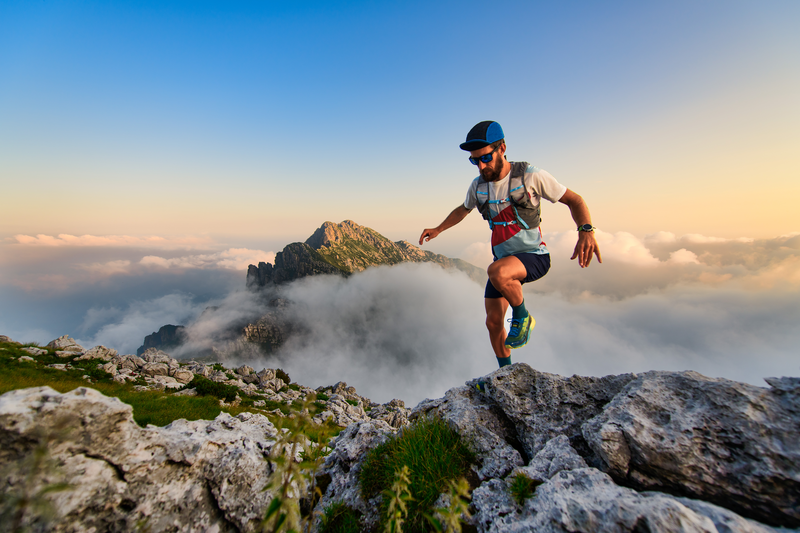
95% of researchers rate our articles as excellent or good
Learn more about the work of our research integrity team to safeguard the quality of each article we publish.
Find out more
ORIGINAL RESEARCH article
Front. Psychiatry , 10 May 2024
Sec. Sleep Disorders
Volume 15 - 2024 | https://doi.org/10.3389/fpsyt.2024.1392732
This article is part of the Research Topic New Insights into Sleep Abnormalities Associated with Alcohol, Cannabis, Cocaine, and Opiate use View all 6 articles
Objective: Previous research indicates associations between cigarette smoking, insulin-like growth factor-1 (IGF1), and sleep disturbances. This study aimed to examine the association between smoking and sleep quality and investigate the moderating role of IGF1.
Methods: This case-control study involved 146 Chinese adult males (53 active smokers and 93 non-smokers) from September 2014 to January 2016. Sleep quality and disturbances were evaluated using the Pittsburgh Sleep Quality Index (PSQI), which includes seven scales. Pearson correlation analysis and logistic regression analysis were utilized to examine the link between IGF1 levels in cerebrospinal fluid (CSF) and PSQI scores. The effect of IGF1 was assessed using the moderation effect and simple slope analysis, with adjustments made for potential confounders.
Results: Active smokers exhibited significantly higher global PSQI scores and lower IGF1 levels in CSF compared to non-smokers. A significant negative correlation was observed between IGF1 and PSQI scores (â = -0.28, P < 0.001), with a stronger association in non-smokers (Pearson r = -0.30) compared to smokers (Pearson r = -0.01). Smoking was associated with higher global PSQI scores (â = 0.282, P < 0.001), and this association was moderated by IGF1 levels in CSF (â = 0.145, P < 0.05), with a stronger effect at high IGF1 levels (Bsimple = 0.402, p < 0.001) compared to low IGF1 levels (Bsimple = 0.112, p = 0.268). Four subgroup analysis revealed similar results for sleep disturbances (Bsimple = 0.628, P < 0.001), with a marginal moderation effect observed on subjective sleep quality (Bsimple = 0.150, P = 0.070). However, independent associations rather than moderating effects were observed between IGF1 and sleep efficiency and daytime disturbance.
Conclusion: We provided evidence to demonstrate the moderation effect of IGF1 on the relationship between smoking and sleep in CSF among Chinese adult males.
Poor sleep quality has become increasingly prevalent in various population over the past decades, affecting more than a quarter of the population worldwide (1, 2). Existing epidemiological surveys have reported that approximately one-third of adults suffer from one or more sleep disorders during their aggregate lifetime (3). Of note, sleep disorder often coexists with physical health conditions and psychological comorbidities (4, 5), which in turn exacerbate the symptoms that profoundly disturb sleep quality (6). Thus, sleep disorders have gained widespread concern from all walks of life as a major public health issue and a health management challenge.
Multiple factors such as unhealthy lifestyles (smoking, drinking, sedentary, diet), chronic diseases (mental illness, metabolic diseases) housing conditions, and socializing status may contribute to abnormal sleep patterns. Among these, cigarettes smoking has been shown to be detrimental to healthy sleep in several studies. A recently published meta-analysis indicated that smoking carried a higher risk of developing sleep-related issues than non-smoking (7), and sleep disorders similarly increased the difficulties for smoking cessation (8). Nevertheless, it has been reported that sleep disorders were still prevalent among smokers despite their intense quitting attempts (9). Indeed, large amounts of nicotine contained in cigarette smoke can readily penetrate the blood-brain barrier, rapidly distribute throughout the brain (10), and stimulate nicotinic receptors to release a series of neurotransmitters that independently or interactively regulate the sleep-wake cycle, thereby exacerbating sleep disorders and affecting overall sleep quality (11, 12).
Moreover, sleep is associated with the optimal production and secretion of hormones, modulated by neuroendocrine signals (13). Recently, increasing interest has been devoted to exploring neurotrophic factors such as Insulin-like growth factor-1 (IGF1). Based on the previous studies, IGF1 is a hormone that plays a crucial role in the regulation of cell growth, differentiation, and metabolism (14). In population-based studies, high levels of peripheral IGF1 were found to be associated with better sleep quality (15). Epidemiological studies have shown that lower levels of IGF1 have been observed in individuals with chronic insomnia, while individuals with sleep extension have significantly higher levels of IGF1 concentrations in the blood compared to individuals with habitual sleep (15, 16). IGF1 has been shown to have both neuroprotective and neurorestorative effects (17), and several studies have suggested that IGF1 may have a protective effect against the negative effects of smoking on sleep. For example, one study found that chronic nicotine exposure has been found to cause sleep disturbance in rats (18), and IGF1 supplementation can improve sleep quality in rats (19). However, there were inconsistent associations of tobacco exposure with IGF1, as well as differences in IGF1 levels between smokers and non-smokers (20–22). Some studies have not found the differences in the effect of smoking on sleep quality at different levels of IGF1, indicating that the relationship between these factors may be complex and multifaceted. Therefore, the aim of this study was to investigate the effect of IGF1 in cerebrospinal fluid (CSF) on the association between smoking and sleep quality.
Considering the low proportion of female smokers in China (2.7%) (23), males were mainly recruited for the present study. The study design and population have been described in detail previously (24). Briefly, 191 subjects without fatal diseases who were scheduled for anterior cruciate ligament (ACL) reconstruction surgery were enrolled from September 2014 to January 2016 in this study. Information on sociodemographic data (age, marriage, and living) and lifestyles was obtained using interview questionnaire. Clinical information (personal and family history of diseases, history of substance abuse and dependence) was collected based on self-report and confirmed by family members. Physical examination (height and weight) was performed by a trained nurse, and body mass index (BMI) was calculated as weight in kilograms divided by height in meters squared. After excluding those with a family history of psychiatric diseases or systemic or central neurological diseases diagnosed by the Mini International Neuropsychiatric Interview, a total of 146 eligible adult males, comprising active smokers (n = 53) and non-smokers (n = 93), were recruited finally. None of the subjects had a history of alcohol abuse or psychiatric diseases identified by the Diagnostic and Statistical Manual of Mental Disorders (4th Edition). This study was conducted following the Declaration of Helsinki, approved by the Institutional Review Board of Inner Mongolian Medical University, and all the participants provided their written informed consent.
The CSF biosamples were derived from lumbar puncture, the details of which have been elaborated in the previous literature (24). On the morning before ACL reconstruction surgery, a trained and licensed anesthesiologist performed the lumbar puncture operation on the subjects under local anesthesia (using 3 mL of 0.5% ropivacaine), thus collecting 5 mL CSF samples intrathecally. Each sample was distributed into 0.5mL-tubes and immediately stored in a -80°C refrigerator for determination within 24 hours. The entire procedure from hospitalization to surgery took no more than 2 days, during which the subjects were not required to quit smoking.
The levels of IGF1 in CSF were measured using atomic absorption spectrophotometry by professional laboratory technicians. The whole process of detection was in accordance with the principle of double-blind.
Non-smokers were defined as subjects who never smoked during their whole life without a history of substance abuse or dependence. Active smokers were those who smoked at least 10 cigarettes a day lasting for over one year. Otherwise, smokers in between–those who smoked less than 10 cigarettes/day–were excluded.
The Pittsburgh Sleep Quality Index (PSQI) is a recognized comprehensive measurement for subjective self-assessment of sleep quality and disturbances within an interval of the past month that was widely used in clinical practice and research, which can identify good and poor sleepers with high specificity, sensitivity and accuracy (25). Guided by the Chinese version of PSQI (26), all participants had to respond on a four-point Likert scale (from 0 to 3, indicating “no difficulty” to “severe difficulty”). Nineteen individual items were integrated into seven subscales: subjective sleep quality, sleep latency, sleep duration, habitual sleep efficiency, sleep disturbances, use of sleeping medication, and daytime dysfunction (25). The sum of these seven components generated a global score ranging from 0 to 21, with higher scores indicating poorer sleep quality and vice versa. Because fewer participants took sleeping medications, the other six subgroups were included in the subsequent analysis.
Categorical variables were described as number (percentage) and compared by Chi-square test. According to the normality distribution (mainly by the Shapiro-Wilk normality test), normally and skewed distributed continuous variables were presented as mean ± standard deviation and median (interquartile range), and the differences between groups were compared using independent t-test and Mann-Whitney U test, respectively. The correlation between the IGF1 levels and PSQI scores was examined by Pearson correlation analysis in the active smoking and non-smoking groups. We conducted the traditional linear regression model to investigate the interactive effect of IGF1 and cigarette dependence on PSQI score. Subsequently, a multivariate logistic regression model was employed to elucidate the associations between IGF1 levels in CSF and six subgroups of PSQI in all the subjects. Both linear regression and logistic regression models were adjusted for age (years, continuous), BMI (kg/m2, continuous), marriage (married/unmarried), and living (living alone/living with one roommate/living with family members). Furthermore, a moderation effect analysis and simple slope analysis were applied to assess the moderating effect of IGF1 on the relationship between smoking and PSQI scores and the significant components of PSQI. All statistical analyses were conducted using the R software (version 4.2.0, R Foundation for Statistical Computing). The moderation analysis was performed using the “Bruce R” package. All tests were two-tailed and a P < 0.05 was considered statistically significant.
The basic characteristics of the 146 participants are presented in Table 1, with a smoking rate of 36.3%. As demonstrated in Table 1, active smokers were more likely to be older, unmarried, living alone, and have a higher BMI (all P < 0.05). Compared with non-smokers, the levels of IGF1 in CSF were significantly lower among active smokers (median levels of 33.0 ng/mL vs. 35.1 ng/mL, P < 0.001), while significantly higher in PSQI scores were observed (4.02 ± 2.27 vs. 2.60 ± 2.46, P < 0.001), particularly for sleep disturbance, sleep latency, and sleep quality. In addition, no differences were found for blood pressure and other components of PSQI between the two groups (P > 0.05).
In the total population, the levels of IGF1 in CSF were negatively correlated with PSQI scores (β = -0.28, P < 0.001) (Figure 1A). However, the correlations differed across groups, where a significant negative correlation existed in non-smokers (Pearson r = -0.30, P < 0.001) but no correlation in active smokers Pearson r = -0.01, P = 0.703) (Figure 1B). Furthermore, the relationships between IGF1 levels (divided into two groups by the median) and six subgroups of PSQI were assessed by logistic regression model (Figure 1C). After adjusting for age, BMI, marriage and living, the levels IGF1 in CSF were inversely associated with sleep disturbances (OR = 0.268, 95% CI = 0.128-0.546, P = 0.001), as well as sleep quality, habitual efficiency, and daytime dysfunction (all P < 0.05).
Figure 1 Correlation analysis between IGF1 and PSQI scores. IGF1, insulin-like growth factor-1; PSQI, Pittsburgh Sleep Quality Index; OR, odds ratio; CI, confidence interval. (A) Linear regression model was used to analyze the relationship between IGF1 levels and PSQI scores in all the groups. (B) Bivariate correlation matrix for the study variables in non-smokers and active smokers using pearson correlation analysis. *P < 0.05. (C) IGF1 (divided into two groups by the median level) and seven components of PSQI (yes/no) were included into the logistics regression model as dichotomous variables to assess the associations between the two. The linear regression and logistic regression models were adjusted for age, body mass index, marriage and living. Statistical significance (P < 0.05) was denoted in boldface.
Based on the correlations in Figure 1, we further estimated the moderating effect of IGF1 on the relationship between smoking and PSQI scores using moderation analysis (Table 2). The first step of moderation analysis is to assess the association between independent and outcome variables. As shown in Model 1 (Table 2), smoking was positively associated with PSQI scores after adjusting for potential confounders (β = 0.282, 95% CI = 0.135-0.428, R2 = 0.109, P < 0.001). In the second step, moderating variables were introduced by including the interaction terms in the linear model. According to Model 3 (Table 2), the positive impact of smoking on PSQI scores was moderated by IGF1 levels in CSF (β = 0.145, 95% CI = 0.004-0.285, R2 = 0.155, P < 0.001) (Figure 2A), with R2 increasing from 0.109 in Model 1 to 0.155 in model 3 and with F values increasing from 5.325 in Model 1 to 5.667 in Model 3 (ΔF = 0.342, p<0.05) (Table 2). To elucidate the moderating role of IGF1 more clearly, we grouped all the subjects by the median level of IGF1 and performed simple slope analysis to investigate the impact of smoking on PSQI scores at different levels of IGF1 in CSF (Figure 2B). The positive predictive effect of smoking on PSQI scores was significantly increased in individuals with higher level of IGF1 (Bsimple = 0.402, P < 0.001), while it was weakened in those with a lower level of IGF1 (Bsimple = 0.112, P = 0.268). Moreover, we also assessed the moderating effect of IGF1 in four subgroups of PSQI which were significant in the logistic model (Figure 1C). Similar results were obtained in sleep disturbances (Bsimple of 0.628 and 0.200 in the groups with high and low IGF1 levels, P < 0.001, Figure 2C), while a marginal moderating effect of IGF1 on sleep quality (Bsimple = 0.150, P = 0.070, Figure 3A). However, there were independently negative associations rather than moderation between IGF1 and habitual efficiency (β = -0.14, P < 0.05) and daytime dysfunction (β = -0.24, P < 0.01, Figure 3B).
Table 2 Linear regression analysis for the moderation effect of IGF1 on the relationship between smoking and PSQI scores.
Figure 2 The moderation by IGF1 for the association of smoking with PSQI. IGF1, insulin-like growth factor-1; PSQI, Pittsburgh Sleep Quality Index. (A) Conceptual model of moderation analysis regarding IGF1 as moderator. (B, C) Simple slope analysis for the moderation effect of IGF1 on the relationship between smoking and PSQI scores (B) as well as sleep disturbance (C). Class (-1) referred to non-smokers and Class (+1) referred to active smokers. The two lines represented the regression line of the association of smoking with PSQI scores (B) and sleep disturbance (C) when IGF1 was at low (circle) or high (triangle) levels. All data was included in the model as numerical variables and reported as moderation analysis. All the models were adjusted for age, BMI, marriage, and living. *P < 0.05, ***P < 0.001.
Figure 3 Simple slope analysis for the moderation effect of IGF-1 on PSQI components. IGF-1, insulin-like growth factor-1. (A, B) Simple slope analysis for the moderation effect of IGF-1 on the relationship between smoking and sleep quality (A) as well as sleep efficiency and daytime dysfunction (B). Class (-1) referred to non-smokers and Class (+1) referred to active smokers. The two lines represented the regression line of the association of smoking with PSQI components when IGF-1 was at low (circle) or high (triangle) levels. All data was included in the model as numerical variables and reported as moderation analysis. All the models were adjusted for age, BMI, marriage, and living. *P < 0.05, **P < 0.01.
In the present study, we found global PSQI scores were significantly higher, while IGF1 levels in CSF were lower in active smokers than non-smokers. In addition, there was a significant negative correlation between CSF IGF1 level and global PSQI score, especially in non-smokers. Furthermore, smoking was positively associated with global PSQI scores (β = 0.282, P < 0.001), which was moderated by IGF1 levels in CSF (β = 0.145, P < 0.05). Our study revealed that IGF1 played a moderating role in the process of smoking-induced sleep disorders, which, to some extent, could provide new insights into the association between cigarette smoking and sleep disorders.
Cigarette smoking is one of the major known contributors to sleep disorders. It has been reported that the smoking rate among poorer sleepers is significantly higher than that of the general population (9). A study dating back to the 1990s showed that smoking was significantly positively associated with sleep disorders (27), which was subsequently supported by various studies in different populations from different regions (28, 29). Sleep quality varies with the characteristics and intensity of smoking, a conclusion further supported by the present study. In addition. there are studies that support the possible interactions between smoking, sleep quality, and respiratory problems. For example, a study by Jang et al. found that smoking was associated with an increased risk of obstructive sleep apnea (OSA), a common respiratory disorder that can lead to poor sleep quality (30). Another study by Caliri et al. found that smoking was associated with increased inflammation and oxidative stress in the airways, which could contribute to the development of respiratory problems such as chronic obstructive pulmonary disease (COPD) (31). Moreover, some studies found that smoking was associated with poor sleep quality, and that the combination of smoking and poor sleep quality was associated with increased inflammation and oxidative stress, suggesting that these factors may interact to exacerbate respiratory problems (32–34). Overall, the existing literature suggests that there are complex interactions between smoking, sleep quality, and respiratory problems, and that these factors may influence each other in important ways. Further research is needed to fully understand these interactions and their clinical implications.
In the present study, IGF1 levels in CSF were lower in active smokers than non-smokers. Previous studies have shown that circulating IGF1 has the ability to reach the central nervous system through either the blood-CSF barrier or the blood-brain barrier (35, 36). Thus, the lack of an increase in peripheral IGF1 can lead to a deficiency of IGF1 in the brain (36). Smoking has been shown to reduce the level of peripheral IGF1 (20, 37) and destroy the blood-brain barrier (38, 39), which can further explain our results.
The main finding of present study is that there was a significant negative correlation between the IGF1 level in CSF and PSQI score, especially in non-smokers. A low serum IGF1 level has been reported to be associated with sleep-related disease, and longer slow wave time could be associated with increased IGF1 levels (40, 41). A recent case-control study in China indicated that serum IGF1 concentration was negatively associated with chronic insomnia, sleep disorders and anxiety scores (15). Behavioral symptoms of circadian rhythm imbalance and sleep-wake disorders were noted to be improved by increasing or releasing free IGF1 in serum (16, 42). Similarly, animal experiments and epidemiological studies have revealed that sleep disorders might inhibit the IGF1 axis, with circulating IGF1 levels significantly declining after sustained sleep deprivation (43, 44). The underlying mechanisms are complex. IGF1 is known for its neuroprotective properties, activating IGF1 receptor to initiate downstream phosphorylation cascades that regulate transcription, synaptic maturation, inhibits apoptosis, and promote growth, differentiation and metabolism of neuronal cells (35). Firstly, this relationship may be attributed to BDNF/IGF1 regulated neuronal plasticity changes, hypothesized to increase slow wave sleep activity (45, 46). Moreover, IGF1 could facilitate the repair of neurons from hypoxia and improve sleep regulation (47). These studies suggest that IGF1 could improve sleep quality to some extent, which is similar to our results.
Moreover, our results imply that the level of IGF1 might differently influence the relationship between smoking and sleep quality. As mentioned, a high IGF1 level is associated with low PSQI scores in both non-smokers and all participants, indicating that IGF1, like cigarettes, leads to a direct effect on sleep quality. However, for participants with different CSF IGF1 levels, we not only found the independent effects from the two factors (β = 0.258***, t = 3.525 for smoking; β = -0.212**, t = -2.967 for IGF1). In addition, there was a complex interaction (β = 0.145*, t = 2.042). For participants with a low CSF IGF1 level, smoking did not activate sleep problems (β = 0.112, P = 0.268), but in those who with a high CSF IGF1 level, the sleep damage caused by smoking was greatly increased (β = 0.402, P < 0.001).
This finding is intriguing. Elevated IGF1 levels have the ability to regulate sleep, as mentioned above. Numerous studies have shown a positive correlation between IGF1 levels and sleep quality. Therefore, given the independent effects of smoking and IGF1, it is expected that elevated IGF1 levels will counteract the sleep disturbances induced by smoking. In the interaction model and its sub-dimensions, this effect is evident. Specifically, IGF1 exerts a significant protective effect on four dimensions of sleep disturbance: sleep quality, sleep efficiency, sleep disturbance and daytime dysfunction (sleep quality: OR = 0.389, p = 0.010; sleep efficiency: OR = 0.066, p = 0.010; daytime dysfunction: OR = 0.333, p = 0.002; sleep disturbance: OR = 0.268, p = 0.001). It is also noteworthy that an interaction similar to the PSQI results was only observed in the sleep disturbance dimension. Smoking and IGF1 had independent effects on sleep (see Figure 3) in the remaining dimensions.
It is likely that this effect is due to the activity of the orexin neurons. A study in 2020 clearly demonstrated that IGF1 in the central nervous system can directly influence the sleep-wake cycle of mice through the activation of orexin neurons. Orexin neurons significantly prolonged sleep duration in mice lacking IGF1 receptors, suggesting the involvement of IGF1 in wakefulness and maintenance via orexin neurons (48). In addition, previous studies have consistently shown a strong link between smoking and orexin expression. Exposure to smoke significantly increases orexin levels, thereby promoting wakefulness (49–51). Consequently, smokers with elevated levels of cerebrospinal fluid IGF1 may experience increased nicotine-induced stimulation of active orexin neurons, leading to this significant positive interaction.
To our knowledge, this is the first study to assess the role of IGF1 in CSF on smoking-induced sleep disorders (indicated by PSQI) in Chinese males. The effect of smoking on PSQI is moderated by different levels of IGF1 in CSF. Admittedly, there are several limitations in this study. First, causal inferences cannot be drawn from the case-control design, and a small sample size may restrict the statistical power to examine associations and moderations. Hence, evidence from prospective studies with larger sample size is warranted. Second, retrospective recall biases may occur using subjective sleep measurements and smoking assessments. Third, anterior cruciate ligament reconstructive surgery may be a potential confounder affecting smoking, sleep quality and biomarkers. Moreover, other potential confounding factor such as obstructive sleep apnea may affect our understanding of the relationship between smoking and sleep. Finally, only men were recruited due to the low smoking rate in women, resulting in limited applicability and generalizability.
The positive effect of smoking on PSQI scores and sleep disturbances were negatively moderated by the levels of IGF1 in cerebrospinal fluid in Chinese adult males. The results of this study have important clinical implications. Firstly, they highlight the importance of considering IGF1 levels in cerebrospinal fluid when assessing the relationship between smoking and sleep quality. Clinicians may need to monitor IGF1 levels in smokers who report poor sleep quality and consider interventions aimed at increasing IGF1 levels, such as exercise or nutritional supplements. Secondly, the findings suggest that targeting IGF1 may be a potential therapeutic strategy for improving sleep quality in smokers. Future studies are needed to explore the underlying mechanisms and to develop effective interventions. Overall, this study contributes to our understanding of the complex interplay between smoking, IGF1, and sleep quality. The findings have important implications for the development of targeted interventions to improve sleep quality in smokers and for the prevention of smoking-related sleep disturbances.
The raw data supporting the conclusions of this article will be made available by the authors, without undue reservation.
The studies involving humans were approved by Institutional Review Board of Inner Mongolian Medical University. The studies were conducted in accordance with the local legislation and institutional requirements. The participants provided their written informed consent to participate in this study.
LS: Writing – original draft. YW: Writing – original draft, Formal Analysis. JL: Writing – review & editing, Resources. MM: Writing – review & editing, Resources. XL: Writing – review & editing. KZ: Writing – original draft, Resources. WH: Writing – review & editing. YK: Writing – review & editing, Resources. FW: Writing – review & editing, Resources. YL: Writing – review & editing, Supervision. YX: Writing – review & editing, Supervision. XJ: Writing – review & editing, Supervision.
The author(s) declare financial support was received for the research, authorship, and/or publication of this article. This work was supported by the Basic Public Welfare Research Project of Zhejiang Province (TGD23H030004), Wenzhou Basic Scientific Research Project (Y20220021), Health Science and Technology Project of Zhejiang Province (2022KY887).
We thank Prof. Li Chen for her statistic help.
The authors declare that the research was conducted in the absence of any commercial or financial relationships that could be construed as a potential conflict of interest.
All claims expressed in this article are solely those of the authors and do not necessarily represent those of their affiliated organizations, or those of the publisher, the editors and the reviewers. Any product that may be evaluated in this article, or claim that may be made by its manufacturer, is not guaranteed or endorsed by the publisher.
1. Morin CM, Benca R. Chronic insomnia. Lancet. (2012) 379:1129–41. doi: 10.1016/S0140-6736(11)60750-2
2. Soldatos CR, Allaert FA, Ohta T, Dikeos DG. How do individuals sleep around the world? Results from a single-day survey in ten countries. Sleep Med. (2005) 6:5–13. doi: 10.1016/j.sleep.2004.10.006
3. Ohayon MM. Epidemiology of insomnia: what we know and what we still need to learn. Sleep Med Rev. (2002) 6:97–111. doi: 10.1053/smrv.2002.0186
5. Sun X, Liu B, Liu S, Wu DJH, Wang J, Qian Y, et al. Sleep disturbance and psychiatric disorders: A bidirectional Mendelian randomisation study. Epidemiol Psychiatr Sci. (2022) 31:e26. doi: 10.1017/S2045796021000810
6. Fang H, Tu S, Sheng J, Shao A. Depression in sleep disturbance: A review on a bidirectional relationship, mechanisms and treatment. J Cell Mol Med. (2019) 23:2324–32. doi: 10.1111/jcmm.14170
7. Amiri S, Behnezhad S. Smoking and risk of sleep-related issues: A systematic review and meta-analysis of prospective studies. Can J Public Health. (2020) 111:775–86. doi: 10.17269/s41997-020-00308-3
8. Short NA, Mathes BM, Gibby B, Oglesby ME, Zvolensky MJ, Schmidt NB. Insomnia symptoms as a risk factor for cessation failure following smoking treatment. Addict Res Theory. (2017) 25:17–23. doi: 10.1080/16066359.2016.1190342
9. Liao Y, Xie L, Chen X, Kelly BC, Qi C, Pan C, et al. Sleep quality in cigarette smokers and nonsmokers: findings from the general population in central China. BMC Public Health. (2019) 19:808. doi: 10.1186/s12889-019-6929-4
10. Benowitz NL. Drug therapy. Pharmacologic aspects of cigarette smoking and nicotine addiction. N Engl J Med. (1988) 319:1318–30. doi: 10.1056/NEJM198811173192005
11. Jaehne A, Unbehaun T, Feige B, Cohrs S, Rodenbeck A, Schutz AL, et al. Sleep Changes in Smokers before, During and 3 Months after Nicotine Withdrawal. Addict Biol. (2015) 20:747–55. doi: 10.1111/adb.12151
12. Li H, Liu Y, Xing L, Yang X, Xu J, Ren Q, et al. Association of cigarette smoking with sleep disturbance and neurotransmitters in cerebrospinal fluid. Nat Sci Sleep. (2020) 12:801–8. doi: 10.2147/NSS.S272883
13. Chennaoui M, Léger D, Gomez-Merino D. Sleep and the Gh/Igf-1 axis: consequences and countermeasures of sleep loss/disorders. Sleep Med Rev. (2020) 49:101223. doi: 10.1016/j.smrv.2019.101223
14. Werner H. The Igf1 signaling pathway: from basic concepts to therapeutic opportunities. Int J Mol Sci. (2023) 24:14882. doi: 10.3390/ijms241914882
15. Zhang Y, Sun Q, Li H, Wang D, Wang Y, Wang Z. Lower serum insulin-like growth factor 1 concentrations in patients with chronic insomnia disorder. Front Psychiatry. (2023) 14:1102642. doi: 10.3389/fpsyt.2023.1102642
16. Chennaoui M, Arnal PJ, Drogou C, Sauvet F, Gomez-Merino D. Sleep extension increases Igf-I concentrations before and during sleep deprivation in healthy young men. Appl Physiol Nutr Metab. (2016) 41:963–70. doi: 10.1139/apnm-2016-0110
17. Huang YY, Wang HF, Wu BS, Ou YN, Ma LZ, Yang L, et al. Clinical laboratory tests and dementia incidence: A prospective cohort study. J Affect Disord. (2024) 351:1–7. doi: 10.1016/j.jad.2024.01.226
18. Salin-Pascual RJ, Moro-Lopez ML, Gonzalez-Sanchez H, Blanco-Centurion C. Changes in sleep after acute and repeated administration of nicotine in the rat. Psychopharmacol (Berl). (1999) 145:133–8. doi: 10.1007/s002130051041
19. Obál F Jr., Kapás L, Bodosi B, Krueger JM. Changes in sleep in response to intracerebral injection of insulin-like growth factor-1 (Ifg-1) in the rat. Sleep Res Online. (1998) 1:87–91.
20. Landin-Wilhelmsen K, Wilhelmsen L, Lappas G, Rosén T, Lindstedt G, Lundberg PA, et al. Serum insulin-like growth factor I in a random population sample of men and women: relation to age, sex, smoking habits, coffee consumption and physical activity, blood pressure and concentrations of plasma lipids, fibrinogen, parathyroid hormone and osteocalcin. Clin Endocrinol (Oxf). (1994) 41:351–7. doi: 10.1111/j.1365-2265.1994.tb02556.x
21. Palmer RM, Wilson RF, Coward PY, Scott DA. Analysis of circulating insulin-like growth factor-1 (Igf-1) and Igf binding protein-3 (Igfbp-3) in tobacco smokers and non-smokers. Tob Induc Dis. (2002) 1:157–70. doi: 10.1186/1617-9625-1-2-157
22. Janssen JA, Stolk RP, Pols HA, Grobbee DE, Lamberts SW. Serum total Igf-I, free Igf-I, and Igfb-1 levels in an elderly population: relation to cardiovascular risk factors and disease. Arterioscler Thromb Vasc Biol. (1998) 18:277–82. doi: 10.1161/01.atv.18.2.277
23. Chinese Center for Disease Control and Prevention. 2015 Chinese Adults Tobacco Survey Report. Beijing (2015).
24. Liu Y, Li H, Wang J, Xue Q, Yang X, Kang Y, et al. Association of cigarette smoking with cerebrospinal fluid biomarkers of neurodegeneration, neuroinflammation, and oxidation. JAMA Netw Open. (2020) 3:e2018777. doi: 10.1001/jamanetworkopen.2020.18777
25. Buysse DJ, Reynolds CF 3rd, Monk TH, Berman SR, Kupfer DJ. The pittsburgh sleep quality index: A new instrument for psychiatric practice and research. Psychiatry Res. (1989) 28:193–213. doi: 10.1016/0165-1781(89)90047-4
26. Tsai PS, Wang SY, Wang MY, Su CT, Yang TT, Huang CJ, et al. Psychometric evaluation of the Chinese version of the Pittsburgh sleep quality index (Cpsqi) in primary insomnia and control subjects. Qual Life Res. (2005) 14:1943–52. doi: 10.1007/s11136-005-4346-x
27. Phillips BA, Danner FJ. Cigarette smoking and sleep disturbance. Arch Intern Med. (1995) 155:734–7. doi: 10.1001/archinte.155.7.734
28. Cohrs S, Rodenbeck A, Riemann D, Szagun B, Jaehne A, Brinkmeyer J, et al. Impaired sleep quality and sleep duration in smokers-results from the German multicenter study on nicotine dependence. Addict Biol. (2014) 19:486–96. doi: 10.1111/j.1369-1600.2012.00487.x
29. Bellatorre A, Choi K, Lewin D, Haynie D, Simons-Morton B. Relationships between smoking and sleep problems in black and white adolescents. Sleep. (2017) 40:zsw031. doi: 10.1093/sleep/zsw031
30. Jang YS, Nerobkova N, Hurh K, Park EC, Shin J. Association between smoking and obstructive sleep Apnea based on the stop-bang index. Sci Rep. (2023) 13:9085. doi: 10.1038/s41598-023-34956-5
31. Caliri AW, Tommasi S, Besaratinia A. Relationships among smoking, oxidative stress, inflammation, macromolecular damage, and cancer. Mutat Res Rev Mutat Res. (2021) 787:108365. doi: 10.1016/j.mrrev.2021.108365
32. Purani H, Friedrichsen S, Allen AM. Sleep quality in cigarette smokers: associations with smoking-related outcomes and exercise. Addict Behav. (2019) 90:71–6. doi: 10.1016/j.addbeh.2018.10.023
33. Irwin MR. Sleep and inflammation: partners in sickness and in health. Nat Rev Immunol. (2019) 19:702–15. doi: 10.1038/s41577-019-0190-z
34. Htoo A, Talwar A, Feinsilver SH, Greenberg H. Smoking and sleep disorders. Med Clin North Am. (2004) 88:1575–91. doi: 10.1016/j.mcna.2004.07.003
35. Benarroch EE. Insulin-like growth factors in the brain and their potential clinical implications. Neurology. (2012) 79:2148–53. doi: 10.1212/WNL.0b013e3182752eef
36. Yan H, Mitschelen M, Bixler GV, Brucklacher RM, Farley JA, Han S, et al. Circulating Igf1 regulates hippocampal Igf1 levels and brain gene expression during adolescence. J Endocrinol. (2011) 211:27–37. doi: 10.1530/joe-11-0200
37. Fang F, Luo ZC, Dejemli A, Delvin E, Zhang J. Maternal smoking and metabolic health biomarkers in newborns. PloS One. (2015) 10:e0143660. doi: 10.1371/journal.pone.0143660
38. Randolph AC, Fukuda S, Ihara K, Enkhbaatar P, Micci MA. Blood-Brain Barrier Dysfunction after Smoke Inhalation Injury, with and without Skin Burn. Shock. (2019) 51:634–49. doi: 10.1097/shk.0000000000001196
39. Archie SR, Sifat AE, Zhang Y, Villalba H, Sharma S, Nozohouri S, et al. Maternal E-cigarette use can disrupt postnatal blood-brain barrier (Bbb) integrity and deteriorates motor, learning and memory function: influence of sex and age. Fluids Barriers CNS. (2023) 20:17. doi: 10.1186/s12987-023-00416-5
40. Galerneau LM, Borel AL, Chabre O, Sapene M, Stach B, Girey-Rannaud J, et al. The somatotropic axis in the sleep Apnea-obesity comorbid duo. Front Endocrinol (Lausanne). (2020) 11:376. doi: 10.3389/fendo.2020.00376
41. Prinz PN, Moe KE, Dulberg EM, Larsen LH, Vitiello MV, Toivola B, et al. Higher plasma Igf-1 levels are associated with increased delta sleep in healthy older men. J Gerontol A Biol Sci Med Sci. (1995) 50:M222–6. doi: 10.1093/gerona/50a.4.m222
42. Kimura S, Toyoura M, Toyota Y, Takaoka Y. Serum concentrations of insulin-like growth factor-1 as a biomarker of improved circadian rhythm sleep-wake disorder in school-aged children. J Clin Sleep Med. (2020) 16:2073–8. doi: 10.5664/jcsm.8778
43. Chennaoui M, Drogou C, Sauvet F, Gomez-Merino D, Scofield DE, Nindl BC. Effect of acute sleep deprivation and recovery on insulin-like growth factor-I responses and inflammatory gene expression in healthy men. Eur Cytokine Netw. (2014) 25:52–7. doi: 10.1684/ecn.2014.0356
44. Everson CA, Crowley WR. Reductions in circulating anabolic hormones induced by sustained sleep deprivation in rats. Am J Physiol Endocrinol Metab. (2004) 286:E1060–70. doi: 10.1152/ajpendo.00553.2003
45. Tononi G, Cirelli C. Sleep function and synaptic homeostasis. Sleep Med Rev. (2006) 10:49–62. doi: 10.1016/j.smrv.2005.05.002
46. Rusch HL, Guardado P, Baxter T, Mysliwiec V, Gill JM. Improved sleep quality is associated with reductions in depression and Ptsd arousal symptoms and increases in Igf-1 concentrations. J Clin Sleep Med. (2015) 11:615–23. doi: 10.5664/jcsm.4770
47. Mysliwiec V, Gill J, Matsangas P, Baxter T, Barr T, Roth BJ. Igf-1: A potential biomarker for efficacy of sleep improvement with automatic airway pressure therapy for obstructive sleep Apnea? Sleep Breath. (2015) 19:1221–8. doi: 10.1007/s11325-015-1142-x
48. Zegarra-Valdivia JA, Pignatelli J, Fernandez de Sevilla ME, Fernandez AM, Munive V, Martinez-RaChadell L, et al. Insulin-like growth factor I modulates sleep through hypothalamic orexin neurons. FASEB J. (2020) 34:15975–90. doi: 10.1096/fj.202001281RR
49. Liu ZB, Song NN, Geng WY, Jin WZ, Li L, Cao YX, et al. Orexin-a and respiration in a rat model of smoke-induced chronic obstructive pulmonary disease. Clin Exp Pharmacol Physiol. (2010) 37:963–8. doi: 10.1111/j.1440-1681.2010.05411.x
50. Hoyer D, Jacobson LH. Orexin in sleep, addiction and more: is the perfect insomnia drug at hand? Neuropeptides. (2013) 47:477–88. doi: 10.1016/j.npep.2013.10.009
Keywords: smoking, PSQI score, sleep disturbances, IGF1, moderation
Citation: Shan L, Wu Y, Lao J, Ma M, Luo X, Zheng K, Hu W, Kang Y, Wang F, Liu Y, Xu Y and Jin X (2024) The positive impact of smoking on poor sleep quality is moderated by IGF1 levels in cerebrospinal fluid: a case-control study among Chinese adults. Front. Psychiatry 15:1392732. doi: 10.3389/fpsyt.2024.1392732
Received: 28 February 2024; Accepted: 24 April 2024;
Published: 10 May 2024.
Edited by:
Lori Keyser-Marcus, Virginia Commonwealth University, United StatesReviewed by:
Gabriela Hurtado-Alvarado, Universidad Nacional Autónoma de México, MexicoCopyright © 2024 Shan, Wu, Lao, Ma, Luo, Zheng, Hu, Kang, Wang, Liu, Xu and Jin. This is an open-access article distributed under the terms of the Creative Commons Attribution License (CC BY). The use, distribution or reproduction in other forums is permitted, provided the original author(s) and the copyright owner(s) are credited and that the original publication in this journal is cited, in accordance with accepted academic practice. No use, distribution or reproduction is permitted which does not comply with these terms.
*Correspondence: Yanlong Liu, YmVuamFtaW5seWxAd211LmVkdS5jbg==; Xiaoya Jin, amlueGlhb3lhQHd6aG9zcGl0YWwuY24=; Yali Xu, eHV5YWxpMDEyOEAxNjMuY29t
†These authors have contributed equally to this work and share first authorship
Disclaimer: All claims expressed in this article are solely those of the authors and do not necessarily represent those of their affiliated organizations, or those of the publisher, the editors and the reviewers. Any product that may be evaluated in this article or claim that may be made by its manufacturer is not guaranteed or endorsed by the publisher.
Research integrity at Frontiers
Learn more about the work of our research integrity team to safeguard the quality of each article we publish.