- 1Department of Traditional Chinese Medicine, Shandong University of Traditional Chinese Medicine, Jinan, China
- 2Department of Intelligent and Information Engineering, Shandong University of Traditional Chinese Medicine, Jinan, China
- 3Innovation Research Institute of Traditional Chinese Medicine, Shandong University of Traditional Chinese Medicine, Jinan, China
- 4Department of Foreign Studies, China University of Petroleum (East China), Qingdao, China
Background: The understanding of the immunological mechanisms underlying bipolar disorder (BD) has enhanced in recent years due to the extensive use of high-density genetic markers for genotyping and advancements in genome-wide association studies (GWAS). However, studies on the relationship between immune cells and the risk of BD remain limited, necessitating further investigation.
Methods: Bidirectional two-sample Mendelian Randomization (MR) analysis was employed to investigate the causal association between immune cell morphologies and bipolar disorder. Immune cell traits were collected from a research cohort in Sardinia, whereas the GWAS summary statistics for BD were obtained from the Psychiatric Genomics Consortium. Sensitivity analyses were conducted, and the combination of MR-Egger and MR-Presso was used to assess horizontal pleiotropy. Cochran’s Q test was employed to evaluate heterogeneity, and the results were adjusted for false discovery rate (FDR).
Results: The study identified six immune cell phenotypes significantly associated with BD incidence (P< 0.01). These phenotypes include IgD- CD27- %lymphocyte, CD33br HLA DR+ CD14- AC, CD8 on CD28+ CD45RA+ CD8br, CD33br HLA DR+ AC, CD14 on CD14+ CD16+ monocyte, and HVEM on CD45RA- CD4+. After adjusting the FDR to 0.2, two immune cell phenotypes remained statistically significant: IgD-CD27-% lymphocyte (OR=1.099, 95% CI: 1.051-1.149, P = 3.51E-05, FDR=0.026) and CD33br HLA DR+ CD14-AC (OR=0.981, 95% CI: 0.971-0.991, P = 2.17E-04, FDR=0.079). In the reverse MR analysis, BD significantly impacted the phenotypes of four monocytes (P< 0.01), including CD64 on CD14+ CD16+ monocyte, CD64 on monocyte, CX3CR1 on CD14- CD16-, CD64 on CD14+ CD16- monocyte. However, after applying the FDR correction (FDR < 0.2), no statistically significant results were observed.
Conclusions: This MR investigation reveals associations between immune cell phenotypes, bipolar disorder, and genetics, providing novel perspectives on prospective therapeutic targets for bipolar disorder.
1 Introduction
Bipolar disorder, a lifelong and recurrent disorder, is characterized by alternating episodes of mania or hypomania and depression (1). Symptoms typically begin between ages 15 and 25 (2), and in severe instances, it can result in cognitive impairment (3), disability (4), and potentially suicide (5). It has been reported that individuals with BD have a suicide risk ranging from 4% to 19%, with 20% to 60% attempting suicide at least once in their lives (6). The etiology of BD remains uncertain, encompassing genetic predispositions, neurotransmitter activity, environmental influences, and immunological mechanisms (7, 8). Currently, lithium is the preferred treatment for BD (9), with cognitive therapy and electroconvulsive therapy as alternatives (10, 11). Timely identification and intervention assist individuals with BD in managing symptoms before severe problems arise, hence improving the prognosis (12). Recently, there has been a notable rise in BD incidence, resulting in an escalating worldwide impact (13).
The immune system and inflammation play significant roles in the pathology of various mental diseases (14). Recent research has demonstrated that the pathophysiology of BD is significantly influenced by inflammatory responses and immune modulation (11). There is a complex association between BD and immune inflammation mechanisms. Meta-analyses have shown increased levels of inflammatory markers, including C-reactive protein, tumor necrosis factor (TNF)-α, and interleukin (IL)-6, in individuals with BD (15). However, one study indicated that inflammatory markers such as interleukin-1 receptor antagonists and TNF-α may vary based on the emotional state of BD, such as normal mood, manic episodes, or depression (16). The longitudinal study has reported that elevated baseline levels of circulating inflammatory markers are associated with an increased risk of developing BD during follow-up (17). Additionally, a MR study has provided weak evidence suggesting that IL-13 and IL-17 may have a protective effect on BD patients (18).
Previous studies have demonstrated a significant association between BD and lymphocytes. Significant differences in T cell subgroups exist between BD patients and healthy controls (19). Additionally, the percentages of total T cells, CD4+ T cells, and CD71+ B cells in BD patients were significantly higher than those in the healthy control group (20). Nevertheless, it is important to acknowledge that the cause-and-effect connection between T cell imbalance and BD remains uncertain (21). Furthermore, a large-scale cross-sectional survey suggests that the ratios of neutrophils to lymphocytes and monocytes to lymphocytes are associated with an increased risk of developing BD (22). Additionally, existing evidence indicates a higher prevalence of autoimmune disorders, such as autoimmune thyroiditis, among individuals with BD, as well as an increased risk of developing BD among those with autoimmune disorders (23). Furthermore, laboratory research findings indicate that lithium treatment is associated with an increased production of natural killer cells induced by circulating cytokines (24). Randomized controlled trials have demonstrated the efficacy of boosting T cell system therapy in addressing immune-inflammatory abnormalities linked to BD and improving antidepressant responsiveness (25). A review article suggests utilizing inflammatory and immune response indicators to monitor the progression of bipolar disorder in patients (26). However, most existing evidence is based on observational studies, and research on the relationship between BD and immune cells may be limited by confounding variables and reverse causality.
As one of the most heritable psychiatric disorders, BD is expected to see a substantial increase in the number of relevant genetic loci in future large-scale studies. This is due to advancements in technology and methodologies, along with the adoption of international consortia and extensive population biobanks, enhancing genetic prediction for BD (27). Additionally, evidence of familial heritability has been discovered for BD (28), focusing on candidate genes such as BDNF, CLOCK, COMT, and DAOA to explore the clinical genetics of BD (7, 29). The review summarizes approaches aimed at identifying safer and more effective medications for individuals with BD through pharmacogenetics research (30). Hence, this study employs GWAS to investigate the complex interplay among individuals with BD, immune inflammation, and genetic predispositions, with the goal of identifying potential therapeutic targets to ameliorate BD symptoms.
MR is a method of causal inference that uses genetic variation as an instrumental variable. It utilizes the natural random allocation of genotypes to infer the effect of biological factors on disease phenotypes (31). MR methods have gained extensive application in causal inference within observational studies because genetic variations are inherent and less susceptible to common confounding factors such as environmental and social elements (32). Prior observational research has suggested an association between immune cell characteristics and BD. This study conducted a comprehensive two-sample MR analysis to investigate the causal relationship between immune cells and BD.
2 Materials and methods
2.1 Study design
A two-sample MR analysis was used to assess the causal association between 731 immune cell characteristics (7 sets) and BD. Genetic variations are utilized as instrumental variables (IVs) to represent risk factors in MR. This approach requires three essential assumptions to establish causal inference. (1) The IVs in this study are genetic variations significantly related to exposure. (2) IVs are independent of both known and unknown confounding influences. (3) IVs impact the outcome solely through exposure, not through alternative pathways. Figure 1 illustrates the analysis workflow. The relevant institutional review board granted approval for this study.
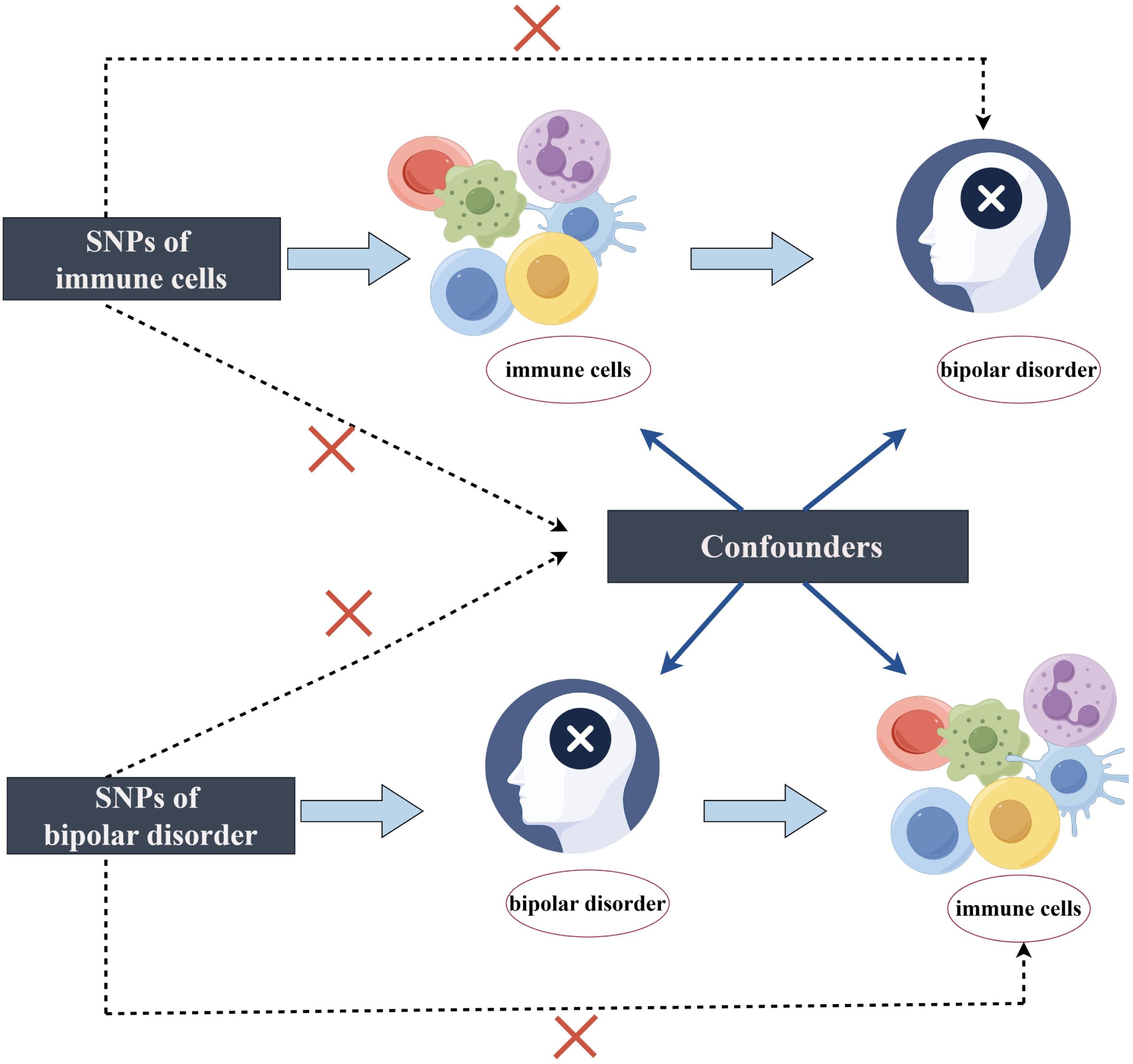
Figure 1. The design of bidirectional Mendelian randomization (MR) study by Figdraw.
2.2 Genome-wide association study data sources for BD
The GWAS summary statistics for BD were obtained from the Psychiatric Genomics Consortium (PGC) (33). The study conducted GWAS on 413,466 individuals of European descent (Ncase = 41,917, Ncontrol = 371,549). BD patients met the international consensus criteria for lifelong BD (DSM-IV, ICD-9, or ICD-10). The GWAS meta-analysis identified 64 independent genetic loci associated with BD, 33 of which were newly discovered, demonstrating genome-wide significance (P < 5 × 10−8).
2.3 Immunity-wide GWAS data sources
The GWAS summary statistics for each immunophenotype can be obtained from the GWAS catalog, with accession numbers ranging from GCST90001391 to GCST90002121, including a cohort of 3,757 Sardinians (34). A high-density array, based on a reference panel of Sardinian sequences (35), was used to estimate approximately 22 million SNPs and tested for correlation after adjusting for covariates such as age, age2, and sex. A total of 731 immunophenotypes were examined, comprising relative cell counts (192), morphologic parameters (32), absolute cell counts (118), and median fluorescence intensities representing surface antigen levels (389).
2.4 Selection of instrumental variables
Based on recent studies, we set the threshold for SNPs related to immune cell phenotypes at P < 1 × 10-5 (34, 36). For SNPs related to BD, we used a threshold of P < 5 × 10-8 (33, 37). Additionally, we conducted a Linkage Disequilibrium check on the aforementioned SNPs (r² = 0.001 and kb = 10,000) (38). Each IV was assessed based on its F-statistic, and only those with F-statistics exceeding 10 were retained for subsequent analysis to ensure robustness and minimize bias from weak instrumental variables (39). SNP harmonization was conducted between the exposure and outcome datasets to maintain consistency in effect allele estimation. Furthermore, SNPs potentially confounded by other variables were filtered out utilizing the Phenoscanner V2 website (http://www.phenoscanner.medschl.cam.ac.uk/).
2.5 Statistical analysis
To investigate the causal association between immune cell phenotypes and the risk of bipolar disorder, this study predominantly used the TwoSampleMR package in R (version 4.3.2). The main MR analysis methods were MR Egger, Weighted Median, Inverse Variance Weighted (IVW), Simple Mode, Weighted Mode, and MR-Presso. Among these, the IVW method served as the principal causal effect estimator in MR research due to its robust capability of detecting causality and yielding substantial testing efficacy (40).
In this study, we used a combination of MR-Egger and MR-Presso to assess horizontal pleiotropy (P < 0.05 indicating horizontal pleiotropy). Heterogeneity of IVs was measured using Cochran’s IVW Q statistics (P < 0.05 indicating heterogeneity). Furthermore, scatter plots and funnel plots were employed. Moreover, leave-one-out sensitivity analyses were conducted to determine if a single SNP influenced the observed causal relationship. Additionally, FDR correction was performed using the Bioladder web tool, as multiplex testing increases the likelihood of type 1 errors (41). To investigate reverse causation, identical techniques were applied to analyze the reverse MR of immune cell morphologies and bipolar disease. Results were considered highly significant when P < 0.01 (42). Additionally, based on prior research, an FDR < 0.2 was considered indicative of a causal relationship (43).
3 Results
3.1 Exploration of the causal effect of immunophenotypes on BD
Initially, the causative impact of 731 immunophenotypes as exposure variables on BD was investigated, with findings displayed in Figure 2. Before FDR correction, six immunophenotypes were found to impact the occurrence of BD (P< 0.01). Specifically, significant negative associations with BD risk were observed for CD33br HLA DR+ CD14-AC on monocyte cells (OR=0.981, 95% CI: 0.971-0.991, P = 2.17E-04), CD8 on CD28+ CD45RA+ CD8br on Treg cells (OR=0.965, 95% CI: 0.943-0.987, P = 0.002), CD33br HLA DR+ AC on monocyte cells (OR=0.979, 95% CI: 0.965-0.993, P = 0.003), CD14 on CD14+ CD16+ monocyte (OR=0.933, 95% CI: 0.889-0.979, P = 0.005), and HVEM on CD45RA-CD4+ on maturation stages of T cells (OR=0.966, 95% CI: 0.942-0.991, P = 0.007), while IgD-CD27-% lymphocyte on B cells (OR=1.099, 95% CI: 1.051-1.149, P = 3.51E-05) showed a significant positive correlation. After adjusting the FDR to 0.2, the risk of BD remained significantly associated with IgD-CD27-% lymphocyte (P = 3.51E-05, FDR = 0.026) and CD33br HLA DR+ CD14-AC (P = 2.17E-04, FDR = 0.079).
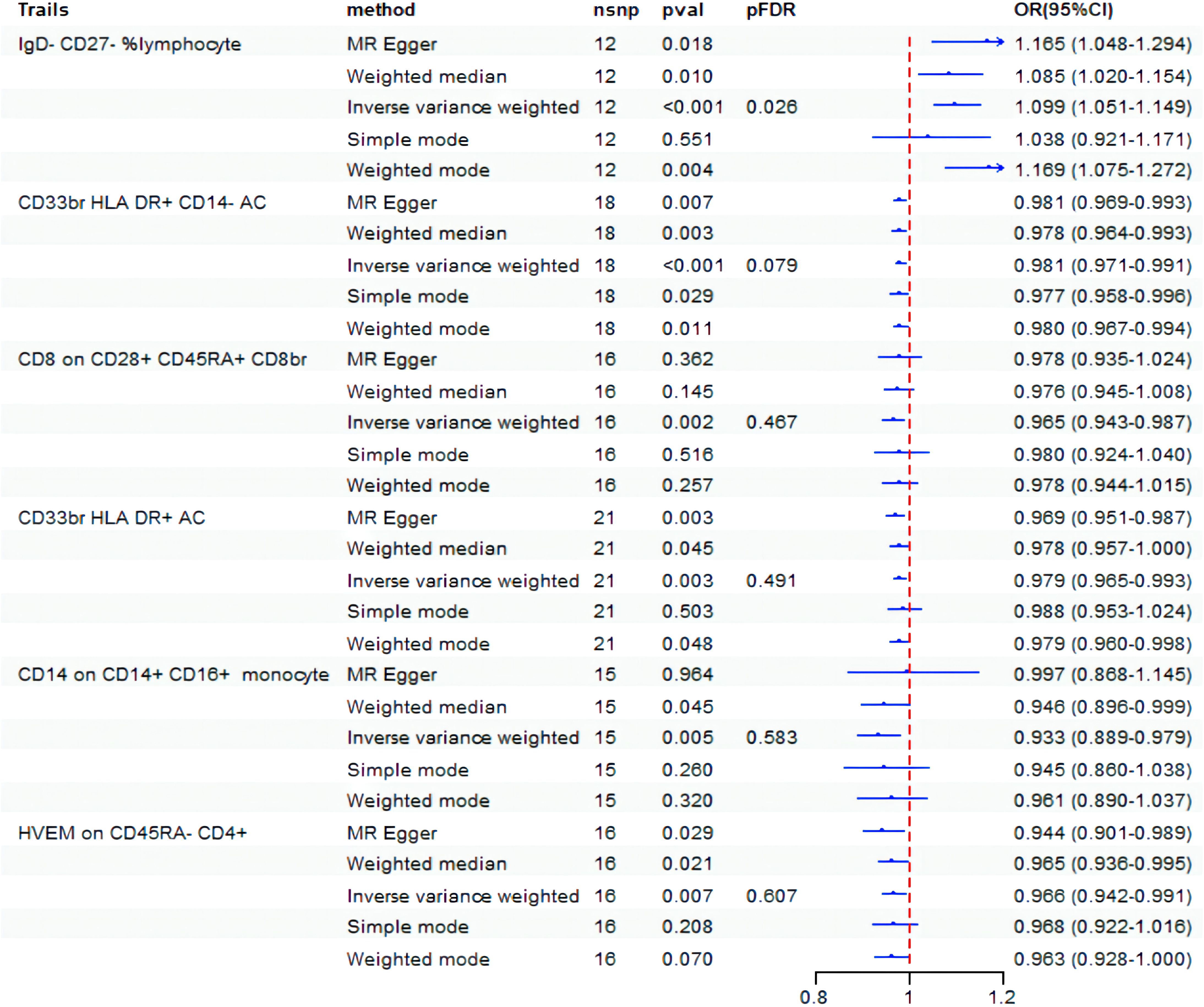
Figure 2. Forest plot showed the causal association between immune cell traits and BD. nsnp, nonsynonymous single-nucleotide polymorphism; OR, odds ratio; CI, confidence interval; PFDR, P value corrected by FDR.
3.2 Exploration of the causal effect of BD on immunophenotypes
Next, we conducted an analysis to examine the causal impact of BD as an exposure variable on 731 immunophenotypes. The results indicated that BD influences four specific immunophenotypes of monocytes, as shown in Figure 3. BD exhibited a negative correlation with CD64 on CD14+ CD16+ monocyte (OR = 0.812, 95% CI: 0.713-0.925, P = 0.001), CD64 on monocyte (OR=0.834, 95% CI: 0.735-0.947, P = 0.005), CX3CR1 on CD14- CD16- (OR = 0.838, 95% CI: 0.740-0.950, P = 0.006), and CD64 on CD14+ CD16- monocyte (OR = 0.837, 95% CI: 0.738-0.950, P = 0.006). However, after FDR correction, no statistically significant results were observed.
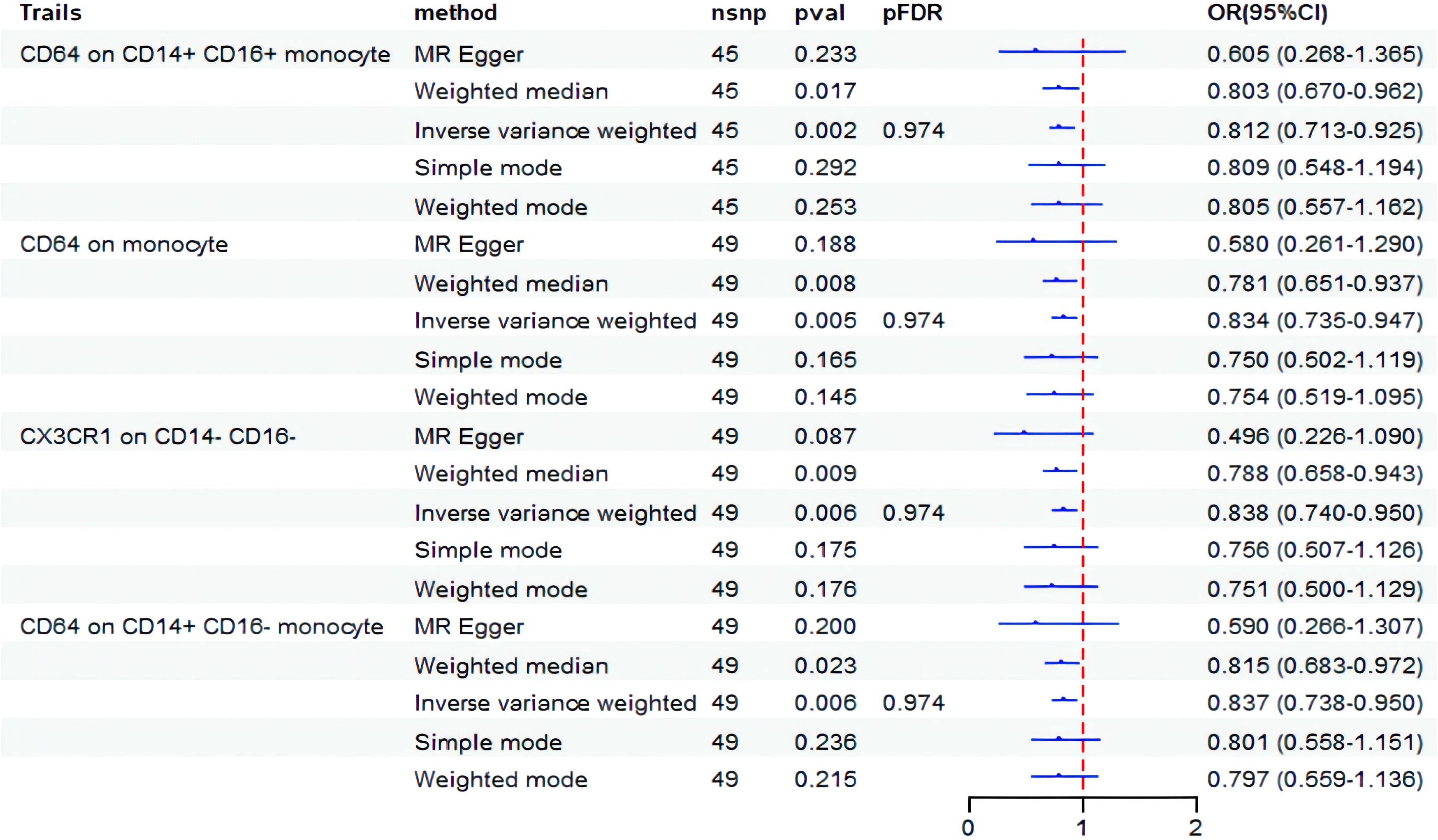
Figure 3. Forest plot showed the causal association between BD and immune cell traits. nsnp, nonsynonymous single-nucleotide polymorphism; OR, odds ratio; CI, confidence interval; PFDR, P value corrected by FDR.
3.3 Sensitivity analysis
In sections 3.1 and 3.2, the MR-Egger and MR-Presso methods indicated no evidence of horizontal pleiotropy, and the Cochran Q test showed no heterogeneity. These findings are presented in Supplementary Tables 1, 2. Subsequently, scatter plots (Supplementary Figures 1 and 2) and funnel plots (Supplementary Figures 3 and 4) further substantiated these findings. Furthermore, the leave-one-out analysis demonstrated the robustness of the MR results (Supplementary Figures 5 and 6), as excluding any single SNP associated with immunophenotypes and BD did not significantly alter the overall findings. Additionally, all IDs with positive outcomes for immune cell morphologies were filtered out using the IVW technique (P < 0.05). A heatmap was used to visually analyze the study findings (Figure 4).
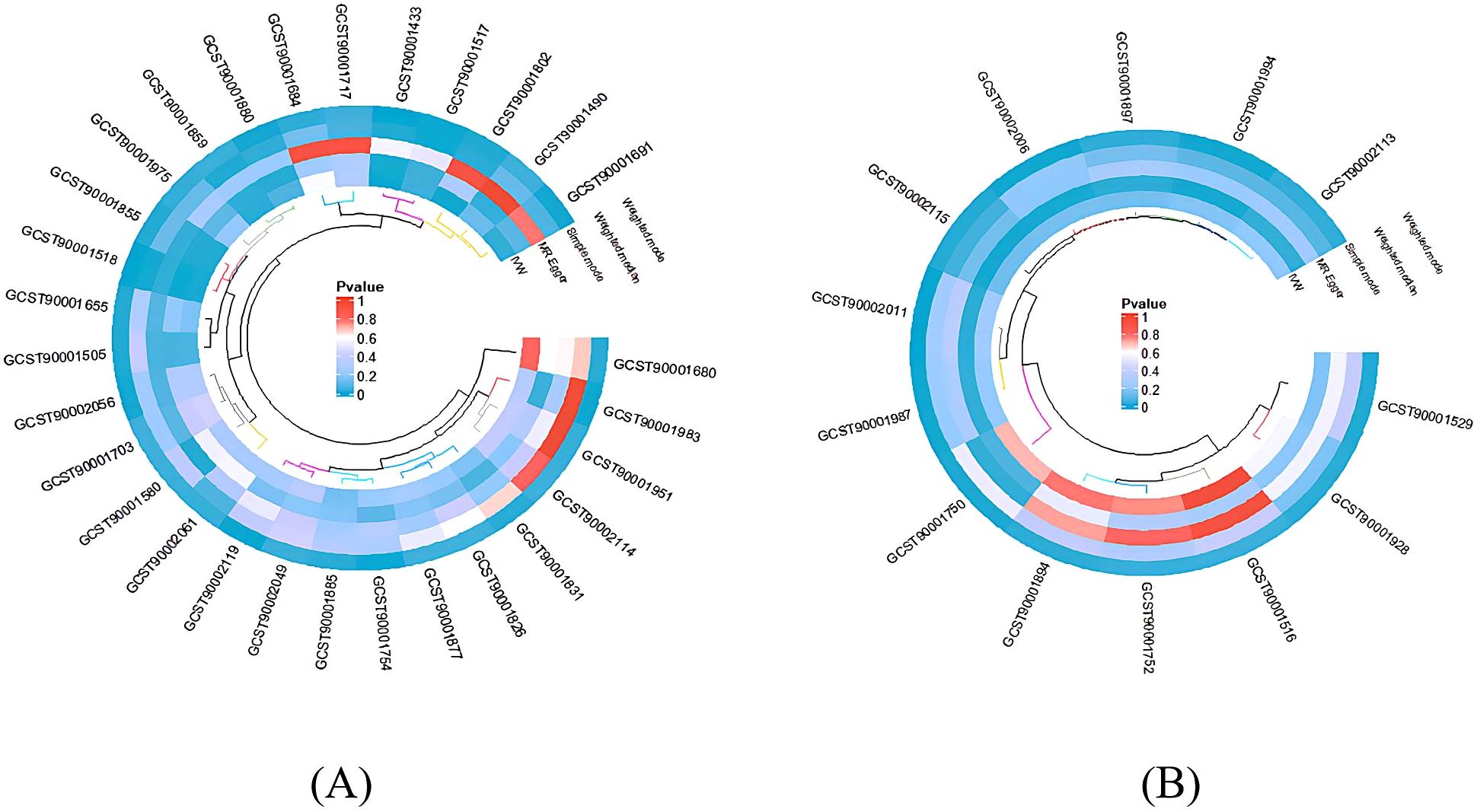
Figure 4. The heatmap depicting the IDs of immune cell phenotypes with positive results and the p-values from the sensitivity analysis. The outer circle represents the IDs of immune cell phenotypes, while the inner circle uses different colors to indicate the p-values of different sensitivity analysis results. (A) IDs showing the causal effect of immune cells on BD; (B) IDs showing the causal effect of BD on immune cells.
4 Discussion
Through a two-sample bidirectional MR study, we identified six immune cell phenotypes that exhibited a significant association with the risk of BD (P< 0.01). Upon adjusting for FDR < 0.20, two of these immune cell morphologies remained associated with the risk of BD. In the reverse MR analysis, our findings suggested a potential causal relationship between BD and four monocyte immune cell phenotypes (P< 0.01). However, after applying FDR correction, none of these associations retained statistical significance.
The current research indicates that B-cell activating factor and A proliferation-inducing ligand, which are critical growth factors for B cells and B cell-driven autoimmunity, exhibit aberrant plasma levels in BD patients. This suggests a pivotal role for B cells in BD (44). Neuroinflammation is one of the causes of BD (45), with B cells facilitating inflammatory responses by expressing inflammation-related genes and ribosomal protein genes (46). B cells can be categorized into four principal subsets based on the differential expression of the immunoglobulins IgD and CD27. IgD−CD27− B cells constitute a heterogeneous population of B cells, which have been shown to be associated with aging and systemic lupus erythematosus (47). Our findings suggest that IgD-CD27-% lymphocytes in B cells might confer a protective effect in BD patients. The CD27-IgD-B cell subset exhibited pronounced pro-inflammatory effects in nonagenarian cells, which were competent to produce pro-inflammatory cytokines (such as TNF, IL-6, and IL-8) (48). However, an analysis of immunosenescence markers and clinical characteristics in BD reveals a tenuous correlation between the percentage of late differentiated B cells (CD3‐CD19+IgD‐CD27‐) and immunological age factors (49). Consequently, the connection between CD27-IgD-cell subsets and BD warrants further exploration.
Treg cells are essential for modulating peripheral immune responses, monitoring the brain’s immune system, and facilitating neuroimmune interactions and coordination (50). Dysfunctional Tregs can lead to abnormal immune activation and neuroinflammation. Increased Treg levels are associated with symptom improvement in mental disorders, while decreased levels correlate with symptom worsening and heightened neuroinflammation (51). In BD patients, TLR-2 and other TLR signaling pathways impair Treg functionality, contributing to neuroinflammation and immune dysregulation (52). Meta-analysis evidence supports that heightened IL-10 levels are associated with increased Treg cells activity in BD (53). Conversely, literature reviews suggest that reductions in Tregs and diminished IL-10 secretion in BD may account for the elevated incidence of autoimmune disorders (54). These observations underscore the potential impact of Treg cells on BD. Our research delineates a negative association between CD8 on CD28+ CD45RA+ CD8br of Treg cells and BD. Emerging studies reveal that during different stages of BD, there is a marked decline in the functional capacities of both Treg cells and effector T cells. In individuals with BD, there is a pronounced reduction in Treg cells expressing CD152 and GARP, along with decreased numbers of CD4+ and CD8+ cells marked by CD71+ (55). CD4+ T cells secrete cytokines to facilitate immune responses, while CD8+ T cells execute cytotoxic activities. Their dysregulation may play a significant role in the pathophysiology of BD (21). Research further indicates that BD patients affected by childhood maltreatment exhibit elevated CD8+ T cells levels compared to their non-maltreated counterparts (56). Additionally, studies have shown correlations between modifications in brain structure and changes in CD4+ and CD8+ cells in individuals with BD (19). Moreover, research suggests that the latent state of HCMV may intensify the immune risk phenotypes associated with BD (55). This aligns with our findings that certain maturation stages of T cells, particularly HVEM expression on CD45RA-CD4+, constitute risk factors for BD.
The research identified CD33br HLA DR+ AC and CD33br HLA DR+ CD14-AC in myeloid cells as risk factors for BD. Previous studies have indicated that common genetic variations at the HLA loci are implicated in increasing susceptibility to BD (57). Moreover, gene expression patterns related to immature neutrophils, a component of myeloid cells, have been observed in peripheral blood cells from patients diagnosed with schizophrenia and bipolar disorder, indicating alterations in the innate immune system (58). Investigations into brain tissue have corroborated some genetic findings associated with BD, such as reduced expression of HLA-DPA1 (59), which encodes the α-chain, while HLA-DR encodes the β-chain, and these chains form the HLA class II molecule. Empirical evidence suggests that the percentages of CD11b/CD33+ hi and CD11b/CD33+ lo cells differed significantly between T carriers and healthy controls in patients with bipolar I disorder in the Han Chinese population (22). This suggests, for the first time, that myeloid-derived suppressor cells might play a role in patients with bipolar I disorder in the Han Chinese population. Thus, further exploration into the association between HLA-DR on myeloid cells and BD is warranted.
Additionally, this study reveals that CD14 expression on CD14+ CD16+ monocytes may impact BD patients, while BD may influence four phenotypic characteristics of monocytes: CD64 on CD14+ CD16- monocytes, CX3CR1 on CD14- CD16- monocytes, CD64 on monocytes, and CD64 on CD14+ CD16+ monocytes. A study using flow cytometry detected differential protein signaling in monocytes between treatment responders and non-responders (60). Pediatric BD patients displayed significantly higher monocyte counts compared to controls, suggesting potential clinical benefits of early BD detection through monocyte counts (61). Monocytes are classified into three categories based on surface markers CD14 and CD16: classical (CD14+CD16-), non-classical (CD14-CD16+), and intermediate (CD14+CD16+) (34). Monocytes exacerbate inflammation by releasing pro-inflammatory cytokines such as IL-10 and IL-15, and this inflammatory activation is closely linked to BD (62). It has been found that BD patients exhibit alterations in the expression of the monocyte marker CD14, and lithium treatment has been noted to exert an immunomodulatory effect on CD14 monocytes and dendritic cells in these patients (20). However, a study found no changes in CD64 mRNA expression among BD patients (63), presenting a possible contradiction to these observations.
In conclusion, the MR analysis has elucidated immune cell phenotypes associated with BD, highlighting immune system activation, inflammation, and bidirectional communication between the brain and the immune system. Neuroinflammation represents one of the key biological mechanisms in BD (64). Immune dysfunction may activate the hypothalamic-pituitary-adrenal axis through various pro-inflammatory cytokines and chemokines, thereby altering cerebral pathways associated with serotonin and catecholamines, leading to mood alterations (65). Furthermore, immune dysregulation may advance the progression of BD by impacting neurotransmitter synthesis, synaptic functionality, neural plasticity (61), brain regions, and neural circuits (66). Additionally, immune dysfunction might disrupt central nervous system operations by modifying the permeability of the blood-brain barrier (67). In turn, the brain can regulate immune responses by controlling the production and release of inflammatory mediators (such as IL-6 and TNF), activating microglia that influence oxidative stress, and modulating the autonomic nervous system (68, 69). Recent studies indicate that epigenetic alterations, including DNA methylation, histone modification, and non-coding RNAs (e.g., miRNA and lncRNA), can induce gene expression variability (70–72). These modifications can be triggered by environmental factors like lifestyle and traumatic experiences, consequently activating pertinent genes and fostering the development of BD (72). Therefore, further investigation into the interplay between inflammation, the immune system, and the brain against a genetic backdrop is crucial for a deeper understanding of the pathophysiological mechanisms of BD and identifying novel therapeutic targets.
However, this research has several limitations. Firstly, due to constraints in the dataset, certain immune cell morphologies could not be analyzed. Secondly, this cohort study, based on a population from Sardinia, lacks stratification by gender and age, limiting the generalizability and accuracy of the conclusions. Finally, the selection of instrumental variables for immune cell phenotypes (P < 1×10−5) and the interpretation of the results (FDR < 0.2) lacked stringent rigor. Therefore, future research should incorporate larger sample sizes and employ more comprehensive causal analysis techniques (such as CAUSE) to further elucidate the relationship between immune cell phenotypes and BD.
5 Conclusion
This study reveals a causal relationship between immune cell phenotypes and bipolar disorder, providing new insights into the pathogenic mechanisms of BD and potential therapeutic targets.
Data availability statement
The original contributions presented in the study are included in the article/Supplementary Material. Further inquiries can be directed to the corresponding author.
Ethics statement
Ethical review and approval was not required for the study of human participants in accordance with the local legislation and institutional requirements.
Author contributions
MW: Writing – review & editing, Methodology, Software, Writing – original draft. SW: Methodology, Writing – review & editing. GY: Methodology, Writing – original draft. MG: Writing – review & editing. XZ: Investigation, Writing – review & editing. ZC: Data curation, Writing – review & editing. DG: Funding acquisition, Supervision, Writing – review & editing.
Funding
The author(s) declare financial support was received for the research, authorship, and/or publication of this article. The study received funding from the National Natural Science Foundation of China (No. 81473558) and the Shandong Provincial Natural Science Foundation (No. ZR2022MH065).
Acknowledgments
Thanks to GWAS database contributors for sharing the data from the publicly accessible online platform.
Conflict of interest
The authors declare that the research was conducted in the absence of any commercial or financial relationships that could be construed as a potential conflict of interest.
Publisher’s note
All claims expressed in this article are solely those of the authors and do not necessarily represent those of their affiliated organizations, or those of the publisher, the editors and the reviewers. Any product that may be evaluated in this article, or claim that may be made by its manufacturer, is not guaranteed or endorsed by the publisher.
Supplementary material
The Supplementary Material for this article can be found online at: https://www.frontiersin.org/articles/10.3389/fpsyt.2024.1411280/full#supplementary-material
References
1. Husain SF, Tang TB, Tam WW, Tran BX, Ho CS, Ho RC. Cortical haemodynamic response during the verbal fluency task in patients with bipolar disorder and borderline personality disorder: a preliminary functional near-infrared spectroscopy study. BMC Psychiatry. (2021) 21:201. doi: 10.1186/s12888-021-03195-1
3. Sole B, Jimenez E, Torrent C, Reinares M, Bonnin C, Torres I, et al. Cognitive impairment in bipolar disorder: treatment and prevention strategies. Int J Neuropsychopharmacol. (2017) 20:670–80. doi: 10.1093/ijnp/pyx032
4. Harrison PJ, Geddes JR, Tunbridge EM. The emerging neurobiology of bipolar disorder. Trends Neurosci. (2018) 41:18–30. doi: 10.1016/j.tins.2017.10.006
5. Dome P, Rihmer Z, Gonda X. Suicide risk in bipolar disorder: a brief review. Med (Kaunas). (2019) 55. doi: 10.3390/medicina55080403
6. Rihmer Z, Gonda X, Döme P. The assessment and management of suicide risk in bipolar disorder. In: The treatment of bipolar disorder: Integrative clinical strategies and future directions. (New York, NY: Oxford University Press) (2017). p. 207–24.
7. Freund N, Juckel G. Bipolar disorder: its etiology and how to model in rodents. Methods Mol Biol. (2019) 2011:61–77. doi: 10.1007/978-1-4939-9554-7_4
8. Oliveira J, Oliveira-Maia AJ, Tamouza R, Brown AS, Leboyer M. Infectious and immunogenetic factors in bipolar disorder. Acta Psychiatr Scand. (2017) 136:409–23. doi: 10.1111/acps.12791
9. Rybakowski JK. Lithium. Eur Neuropsychopharmacol. (2022) 57:86–7. doi: 10.1016/j.euroneuro.2022.01.111
10. Smith S, Falk A, Joseph R, Wilk A. Mood and anxiety disorders: bipolar disorder. FP Essent. (2023) 527:13–8.
11. Madireddy S, Madireddy S. Therapeutic interventions to mitigate mitochondrial dysfunction and oxidative stress-induced damage in patients with bipolar disorder. Int J Mol Sci. (2022) 23. doi: 10.3390/ijms23031844
12. Brietzke E, Rosa AR, Pedrini M, Noto MN, Kapczinski F, Scott J. Challenges and developments in research of the early stages of bipolar disorder. Braz J Psychiatry. (2016) 38:329–37. doi: 10.1590/1516-4446-2016-1975
13. Zhong Y, Chen Y, Su X, Wang M, Li Q, Shao Z, et al. Global, regional and national burdens of bipolar disorders in adolescents and young adults: a trend analysis from 1990 to 2019. Gen Psychiatr. (2024) 37. doi: 10.1136/gpsych-2023-101255
14. Bhatt S, Dhar AK, Samanta MK, Suttee A. Effects of current psychotropic drugs on inflammation and immune system. Adv Exp Med Biol. (2023) 1411:407–34. doi: 10.1007/978-981-19-7376-5_18
15. Solmi M, Suresh SM, Osimo EF, Fornaro M, Bortolato B, Croatto G, et al. Peripheral levels of c-reactive protein, tumor necrosis factor-alpha, interleukin-6, and interleukin-1beta across the mood spectrum in bipolar disorder: a meta-analysis of mean differences and variability. Brain Behav Immun. (2021) 97:193–203. doi: 10.1016/j.bbi.2021.07.014
16. Rowland T, Perry BI, Upthegrove R, Barnes N, Chatterjee J, Gallacher D, et al. Neurotrophins, cytokines, oxidative stress mediators and mood state in bipolar disorder: systematic review and meta-analyses. Br J Psychiatry. (2018) 213:514–25. doi: 10.1192/bjp.2018.144
17. Hayes JF, Khandaker GM, Anderson J, Mackay D, Zammit S, Lewis G, et al. Childhood interleukin-6, c-reactive protein and atopic disorders as risk factors for hypomanic symptoms in young adulthood: a longitudinal birth cohort study. Psychol Med. (2017) 47:23–33. doi: 10.1017/S0033291716001574
18. Perry BI, Upthegrove R, Kappelmann N, Jones PB, Burgess S, Khandaker GM. Associations of immunological proteins/traits with schizophrenia, major depression and bipolar disorder: a bi-directional two-sample mendelian randomization study. Brain Behav Immun. (2021) 97:176–85. doi: 10.1016/j.bbi.2021.07.009
19. Escelsior A, Inuggi A, Sterlini B, Bovio A, Marenco G, Bode J, et al. T-cell immunophenotype correlations with cortical thickness and white matter microstructure in bipolar disorder. J Affect Disord. (2024) 348:179–90. doi: 10.1016/j.jad.2023.12.054
20. Wu TN, Lee CS, Wu BJ, Sun HJ, Chang CH, Chen CY, et al. Immunophenotypes associated with bipolar disorder and lithium treatment. Sci Rep. (2019) 9:17453. doi: 10.1038/s41598-019-53745-7
21. Chen Z, Huang Y, Wang B, Peng H, Wang X, Wu H, et al. T cells: an emerging cast of roles in bipolar disorder. Transl Psychiatry. (2023) 13:153. doi: 10.1038/s41398-023-02445-y
22. Wei Y, Feng J, Ma J, Chen D, Chen J. Neutrophil/lymphocyte, platelet/lymphocyte and monocyte/lymphocyte ratios in patients with affective disorders. J Affect Disord. (2022) 309:221–8. doi: 10.1016/j.jad.2022.04.092
23. Li H, Hong W, Zhang C, Wu Z, Wang Z, Yuan C, et al. Il-23 and tgf-beta1 levels as potential predictive biomarkers in treatment of bipolar I disorder with acute manic episode. J Affect Disord. (2015) 174:361–6. doi: 10.1016/j.jad.2014.12.033
24. Furlan R, Melloni E, Finardi A, Vai B, Di Toro S, Aggio V, et al. Natural killer cells protect white matter integrity in bipolar disorder. Brain Behav Immun. (2019) 81:410–21. doi: 10.1016/j.bbi.2019.06.037
25. Poletti S, Zanardi R, Mandelli A, Aggio V, Finardi A, Lorenzi C, et al. Low-dose interleukin 2 antidepressant potentiation in unipolar and bipolar depression: safety, efficacy, and immunological biomarkers. Brain Behav Immun. (2024) 118:52–68. doi: 10.1016/j.bbi.2024.02.019
26. Sayana P, Colpo GD, Simoes LR, Giridharan VV, Teixeira AL, Quevedo J, et al. A systematic review of evidence for the role of inflammatory biomarkers in bipolar patients. J Psychiatr Res. (2017) 92:160–82. doi: 10.1016/j.jpsychires.2017.03.018
27. O’Connell KS, Coombes BJ. Genetic contributions to bipolar disorder: current status and future directions. Psychol Med. (2021) 51:2156–67. doi: 10.1017/S0033291721001252
28. Song J, Bergen SE, Kuja-Halkola R, Larsson H, Landen M, Lichtenstein P. Bipolar disorder and its relation to major psychiatric disorders: a family-based study in the swedish population. Bipolar Disord. (2015) 17:184–93. doi: 10.1111/bdi.12242
29. Ahmadi L, Kazemi NS, Behbahani P, Khajeddin N, Pourmehdi-Boroujeni M. Genetic variations of daoa (rs947267 and rs3918342) and comt genes (rs165599 and rs4680) in schizophrenia and bipolar i disorder. Basic Clin Neurosci. (2018) 9:429–38. doi: 10.32598/bcn.9.6.429
30. Gordovez F, Mcmahon FJ. The genetics of bipolar disorder. Mol Psychiatry. (2020) 25:544–59. doi: 10.1038/s41380-019-0634-7
31. Xue H, Liu S, Zeng L, Fan W. Causal effect of systemic lupus erythematosus on psychiatric disorders: a two-sample mendelian randomization study. J Affect Disord. (2024) 347:422–8. doi: 10.1016/j.jad.2023.11.033
32. Plotnikov D, Guggenheim JA. Mendelian randomisation and the goal of inferring causation from observational studies in the vision sciences. Ophthalmic Physiol Opt. (2019) 39:11–25. doi: 10.1111/opo.12596
33. Mullins N, Forstner AJ, O’Connell KS, Coombes B, Coleman J, Qiao Z, et al. Genome-wide association study of more than 40,000 bipolar disorder cases provides new insights into the underlying biology. Nat Genet. (2021) 53:817–29. doi: 10.1038/s41588-021-00857-4
34. Orru V, Steri M, Sidore C, Marongiu M, Serra V, Olla S, et al. Complex genetic signatures in immune cells underlie autoimmunity and inform therapy. Nat Genet. (2020) 52:1036–45. doi: 10.1038/s41588-020-0684-4
35. Sidore C, Busonero F, Maschio A, Porcu E, Naitza S, Zoledziewska M, et al. Genome sequencing elucidates sardinian genetic architecture and augments association analyses for lipid and blood inflammatory markers. Nat Genet. (2015) 47:1272–81. doi: 10.1038/ng.3368
36. Wang A, Zhang J. Causal role of immune cells in psoriasis: a mendelian randomization analysis. Front Immunol. (2024) 15:1326717. doi: 10.3389/fimmu.2024.1326717
37. Hu Y, Xiong Z, Huang P, He W, Zhong M, Zhang D, et al. Association of mental disorders with sepsis: a bidirectional mendelian randomization study. Front Public Health. (2024) 12:1327315. doi: 10.3389/fpubh.2024.1327315
38. Ma Z, Zhao M, Zhao H, Qu N. Causal role of immune cells in generalized anxiety disorder: mendelian randomization study. Front Immunol. (2023) 14:1338083. doi: 10.3389/fimmu.2023.1338083
39. Sun K, Gao Y, Wu H, Huang X. The causal relationship between gut microbiota and type 2 diabetes: a two-sample mendelian randomized study. Front Public Health. (2023) 11:1255059. doi: 10.3389/fpubh.2023.1255059
40. Bowden J, Del GMF, Minelli C, Davey SG, Sheehan N, Thompson J. A framework for the investigation of pleiotropy in two-sample summary data mendelian randomization. Stat Med. (2017) 36:1783–802. doi: 10.1002/sim.7221
41. Burgess S, Bowden J, Fall T, Ingelsson E, Thompson SG. Sensitivity analyses for robust causal inference from mendelian randomization analyses with multiple genetic variants. Epidemiology. (2017) 28:30–42. doi: 10.1097/EDE.0000000000000559
42. Aru N, Yang C, Chen Y, Liu J. Causal association of immune cells and polycystic ovarian syndrome: a mendelian randomization study. Front Endocrinol (Lausanne). (2023) 14:1326344. doi: 10.3389/fendo.2023.1326344
43. Wang C, Zhu D, Zhang D, Zuo X, Yao L, Liu T, et al. Causal role of immune cells in schizophrenia: mendelian randomization (mr) study. BMC Psychiatry. (2023) 23:590. doi: 10.1186/s12888-023-05081-4
44. Engh JA, Ueland T, Agartz I, Andreou D, Aukrust P, Boye B, et al. Plasma levels of the cytokines b cell-activating factor (baff) and a proliferation-inducing ligand (april) in schizophrenia, bipolar, and major depressive disorder: a cross sectional, multisite study. Schizophr Bull. (2022) 48:37–46. doi: 10.1093/schbul/sbab106
45. Rantala MJ, Luoto S, Borraz-Leon JI, Krams I. Bipolar disorder: an evolutionary psychoneuroimmunological approach. Neurosci Biobehav Rev. (2021) 122:28–37. doi: 10.1016/j.neubiorev.2020.12.031
46. Qi L, Qiu Y, Li S, Yi N, Li C, Teng Z, et al. Single-cell immune profiling reveals broad anti-inflammation response in bipolar disorder patients with quetiapine and valproate treatment. iScience. (2023) 26:107057. doi: 10.1016/j.isci.2023.107057
47. Beckers L, Somers V, Fraussen J. IgD (-) CD27(-) double negative (dn) b cells: origins and functions in health and disease. Immunol Lett. (2023) 255:67–76. doi: 10.1016/j.imlet.2023.03.003
48. Nevalainen T, Autio A, Kummola L, Salomaa T, Junttila I, Jylha M, et al. Cd27- igd- b cell memory subset associates with inflammation and frailty in elderly individuals but only in males. Immun Ageing. (2019) 16:19. doi: 10.1186/s12979-019-0159-6
49. Rizzo LB, Swardfager W, Maurya PK, Graiff MZ, Pedrini M, Asevedo E, et al. An immunological age index in bipolar disorder: a confirmatory factor analysis of putative immunosenescence markers and associations with clinical characteristics. Int J Methods Psychiatr Res. (2018) 27:e1614. doi: 10.1002/mpr.1614
50. Debnath M, Raison CL, Maes M, Berk M. Role of the t-cell network in psychiatric disorders. Immuno-Psychiatry: Facts Prospects. (Cham, Switzerland: Springer Nature Switzerland AG) (2021), 109–32.
51. Corsi-Zuelli F, Deakin B, de Lima M, Qureshi O, Barnes NM, Upthegrove R, et al. T regulatory cells as a potential therapeutic target in psychosis? Current challenges and future perspectives. Brain Behav Immun Health. (2021) 17:100330. doi: 10.1016/j.bbih.2021.100330
52. Wieck A, Grassi-Oliveira R, Do PC, Viola TW, Petersen LE, Porto B, et al. Toll-like receptor expression and function in type I bipolar disorder. Brain Behav Immun. (2016) 54:110–21. doi: 10.1016/j.bbi.2016.01.011
53. Modabbernia A, Taslimi S, Brietzke E, Ashrafi M. Cytokine alterations in bipolar disorder: a meta-analysis of 30 studies. Biol Psychiatry. (2013) 74:15–25. doi: 10.1016/j.biopsych.2013.01.007
54. Qiu R, Zhou L, Ma Y, Zhou L, Liang T, Shi L, et al. Regulatory t cell plasticity and stability and autoimmune diseases. Clin Rev Allergy Immunol. (2020) 58:52–70. doi: 10.1007/s12016-018-8721-0
55. Maes M, Nani JV, Noto C, Rizzo L, Hayashi M, Brietzke E. Impairments in peripheral blood t effector and t regulatory lymphocytes in bipolar disorder are associated with staging of illness and anti-cytomegalovirus igg levels. Mol Neurobiol. (2021) 58:229–42. doi: 10.1007/s12035-020-02110-1
56. Foiselle M, Lajnef M, Hamdani N, Boukouaci W, Wu CL, Naamoune S, et al. Immune cell subsets in patients with bipolar disorder or schizophrenia with history of childhood maltreatment. Brain Behav Immun. (2023) 112:42–50. doi: 10.1016/j.bbi.2023.05.015
57. Purcell SM, Wray NR, Stone JL, Visscher PM, O’Donovan MC, Sullivan PF, et al. Common polygenic variation contributes to risk of schizophrenia and bipolar disorder. Nature. (2009) 460:748–52. doi: 10.1038/nature08185
58. Torsvik A, Brattbakk HR, Trentani A, Holdhus R, Stansberg C, Bartz-Johannessen CA, et al. Patients with schizophrenia and bipolar disorder display a similar global gene expression signature in whole blood that reflects elevated proportion of immature neutrophil cells with association to lipid changes. Transl Psychiatry. (2023) 13:147. doi: 10.1038/s41398-023-02442-1
59. Morgan LZ, Rollins B, Sequeira A, Byerley W, Delisi LE, Schatzberg AF, et al. Quantitative trait locus and brain expression of hla-dpa1 offers evidence of shared immune alterations in psychiatric disorders. Microarrays (Basel). (2016) 5. doi: 10.3390/microarrays5010006
60. Gao K, Ayati M, Koyuturk M, Calabrese JR, Ganocy SJ, Kaye NM, et al. Protein biomarkers in monocytes and cd4(+) lymphocytes for predicting lithium treatment response of bipolar disorder: a feasibility study with tyramine-based signal-amplified flow cytometry. Psychopharmacol Bull. (2022) 52:8–35.
61. Ceylan MF, Tural HS, Kasak M, Senat A, Erel O. Increased prolidase activity and high blood monocyte counts in pediatric bipolar disorder. Psychiatry Res. (2019) 271:360–4. doi: 10.1016/j.psychres.2018.11.066
62. Hughes HK, Yang H, Lesh TA, Carter CS, Ashwood P. Evidence of innate immune dysfunction in first-episode psychosis patients with accompanying mood disorder. J Neuroinflammation. (2022) 19:287. doi: 10.1186/s12974-022-02648-y
63. North HF, Weissleder C, Fullerton JM, Sager R, Webster MJ, Weickert CS. A schizophrenia subgroup with elevated inflammation displays reduced microglia, increased peripheral immune cell and altered neurogenesis marker gene expression in the subependymal zone. Transl Psychiatry. (2021) 11:635. doi: 10.1038/s41398-021-01742-8
64. Jakobsson J, Bjerke M, Sahebi S, Isgren A, Ekman CJ, Sellgren C, et al. Monocyte and microglial activation in patients with mood-stabilized bipolar disorder. J Psychiatry Neurosci. (2015) 40:250–8. doi: 10.1503/jpn.140183
65. Barichello T, Giridharan VV, Bhatti G, Sayana P, Doifode T, Macedo D, et al. Inflammation as a mechanism of bipolar disorder neuroprogression. Curr Top Behav Neurosci. (2021) 48:215–37. doi: 10.1007/7854_2020_173
66. Goldsmith DR, Bekhbat M, Mehta ND, Felger JC. Inflammation-related functional and structural dysconnectivity as a pathway to psychopathology. Biol Psychiatry. (2023) 93:405–18. doi: 10.1016/j.biopsych.2022.11.003
67. Zhao NO, Topolski N, Tusconi M, Salarda EM, Busby CW, Lima C, et al. Blood-brain barrier dysfunction in bipolar disorder: molecular mechanisms and clinical implications. Brain Behav Immun Health. (2022) 21:100441. doi: 10.1016/j.bbih.2022.100441
68. Pape K, Tamouza R, Leboyer M, Zipp F. Immunoneuropsychiatry - novel perspectives on brain disorders. Nat Rev Neurol. (2019) 15:317–28. doi: 10.1038/s41582-019-0174-4
69. Rosenblat JD, Mcintyre RS. Bipolar disorder and immune dysfunction: epidemiological findings, proposed pathophysiology and clinical implications. Brain Sci. (2017) 7. doi: 10.3390/brainsci7110144
70. Cattane N, Courtin C, Mombelli E, Maj C, Mora C, Etain B, et al. Transcriptomics and mirnomics data integration in lymphoblastoid cells highlights the key role of immune-related functions in lithium treatment response in bipolar disorder. BMC Psychiatry. (2022) 22:665. doi: 10.1186/s12888-022-04286-3
71. Misiak B, Ricceri L, Sasiadek MM. Transposable elements and their epigenetic regulation in mental disorders: current evidence in the field. Front Genet. (2019) 10:580. doi: 10.3389/fgene.2019.00580
Keywords: bipolar disorder, immune cells, Mendelian randomization study, causal association, SNP
Citation: Wang M, Wang S, Yuan G, Gao M, Zhao X, Chu Z and Gao D (2024) Causal role of immune cells in bipolar disorder: a Mendelian randomization study. Front. Psychiatry 15:1411280. doi: 10.3389/fpsyt.2024.1411280
Received: 02 April 2024; Accepted: 30 July 2024;
Published: 16 August 2024.
Edited by:
Yixiao Fu, First Affiliated Hospital of Chongqing Medical University, ChinaReviewed by:
Isaac R. Melamed, IMMUNOe Health and Research Centers, Colorado, United StatesZhijie Han, Chongqing Medical University, China
Copyright © 2024 Wang, Wang, Yuan, Gao, Zhao, Chu and Gao. This is an open-access article distributed under the terms of the Creative Commons Attribution License (CC BY). The use, distribution or reproduction in other forums is permitted, provided the original author(s) and the copyright owner(s) are credited and that the original publication in this journal is cited, in accordance with accepted academic practice. No use, distribution or reproduction is permitted which does not comply with these terms.
*Correspondence: Dongmei Gao, Z2FvZG9uZ21laTc4MDhAc2R1dGNtLmVkdS5jbg==