- 1Affiliated Mental Health Center & Hangzhou Seventh People’s Hospital, Zhejiang University School of Medicine, Hangzhou, Zhejiang, China
- 2School of Psychology, Nanjing Normal University, Nanjing, China
Background: Obsessive-compulsive disorder (OCD) is a chronic psychiatric illness with complex clinical manifestations. Cognitive dysfunction may underlie OC symptoms. The frontoparietal network (FPN) is a key region involved in cognitive control. However, the findings of impaired FPN regions have been inconsistent. We employed meta-analysis to identify the fMRI-specific abnormalities of the FPN in OCD.
Methods: PubMed, Web of Science, Scopus, and EBSCOhost were searched to screen resting-state functional magnetic resonance imaging (rs-fMRI) studies exploring dysfunction in the FPN of OCD patients using three indicators: the amplitude of low-frequency fluctuation/fractional amplitude of low-frequency fluctuation (ALFF/fALFF), regional homogeneity (ReHo) and functional connectivity (FC). We compared all patients with OCD and control group in a primary analysis, and divided the studies by medication in secondary meta-analyses with the activation likelihood estimation (ALE) algorithm.
Results: A total of 31 eligible studies with 1359 OCD patients (756 men) and 1360 healthy controls (733 men) were included in the primary meta-analysis. We concluded specific changes in brain regions of FPN, mainly in the left dorsolateral prefrontal cortex (DLPFC, BA9), left inferior frontal gyrus (IFG, BA47), left superior temporal gyrus (STG, BA38), right posterior cingulate cortex (PCC, BA29), right inferior parietal lobule (IPL, BA40) and bilateral caudate. Additionally, altered connectivity within- and between-FPN were observed in the bilateral DLPFC, right cingulate gyrus and right thalamus. The secondary analyses showed improved convergence relative to the primary analysis.
Conclusion: OCD patients showed dysfunction FPN, including impaired local important nodal brain regions and hypoconnectivity within the FPN (mainly in the bilateral DLPFC), during the resting state. Moreover, FPN appears to interact with the salience network (SN) and default mode network (DMN) through pivotal brain regions. Consistent with the hypothesis of fronto-striatal circuit dysfunction, especially in the dorsal cognitive circuit, these findings provide strong evidence for integrating two pathophysiological models of OCD.
Introduction
Obsessive–compulsive disorder (OCD) is a common and destructive disorder with a lifetime prevalence of 1%-3% (1), characterized by intrusive thoughts (obsessions) and mental or physical ritualistic behaviors (compulsions). Cognitive rigidity is an important feature of OCD. Studies have shown that impaired cognitive flexibility is one of the core cognitive bases of OCD, which is closely associated with the dysfunction of the frontoparietal network (FPN) (2, 3). The FPN flexibly helps respond to stimuli or external tasks, and drives rapid, appropriate and purposeful coordinated behavior, which plays an important role in cognitive control (4, 5). Many studies have paid attention to functional alterations in the FPN in OCD using the resting-state functional magnetic resonance imaging (rs-fMRI), but consistent conclusions have not been reached. Therefore, we aimed to explore the fMRI-specific abnormalities of the FPN in OCD.
Rs-fMRI can detect deficits in disease-related neural activity in patients and is widely used in the study of the neural mechanism of OCD (6). The amplitude of low-frequency fluctuation (ALFF)/fractional amplitude of low-frequency fluctuation (fALFF), regional homogeneity (ReHo) and functional connectivity (FC) are currently the most commonly used methods to describe resting brain function. ALFF/fALFF and ReHo reflect the intensity and regional synchronization of spontaneous neural activity, respectively, providing information about local alterations in brain function (7). FC reflects the collaborative relationship between different brain regions, providing information about the global properties of intrinsically coupled brain networks (8). Thus, those methods obtaining functional information from multiple dimensions can investigate brain networks and understand the functional changes in OCD more comprehensively and deeply.
Previous rs-fMRI studies have reported abnormalities in intrinsic large-scale functional networks including the FPN, salience network (SN) and default mode network (DMN) in OCD patients (9). The FPN, as a key node in the triple network model (FPN, SN and DMN) linking with the classic fronto-striatal circuit, is regarded as the potential core of the pathophysiological mechanism of OCD (10). FPN comprises a wide-spread network including the dorsolateral prefrontal cortex (DLPFC), inferior parietal lobule (IPL) (11), inferior frontal gyrus (IFG) (12), superior temporal gyrus (STG) (13), middle temporal gyrus (MTG), supramarginal gyrus (14), posterior cingulate cortex (PCC, BA29) (15, 16), anterior cingulate cortex (ACC), frontal operculum (FO) and caudate (17). Several rs-fMRI studies have reported FPN hypoconnectivity (18, 19), hyperconnectivity (20–22) or even no significant (23) in patients with OCD compared with healthy controls (HCs). Previous studies on the FPN in OCD have shown inconsistent results. To date, no meta-analysis has focused on FPN of OCD. Certainly, two meta-analyses of seed-based resting-state functional connectivity in OCD have reported dysfunction in a portion of regions in the FPN. Gürsel reported FPN (mainly in the DLPFC) hypoconnectivity within-network and between-network with DMN (10). However, Liu only found dysconnectivity between the striatum and FPN (DLPFC and IPL) (24). A recent study, based on Gürsel’s meta-analysis results, conducted seed-to-voxel FC analyses and found no significant difference between OCD and HC in the FPN (14). Insufficient sample size and uncontrolled medication may have contributed to the observed differences. In addition, we suggested that the previous meta-analysis may be insufficient to summarize the FPN. It is necessitating further meta-analysis in FPN of OCD to quantify the evidence.
Thus, the main objective of the present study was to provide a contemporary, quantitative comparison of specific functional alterations of the FPN in OCD patients and HC by using the ALE algorithm. Further, we aimed to explore the changes within-network and between-networks in the FPN using three indicators (ALFF/fALFF, ReHo and FC). Based on previous knowledge, we hypothesized that (1) the ALFF/fALFF, ReHo and FC of the FPN would show special biomarkers, and (2) specific brain regions within the FPN would interact with other networks.
Methods
Literature search and study selection
We searched PubMed, Web of Science, Scopus, and EBSCOhost for studies in peer-reviewed journals through December 3rd, 2023. The search keywords were (obsessive–compulsive disorder or OCD) AND (functional magnetic resonance imaging or resting state) AND (functional connectivity or FC) AND (frontoparietal network) (2), (obsessive–compulsive disorder or OCD) AND (functional magnetic resonance imaging or resting state) AND (regional homogeneity or ReHo) (3), (obsessive–compulsive disorder or OCD) AND (functional magnetic resonance imaging or resting state) AND (amplitude of low-frequency fluctuations or ALFF or fractional amplitude of low-frequency fluctuations or fALFF).
The inclusion criteria were (1) reported comparisons of adult OCD patients and healthy controls (HC); (2) included an analysis of the FPN in the resting state;(3) employed whole brain analysis; (3) reported the peak coordinates of FPN regions in standard Montreal Neurological Institute (MNI) or Talairach space; and (4) published in English in peer-reviewed journals. Studies focused on pediatric populations, restricted to region of interest (ROI), did not report related peak coordinates were excluded. The reviews, meta-analyses and case reports were also excluded. If peak coordinates were not reported in the paper, we attempt to obtain them by contacting the corresponding author via email. And if there is overlap in the samples, the study with the larger sample size will be included in the meta-analysis.
Based on a preregistered protocol (PROSPERO CRD42023479734), this meta-analysis was conducted according to the PRISMA statement guidelines.
Quality assessment and data extraction
The quality of each included study was assessed using a 12-point checklist (25) based on the reported demographic and clinical characteristics of the participants, as well as imaging methodology. Any study with a score >6.0 was included in the meta-analysis. More details are provided in the Supplementary Material (Supplementary Table S1).
For eligible study, clinical features (e.g. the sample size, mean age, sex composition, education level, mean age of onset, mean illness duration, mean total Yale-Brown Obsessive-Compulsive Scale (Y-BOCS) score, medication status and comorbidity) and neuroimaging characteristics (e.g. fMRI method, statistical threshold) were recorded. Then, the activity coordinates of the abnormal brain regions in FPN were used as inputs for GingerALE (26). Two researchers (JP. Y. and QW.X) independently conducted literature searches, study selection, data extraction and quality assessment. Any discrepancies were discussed with another author (C.Z.) to be mediated.
Primary and secondary meta-analysis
The studies that met the inclusion criteria were all included in the primary meta-analysis, which explored characteristic changes in the FPN in patients with OCD. Furthermore, we were concerned about the impact of drugs on the results, prompting us to conduct secondary meta-analyses to evaluate potential effects in a drug-free group. Due to the limited number of included articles, we only included the ALFF and ReHO (OCD>HC) papers in the subgroup analysis.
ALE meta-analysis
Voxel-based meta-analysis was performed using the GingerALE 3.0.2 software (http://www.brainmap.org), which is widely used in neuroimaging meta-analyses (27). Activation likelihood estimation (ALE) was used to assess the spatial convergence of the differences in ReHo, ALFF/fALFF and FC brain activity between OCD and HCs by analyzing the foci across studies using a random effects model. The extracted data was collated into six text files and entered into the ALE algorithm. The ALE algorithm considers the foci in text files as the spatial centers of the 3D Gaussian probability distribution and obtains the full-width half-maximum for the Gaussian distribution based on the sample size (27). Then, the ALE generated modeled activation (MA) maps and calculated ALE scores (28). The present study was conducted using a cluster-level inference corrected threshold of p < 0.05 (cluster-forming voxel p < 0.01, uncorrected) (29). The cluster-level inference corrected threshold sets the cluster minimum volume so that, for example, at a cluster-level inference threshold of 0.05, only 5% of the simulated data’s clusters exceed this size. Then, the DPABI software (http://fmri.org/dpabi) was used to visualize the ALE results. Finally, a jackknife sensitivity analysis was conducted by repeating the main analysis n times (where n equals the number of datasets included). In this analysis, one study at a time was excluded to assess if the results remained significant, thus evaluating the robustness of the results. However, due to the limited number of included studies, sensitivity analysis was only performed for the main meta-analysis.
Results
Studies included in meta-analysis
A total of 31 eligible studies comprising ALFF/fALFF 18 experiments, 1560 subjects and 59 foci; ReHo 14 experiments, 977 subjects and 63 foci; FC 12 experiments, 1149 subjects and 43 foci were included in the primary meta-analysis (Table 1). Six out of the 31 studies provided two experiments each. These studies typically contained two OCD subgroups, matched with only one HC group (40, 42, 44, 52–54). A total of 22 eligible studies comprising ALFF/fALFF 15 experiments, 1358 subjects and 50 foci; ReHo 9 experiments, 726 subjects and 29 foci were included in the secondary meta-analyses. The process of retrieval and selection is illustrated in the flowchart (Figure 1).
ALE meta-analysis results
We found specific changes in brain regions of FPN in OCD patients as compared to HC, mainly in the left dorsolateral prefrontal cortex (DLPFC, BA9), left inferior frontal gyrus (IFG, BA47), left superior temporal gyrus (STG, BA38), right posterior cingulate cortex (PCC, BA29), right inferior parietal lobule (IPL, BA40) and bilateral caudate. Additionally, altered connectivity within- and between-FPN were observed in the bilateral DLPFC, right cingulate gyrus and right thalamus.
Altered ALFF/fALFF in OCD
The primary meta-analysis of the 15 studies revealed two clusters with a significant likelihood of higher activation (14 experiments comprising 26 foci and 1315 subjects) and a single cluster showing lower activation or “deactivation” (14 experiments comprising 33 foci and 1181 subjects). Compared with HC, OCD patients exhibited increased ALFF/fALFF in the left medial frontal gyrus (MFG, BA9 and 10), left inferior frontal gyrus (IFG, BA47), left superior temporal gyrus (STG, BA38) and left insula. Conversely, patients with OCD showed decreased ALFF/fALFF in the right inferior parietal lobule (IPL, BA40) and right precuneus (BA7) (Table 2; Figure 2).
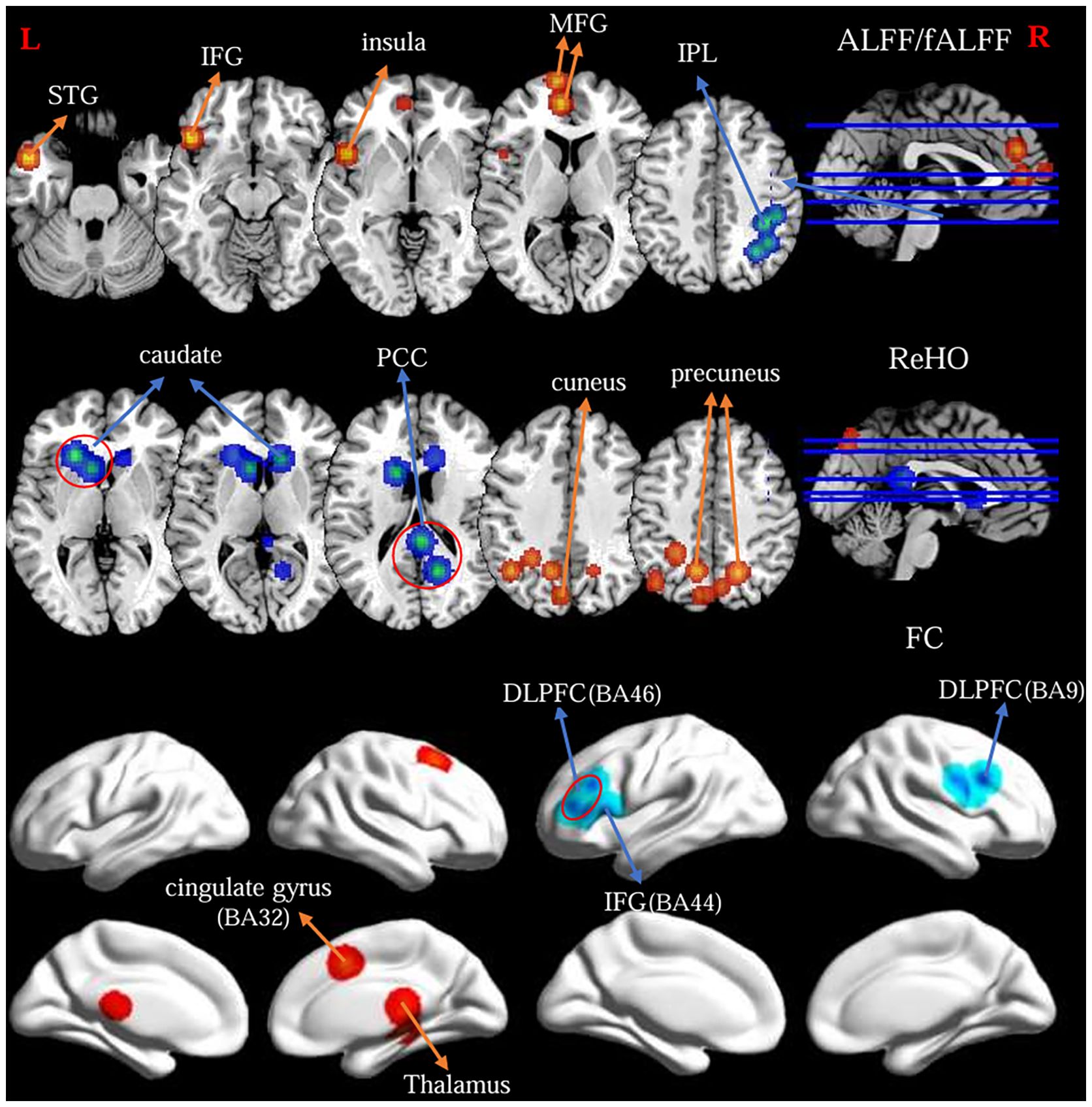
Figure 2 Findings from primary meta-analysis of the fMRI-specific differences of the FPN between OCD and HC. Warm/cool colors indicate regions showing activation/hyperconnectivity (or OCD > HC) and deactivation/hypoconnectivity (or OCD < HC), respectively.
The secondary meta-analyses of the 13 studies identified two clusters with a significant likelihood of higher activation (12 experiments comprising 23 foci and 1156 subjects) and two clusters showing lower activation or “deactivation” (10 experiments comprising 27 foci and 937 subjects). In addition to replicating the primary analysis results, the secondary analysis also revealed that OCD showed decreased ALFF/fALFF in the left insula(BA13) and left STG (BA41) compared to HC (Table 3).
Altered ReHO in OCD
The primary meta-analysis of the 11 studies revealed a single cluster showing a significant likelihood of higher activation (13 experiments comprising 47 foci and 945 subjects) and two clusters indicating lower activation or “deactivation” (7 experiments comprising 16 foci and 311 subjects). OCD patients exhibited increased ReHo in the bilateral precuneus (BA7) and left cuneus (B19). Besides, patients with OCD showed decreased ReHo in bilateral caudate body, left caudate head and right posterior cingulate cortex (BA29 and 30) (Table 2; Figure 2).
The secondary meta-analyses of the 8 studies comprising 9 experiments, 726 subjects and 29 foci did not identified clusters (Table 3).
Altered FC in OCD
ALE meta-analysis of the 11 studies revealed three clusters with a significant likelihood of strengthened connectivity (8 experiments comprising 18 foci and 725 subjects) and four clusters of weakened connectivity (7 experiments comprising 25 foci and 654 subjects). The results showed increased FC in the right cingulate gyrus (BA32), right thalamus. Differently, patients with OCD showed decreased FC of the FPN in the bilateral dorsolateral prefrontal cortex (DLPFC, BA9 and 46), left inferior frontal gyrus (IFG, BA44) and right precentral gyrus (BA9) (Table 2; Figure 2).
Jackknife sensitivity analysis
The jackknife sensitivity analysis showed that the decreased FC in the left DLPFC/IFG, the increased ReHo in the left precuneus and the decreased ReHo in the left caudate were the most robust and replicable data. Moreover, the increased FC in the right cingulate gyrus remained highly replicable (Table 2). More details are provided in the Supplementary Material (Supplementary Table S2).
Discussion
Our meta-analysis was the first to assess the functional integrity of the FPN in OCD using fMRI across various dimensions (ALFF/fALFF, ReHo and FC). We found that hypoconnectivity in the bilateral dorsolateral prefrontal cortex (DLPFC, BA9 and 46) is the most central feature of the FPN. Moreover, the dysfunction between FPN, fronto-striatal circuit, DMN and SN in OCD was reported. Further, we discussed the main findings.
Dorsal striatum
In meta-analysis, the bilateral caudate body and left caudate head showed decreased ReHo in OCD compared to HC. This finding supports the important role of dorsal striatal dysfunction in the FPN of OCD. The caudate nucleus projects to the thalamus and is regulated by DLPFC, and together they form the dorsal cognitive circuit involved in executive functions and top-down control of emotional and motor processes (56). Abnormal activity in the caudate nucleus has been consistently reported in previous studies of OCD (57). It is associated with cognitive functions, such as response inhibition and flexibility (58), and emotion regulation (59). A recent rs-fMRI meta-analysis demonstrated lower left caudate activation in OCD vs. HC (60). This finding was consistent with our study, indicating reduced flexibility of response in OCD patients. Furthermore, hypoactivation of the right caudate body was observed in OCD during executive functioning tasks (61). Several others meta-analysis have also reported that hypoactivation of the bilateral caudate in inhibitory control (62) and the left caudate body in symptom provocation that elicits intense negative emotions (63). Thus, caudate abnormality appears to be a specific feature of OCD. We suggested that the decreased ReHo in the bilateral caudate body and left caudate head indicates poor coordination of local neural activity, which may be related to dysregulated emotion and behavior in OCD and plays a significant role in the pathophysiology of OCD.
Thalamus
The increased FC in the right thalamus was reported in OCD patients relative to HC. The thalamus, as a relay station, plays a crucial role in fronto-striatal circuit of OCD. It integrates incoming sensory information from the basal ganglia, especially in the caudate nucleus, with higher cortical (DLPFC) functions, and participates in cognitive and motor functions (64). Inefficient thalamic gating, caused by the dysfunction of the caudate nucleus, leads to hyperactivation of the DLPFC (corresponding to intrusive thoughts) and ACC (associated with non-specific anxiety) (65). Previous studies have reported increased FC in the thalamus at rest (66, 67). The increased FC of the thalamus is often interpreted as a compensatory brain activity that activates connected brain regions and enhances the ability of information integration (68). Recent studies have also shown increased FC between the regions of the FPN (DLPFC) and the thalamus in OCD and their first-degree relatives, in line with the findings in our meta-analysis. It has been proposed that this may be a candidate endophenotype markers of OCD (20).
Dorsolateral prefrontal cortex (DLPFC)
Our meta-analysis revealed a decreased FC of the FPN in the bilateral DLPFC (BA9 and 46) in OCD. The DLPFC is involved in executive functions and emotion regulation (69). It is a key node in the FPN involved in cognitive control and the fronto-striatal loop in habitual behavior (70, 71). The decreased FC in the bilateral DLPFC may indicate a top-down disruption between these networks and underlie the pathophysiology and clinical symptoms of OCD (72). Likewise, increased ALFF/fALFF in BA9 was also reported in OCD during rest in our results. This may be related to caudate hypofunction and thalamic gating dysfunctions, as mentioned previously. In addition, hyperactivation of the left BA9 may be observed as a compensatory activation that compensates for the dysregulation by recruiting cortical areas. The larger range of the frontal cortex, including the left MFG (BA9 and 10) and the left IFG (BA47), showed higher activation in OCD patients relative to HC in our study. OCD struggle to control compulsive thoughts, leading to heightened vigilance. This can overwhelm the executive system, deplete cognitive resources, worse cognitive impairment and emotional dysregulation, and trigger compulsive behaviors (64). This result is consistent with the finding that electrical and magnetic stimulation of the left DLPFC appeared to have clinical efficacy for treatment-resistant OCD (73).
Cingulate cortex
The cingulate cortex, as part of the limbic system, is usually divided into the anterior region (ACC) and the posterior region (PCC), which have different functions and are implicated in the pathophysiology of OCD (74). The ACC is involved in executive control and PCC in evaluative functions and human awareness. Specifically, the ACC monitors self-generated threats by scanning the internal mental environment, whereas the PCC scans the external environment to monitor for environmental threats (75–77). Previous fMRI studies have reported ACC hyperactivation (31, 78) and PCC hypoactivation (49, 79) in OCD at rest, consistent with the observation of decreased ReHo in the right PCC (BA29 and 30) in our meta-analysis. Hypoactivation of the right PCC may be associated with diminished perception and attention to external events, which might explain the symptoms of intrusive thoughts and repetitive behavior in OCD (50). However, the higher ACC activation was not observed in our results, and we speculate that this may be related to the fact that some OCD patients are taking medication. As previously reported, treatment may reduce ACC spontaneous activity (80, 81).
However, ALE revealed increased FC in the right cingulate gyrus (BA32) in OCD than in HC. Previous studies have shown that the fronto-cingulate system plays a key role in error detection and control. Increased FC between the ACC and DLPFC is thought to be related to abnormal error processing in OCD patients (82). Other studies have shown that increased FC in the ACC was correlated with symptom severity in OCD patients (32). Hence, the current findings emphasize the important role of the ACC in cognitive control and further support the role of neural activity in the right cingulate cortex in the core deficits of OCD.
Interactive neural network
Based on ALE results, we found characteristic alterations in the FPN of OCD, as well as underlying brain regions that interact with other networks, such as the DMN, SN and fronto-striatal loop. The triple network model and classical fronto-striatal circuit may underlie the pathophysiology of OCD (83, 84).Furthmore, the mate-analysis emphasizes the importance of the FPN in OCD pathology.
The dysfunction of the bilateral DLPFC (BA9 and 46), left IFG (BA47), right PCC (BA29), left STG, right IPL and bilateral caudate was reported in the FPN of OCD at rest. The FPN is a flexible cognitive control center that typically regulates and adjusts behavior in a goal-directed manner during both resting and task states (85). The IFG is the core system for goal-directed tasks, involved in response inhibition (86). The STG is associated with social cognition, particularly involving interpretation/speculation about the intentions or goal-directed behaviors of others (42, 87). The IPL is associated with attentional set shifting and response inhibition (88). Previous studies have suggested that hypoactivation of the IPL perhaps reflect impaired attention to stop signals in OCD, leading to compulsive behavior (48). These important nodes of the FPN exhibited varying degrees of dysfunction; specifically, reduced FC in the DLPFC, the core brain region of the FPN, may suggest indicate ineffective connections within the FPN. The abnormal connectivity pattern of the FPN is related to dysfunction of cognitive control (89). Moreover, a rs-fMRI study on OCD reported that the connectivity within-FPN is negatively associated with disease severity (22). It further emphasizes the important role this network plays in pathophysiology and clinical manifestations of OCD.
ALE showed greater FC in the right cingulate gyrus (BA32) in OCD in results of FC. The ACC is core region of the SN (45). Thus, this finding may indicate a stronger functional connectivity between the FPN and SN. Together, they form a “task-positive” system that supports cognitive control (71). We speculate that this may indicate inefficiency of the cognitive control networks in OCD patients due to the hypoconnectivity within FPN. Increased connectivity is considered a complementary mechanism (90) that enhances cognitive ability and maintains normal function. Generally, intrusive thoughts are more likely to occur when the mind is not engaged in cognitively demanding tasks, such as during rest (91, 92). Hence, OCD patients may experience more cognitive and emotional challenges during relaxation time, needing to suppress OC symptoms and anxious emotions in the resting state. The atypical recruitment of the “task-positive” system may help OCD patients control these thoughts and related avoidance behaviors (93). Besides, we also found higher right insula activation during the resting state in OCD from meta results. The insula is another core region of the SN, which is involved in detecting salient stimuli (94). The hyperactive insula may be linked to a stronger ability to perceive error-related signals, which can help patients with OCD to avoid making more mistakes (3).
The DMN is a significant large-scale intrinsic network involved in self-referential thoughts, mind-wandering and internal processes (95). The alterations of DMN function may be thought to underlie the intrusive thoughts and anxiety experienced in OCD patients (93). As the task-negative network, the DMN interacts reciprocally with the task-positive system (96). In our results, we did not directly find abnormal FC between FPN and DMN. However, local functional abnormalities in DMN core nodes (97), including MFG, PCC and precuneus, associated with the FPN were observed. There are also overlapping areas of the brain between the FPN (MFC, BA 9; PCC, BA29) and the DMN (MFC, BA10; PCC, BA30) in structure. We suggest that the FPN may directly influence DMN activity through these overlapping brain regions. Previous fMRI studies have confirmed that real-time regulation of the PCC can lead to change in activity in other brain regions and functional alterations of the DMN (98). Further, the FC between the FPN and DMN for OCD remains to be investigated.
The hypoconnectivity within the FPN, the better connectivity between FPN and SN, and the potential interaction between FPN and DMN were observed during the resting state in our study. Treatment may alter the connectivity within- and between-FPN in OCD. Some studies have reported that OCD patients showed significantly increased connectivity within the FPN after undergoing exposure and response prevention (ERP) therapy (71) and pharmacological (99) treatment. The greater connectivity within the FPN can enhance cognitive control functions (100). Some studies focusing on patients with attention deficit hyperactivity disorder (ADHD) found increased connectivity between the FPN and SN (101). This change in connectivity plays an important role in helping patients implements cognitive control. ADHD patients can be treated with working memory training to modulate widespread FPN and SN areas (102). However, the functional changes between FPN and SN after treatment in OCD need to be further studied. In addition, the decreased connectivity between FPN and DMN was observed in OCD following ERP treatment (71). This may be related to the fact that patients must repeatedly engage in cognitive control processes to resist compulsive impulses during treatment. Other studies have shown that the connectivity between DMN and FPN significantly predicted response to ERP (103).
In summary, the atypical recruitment of the FPN appears to couple with abnormal activity in the SN and DMN, resulting in impaired cognitive performance in OCD patients. Notably, the functional specific alterations of the FPN findings match the structures of the fronto-striatal circuit, especially in the dorsal cognitive circuit (DLPFC-caudate-thalamus) (56). The imbalance of functional activity within these two systems (Figure 3), the triple network model and the dorsal cognitive circuit, may reflect the essence of the pathophysiological of OCD and underlie the OC symptoms.
Limitations
This study has several limitations. First, the number of studies was insufficient for symptom subtype analysis. Second, although we conducted subgroup analyses of drug-free OCD patients, the number of studies was limited, and it was still not possible to accurately assess the potential impact of the drug on the likelihood of activation. Future studies should increase the sample size, distinguish the symptom subtypes, and control for the influence of medication and comorbidity, which may facilitate a more thorough exploration of pathogenesis of OCD. Finally, we were unable to perform correlation analysis and confidence interval analysis due to the limitation of the ALE method.
Conclusions
We conducted a meta-analysis to examine specific functional alterations of FPN in OCD patients compared to HC. The study observed the functional impairment and potential compensation mechanisms of the FPN. We concluded that OCD patients showed local brain functional changes, including higher activation in the left DLPFC (BA9), left IFG and left STG, as well as lower activation in the right IPL, right PCC (BA29) and bilateral caudate. Additionally, there was hypoconnectivity within the FPN, particularly in the bilateral DLPFC. And FPN appears to couple with the SN and DMN through pivotal brain regions, including right cingulate gyrus, left MFC, and right PCC. Moreover, these impaired brain areas overlap with the classic fronto-striatal circuit, especially in the dorsal cognitive circuit. Thus, two pathophysiological models of OCD could be integrated into a common framework to explain core OC symptoms.
Author contributions
JY: Conceptualization, Data curation, Formal analysis, Methodology, Software, Visualization, Writing – original draft. QX: Data curation, Validation, Writing – review & editing. LM: Formal analysis, Writing – review & editing. YH: Formal analysis, Writing – review & editing. WZ: Supervision, Writing – review & editing. YL: Supervision, Writing – review & editing. YW: Formal analysis, Writing – review & editing. WT: Conceptualization, Writing – review & editing. CZ: Data curation, Funding acquisition, Supervision, Writing – review & editing. XJ: Conceptualization, Supervision, Writing – review & editing.
Funding
The author(s) declare financial support was received for the research, authorship, and/or publication of this article. This work is supported by grants from the Medical Health Science and Technology Project of Zhejiang Provincial Health Commission (2022KY993 to CZ).
Conflict of interest
The authors declare that the research was conducted in the absence of any commercial or financial relationships that could be construed as a potential conflict of interest.
Publisher’s note
All claims expressed in this article are solely those of the authors and do not necessarily represent those of their affiliated organizations, or those of the publisher, the editors and the reviewers. Any product that may be evaluated in this article, or claim that may be made by its manufacturer, is not guaranteed or endorsed by the publisher.
Supplementary material
The Supplementary Material for this article can be found online at: https://www.frontiersin.org/articles/10.3389/fpsyt.2024.1401623/full#supplementary-material
References
1. Stein DJ, Costa DLC, Lochner C, Miguel EC, Reddy YCJ, Shavitt RG, et al. Obsessive-compulsive disorder. Nat Rev Dis Primers. (2019) 5:52. doi: 10.1038/s41572–019-0102–3
2. Ramakrishnan S, Robbins TW, Zmigrod L. Cognitive rigidity, habitual tendencies, and obsessive-compulsive symptoms: individual differences and compensatory interactions. Front Psychiatry. (2022) 13:865896. doi: 10.3389/fpsyt.2022.865896
3. Liu Q, Gao F, Wang X, Xia J, Yuan G, Zheng S, et al. Cognitive inflexibility is linked to abnormal frontoparietal-related activation and connectivity in obsessive-compulsive disorder. Hum Brain Mapp. (2023) 44:5460–70. doi: 10.1002/hbm.26457
4. Caldinelli C, Cusack R. The fronto-parietal network is not a flexible hub during naturalistic cognition. Hum Brain Mapp. (2022) 43:750–9. doi: 10.1002/hbm.25684
5. Wood JL, Nee DE. Cingulo-opercular subnetworks motivate frontoparietal subnetworks during distinct cognitive control demands. J neuroscience: Off J Soc Neurosci. (2023) 43:1225–37. doi: 10.1523/jneurosci.1314–22.2022
6. Raimondo L, Oliveira Ĺ AF, Heij J, Priovoulos N, Kundu P, Leoni RF, et al. Advances in resting state fmri acquisitions for functional connectomics. NeuroImage. (2021) 243:118503. doi: 10.1016/j.neuroimage.2021.118503
7. Bu X, Hu X, Zhang L, Li B, Zhou M, Lu L, et al. Investigating the predictive value of different resting-state functional mri parameters in obsessive-compulsive disorder. Trans Psychiatry. (2019) 9:17. doi: 10.1038/s41398–018-0362–9
8. Cao L, Li H, Hu X, Liu J, Gao Y, Liang K, et al. Distinct alterations of amygdala subregional functional connectivity in early- and late-onset obsessive-compulsive disorder. J Affect Disord. (2022) 298:421–30. doi: 10.1016/j.jad.2021.11.005
9. Xu Y, Han S, Wei Y, Zheng R, Cheng J, Zhang Y. Abnormal resting-state effective connectivity in large-scale networks among obsessive-compulsive disorder. psychol Med. (2024) 54:350–8. doi: 10.1017/s0033291723001228
10. Gürsel DA, Avram M, Sorg C, Brandl F, Koch K. Frontoparietal areas link impairments of large-scale intrinsic brain networks with aberrant fronto-striatal interactions in ocd: A meta-analysis of resting-state functional connectivity. Neurosci Biobehav Rev. (2018) 87:151–60. doi: 10.1016/j.neubiorev.2018.01.016
11. Sezer I, Pizzagalli DA, Sacchet MD. Resting-state fmri functional connectivity and mindfulness in clinical and non-clinical contexts: A review and synthesis. Neurosci Biobehav Rev. (2022) 135:104583. doi: 10.1016/j.neubiorev.2022.104583
12. Briend F, Armstrong WP, Kraguljac NV, Keilhloz SD, Lahti AC. Aberrant static and dynamic functional patterns of frontoparietal control network in antipsychotic-naïve first-episode psychosis subjects. Hum Brain Mapp. (2020) 41:2999–3008. doi: 10.1002/hbm.24992
13. Sharma A, Kumar A, Singh S, Bhatia T, Beniwal RP, Khushu S, et al. Altered resting state functional connectivity in early course schizophrenia. Psychiatry Res Neuroimaging. (2018) 271:17–23. doi: 10.1016/j.pscychresns.2017.11.013
14. Geffen T, Smallwood J, Finke C, Olbrich S, Sjoerds Z, Schlagenhauf F. Functional connectivity alterations between default mode network and occipital cortex in patients with obsessive-compulsive disorder (Ocd). NeuroImage Clin. (2022) 33:102915. doi: 10.1016/j.nicl.2021.102915
15. Goodman ZT, Bainter SA, Kornfeld S, Chang C, Nomi JS, Uddin LQ. Whole-brain functional dynamics track depressive symptom severity. Cereb Cortex (New York NY: 1991). (2021) 31:4867–76. doi: 10.1093/cercor/bhab047
16. Di X, Biswal BB. Modulatory interactions between the default mode network and task positive networks in resting-state. PeerJ. (2014) 2:e367. doi: 10.7717/peerj.367
17. Gao Y, Shuai D, Bu X, Hu X, Tang S, Zhang L, et al. Impairments of large-scale functional networks in attention-deficit/hyperactivity disorder: A meta-analysis of resting-state functional connectivity. psychol Med. (2019) 49:2475–85. doi: 10.1017/S003329171900237X
18. Yang X, Hu X, Tang W, Li B, Yang Y, Gong Q, et al. Intrinsic brain abnormalities in drug-naive patients with obsessive-compulsive disorder: A resting-state functional mri study. J Affect Disord. (2019) 245:861–8. doi: 10.1016/j.jad.2018.11.080
19. Jia C, Ou Y, Chen Y, Ma J, Zhan C, Lv D, et al. Disrupted asymmetry of inter- and intra-hemispheric functional connectivity at rest in medication-free obsessive-compulsive disorder. Front Neurosci. (2021) 15:634557. doi: 10.3389/fnins.2021.634557
20. Tomiyama H, Murayama K, Nemoto K, Hasuzawa S, Mizobe T, Kato K, et al. Alterations of default mode and cingulo-opercular salience network and frontostriatal circuit: A candidate endophenotype of obsessive-compulsive disorder. Prog Neuropsychopharmacol Biol Psychiatry. (2022) 116:110516. doi: 10.1016/j.pnpbp.2022.110516
21. Stern ER, Fitzgerald KD, Welsh RC, Abelson JL, Taylor SF. Resting-state functional connectivity between fronto-parietal and default mode networks in obsessive-compulsive disorder. PloS One. (2012) 7:e36356. doi: 10.1371/journal.pone.0036356
22. Göttlich M, Krämer UM, Kordon A, Hohagen F, Zurowski B. Decreased limbic and increased fronto-parietal connectivity in unmedicated patients with obsessive-compulsive disorder. Hum Brain Mapp. (2014) 35:5617–32. doi: 10.1002/hbm.22574
23. de Vries FE, de Wit SJ, van den Heuvel OA, Veltman DJ, Cath DC, van Balkom A, et al. Cognitive control networks in ocd: A resting-state connectivity study in unmedicated patients with obsessive-compulsive disorder and their unaffected relatives. World J Biol psychiatry: Off J World Fed Societies Biol Psychiatry. (2019) 20:230–42. doi: 10.1080/15622975.2017.1353132
24. Liu J, Cao L, Li H, Gao Y, Bu X, Liang K, et al. Abnormal resting-state functional connectivity in patients with obsessive-compulsive disorder: A systematic review and meta-analysis. Neurosci Biobehav Rev. (2022) 135. doi: 10.1016/j.neubiorev.2022.104574
25. Zhu T, Wang Z, Zhou C, Fang X, Huang C, Xie C, et al. Meta-analysis of structural and functional brain abnormalities in schizophrenia with persistent negative symptoms using activation likelihood estimation. Front Psychiatry. (2022) 13:957685. doi: 10.3389/fpsyt.2022.957685
26. Turkeltaub PE, Eickhoff SB, Laird AR, Fox M, Wiener M, Fox P. Minimizing within-Experiment and within-Group Effects in Activation Likelihood Estimation Meta-Analyses. Hum Brain Mapp. (2012) 33:1–13. doi: 10.1002/hbm.21186
27. Gray JP, Müller VI, Eickhoff SB, Fox PT. Multimodal abnormalities of brain structure and function in major depressive disorder: A meta-analysis of neuroimaging studies. Am J Psychiatry. (2020) 177:422–34. doi: 10.1176/appi.ajp.2019.19050560
28. Laird AR, Eickhoff SB, Kurth F, Fox PM, Uecker AM, Turner JA, et al. Ale meta-analysis workflows via the brainmap database: progress towards a probabilistic functional brain atlas. Front neuroinformatics. (2009) 3:23.2009. doi: 10.3389/neuro.11.023.2009
29. Eickhoff SB, Bzdok D, Laird AR, Kurth F, Fox PT. Activation likelihood estimation meta-analysis revisited. NeuroImage. (2012) 59:2349–61. doi: 10.1016/j.neuroimage.2011.09.017
30. Yang T, Cheng Y, Li H, Jiang H, Luo C, Shan B, et al. Abnormal regional homogeneity of drug-naïve obsessive-compulsive patients. Neuroreport. (2010) 21(11):786–90. doi: 10.1097/WNR.0b013e32833cadf0
31. Hou J, Wu W, Lin Y, Wang J, Zhou D, Guo J, et al. Localization of cerebral functional deficits in patients with obsessive-compulsive disorder: A resting-state fmri study. J Affect Disord. (2012) 138:313–21. doi: 10.1016/j.jad.2012.01.022
32. Cheng Y, Xu J, Nie B, Luo C, Yang T, Li H, et al. Abnormal resting-state activities and functional connectivities of the anterior and the posterior cortexes in medication-naïve patients with obsessive-compulsive disorder. PloS One. (2013) 8:e67478. doi: 10.1371/journal.pone.0067478
33. Ping L, Su-Fang L, Hai-Ying H, Zhang-Ye D, Jia L, Zhi-Hua G, et al. Abnormal spontaneous neural activity in obsessive-compulsive disorder: a resting-state functional magnetic resonance imaging study. PloS One. (2013) 8(6):e67262. doi: 10.1371/journal.pone.0067262
34. Yang XY, Sun J, Luo J, Zhong ZX, Li P, Yao SM, et al. Regional Homogeneity of spontaneous brain activity in adult patients with obsessive-compulsive disorder before and after cognitive behavioural therapy. J Affect Disord. (2015) 188:243–51. doi: 10.1016/j.jad.2015.07.048
35. Chen Y, Meng X, Hu Q, Cui H, Ding Y, Kang L, et al. Altered Resting-state functional organization within the central executive network in obsessive-compulsive disorder. Psychiatry Clin Neurosci. (2016) 70(10):448–56. doi: 10.1111/pcn.12419
36. Niu Q, Yang L, Song X, Chu C, Liu H, Zhang L, et al. Abnormal resting-state brain activities in patients with first-episode obsessive-compulsive disorder. Neuropsychiatr Dis Treat. (2017) 13:507–13. doi: 10.2147/ndt.S117510
37. Qiu L, Fu X, Wang S, Tang Q, Chen X, Cheng L, et al. Abnormal regional spontaneous neuronal activity associated with symptom severity in treatment-naive patients with obsessive-compulsive disorder revealed by resting-state functional mri. Neurosci Lett. (2017) 640:99–104. doi: 10.1016/j.neulet.2017.01.024
38. Giménez M, Guinea-Izquierdo A, Villalta-Gil V, Martínez-Zalacaín I, Segalàs C, Subirà M, et al. Brain alterations in low-frequency fluctuations across multiple bands in obsessive compulsive disorder. Brain Imaging Behav. (2017) 11(6):1690–706. doi: 10.1007/s11682-016-9601-y
39. Zhao HZ, Wang CH, Gao ZZ, Ma JD, Huang P, Li HF, et al. Effectiveness of cognitive-coping therapy and alteration of resting-state brain function in obsessive-compulsive disorder. J Affect Disord. (2017) 208:184–90. doi: 10.1016/j.jad.2016.10.015
40. Fan J, Zhong M, Gan J, Liu W, Niu C, Liao H, et al. Spontaneous neural activity in the right superior temporal gyrus and left middle temporal gyrus is associated with insight level in obsessive-compulsive disorder. J Affect Disord. (2017) 207:203–11. doi: 10.1016/j.jad.2016.08.027
41. Li K, Zhang H, Yang Y, Zhu J, Wang B, Shi Y, et al. Abnormal functional network of the thalamic subregions in adult patients with obsessive-compulsive disorder. Behav Brain Res. (2019) 371:111982. doi: 10.1016/j.bbr.2019.111982
42. Xia J, Fan J, Du H, Liu W, Li S, Zhu J, et al. Abnormal spontaneous neural activity in the medial prefrontal cortex and right superior temporal gyrus correlates with anhedonia severity in obsessive-compulsive disorder. J Affect Disord. (2019) 259:47–55. doi: 10.1016/j.jad.2019.08.019
43. Hu X, Zhang L, Bu X, Li H, Li B, Tang W, et al. Localized connectivity in obsessive-compulsive disorder: an investigation combining univariate and multivariate pattern analyses. Front Behav Neurosci. (2019) 13:122. doi: 10.3389/fnbeh.2019.00122
44. Xia J, Fan J, Liu W, Du H, Zhu J, Yi J, et al. Functional connectivity within the salience network differentiates autogenous- from reactive-type obsessive-compulsive disorder. Prog Neuropsychopharmacol Biol Psychiatry. (2020) 98:109813. doi: 10.1016/j.pnpbp.2019.109813
45. Gürsel DA, Reinholz L, Bremer B, Schmitz-Koep B, Franzmeier N, Avram M, et al. Frontoparietal and salience network alterations in obsessive–compulsive disorder: insights from independent component and sliding time window analyses. J Psychiatry neuroscience: JPN. (2020) 45:214–21. doi: 10.1503/jpn.190038
46. Long J, Luo L, Guo Y, You W, Li Q, Li B, et al. Altered Spontaneous activity and effective connectivity of the anterior cingulate cortex in obsessive–compulsive disorder. J Comp Neurol. (2021) 529(2):296–310. doi: 10.1002/cne.24948
47. Yu XM, Qiu LL, Huang HX, Zuo X, Zhou ZH, Wang S, et al. Comparison of resting-state spontaneous brain activity between treatment-naive schizophrenia and obsessive-compulsive disorder. BMC Psychiatry. (2021) 21(1):544. doi: 10.1186/s12888-021-03554-y
48. Liu J, Bu X, Hu X, Li H, Cao L, Gao Y, et al. Temporal variability of regional intrinsic neural activity in drug-naïve patients with obsessive-compulsive disorder. Hum Brain Mapp. (2021) 42:3792–803. doi: 10.1002/hbm.25465
49. Zhang Y, Liao J, Li Q, Zhang X, Liu L, Yan J, et al. Altered resting-state brain activity in schizophrenia and obsessive-compulsive disorder compared with non-psychiatric controls: commonalities and distinctions across disorders. Front Psychiatry. (2021) 12:681701. doi: 10.3389/fpsyt.2021.681701
50. Yan H, Shan X, Li H, Liu F, Guo W. Abnormal spontaneous neural activity as a potential predictor of early treatment response in patients with obsessive-compulsive disorder. J Affect Disord. (2022) 309:27–36. doi: 10.1016/j.jad.2022.04.125
51. Han S, Xu Y, Guo HR, Fang K, Wei Y, Liu L, et al. Two Distinct Subtypes of Obsessive Compulsive Disorder Revealed by a Framework Integrating Multimodal Neuroimaging Information. Human brain mapping. (2022) 43(14):4254–65. doi: 10.1002/hbm.25951
52. Yu J, Xie M, Song S, Zhou P, Yuan F, Ouyang M, et al. Functional connectivity within the frontal-striatal network differentiates checkers from washers of obsessive-compulsive disorder. Brain Sci. (2022) 12. doi: 10.3390/brainsci12080998
53. Ma Y, Zhao Q, Xu T, Wang P, Gu Q, Wang Z. Resting state functional brain imaging in obsessive-compulsive disorder across genders. World J Biol psychiatry: Off J World Fed Societies Biol Psychiatry. (2022) 23:191–200. doi: 10.1080/15622975.2021.1938669
54. Yuan X, Zhu Y, Xiao L, Chuan Liu Z, Zou J, Hu Z, et al. Regional homogeneity in patients with obsessive–compulsive disorder and depression: A resting state functional magnetic resonance imaging study. Neurosci Lett. (2023) 817. doi: 10.1016/j.neulet.2023.137528
55. Wu X, Yang Q, Xu C, Huo H, Seger CA, Peng Z, et al. Connectome-based predictive modeling of compulsion in obsessive-compulsive disorder. Cereb Cortex (New York NY 1991). (2023) 33(4):1412–25. doi: 10.1093/cercor/bhac145
56. Shephard E, Stern ER, van den Heuvel OA, Costa DLC, Batistuzzo MC, Godoy PBG, et al. Toward a neurocircuit-based taxonomy to guide treatment of obsessive-compulsive disorder. Mol Psychiatry. (2021) 26:4583–604. doi: 10.1038/s41380–020-01007–8
57. Yun JY, Boedhoe PSW, Vriend C, Jahanshad N, Abe Y, Ameis SH, et al. Brain structural covariance networks in obsessive-compulsive disorder: A graph analysis from the enigma consortium. Brain: J Neurol. (2020) 143:684–700. doi: 10.1093/brain/awaa001
58. Tomiyama H, Nakao T, Murayama K, Nemoto K, Ikari K, Yamada S, et al. Dysfunction between dorsal caudate and salience network associated with impaired cognitive flexibility in obsessive-compulsive disorder: A resting-state fmri study. NeuroImage Clin. (2019) 24:102004. doi: 10.1016/j.nicl.2019.102004
59. Fournier JC, Bertocci M, Ladouceur CD, Bonar L, Monk K, Abdul-Waalee H, et al. Neural function during emotion regulation and future depressive symptoms in youth at risk for affective disorders. Neuropsychopharmacology: Off Publ Am Coll Neuropsychopharmacol. (2021) 46:1340–7. doi: 10.1038/s41386-021-01001-w
60. Li H, Wang Y, Xi H, Zhang J, Zhao M, Jia X. Alterations of regional spontaneous brain activity in obsessive-compulsive disorders: A meta-analysis. J Psychiatr Res. (2023) 165:325–35. doi: 10.1016/j.jpsychires.2023.07.036
61. Del Casale A, Rapinesi C, Kotzalidis GD, De Rossi P, Curto M, Janiri D, et al. Executive functions in obsessive-compulsive disorder: an activation likelihood estimate meta-analysis of fmri studies. World J Biol psychiatry: Off J World Fed Societies Biol Psychiatry. (2016) 17:378–93. doi: 10.3109/15622975.2015.1102323
62. Norman LJ, Taylor SF, Liu Y, Radua J, Chye Y, De Wit SJ, et al. Error processing and inhibitory control in obsessive-compulsive disorder: A meta-analysis using statistical parametric maps. Biol Psychiatry. (2019) 85:713–25. doi: 10.1016/j.biopsych.2018.11.010
63. Yu J, Zhou P, Yuan S, Wu Y, Wang C, Zhang N, et al. Symptom provocation in obsessive-compulsive disorder: A voxel-based meta-analysis and meta-analytic connectivity modeling. J Psychiatr Res. (2022) 146:125–34. doi: 10.1016/j.jpsychires.2021.12.029
64. Friedlander L, Desrocher M. Neuroimaging studies of obsessive-compulsive disorder in adults and children. Clin Psychol Rev. (2006) 26:32–49. doi: 10.1016/j.cpr.2005.06.010
65. Del Casale A, Kotzalidis GD, Rapinesi C, Serata D, Ambrosi E, Simonetti A, et al. Functional neuroimaging in obsessive-compulsive disorder. Neuropsychobiology. (2011) 64:61–85. doi: 10.1159/000325223
66. Fajnerova I, Gregus D, Francova A, Noskova E, Koprivova J, Stopkova P, et al. Functional connectivity changes in obsessive-compulsive disorder correspond to interference control and obsessions severity. Front Neurol. (2020) 11:568. doi: 10.3389/fneur.2020.00568
67. Moreira PS, Marques P, Soriano-Mas C, Magalhães R, Sousa N, Soares JM, et al. The neural correlates of obsessive-compulsive disorder: A multimodal perspective. Trans Psychiatry. (2017) 7:e1224. doi: 10.1038/tp.2017.189
68. Lv D, Ou Y, Wang Y, Ma J, Zhan C, Yang R, et al. Altered functional connectivity strength at rest in medication-free obsessive-compulsive disorder. Neural plasticity. (2021) 2021:3741104. doi: 10.1155/2021/3741104
69. Picó-Pérez M, Moreira PS, de Melo Ferreira V, Radua J, Mataix-Cols D, Sousa N, et al. Modality-specific overlaps in brain structure and function in obsessive-compulsive disorder: multimodal meta-analysis of case-control mri studies. Neurosci Biobehav Rev. (2020) 112:83–94. doi: 10.1016/j.neubiorev.2020.01.033
70. Kasal MI, Besiroglu L, Zorlu N, Dikmeer N, Bilge A, Durmaz E, et al. Fronto-striatal structures related with model-based control as an endophenotype for obsessive-compulsive disorder. Sci Rep. (2021) 11:11951. doi: 10.1038/s41598–021-91179–2
71. Becker HC, Beltz AM, Himle JA, Abelson JL, Block SR, Taylor SF, et al. Changes in brain network connections after exposure and response prevention therapy for obsessive-compulsive disorder in adolescents and adults. Biol Psychiatry Cogn Neurosci Neuroimaging. (2023). doi: 10.1016/j.bpsc.2023.09.009
72. Li H, Hu X, Gao Y, Cao L, Zhang L, Bu X, et al. Neural primacy of the dorsolateral prefrontal cortex in patients with obsessive-compulsive disorder. NeuroImage Clin. (2020) 28:102432. doi: 10.1016/j.nicl.2020.102432
73. Jahanbakhsh G, Alireza Haji Seyed Javadi S, Majidi M, Khademi M, Karimi R. Effectiveness of adjunctive low-frequency repetitive transcranial magnetic stimulation therapy over the left dorsolateral prefrontal cortex in patients with obsessive-compulsive disorder refractory to medical treatment:A double-blind, randomized clinical trial. Asian J Psychiatry. (2023) 80:103384. doi: 10.1016/j.ajp.2022.103384
74. Peng Z, Lui SS, Cheung EF, Jin Z, Miao G, Jing J, et al. Brain structural abnormalities in obsessive-compulsive disorder: converging evidence from white matter and grey matter. Asian J Psychiatry. (2012) 5:290–6. doi: 10.1016/j.ajp.2012.07.004
75. Seamans JK, Floresco SB. Event-based control of autonomic and emotional states by the anterior cingulate cortex. Neurosci Biobehav Rev. (2022) 133:104503. doi: 10.1016/j.neubiorev.2021.12.026
76. Fiddick L. There is more than the amygdala: potential threat assessment in the cingulate cortex. Neurosci Biobehav Rev. (2011) 35:1007–18. doi: 10.1016/j.neubiorev.2010.09.014
77. Salvato G, Berlingeri M, De Maio G, Curto F, Chieregato A, Magnani FG, et al. Autonomic responses to emotional linguistic stimuli and amplitude of low-frequency fluctuations predict outcome after severe brain injury. NeuroImage Clin. (2020) 28:102356. doi: 10.1016/j.nicl.2020.102356
78. Yang Z, Xiao S, Su T, Gong J, Qi Z, Chen G, et al. A multimodal meta-analysis of regional functional and structural brain abnormalities in obsessive-compulsive disorder. Eur Arch Psychiatry Clin Neurosci. (2023). doi: 10.1007/s00406–023-01594-x
79. Xu Y, Guo H, Zheng R, Wei Y, Wen B, Fang K, et al. Decreased intrinsic neural timescales in obsessive compulsive disorder and two distinct subtypes revealed by heterogeneity through discriminative analysis. J Affect Disord. (2023) 340:667–74. doi: 10.1016/j.jad.2023.07.112
80. Perani D, Colombo C, Bressi S, Bonfanti A, Grassi F, Scarone S, et al. [18f]Fdg pet study in obsessive-compulsive disorder. A clinical/metabolic correlation study after treatment. Br J psychiatry: J Ment Sci. (1995) 166:244–50. doi: 10.1192/bjp.166.2.244
81. Poli A, Pozza A, Orrù G, Conversano C, Ciacchini R, Pugi D, et al. Neurobiological outcomes of cognitive behavioral therapy for obsessive-compulsive disorder: A systematic review. Front Psychiatry. (2022) 13:1063116. doi: 10.3389/fpsyt.2022.1063116
82. Schlösser RG, Wagner G, Schachtzabel C, Peikert G, Koch K, Reichenbach JR, et al. Fronto-cingulate effective connectivity in obsessive compulsive disorder: A study with fmri and dynamic causal modeling. Hum Brain Mapp. (2010) 31:1834–50. doi: 10.1002/hbm.20980
83. Lee SW, Kim E, Jang TY, Choi H, Kim S, Song H, et al. Alterations of power spectral density in salience network during thought-action fusion induction paradigm in obsessive-compulsive disorder. Clin Psychopharmacol neuroscience: Off Sci J Korean Coll Neuropsychopharmacol. (2022) 20:415–26. doi: 10.9758/cpn.2022.20.3.415
84. Bora E. Meta-analysis of neurocognitive deficits in unaffected relatives of obsessive-compulsive disorder (Ocd): comparison with healthy controls and patients with ocd. psychol Med. (2020) 50:1257–66. doi: 10.1017/s0033291720001634
85. Marek S, Dosenbach NUF. The frontoparietal network: function, electrophysiology, and importance of individual precision mapping. Dialogues Clin Neurosci. (2018) 20:133–40. doi: 10.31887/DCNS.2018.20.2/smarek
86. Puiu AA, Wudarczyk O, Kohls G, Bzdok D, Herpertz-Dahlmann B, Konrad K. Meta-analytic evidence for a joint neural mechanism underlying response inhibition and state anger. Hum Brain Mapp. (2020) 41:3147–60. doi: 10.1002/hbm.25004
87. Lee SW, Kim S, Lee S, Seo HS, Cha H, Chang Y, et al. Neural mechanisms of acceptance-commitment therapy for obsessive-compulsive disorder: A resting-state and task-based fmri study. psychol Med. (2023), 1–11. doi: 10.1017/s0033291723001769
88. Eng GK, Sim K, Chen SH. Meta-analytic investigations of structural grey matter, executive domain-related functional activations, and white matter diffusivity in obsessive compulsive disorder: an integrative review. Neurosci Biobehav Rev. (2015) 52:233–57. doi: 10.1016/j.neubiorev.2015.03.002
89. Sheffield JM, Repovs G, Harms MP, Carter CS, Gold JM, MacDonald AW 3rd, et al. Fronto-parietal and cingulo-opercular network integrity and cognition in health and schizophrenia. Neuropsychologia. (2015) 73:82–93. doi: 10.1016/j.neuropsychologia.2015.05.006
90. Ghannad-Rezaie M, Eimon PM, Wu Y, Yanik MF. Engineering brain activity patterns by neuromodulator polytherapy for treatment of disorders. Nat Commun. (2019) 10:2620. doi: 10.1038/s41467–019-10541–1
91. Kühn S, Vanderhasselt MA, De Raedt R, Gallinat J. The neural basis of unwanted thoughts during resting state. Soc Cogn Affect Neurosci. (2014) 9:1320–4. doi: 10.1093/scan/nst117
92. O'Callaghan C, Walpola IC, Shine JM. Neuromodulation of the mind-wandering brain state: the interaction between neuromodulatory tone, sharp wave-ripples and spontaneous thought. Philos Trans R Soc London Ser B Biol Sci. (2021) 376:20190699. doi: 10.1098/rstb.2019.0699
93. Fitzgerald KD, Schroder HS, Marsh R. Cognitive control in pediatric obsessive-compulsive and anxiety disorders: brain-behavioral targets for early intervention. Biol Psychiatry. (2021) 89:697–706. doi: 10.1016/j.biopsych.2020.11.012
94. Ince S, Steward T, Harrison BJ, Jamieson AJ, Davey CG, Agathos JA, et al. Subcortical contributions to salience network functioning during negative emotional processing. NeuroImage. (2023) 270:119964. doi: 10.1016/j.neuroimage.2023.119964
95. Aguilar DD, McNally JM. Subcortical control of the default mode network: role of the basal forebrain and implications for neuropsychiatric disorders. Brain Res Bull. (2022) 185:129–39. doi: 10.1016/j.brainresbull.2022.05.005
96. Sha Z, Wager TD, Mechelli A, He Y. Common dysfunction of large-scale neurocognitive networks across psychiatric disorders. Biol Psychiatry. (2019) 85:379–88. doi: 10.1016/j.biopsych.2018.11.011
97. Menon V. 20 years of the default mode network: A review and synthesis. Neuron. (2023) 111:2469–87. doi: 10.1016/j.neuron.2023.04.023
98. Zhang G, Zhang H, Li X, Zhao X, Yao L, Long Z. Functional alteration of the dmn by learned regulation of the pcc using real-time fmri. IEEE Trans Neural Syst Rehabil engineering: Publ IEEE Eng Med Biol Soc. (2013) 21:595–606. doi: 10.1109/tnsre.2012.2221480
99. Shin DJ, Jung WH, He Y, Wang J, Shim G, Byun MS, et al. The effects of pharmacological treatment on functional brain connectome in obsessive-compulsive disorder. Biol Psychiatry. (2014) 75:606–14. doi: 10.1016/j.biopsych.2013.09.002
100. Marek S, Hwang K, Foran W, Hallquist MN, Luna B. The contribution of network organization and integration to the development of cognitive control. PloS Biol. (2015) 13:e1002328. doi: 10.1371/journal.pbio.1002328
101. Cai W, Griffiths K, Korgaonkar MS, Williams LM, Menon V. Inhibition-related modulation of salience and frontoparietal networks predicts cognitive control ability and inattention symptoms in children with adhd. Mol Psychiatry. (2021) 26:4016–25. doi: 10.1038/s41380–019-0564–4
102. Salmi J, Soveri A, Salmela V, Alho K, Leppämäki S, Tani P, et al. Working memory training restores aberrant brain activity in adult attention-deficit hyperactivity disorder. Hum Brain Mapp. (2020) 41:4876–91. doi: 10.1002/hbm.25164
103. Cyr M, Pagliaccio D, Yanes-Lukin P, Fontaine M, Rynn MA, Marsh R. Altered network connectivity predicts response to cognitive-behavioral therapy in pediatric obsessive-compulsive disorder. Neuropsychopharmacology: Off Publ Am Coll Neuropsychopharmacol. (2020) 45:1232–40. doi: 10.1038/s41386–020-0613–3
Keywords: obsessive-compulsive disorder, magnetic resonance imaging, resting state, frontoparietal network, fronto-striatal circuit, meta-analysis
Citation: Yu J, Xu Q, Ma L, Huang Y, Zhu W, Liang Y, Wang Y, Tang W, Zhu C and Jiang X (2024) Convergent functional change of frontoparietal network in obsessive-compulsive disorder: a voxel-based meta-analysis. Front. Psychiatry 15:1401623. doi: 10.3389/fpsyt.2024.1401623
Received: 15 March 2024; Accepted: 11 June 2024;
Published: 08 July 2024.
Edited by:
Stefan Borgwardt, University of Lübeck, GermanyReviewed by:
Ping Li, Qiqihar Medical University, ChinaHasan Bakay, Meram Faculty of Medicine, Türkiye
Haisan Zhang, Second Affiliated Hospital of Xinxiang Medical University, China
Copyright © 2024 Yu, Xu, Ma, Huang, Zhu, Liang, Wang, Tang, Zhu and Jiang. This is an open-access article distributed under the terms of the Creative Commons Attribution License (CC BY). The use, distribution or reproduction in other forums is permitted, provided the original author(s) and the copyright owner(s) are credited and that the original publication in this journal is cited, in accordance with accepted academic practice. No use, distribution or reproduction is permitted which does not comply with these terms.
*Correspondence: Cheng Zhu, Zhucheng003042@126.com; Xiaoying Jiang, turuirui2011@126.com