely related to IL-6 and suppressed inflammation (87, 88). Akkermansia may further alleviate depression by modulating the GM composition and metabolites, which in turn upregulate molecules associated with pathological changes in depression (corticosterone, dopamine, and BDNF) as well as antidepressant markers (β-alanyl-3-methyl-L-histidine and edaravone) (89). In addition, Lactobacillus testosteroni La 1 or LcS activates gastric vagal afferents, leading to inhibition of the hypothalamic-pituitary-adrenal (HPA) axis and subsequent reduction of renal sympathetic nerve activity. Meanwhile, Lactobacillus short left SBC 8803 promotes small intestinal 5-hydroxytryptamine secretion, thereby activating the intestinal branch of vagal afferents. Collectively, these findings suggest that probiotic strains modulate the composition and metabolites of the intestinal microflora, promoting neurogenesis, inhibiting inflammatory responses, and modulating the neuroendocrine system to downregulate the stress-induced activation of the HPA axis, thereby controlling depressive symptoms (83) (Figure 1).
Figure 1. Mechanism of Lactobacillus treating MDD and BD. Trp, Tryptophan; HPA, hypothalamic-pituitary-adrenal; C3H5O3-/C2H3O2-, Lactate/acetate; C4H8O2-, Butyrate; BDNF, brain-derived neurotrophic factor; IL-6, Interleukin-6.
Elevated activity in the tryptophan metabolic pathway within the circulating microbiome of non-responders to antidepressant therapy has been associated with reduced availability of tryptophan, the sole precursor for serotonin synthesis. This reduction consequently impairs serotonin production (71). A prospective open trial suggested that Clostridium butyricum MIYAIRI 588 in combination with antidepressants is effective in improving depressive symptoms in patients with antidepressant-resistant MDD (90). Based on the role of Lactobacillus in increasing tryptophan levels and promoting neurogenesis, we hypothesize that a combination of Lactobacillus and antidepressants may offer more pronounced improvements in depressive symptoms for patients with antidepressant-resistant MDD than the combination with Clostridium butyricum. In another clinical trial, a probiotic/magnesium spirulina formula (comprising Lactobacillus acidophilus, Bifidobacterium bifidum, Streptococcus thermophilus) used as an adjunct to SSRI intervention in patients with drug-resistant MDD led to significant improvements in depressive symptoms and quality of life, whereas after discontinuing the probiotic adjuvant, patients’ depressive symptoms recurred (91). These findings suggest that, in addition to ameliorating depressive symptoms via multiple pathways, probiotics may serve as a potent adjunctive agent in improving antidepressant drug resistance and inhibiting the recurrence of depression.
FMT is considered a generally safe treatment with minimal adverse effects, which is achieved by transferring feces from a healthy donor to a patient with GM disorders to directly restore the GM composition of the recipient (92, 93). FMT has demonstrated efficacy in the treatment of recurrent Clostridium difficile infections and has also shown therapeutic potential in several diseases associated with intestinal microbiota dysregulation, such as ulcerative colitis, irritable bowel syndrome, and hepatic encephalopathy (94). Given the crucial role of the MGBA in regulating mood, behavior, and cognition, and the involvement of GM disturbances in the pathogenesis of depression, FMT holds promise as a potential therapy for depression (95). Moreover, FMT in patients with MDD or BD can induce depressive-like symptoms. It has been shown that FMT from MDD patients or rodents with depressive-like behaviors can induce similar behaviors in recipient rodents through systemic inflammation. Notably, severing the vagus nerve prevents this effect, as well as the antidepressant effects of SSRIs (96). Another study showed that activation of NACHT, LRR and PYD structural domain protein 3 inflammatory vesicles led to a decrease in Trichoderma, Ruminalococcaceae, and Prevotella, while promoting an enrichment of Mycobacterium anisopliae, resulting in depression-like symptoms (97). These results suggest that the MGBA may exert bidirectional effects and participate in the onset and progression of depression. In a clinical study, oral administration of FMT capsules to depression patients with irritable bowel syndrome resulted in a significant increase in bacterial alpha diversity and the abundance of bacterial communities, predominantly Bacteroides immitis and Bacteroides thicketi, alongside significant improvements in depressive symptoms (95). In addition, spinal cord injured depressed rats showed a significant reduction in depressive and anxiety-like behaviors following FMT from healthy rats (97). These results indicate a potential positive role for FMT in the treatment of depression; however, there remains a notable lack of research on its effects in patients with MDD or BD.
MDD and BD are significant contributors to the global disease burden, often resulting in severe cognitive impairment that substantially affects patients’ social functioning and quality of life (97). Gut microbes can influence brain function through neural, immune, and metabolic pathways, either directly via the vagus nerve or indirectly via gut- and microbial-derived metabolites, as well as gut hormones and endocrine peptides, and disruptions in their composition are strongly associated with the development of depressive behaviors (98). This review explores the altered composition of gut microorganisms in patients with MDD and BD, aiming to identify specific microbial signatures that could serve as therapeutic targets for probiotic and FMT (33, 99). Furthermore, the positive effects of pharmacological treatment, probiotic interventions, and FMT in restoring the composition of the gut microflora and thus improving depressive symptoms, as well as possible mechanisms, are elucidated.
Participants with MDD exhibit altered beta diversity, while those with BD show reduced alpha diversity, which may serve as a distinguishing feature between the two. The specific alterations in gut flora as potential biomarkers need to be further investigated. Additionally, the role of gut microbiota-derived metabolites represents a promising avenue for future research, offering new insights into pathogenic mechanisms and potential therapeutic strategies.
Antidepressant non-responders demonstrate a reduced abundance of Firmicutes and elevated levels of tryptophan metabolism (71). Meanwhile, butyrate-producing bacteria have shown a positive effect in the treatment of antidepressants non-responders, possibly due to their role in promoting of neurogenesis (33). The multifaceted effects of Lactobacillus in enhancing neurogenesis and increasing tryptophan levels suggest that it may be a more effective adjunctive treatment for individuals who do not respond to antidepressants. However, this hypothesis requires further investigation to be validated. Improving the abnormal composition of the microflora and modulating the microflora network to down-regulate tryptophan metabolism is a possible direction to fundamentally improve antidepressant resistance. Although conventional probiotic treatments, primarily composed of Bifidobacterium spp. and Lactobacillus spp., are generally considered safe, their efficacy in fully correcting microbiota dysbiosis remains suboptimal (99). FMT offers a more direct approach to restoring the disturbed microbial composition, and the similarity between the recipient’s and donor’s microbiota after transplantation is positively correlated with therapeutic outcomes. However, the evidence supporting FMT in patients with MDD and BD remains limited (95). Given the vast complexity of the human microbiota network and the current lack of comprehensive studies, further research is urgently needed to advance our understanding of microbiota regulation as a therapeutic strategy.
JZ: Writing – review & editing, Writing – original draft. JL: Writing – review & editing, Writing – original draft. JF: Writing – review & editing. XL: Writing – review & editing. QH: Writing – review & editing.
The author(s) declare financial support was received for the research, authorship, and/or publication of this article. This work was supported by the National Undergraduate Training Programs for Innovation and Entrepreneurship (No. 202310632005) and the Joint Foundation of Xuyong County People’s Hospital and Southwest Medical University (No. 2023XYXNYD13).
The authors declare that the research was conducted in the absence of any commercial or financial relationships that could be construed as a potential conflict of interest.
All claims expressed in this article are solely those of the authors and do not necessarily represent those of their affiliated organizations, or those of the publisher, the editors and the reviewers. Any product that may be evaluated in this article, or claim that may be made by its manufacturer, is not guaranteed or endorsed by the publisher.
1. Whiteford HA, Degenhardt L, Rehm J, Baxter AJ, Ferrari AJ, Erskine HE, et al. Global burden of disease attributable to mental and substance use disorders: findings from the global burden of disease study 2010. Lancet. (2013) 382:1575–86. doi: 10.1016/S0140-6736(13)61611-6
2. Alli SR, Gorbovskaya I, Liu JCW, Kolla NJ, Brown L, Muller DJ. The gut microbiome in depression and potential benefit of prebiotics, probiotics and synbiotics: A systematic review of clinical trials and observational studies. Int J Mol Sci. (2022) 23:4494. doi: 10.3390/ijms23094494
3. Lim GY, Tam WW, Lu Y, Ho CS, Zhang MW, Ho RC. Prevalence of depression in the community from 30 countries between 1994 and 2014. Sci Rep. (2018) 8:2861. doi: 10.1038/s41598-018-21243-x
5. Merikangas KR, Jin R, He JP, Kessler RC, Lee S, Sampson NA, et al. Prevalence and correlates of bipolar spectrum disorder in the world mental health survey initiative. Arch Gen Psychiatry. (2011) 68:241–51. doi: 10.1001/archgenpsychiatry.2011.12
6. Chen C, Shan W. Pharmacological and non-pharmacological treatments for major depressive disorder in adults: A systematic review and network meta-analysis. Psychiatry Res. (2019) 281:112595. doi: 10.1016/j.psychres.2019.112595
7. Mrozek W, Socha J, Sidorowicz K, Skrok A, Syrytczyk A, Piatkowska-Chmiel I, et al. Pathogenesis and treatment of depression: role of diet in prevention and therapy. Nutrition. (2023) 115:112143. doi: 10.1016/j.nut.2023.112143
8. Lucidi L, Pettorruso M, Vellante F, Di Carlo F, Ceci F, Santovito MC, et al. Gut microbiota and bipolar disorder: an overview on a novel biomarker for diagnosis and treatment. Int J Mol Sci. (2021) 22:3723. doi: 10.3390/ijms22073723
9. Du Y, Gao XR, Peng L, Ge JF. Crosstalk between the microbiota-gut-brain axis and depression. Heliyon. (2020) 6:e04097. doi: 10.1016/j.heliyon.2020.e04097
10. Radford-Smith DE, Anthony DC. Prebiotic and probiotic modulation of the microbiota-gut-brain axis in depression. Nutrients. (2023) 15:1880. doi: 10.3390/nu15081880
11. Simpson CA, Diaz-Arteche C, Eliby D, Schwartz OS, Simmons JG, Cowan CSM. The gut microbiota in anxiety and depression - a systematic review. Clin Psychol Rev. (2021) 83:101943. doi: 10.1016/j.cpr.2020.101943
12. Cryan JF, O’Riordan KJ, Cowan CSM, Sandhu KV, Bastiaanssen TFS, Boehme M, et al. The microbiota-gut-brain axis. Physiol Rev. (2019) 99:1877–2013. doi: 10.1152/physrev.00018.2018
13. Cruz-Pereira JS, Rea K, Nolan YM, O’Leary OF, Dinan TG, Cryan JF. Depression’s unholy trinity: dysregulated stress, immunity, and the microbiome. Annu Rev Psychol. (2020) 71:49–78. doi: 10.1146/annurev-psych-122216-011613
14. Hu S, Li A, Huang T, Lai J, Li J, Sublette ME, et al. Gut microbiota changes in patients with bipolar depression. Adv Sci (Weinh). (2019) 6:1900752. doi: 10.1002/advs.201900752
15. Zhong Q, Chen JJ, Wang Y, Shao WH, Zhou CJ, Xie P. Differential gut microbiota compositions related with the severity of major depressive disorder. Front Cell Infect Microbiol. (2022) 12:907239. doi: 10.3389/fcimb.2022.907239
16. Liang S, Wu X, Hu X, Wang T, Jin F. Recognizing depression from the microbiota(-)Gut(-)Brain axis. Int J Mol Sci. (2018) 19:1592. doi: 10.3390/ijms19061592
17. Hashimoto K. Gut-microbiota-brain axis by bile acids in depression. Psychiatry Clin Neurosci. (2022) 76:281. doi: 10.1111/pcn.13370
18. Zhang C, Rong H. Genetic advance in depressive disorder. Adv Exp Med Biol. (2019) 1180:19–57. doi: 10.1007/978-981-32-9271-0_2
19. Hashimoto K. Metabolomics of major depressive disorder and bipolar disorder: overview and future perspective. Adv Clin Chem. (2018) 84:81–99. doi: 10.1016/bs.acc.2017.12.005
20. Wei S, Bahl MI, Baunwall SMD, Hvas CL, Licht TR. Determining gut microbial dysbiosis: A review of applied indexes for assessment of intestinal microbiota imbalances. Appl Environ Microbiol. (2021) 87:e00395–21. doi: 10.1128/AEM.00395-21
21. McGuinness AJ, Davis JA, Dawson SL, Loughman A, Collier F, O’Hely M, et al. A systematic review of gut microbiota composition in observational studies of major depressive disorder, bipolar disorder and schizophrenia. Mol Psychiatry. (2022) 27:1920–35. doi: 10.1038/s41380-022-01456-3
22. Simpson CA, Schwartz OS, Simmons JG. The human gut microbiota and depression: widely reviewed, yet poorly understood. J Affect Disord. (2020) 274:73–5. doi: 10.1016/j.jad.2020.05.115
23. Knudsen JK, Bundgaard-Nielsen C, Leutscher P, Hjerrild S, Nielsen RE, Sorensen S. Differences in bacterial taxa between treatment-naive patients with major depressive disorder and non-affected controls may be related to a proinflammatory profile. BMC Psychiatry. (2024) 24:84. doi: 10.1186/s12888-024-05547-z
24. Chung YE, Chen HC, Chou HL, Chen IM, Lee MS, Chuang LC, et al. Exploration of microbiota targets for major depressive disorder and mood related traits. J Psychiatr Res. (2019) 111:74–82. doi: 10.1016/j.jpsychires.2019.01.016
25. Liu RT, Rowan-Nash AD, Sheehan AE, Walsh RFL, Sanzari CM, Korry BJ, et al. Reductions in anti-inflammatory gut bacteria are associated with depression in a sample of young adults. Brain Behav Immun. (2020) 88:308–24. doi: 10.1016/j.bbi.2020.03.026
26. Ritchie G, Strodl E, Parham S, Bambling M, Cramb S, Vitetta L. An exploratory study of the gut microbiota in major depression with anxious distress. J Affect Disord. (2023) 320:595–604. doi: 10.1016/j.jad.2022.10.001
27. Naseribafrouei A, Hestad K, Avershina E, Sekelja M, Linlokken A, Wilson R, et al. Correlation between the human fecal microbiota and depression. Neurogastroenterol Motil. (2014) 26:1155–62. doi: 10.1111/nmo.12378
28. Knudsen JK, Bundgaard-Nielsen C, Hjerrild S, Nielsen RE, Leutscher P, Sorensen S. Gut microbiota variations in patients diagnosed with major depressive disorder-a systematic review. Brain Behav. (2021) 11:e02177. doi: 10.1002/brb3.2177
29. Kelly JR, Borre YC, O' Brien C, Patterson E, El Aidy S, Deane J, et al. Transferring the blues: depression-associated gut microbiota induces neurobehavioural changes in the rat. J Psychiatr Res. (2016) 82:109–18. doi: 10.1016/j.jpsychires.2016.07.019
30. Sun N, Zhang J, Wang J, Liu Z, Wang X, Kang P, et al. Abnormal gut microbiota and bile acids in patients with first-episode major depressive disorder and correlation analysis. Psychiatry Clin Neurosci. (2022) 76:321–8. doi: 10.1111/pcn.13368
31. Jiang H, Ling Z, Zhang Y, Mao H, Ma Z, Yin Y, et al. Altered fecal microbiota composition in patients with major depressive disorder. Brain Behav Immun. (2015) 48:186–94. doi: 10.1016/j.bbi.2015.03.016
32. Ye X, Wang D, Zhu H, Wang D, Li J, Tang Y, et al. Gut microbiota changes in patients with major depressive disorder treated with vortioxetine. Front Psychiatry. (2021) 12:641491. doi: 10.3389/fpsyt.2021.641491
33. Lai WT, Zhao J, Xu SX, Deng WF, Xu D, Wang MB, et al. Shotgun metagenomics reveals both taxonomic and tryptophan pathway differences of gut microbiota in bipolar disorder with current major depressive episode patients. J Affect Disord. (2021) 278:311–9. doi: 10.1016/j.jad.2020.09.010
34. Thapa S, Sheu JC, Venkatachalam A, Runge JK, Luna RA, Calarge CA. Gut microbiome in adolescent depression. J Affect Disord. (2021) 292:500–7. doi: 10.1016/j.jad.2021.05.107
35. Sublette ME, Cheung S, Lieberman E, Hu S, Mann JJ, Uhlemann AC, et al. Bipolar disorder and the gut microbiome: A systematic review. Bipolar Disord. (2021) 23:544–64. doi: 10.1111/bdi.13049
36. Painold A, Morkl S, Kashofer K, Halwachs B, Dalkner N, Bengesser S, et al. A step ahead: exploring the gut microbiota in inpatients with bipolar disorder during a depressive episode. Bipolar Disord. (2019) 21:40–9. doi: 10.1111/bdi.12682
37. Dai W, Liu J, Qiu Y, Teng Z, Li S, Yuan H, et al. Gut microbial dysbiosis and cognitive impairment in bipolar disorder: current evidence. Front Pharmacol. (2022) 13:893567. doi: 10.3389/fphar.2022.893567
38. Nikolova VL, Smith MRB, Hall LJ, Cleare AJ, Stone JM, Young AH. Perturbations in gut microbiota composition in psychiatric disorders: A review and meta-analysis. JAMA Psychiatry. (2021) 78:1343–54. doi: 10.1001/jamapsychiatry.2021.2573
39. Zheng P, Yang J, Li Y, Wu J, Liang W, Yin B, et al. Gut microbial signatures can discriminate unipolar from bipolar depression. Adv Sci (Weinh). (2020) 7:1902862. doi: 10.1002/advs.201902862
40. Xiao S, Yang Z, Yan H, Chen G, Zhong S, Chen P, et al. Gut proinflammatory bacteria is associated with abnormal functional connectivity of hippocampus in unmedicated patients with major depressive disorder. Transl Psychiatry. (2024) 14:292. doi: 10.1038/s41398-024-03012-9
41. Dong Z, Shen X, Hao Y, Li J, Xu H, Yin L, et al. Gut microbiome: A potential indicator for predicting treatment outcomes in major depressive disorder. Front Neurosci. (2022) 16:813075. doi: 10.3389/fnins.2022.813075
42. Zhang Q, Yun Y, An H, Zhao W, Ma T, Wang Z, et al. Gut microbiome composition associated with major depressive disorder and sleep quality. Front Psychiatry. (2021) 12:645045. doi: 10.3389/fpsyt.2021.645045
43. Chen YH, Xue F, Yu SF, Li XS, Liu L, Jia YY, et al. Gut microbiota dysbiosis in depressed women: the association of symptom severity and microbiota function. J Affect Disord. (2021) 282:239–400. doi: 10.1016/j.jad.2020.12.143
44. Yang J, Zheng P, Li Y, Wu J, Tan X, Zhou J, et al. Landscapes of bacterial and metabolic signatures and their interaction in major depressive disorders. Sci Adv. (2020) 6:525–51. doi: 10.1126/sciadv.aba8555
45. Huang Y, Shi X, Li Z, Shen Y, Shi X, Wang L, et al. Possible association of Firmicutes in the gut microbiota of patients with major depressive disorder. Neuropsychiatr Dis Treat. (2018) 14:3329-37. doi: 10.2147/NDT.S188340
46. Lin P, Ding B, Feng C, Yin S, Zhang T, Qi X, et al. Prevotella and klebsiella proportions in fecal microbial communities are potential characteristic parameters for patients with major depressive disorder. J Affect Disord. (2017) 207:300–4. doi: 10.1016/j.jad.2016.09.051
47. Knuesel T, Mohajeri MH. The role of the gut microbiota in the development and progression of major depressive and bipolar disorder. Nutrients. (2021) 14:1303405. doi: 10.3390/nu14010037
48. Leser T, Baker A. Bifidobacterium adolescentis - a beneficial microbe. Benef Microbes. (2023) 14:525–51. doi: 10.1163/18762891-20230030
49. O’Neill S, Minehan M, Knight-Agarwal CR, Pyne DB. Alterations in gut microbiota caused by major depressive disorder or a low fodmap diet and where they overlap. Front Nutr. (2023) 10:1303405. doi: 10.3389/fnut.2023.1303405
50. Liu L, Wang H, Chen X, Zhang Y, Zhang H, Xie P. Gut microbiota and its metabolites in depression: from pathogenesis to treatment. EBioMedicine. (2023) 90:104527. doi: 10.1016/j.ebiom.2023.104527
51. Maes M, Kubera M, Leunis JC. The gut-brain barrier in major depression: intestinal mucosal dysfunction with an increased translocation of lps from gram negative enterobacteria (Leaky gut) plays a role in the inflammatory pathophysiology of depression. Neuro Endocrinol Lett. (2008) 29:117–24. doi: 10.1038/s41398-021-01755-3
52. Caso JR, MacDowell KS, Gonzalez-Pinto A, Garcia S, de Diego-Adelino J, Carceller-Sindreu M, et al. Gut microbiota, innate immune pathways, and inflammatory control mechanisms in patients with major depressive disorder. Transl Psychiatry. (2021) 11:645. doi: 10.1038/s41398-021-01755-3
53. Chen CY, Wang YF, Lei L, Zhang Y. Impacts of microbiota and its metabolites through gut-brain axis on pathophysiology of major depressive disorder. Life Sci. (2024) 351:122815. doi: 10.1016/j.lfs.2024.122815
54. Guo F, Jing L, Xu Y, Zhang K, Li Y, Sun N, et al. Gut microbiota and inflammatory factor characteristics in major depressive disorder patients with anorexia. BMC Psychiatry. (2024) 24:334. doi: 10.1186/s12888-024-05778-0
55. Flowers SA, Ward KM, Clark CT. The gut microbiome in bipolar disorder and pharmacotherapy management. Neuropsychobiology. (2020) 79:43–9. doi: 10.1159/000504496
56. Evans SJ, Bassis CM, Hein R, Assari S, Flowers SA, Kelly MB, et al. The gut microbiome composition associates with bipolar disorder and illness severity. J Psychiatr Res. (2017) 87:23–9. doi: 10.1016/j.jpsychires.2016.12.007
57. Huang T, Shang Y, Dai C, Zhang Q, Hu S, Xie J. Gut microbiota and its relation to inflammation in patients with bipolar depression: A cross-sectional study. Ann Gen Psychiatry. (2023) 22:21. doi: 10.1186/s12991-023-00453-2
58. Lu Q, Lai J, Lu H, Ng C, Huang T, Zhang H, et al. Gut microbiota in bipolar depression and its relationship to brain function: an advanced exploration. Front Psychiatry. (2019) 10:784. doi: 10.3389/fpsyt.2019.00784
59. Coello K, Hansen TH, Sorensen N, Munkholm K, Kessing LV, Pedersen O, et al. Gut microbiota composition in patients with newly diagnosed bipolar disorder and their unaffected first-degree relatives. Brain Behav Immun. (2019) 75:112–8. doi: 10.1016/j.bbi.2018.09.026
60. Huang TT, Lai JB, Du YL, Xu Y, Ruan LM, Hu SH. Current understanding of gut microbiota in mood disorders: an update of human studies. Front Genet. (2019) 10:98. doi: 10.3389/fgene.2019.00098
61. Zeng MY, Inohara N, Nunez G. Mechanisms of inflammation-driven bacterial dysbiosis in the gut. Mucosal Immunol. (2017) 10:18–26. doi: 10.1038/mi.2016.75
62. Jazvinscak Jembrek M, Orsolic N, Karlovic D, Peitl V. Flavonols in action: targeting oxidative stress and neuroinflammation in major depressive disorder. Int J Mol Sci. (2023) 24:112–8. doi: 10.3390/ijms24086888
63. Rogers MA, Greene MT, Young VB, Saint S, Langa KM, Kao JY, et al. Depression, antidepressant medications, and risk of clostridium difficile infection. BMC Med. (2013) 11:121. doi: 10.1186/1741-7015-11-121
64. Rong H, Xie XH, Zhao J, Lai WT, Wang MB, Xu D, et al. Similarly in depression, nuances of gut microbiota: evidences from a shotgun metagenomics sequencing study on major depressive disorder versus bipolar disorder with current major depressive episode patients. J Psychiatr Res. (2019) 113:90–9. doi: 10.1016/j.jpsychires.2019.03.017
65. Li Y, Zhang H, Zheng P, Yang J, Wu J, Huang Y, et al. Perturbed gut microbiota is gender-segregated in unipolar and bipolar depression. J Affect Disord. (2022) 317:166–75. doi: 10.1016/j.jad.2022.08.027
66. Rink L, Adams A, Braun C, Bschor T, Kuhr K, Baethge C. Dose-response relationship in selective serotonin and norepinephrine reuptake inhibitors in the treatment of major depressive disorder: A meta-analysis and network meta-analysis of randomized controlled trials. Psychother Psychosom. (2022) 91:84–93. doi: 10.1159/000520554
67. Winter G, Hart RA, Charlesworth RPG, Sharpley CF. Gut microbiome and depression: what we know and what we need to know. Rev Neurosci. (2018) 29:629–43. doi: 10.1515/revneuro-2017-0072
68. Mohajeri MH, La Fata G, Steinert RE, Weber P. Relationship between the gut microbiome and brain function. Nutr Rev. (2018) 76:481–96. doi: 10.1093/nutrit/nuy009
69. Lukic I, Getselter D, Ziv O, Oron O, Reuveni E, Koren O, et al. Antidepressants affect gut microbiota and ruminococcus flavefaciens is able to abolish their effects on depressive-like behavior. Transl Psychiatry. (2019) 9:133. doi: 10.1038/s41398-019-0466-x
70. Shen Y, Yang X, Li G, Gao J, Liang Y. The change of gut microbiota in mdd patients under ssris treatment. Sci Rep. (2021) 11:14918. doi: 10.1038/s41598-021-94481-1
71. Ciocan D, Cassard AM, Becquemont L, Verstuyft C, Voican CS, El Asmar K, et al. Blood Microbiota and Metabolomic Signature of Major Depression before and after Antidepressant Treatment: A Prospective Case-Control Study. J Psychiatry Neurosci. (2021) 46:E358–E68. doi: 10.1503/jpn.200159
72. Gao M, Tu H, Liu P, Zhang Y, Zhang R, Jing L, et al. Association analysis of gut microbiota and efficacy of ssris antidepressants in patients with major depressive disorder. J Affect Disord. (2023) 330:40–7. doi: 10.1016/j.jad.2023.02.143
73. Flowers SA, Evans SJ, Ward KM, McInnis MG, Ellingrod VL. Interaction between atypical antipsychotics and the gut microbiome in a bipolar disease cohort. Pharmacotherapy. (2017) 37:261–7. doi: 10.1002/phar.1890
74. Cussotto S, Strain CR, Fouhy F, Strain RG, Peterson VL, Clarke G, et al. Differential effects of psychotropic drugs on microbiome composition and gastrointestinal function. Psychopharmacol (Berl). (2019) 236:1671–85. doi: 10.1007/s00213-018-5006-5
75. Huang N, Hua D, Zhan G, Li S, Zhu B, Jiang R, et al. Role of Actinobacteria and coriobacteriia in the antidepressant effects of ketamine in an inflammation model of depression. Pharmacol Biochem Behav. (2019) 176:93–100. doi: 10.1016/j.pbb.2018.12.001
76. Yang C, Qu Y, Fujita Y, Ren Q, Ma M, Dong C, et al. Possible role of the gut microbiota-brain axis in the antidepressant effects of (R)-ketamine in a social defeat stress model. Transl Psychiatry. (2017) 7:1294. doi: 10.1038/s41398-017-0031-4
77. Qu Y, Yang C, Ren Q, Ma M, Dong C, Hashimoto K. Comparison of (R)-ketamine and lanicemine on depression-like phenotype and abnormal composition of gut microbiota in a social defeat stress model. Sci Rep. (2017) 7:15725. doi: 10.1038/s41598-017-16060-7
78. Getachew B, Aubee JI, Schottenfeld RS, Csoka AB, Thompson KM, Tizabi Y. Ketamine interactions with gut-microbiota in rats: relevance to its antidepressant and anti-inflammatory properties. BMC Microbiol. (2018) 18:222. doi: 10.1186/s12866-018-1373-7
79. Xu F, Xie Q, Kuang W, Dong Z. Interactions between antidepressants and intestinal microbiota. Neurotherapeutics. (2023) 20:359–71. doi: 10.1007/s13311-023-01362-8
80. Kishi T, Ikuta T, Sakuma K, Okuya M, Hatano M, Matsuda Y, et al. Antidepressants for the treatment of adults with major depressive disorder in the maintenance phase: A systematic review and network meta-analysis. Mol Psychiatry. (2023) 28:402–9. doi: 10.1038/s41380-022-01824-z
81. Cipriani A, Furukawa TA, Salanti G, Chaimani A, Atkinson LZ, Ogawa Y, et al. Comparative efficacy and acceptability of 21 antidepressant drugs for the acute treatment of adults with major depressive disorder: A systematic review and network meta-analysis. Lancet. (2018) 391:1357–66. doi: 10.1016/S0140-6736(17)32802-7
82. Li HY, Zhou DD, Gan RY, Huang SY, Zhao CN, Shang A, et al. Effects and mechanisms of probiotics, prebiotics, synbiotics, and postbiotics on metabolic diseases targeting gut microbiota: A narrative review. Nutrients. (2021) 13:1357–66. doi: 10.3390/nu13093211
83. Suda K, Matsuda K. How microbes affect depression: underlying mechanisms via the gut-brain axis and the modulating role of probiotics. Int J Mol Sci. (2022) 23:3211. doi: 10.3390/ijms23031172
84. Obi-Azuike C, Ebiai R, Gibson T, Hernandez A, Khan A, Anugwom G, et al. A systematic review on gut-brain axis aberrations in bipolar disorder and methods of balancing the gut microbiota. Brain Behav. (2023) 13:e3037. doi: 10.1002/brb3.3037
85. Lai W-t, Deng W-f, Xu S-x, Zhao J, Xu D, Liu Y-h, et al. Shotgun metagenomics reveals both taxonomic and tryptophan pathway differences of gut microbiota in major depressive disorder patients. psychol Med. (2019) 51:90–101. doi: 10.1017/s0033291719003027
86. Park C, Brietzke E, Rosenblat JD, Musial N, Zuckerman H, Ragguett RM, et al. Probiotics for the treatment of depressive symptoms: an anti-inflammatory mechanism? Brain Behav Immun. (2018) 73:115–24. doi: 10.1016/j.bbi.2018.07.006
87. Anhe FF, Roy D, Pilon G, Dudonne S, Matamoros S, Varin TV, et al. A polyphenol-rich cranberry extract protects from diet-induced obesity, insulin resistance and intestinal inflammation in association with increased akkermansia spp. Population in the gut microbiota of mice. Gut. (2015) 64:872–83. doi: 10.1136/gutjnl-2014-307142
88. Chen HM, Kuo PH, Hsu CY, Chiu YH, Liu YW, Lu ML, et al. Psychophysiological effects of lactobacillus plantarum ps128 in patients with major depressive disorder: A preliminary 8-week open trial. Nutrients. (2021) 13:872–3. doi: 10.3390/nu13113731
89. Ding Y, Bu F, Chen T, Shi G, Yuan X, Feng Z, et al. A next-generation probiotic: akkermansia muciniphila ameliorates chronic stress-induced depressive-like behavior in mice by regulating gut microbiota and metabolites. Appl Microbiol Biotechnol. (2021) 105:8411–26. doi: 10.1007/s00253-021-11622-2
90. Miyaoka T, Kanayama M, Wake R, Hashioka S, Hayashida M, Nagahama M, et al. Clostridium butyricum miyairi 588 as adjunctive therapy for treatment-resistant major depressive disorder: A prospective open-label trial. Clin Neuropharmacol. (2018) 41:151–5. doi: 10.1097/WNF.0000000000000299
91. Bambling M, Edwards SC, Hall S, Vitetta L. A combination of probiotics and magnesium orotate attenuate depression in a small ssri resistant cohort: an intestinal anti-inflammatory response is suggested. Inflammopharmacology. (2017) 25:271–4. doi: 10.1007/s10787-017-0311-x
92. Wang JW, Kuo CH, Kuo FC, Wang YK, Hsu WH, Yu FJ, et al. Fecal microbiota transplantation: review and update. J Formos Med Assoc. (2019) 118 Suppl 1:S23–31. doi: 10.1016/j.jfma.2018.08.011
93. Rode AA, Chehri M, Petersen AM, Bytzer P. Faecal microbiota transplantation for the treatment of bowel disease. Ugeskr Laeger. (2017) 179:S23–31. doi: 10.1016/j.jfma.2018.08.011
94. Ooijevaar RE, Terveer EM, Verspaget HW, Kuijper EJ, Keller JJ. Clinical application and potential of fecal microbiota transplantation. Annu Rev Med. (2019) 70:335–51. doi: 10.1146/annurev-med-111717-122956
95. Doll JPK, Vazquez-Castellanos JF, Schaub AC, Schweinfurth N, Kettelhack C, Schneider E, et al. Fecal microbiota transplantation (Fmt) as an adjunctive therapy for depression-case report. Front Psychiatry. (2022) 13:815422. doi: 10.3389/fpsyt.2022.815422
96. Hashimoto K. Neuroinflammation through the vagus nerve-dependent gut-microbiota-brain axis in treatment-resistant depression. Prog Brain Res. (2023) 278:61–77. doi: 10.1016/bs.pbr.2023.01.003
97. Pearson O, Uglik-Marucha N, Miskowiak KW, Cairney SA, Rosenzweig I, Young AH, et al. The relationship between sleep disturbance and cognitive impairment in mood disorders: A systematic review. J Affect Disord. (2023) 327:207–16. doi: 10.1016/j.jad.2023.01.114
98. Sonali S, Ray B, Ahmed Tousif H, Rathipriya AG, Sunanda T, Mahalakshmi AM, et al. Mechanistic insights into the link between gut dysbiosis and major depression: an extensive review. Cells. (2022) 11:207–16. doi: 10.3390/cells11081362
Keywords: major depressive disorder (MDD), bipolar disorder (BD), gut microbiota (GM), therapeutic options, microbe-gut-brain axis (MGBA)
Citation: Zhao J, Liu J, Feng J, Liu X and Hu Q (2024) The gut microbiota-brain connection: insights into major depressive disorder and bipolar disorder. Front. Psychiatry 15:1421490. doi: 10.3389/fpsyt.2024.1421490
Received: 23 April 2024; Accepted: 14 October 2024;
Published: 05 November 2024.
Edited by:
Benno Haarman, University Medical Center Groningen, NetherlandsReviewed by:
Margarita Rivera, University of Granada, SpainCopyright © 2024 Zhao, Liu, Feng, Liu and Hu. This is an open-access article distributed under the terms of the Creative Commons Attribution License (CC BY). The use, distribution or reproduction in other forums is permitted, provided the original author(s) and the copyright owner(s) are credited and that the original publication in this journal is cited, in accordance with accepted academic practice. No use, distribution or reproduction is permitted which does not comply with these terms.
*Correspondence: Xing Liu, bGVvc2hpbmx6QDE2My5jb20=; Qinxue Hu, aHVxaW54dWUxNDBAc3dtdS5lZHUuY24=
†These authors have contributed equally to this work
Disclaimer: All claims expressed in this article are solely those of the authors and do not necessarily represent those of their affiliated organizations, or those of the publisher, the editors and the reviewers. Any product that may be evaluated in this article or claim that may be made by its manufacturer is not guaranteed or endorsed by the publisher.
Research integrity at Frontiers
Learn more about the work of our research integrity team to safeguard the quality of each article we publish.