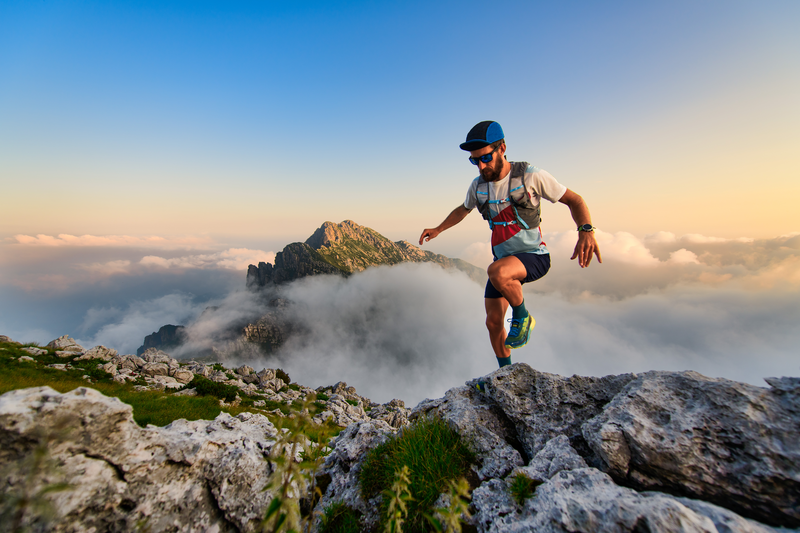
94% of researchers rate our articles as excellent or good
Learn more about the work of our research integrity team to safeguard the quality of each article we publish.
Find out more
ORIGINAL RESEARCH article
Front. Psychiatry , 11 June 2024
Sec. Psychopharmacology
Volume 15 - 2024 | https://doi.org/10.3389/fpsyt.2024.1380123
This article is part of the Research Topic Women in Psychiatry 2023: Psychopharmacology View all 7 articles
Introduction: Abuse or misuse of tobacco, e-cigarettes, or antidepressants may have serious clinical consequences during adolescence, a sensitive period during brain development when the distinct neurobiology of adolescent serotonin (5-HT) and dopamine (DA) systems create unique behavioral vulnerabilities to drugs of abuse.
Methods: Using a pharmacological approach, we modeled the behavioral and neurochemical effects of subchronic (4-day) nicotine (60µg/kg, i.v.) or fluoxetine (1mg/kg, i.v.) exposure in adolescent and adult male rats.
Results: Nicotine and fluoxetine significantly enhance quinpirole-induced locomotor activity and initial cocaine self-administration in adolescents, but not adults. These effects were blocked by serotonin 5-HT1A receptor antagonists, WAY-100,635 (100 µg/kg, i.v.) or S-15535 (300 µg/kg, i.v.). Neurochemical and anatomical autoradiographic analysis of 8-OH-DPAT-stimulated [35S]GTPγS reveal that prior exposure to nicotine and fluoxetine results in both overlapping and distinct effects on regional 5-HT1A receptor activity. Both fluoxetine and nicotine enhance adolescent 5-HT1A receptor activity in the primary motor cortex (M1), whereas fluoxetine alone targets prefrontal cortical neurocircuitry and nicotine alone targets the amygdala.
Discussion: Given their different pharmacological profiles, comparison between WAY-100,635 and S-15535 indicates that postsynaptic 5-HT1A receptors mediate the behavioral effects of prior nicotine and fluoxetine exposure. In addition, within the adolescent M1, maladaptive changes in 5-HT signaling and 5-HT1A activity after nicotine or fluoxetine exposure may potentiate hyper-responsiveness to dopaminergic drugs and prime adolescent vulnerability for future substance abuse.
Adolescence is a period of brain development during which neurotransmitter systems are actively maturing, with dopamine (DA) exhibiting particularly dynamic changes (1–3). These neurochemical changes not only underlie executive, cognitive, and emotional maturation, but also mark adolescence as a vulnerable transition period when the initiation of substance abuse and onset of psychiatric disorders typically emerge (4, 5).
Among teenagers, recreational use of electronic nicotine delivery systems (e-cigarettes) has escalated exponentially (6, 7). Originally marketed as a smoking cessation aid, recreational e-cigarette use, or vaping, is currently more prevalent than combustible cigarette use among young people (8). Furthermore, teenagers who vape are more likely to smoke, and e-cigarettes may be a “gateway” to future tobacco abuse (9). Consequently, the emerging popularity of e-cigarettes poses an urgent threat for adolescent health, as tobacco remains the leading cause of disease and death worldwide and the negative health effects of e-cigarette use has yet to be fully understood.
Initiation of smoking typically occurs during adolescence, with approximately 90% of adult smokers starting before age 18 (10). Furthermore, adolescent nicotine and tobacco use are associated with disproportionately higher rates of future substance abuse (11–14) and depression compared to their nonsmoking peers (15). To treat teen depression, fluoxetine (Prozac), a selective serotonin reuptake inhibitor (SSRI), is commonly prescribed as an antidepressant (16). Although beneficial, SSRIs can increase suicidal thoughts and behaviors, agitation, anxiety, and lead to further depression in teenagers (17–19).
Adolescent rodents, conservatively defined as between postnatal day (P) 28 and 42 (20), demonstrate unique responses to both SSRIs and nicotine. Chronic SSRI treatment upregulates hippocampal neurotrophic factors in adult rats, but pro-apoptotic factors in adolescents (21). Fluoxetine also enhances cell proliferation in adult rats, with little change in adolescents (22). Compared to adults, adolescent rats associate a greater rewarding effect with nicotine in conditioned place preference and self-administration studies (23–28). In contrast, adolescents display both less aversion (25, 28, 29) and blunted withdrawal symptoms to nicotine compared to adults (30, 31). Nicotine induces inflammatory markers in adolescent brain, in contrast to the anti-inflammatory effect observed in adults (32). Nicotine also exerts unique effects on adolescent DA and 5-HT systems; specifically, receptor activation of both DA and 5-HT receptor subtypes underlies nicotine-induced increases of drug reinforcement in adolescents, but not adults (32, 33).
In adolescent rodents, but not adults, brief exposure to nicotine enhances cocaine locomotor sensitization, conditioned place preference, and self-administration as well as quinpirole-induced activity (33–37). Not only does nicotine selectively increase 5-HT content and transporter binding in adolescent forebrain regions, but several unique behavioral effects of adolescent nicotine exposure are blocked by co-administration of a 5-HT1A receptor (5-HT1AR) antagonist (33). Together, these findings suggest that age-specific alterations in 5-HT and 5-HT1AR signaling during adolescence may underlie enhanced behavioral sensitivity to DA drugs after nicotine exposure.
We have now used a behavioral pharmacology approach to test whether direct changes to endogenous 5-HT signaling with fluoxetine in adolescence can also potentiate DA-mediated behaviors, including cocaine self-administration and ambulatory activity induced by quinpirole, a D2 receptor agonist. In addition, we have characterized the possible role of 5-HT1ARs, using the antagonist WAY-100,635 and the partial agonist S-15535, in the effects of nicotine and fluoxetine on the adolescent brain. Furthermore, through [35S]GTPγS binding, an in vitro approach to examine receptor-G protein coupling, we have shown unique effects of adolescent fluoxetine and nicotine exposure on 5-HT1AR function.
Male Sprague-Dawley rats (Charles River Labs, Hollister, CA) arrived at P17 with dams or at P75. Rats were group-housed in an AAALAC-accredited vivarium on a 12 hr light/dark cycle with unlimited access to food and water. Pups were weaned on P21. All experiments were performed during the light cycle and carried out in accordance with the Institutional Animal Care and Use Committee at the University of California, Irvine.
Fluoxetine HCl (Sigma, St. Louis, MO) was dissolved in sterile water. Nicotine hydrogen tartrate (Sigma, St. Louis, MO) was dissolved in sterile saline and adjusted to pH 7.2 – 7.4; doses were calculated as free base. Cocaine HCl (Sigma, St. Louis, MO) (–),-quinpirole HCl (Tocris Bioscience, Bristol, UK), and WAY-100,635 (Sigma, St. Louis, MO) were dissolved in sterile saline. S-15535 (Sigma, St. Louis, MO) was dissolved in sterile saline that was acidified with lactic acid, and pH was adjusted to as close to neutrality as possible (pH > 5.0). L-741,626 (Tocris Bioscience, Bristol, UK) was dissolved in 50% ethanol/saline. Propofol was purchased from Abbot Laboratories (Chicago, IL).
Rats, aged P24 or P82, were anesthetized with equithesin (0.0035 ml/g) and surgically implanted with an indwelling catheter in their jugular vein (37). During 3 days of recovery, catheters were flushed daily with heparinized saline solution (1000 units heparin per 30 ml bacteriostatic saline) to maintain patency. Catheter patency was verified by testing with propofol (5 mg/kg, i.v.) after drug pretreatment, and only animals showing rapid anesthesia were included in analyses.
Adolescent (P28–31) and adult (P86–89) rats received a drug pretreatment for 4 consecutive days (32). Daily intravenous injections of fluoxetine (1 mg/kg), WAY-100,635 (100 µg/kg), fluoxetine + WAY-100,635, nicotine (60 µg/kg), or saline were administered during adolescence or adulthood. Behavioral testing was conducted the following day on either P32 or P90 (Supplementary Figure 1).
One day after pretreatment, at P32 for adolescents and P90 for adults, locomotor activity was recorded in an open field chamber (43.2 × 43.2 × 30.5 cm3) by 16 photobeams along the sides of each wall (MED Associates, Inc., St. Albans, VT). Following 30 min of habituation in the test chamber, rats received an injection of either quinpirole (0.4 mg/kg, i.p.), a selective D2 and D3 receptor agonist, or vehicle and returned for 30 min monitoring of horizontal activity. For acute 5-HT1AR antagonist studies, rats were given a single infusion of WAY-100,635 (100 µg/kg, i.v.), S-15535 (300 µg/kg, i.v.), or vehicle 20 min prior to locomotor testing.
One day after pretreatment, at P32 for adolescents and P90 for adults, acquisition of cocaine self-administration was evaluated in an operant chamber containing two nose poke holes (MED Associates, Inc., St. Albans, VT). Rats self-administered cocaine (0.5 mg/kg, i.v., 20 µl, 1.1s) in a single 1 hr session on a fixed ratio 1 (FR1) schedule. During each infusion, the cue light above the reinforced hole was illuminated; afterward the house light shut off for a 20 sec timeout where responses were counted but had no effect. To control for nonspecific activity, responses for the non-reinforced hole were recorded but had no programmed consequences. For acute 5-HT1AR antagonist studies, rats were given a single infusion of WAY-100,635 (100 µg/kg, i.v.), L-741,626 (2 mg/kg, i.p.), or vehicle 20 min prior to self-administration testing.
On P32 or P90, 24 hrs after the last pretreatment infusion, brains were collected, rapidly frozen, and stored at -70°C until processing. Coronal sections of 20 µm thickness were cut in a cryostat maintained at -20°C. Sections were mounted onto ice-cold, gelatin-coated glass slides (Fisher Scientific, Waltham, MA). Slides were air dried for 30 min at room temperature and processed for 8-OH-DPAT-stimulated [35S]GTPγS binding.
8-OH-DPAT-stimulated [35S]GTPγS (Perkin Elmer, Boston, MA) autoradiography was as described by Hensler et al. (2007) (38) with slight modification. Slide-mounted sections were equilibrated in assay buffer (50 mM HEPES; 3 mM magnesium perchlorate; 0.2 mM EGTA; 100 mM NaCl; and 0.2 mM dithiothreitol; pH 7.4) for 10 min at room temperature. Sections were pre-incubated in 5’-guanylate diphosphate (2 mM GDP) for 15 min at room temperature, and then incubated for 60 min in HEPES buffer containing GDP (2 mM) and [35S]GTPγS (40 pM), with or without 8-OH-DPAT (1 µM). Basal [35S]GTPγS binding was determined in the absence of 8-OH-DPAT. Nonspecific [35S]GTPγS binding was determined in the presence of unlabeled GTPγS (10 µM). The incubation was stopped by two 5 min washes in ice-cold HEPES buffer (50 mM, pH 7.4), followed by a brief rinse in ice-cold deionized water. Sections were air dried at room temperature for 1 hr and exposed to Kodak Biomax MR film for 48 hrs.
Digitized autoradiograms were quantified with a MicroComputer Imaging Device (MCID Imaging Research, St. Catherine, Ontario, Canada; Supplementary Figure 2). Autoradiograms of 8-OH-DPAT-stimulated [35S]GTPγS binding were simultaneously exposed with [14C] standards (39). Standard calibration curves were generated from [14C] standards of optical density against radioligand concentration (dpm/mg tissue) and used to transform regional density values into relative radioactivity measures. Nonspecific binding of [35S]GTPγS was subtracted from basal binding and from specific, agonist-stimulated, binding. Specific binding was expressed as percent change from basal. Areas that were analyzed included subregions of the cortex (prelimbic, infralimbic, ventrolateral orbital, cingulate, agranular insular, primary motor, and secondary motor); amygdala (basolateral, central, and medial); striatum (caudate putamen, nucleus accumbens shell and core); hypothalamus (paraventricular nucleus and lateral); dorsal and ventral hippocampus; dorsal and median raphe; bed nucleus of the stria terminalis; periventricular thalamus; and ventral tegmental area.
No statistical methods were used to predetermine sample sizes. Sample sizes used were similar to those reported in previous publications (32–36). Analysis of digitized autoradiograms were performed blind.
Locomotor activity data are expressed as mean ± SEM and were analyzed by 3-way ANOVA comparing Age × Pretreatment × Drug or 2-way ANOVA comparing Pretreatment × Drug. All significant main effects were further analyzed by one-way ANOVA with Bonferroni post hoc comparisons. For acquisition of cocaine or sucrose self-administration, reinforced (R) and non-reinforced (NR) response data are expressed as mean ± SEM and were analyzed by 2-way ANOVA for Pretreatment × R/NR Responses, with repeated measures on Responses. Significant effects of reinforcement were analyzed by Bonferroni-corrected paired t-tests for each pretreatment group. Anatomical data for each brain region are expressed as mean ± SEM and were analyzed separately using 2-way ANOVA comparing Pretreatment × Age. Any significant main effects were further compared using Dunnett’s post hoc test. All analyses were completed using SPSS Statistics software. Outliers (≥2 SD from group mean) were removed prior to analysis.
As shown in Figure 1 fluoxetine pretreatment of adolescents, but not adults, increased quinpirole-induced locomotor activity via a 5-HT1AR mechanism. Overall effects of Age (F1,126 = 96.189, p < 0.001), Pretreatment (F3,126 = 3.255, p < 0.05), and Drug (F1,126 = 111.913, p < 0.001) were seen, as well as significant interactions of Age × Pretreatment (F3,126 = 4.696, p < 0.01), Age × Drug (F1,126 = 88.538, p < 0.001), Pretreatment × Drug (F3,126 = 4.301, p < 0.01], and Age × Pretreatment × Drug (F3,126 = 4.937, p < 0.01). Quinpirole induced significant locomotor activity in adolescents (p < 0.001; Figure 1A), which was enhanced by fluoxetine pretreatment (p < 0.01). Co-administration of the 5-HT1A receptor antagonist, WAY-100,635, during pretreatment blocked fluoxetine enhancement of quinpirole-induced locomotion in adolescents (p < 0.001). As has been shown previously (33), quinpirole did not induce significant locomotor activity in adults; nor did fluoxetine or WAY-100,635 pretreatment influence locomotor activity in this age group (Figure 1B).
Figure 1 Age differences in the effect of fluoxetine and WAY-100,635 pretreatment on quinpirole-induced locomotion. (A) Quinpirole (0.4 mg/kg, i.p.) significantly induced locomotion in adolescents (***p < 0.001 vs. vehicle). Fluoxetine (1mg/kg, i.v.)-pretreated adolescents showed enhanced locomotor activity following administration of quinpirole (++p < 0.01 vs. saline), an effect that was blocked by pretreatment with WAY-100,635 (100 µg/kg, i.v.; +++p = 0.001 vs. FLX+WAY). WAY-100,635 pretreatment alone did not alter quinpirole-induced locomotor activity (n = 9 saline-vehicle, 9 saline-quinpriole, 10 FLX-vehicle, 10 FLX-quinpirole, 9 WAY-vehicle, 9 WAY-quinpirole, 11 FLX+WAY-vehicle, 9 FLX+WAY-quinpirole). (B) In adults, there were no significant effects of pretreatment or quinpirole on locomotor activity across groups (n = 9 saline-vehicle, 8 saline-quinpriole, 9 FLX-vehicle, 8 FLX-quinpirole, 8 WAY-vehicle, 8 WAY-quinpirole, 8 FLX+WAY-vehicle, 8 FLX+WAY-quinpirole). Data analyzed with analyzed by 3-way ANOVA, with Bonferroni post hoc tests. Bars represent mean ± SEM.
To examine the role of endogenous 5-HT in drug reinforcement, we evaluated the effect of fluoxetine pretreatment on subsequent cocaine self-administration in adolescents (Figure 2). A significant effect of Response (F1,47 = 23.095, p < 0.001) and a Response x Pretreatment interaction (F3,47 = 14.912, p < 0.001) were observed. Whereas saline-pretreated adolescent rats did not discriminate between reinforced and non-reinforced nosepokes in a novel self-administration chamber, those pretreated with fluoxetine exhibited significantly higher preference for the reinforced hole (p < 0.001) and higher reinforced responses than saline-pretreated controls (p < 0.001). Enhancement of cocaine reinforcement by fluoxetine treatment was blocked by co-administration of WAY-100,635, indicating that the effect was mediated by 5-HT1A receptors. In contrast, fluoxetine pretreatment had no effect on initial cocaine self-administration in adults (Supplementary Figure 3).
Figure 2 Fluoxetine pretreatment during adolescence enhanced cocaine self-administration, an effect blocked by co-administration of WAY-100,635. Fluoxetine (1mg/kg, i.v.)-pretreated adolescents had significantly higher reinforced responses for cocaine (0.5 mg/kg/inf, i.v.) compared to non-reinforced responses (***p < 0.001). Fluoxetine-pretreated adolescents also had greater total reinforced responses for cocaine compared to other pretreatment groups (+++p < 0.001 vs. saline, +p < 0.05 vs. WAY and FLX+WAY). WAY-100,635 (100 µg/kg, i.v.) alone did not alter cocaine self-administration. Data were analyzed by 2-way ANOVA, with repeated measures on Responses. Significant effects of reinforcement were analyzed by Bonferroni-corrected paired t-tests for each pretreatment group. Bars represent mean ± SEM. n = 13 saline, 14 FLX, 13 WAY, 11 FLX + WAY.
Adolescent treatment with fluoxetine or nicotine induces persistent alteration in 5-HT1A receptor signaling, even after drug pretreatment has ended. Following adolescent pretreatment with either drug, enhanced quinpirole-induced locomotor activity is observed which is blocked by acute administration of the 5-HT1A receptor antagonist, WAY-100,635, or partial agonist, S-15535 (Figure 3). A significant overall effect of Antagonist (F2,131 = 10.195, p < 0.001) and a Pretreatment x Antagonist interaction (F4,131 = 4.488, p < 0.01) were observed. Pretreatment with nicotine or fluoxetine significantly enhanced quinpirole-induced locomotion in controls (p < 0.001), but not in animals treated acutely with WAY-100,635 or S-15535 (Figure 3).
Figure 3 Acute 5-HT1A receptor antagonism blocks enhancement of quinpirole-induced locomotion in nicotine- and fluoxetine-pretreated adolescent rats. Nicotine and fluoxetine pretreatment enhance quinpirole-induced locomotor activity (+++p < 0.001 vs. saline pretreatment). Acute administration of WAY-100,635 (100 µg/kg, i.v.) or S-15535 (300 µg/kg, i.v.) blocks the enhancement of quinpirole-induced locomotion in adolescents pretreated with nicotine (60 µg/kg, i.v.) or fluoxetine (1mg/kg, i.v.) (**p < 0.01 vs vehicle). Data analyzed with analyzed by 2-way ANOVA, with Bonferroni post hoc tests. Bars represent mean ± SEM. n = 25 saline-vehicle, 23 saline-WAY, 12 saline-S-15535, 28 nicotine-vehicle, 14 nicotine-WAY, 12 nicotine-S-15535, 12 fluoxetine-vehicle, 12 fluoxetine-WAY, 12 fluoxetine-S-15535.
Following nicotine or fluoxetine pretreatment, enhanced acquisition of cocaine self-administration is seen in adolescent rats, with a significant effect of Pretreatment (F2,62 = 5.748, p < 0.01), Response (F1,62 = 47.807, p < 0.001), and a Response x Pretreatment interaction (F2,62 = 10.882, p < 0.001). Both nicotine and fluoxetine pretreatment significantly increased reinforced responses and preference for the reinforced hole compared to saline-pretreated controls (p < 0.001; Figure 4A). These pretreatment effects on acquisition of cocaine self-administration were no longer present following acute blockade of 5-HT1A receptors with WAY-100,635 (Figure 4B) or acute blockade of D2 receptors with L-741,626 (Figure 4C). In contrast, pretreatment had no effect on initial sucrose self-administration in adolescents (Supplementary Figure 4).
Figure 4 Post-treatment 5-HT1A and D2 receptor activation mediate enhancement of cocaine self-administration after nicotine and fluoxetine pretreatment. (A) Adolescent rats pretreated with nicotine (60 µg/kg, i.v.) or fluoxetine (2 mg/kg, i.v.) had significantly greater reinforced responses compared to non-reinforced responses (***p < 0.001) and higher reinforced responses for cocaine (+++p < 0.001 vs. saline) (n = 21 saline-veh, 21 nicotine-veh, 23 fluoxetine-veh). Enhancements of cocaine self-administration are no longer present after (B) acute 5-HT1A receptor antagonism with WAY-100,635 (100 µg/kg, i.v.) (n = 8 saline-WAY, 7 nicotine-WAY, 8 fluoxetine-WAY) or (C) acute D2 receptor antagonism with L-741,626 (2 mg/kg, i.p.) (n = 12 saline-L-741626, 11 nicotine- L-741626, 11 fluoxetine- L-741626). Data were analyzed by 2-way ANOVA, with repeated measures on Responses. Significant effects of reinforcement were analyzed by Bonferroni-corrected paired t-tests for each pretreatment group. Bars represent mean ± SEM.
Given behavioral evidence of changes in 5-HT1A receptor activity following pretreatment with fluoxetine or nicotine in adolescents, we used a neuroanatomical approach to assess the regional specificity of these functional effects (Supplementary Table 1). With both pretreatments, drug- and region-specific changes were observed in subsequent acute stimulation of [35S]GTPγS binding to G proteins by the 5-HT1A receptor agonist, 8-OH-DPAT. In the infralimbic cortex (IL), adolescent fluoxetine pretreatment enhanced 5-HT1A receptor activity, with a significant overall effect of Pretreatment [F2,52 = 3.754, p<0.05] and an Age × Pretreatment interaction [F2,52 = 4.630, p<0.05]. Post hoc analysis showed that adolescent rats pretreated with fluoxetine had significantly greater 8-OH-DPAT stimulated [35S]GTPγS binding in the IL compared to saline-pretreated rats (p<0.01; Table 1). These effects were not seen in adolescents pretreated with nicotine or in adults.
Table 1 Nicotine and fluoxetine age-specifically and region-specifically alters 5-HT1AR activity in the infralimbic cortex (IL), medial amygdala (MeA), and primary motor cortex (M1).
Adolescent nicotine pretreatment enhanced 5-HT1A receptor activity in the medial amygdala, with significant overall effects of Pretreatment [F2,55 = 6.389, p<0.01] and Age [F1,55 = 5.547, p<0.05]. Post hoc analysis showed that adolescent rats pretreated with nicotine had significantly greater 8-OH-DPAT stimulated [35S]GTPγS binding in this region compared to saline-pretreated rats (p<0.05; Table 1). These effects were not seen in adolescents pretreated with fluoxetine or in adults.
The primary motor cortex was the only region to show significant enhancement of 5-HT1A receptor activity following adolescent pretreatment with either fluoxetine or nicotine, with a significant overall Age × Pretreatment interaction [F2,54 = 4.919, p<0.05]. Post hoc analysis showed that both nicotine- and fluoxetine-pretreated adolescent rats had significantly higher 8-OH-DPAT stimulated [35S]GTPγS binding than saline-pretreated controls (p<0.05; Table 1). Drug pretreatment had no significant effects on 5-HT1A receptor activity in adult.
Consistent with a growing literature (3, 20, 40), we have shown that adolescents and adults respond quite differently to commonly used drugs, including nicotine and the SSRI, fluoxetine. Both drugs act via the 5-HT system to induce age-dependent changes in 5-HT1AR and D2 receptor activity. During adolescence, it is widely accepted that DA systems actively mature, whereas 5-HT systems exhibit comparatively low developmental activity since 5-HT neurons are among the first to mature during early brain development (41–43). In contrast, our present findings suggest that there are unique 5HT-DA interactions that occur during adolescence that influence the maturation of brain reward systems.
Brief nicotine pretreatment during early adolescence has been shown to uniquely enhance DA-mediated behaviors, including cocaine self-administration, conditioned place preference, and locomotor sensitization as well as ambulatory activity induced by the D2 receptor agonist, quinpirole,32–36. We have previously shown that adolescent nicotine enhancement of quinpirole locomotion and cocaine self-administration is both mediated by 5-HT release and blocked by co-administration of selective 5-HT1AR antagonists (33). These findings are consistent with prior reports of unique activation of raphe by adolescent nicotine treatment (44). We now demonstrate that increased 5-HT activation of 5-HT1ARs, resulting from fluoxetine blockade of transporter function, also increases quinpirole-induced locomotor activity and cocaine self-administration in adolescents but not adults. In the absence of a drug pretreatment, there are no age differences in the acquisition of cocaine self-administration (44), which indicates that endogenous 5-HT signaling regulates adolescent behavioral sensitivity to cocaine.
Treatment with 5-HT1AR antagonists during behavioral testing eliminates fluoxetine and nicotine pretreatment effects on quinpirole locomotion and cocaine reinforcement. This finding indicates that persistent enhancement of 5-HT1AR function mediates the behavioral effects of prior adolescent nicotine and fluoxetine exposure even after discontinuation of drug treatment. Since 5-HT1ARs are located both pre- and post-synaptically (45), we compared the behavioral effects of two antagonists with differing pharmacological profiles to determine which 5-HT1ARs mediated adolescent fluoxetine and nicotine effects. As an antagonist, WAY-100,635 is equally effective at pre- and post-synaptic 5-HT1ARs. In contrast, S-15535 is a partial 5-HT1AR agonist, which acts functionally as an antagonist at postsynaptic receptors, but as an agonist at presynaptic autoreceptors (46). As with WAY-100635, acute administration of S-15535 immediately before behavioral testing blocked nicotine and fluoxetine enhancement of quinpirole-induced locomotion, indicating an involvement of postsynaptic 5-HT1ARs.
Prior studies have shown that chronic fluoxetine leads to increased 5-HT system responsiveness after drug cessation, possibly because of 5-HT1AR autoreceptor desensitization (47). However, this effect is seen in both adolescents and adults, and does not result in changes in baseline monoamine levels. It is therefore unlikely to explain the increased postsynaptic 5-HT1AR sensitivity that we have observed following subchronic fluoxetine treatment in adolescents. Furthermore, using an in vitro approach to examine receptor-G protein coupling, we have shown unique effects of adolescent fluoxetine and nicotine exposure on 5-HT1AR function.
Fluoxetine enhanced 5-HT1AR activity in the IL, a prefrontal cortical region important for emotional learning and habit behavior (48, 49). 5-HT1ARs in this region have been implicated in modulating emotional response to stressful situations (50). In contrast, nicotine enhanced 5-HT1AR activity in the medial amygdala, a region of the amygdala implicated in control of sexual and social behaviors (51, 52). 5-HT1ARs in this region have been implicated in innate fear modulation (53). However, both fluoxetine and nicotine both enhanced 5-HT1AR activity only in the M1, a cortical motor region that experiences continuous growth in serotoninergic innervation until late adolescence (54). The M1 is actively engaged during initial motor consolidation and plays a critical role in acquisition of skill learning (55). 5-HT1ARs within M1 decrease corticostriatal activity (56) and modulate DA-mediated motor function (57). Since both fluoxetine and nicotine enhanced 5-HT1ARs activity within this region, it is possible that M1 may be the site of action of these drugs in enhancing adolescent DA-mediated behaviors.
The induction of locomotor activity by quinpirole in adolescents is mediated by D2 receptors and is age-specific (36). As confirmed in the present study, adults do not show a locomotor response to quinpirole (33, 58), a finding that is consistent with the maturation of DA systems and D2 receptor function that occurs during adolescence (1, 3, 5). We have previously shown that adolescent nicotine pretreatment enhances quinpirole-induced locomotor activity via a 5-HT1AR mechanism and increases D2 receptor expression and functional activity in the striatum (32, 33). Involvement of the D2 receptor is further indicated by the finding that D2 receptor blockade or knockdown during nicotine pretreatment of adolescents blocks subsequent enhancement of cocaine self-administration via a microglia-specific mechanism (33). We now show that fluoxetine pretreatment enhances adolescent locomotor response to quinpirole, indicating that increased serotonergic signaling during pretreatment potentiates subsequent D2 receptor function in this age group. Adolescent nicotine and fluoxetine enhancement of cocaine self-administration is also mediated by increased functional activity of D2 receptors, since the effect is blocked by treatment with a D2-specific antagonist, L-741,626, during self-administration testing. The finding that cocaine self-administration in adolescents is mediated by D2 receptors is in marked contrast to adults, where D1 receptors mediate cocaine reinforcement (59). Indeed, D2 receptors do not mediate cocaine self-administration in adults but are, instead, involved in mechanisms that limit intake of high-dose cocaine (60).
As shown in the present study, fluoxetine and nicotine enhancement of DA-mediated behaviors in adolescents are mediated by functional sensitization of both 5-HT1AR and D2 receptors. Enhanced behavioral response following pretreatment with either drug is eliminated by administration of either 5-HT1AR or D2 receptor antagonists immediately prior to testing. This suggests that there is a close interaction of 5-HT1AR and D2 receptors in adolescent brain that modulates reward circuitry. The mechanisms underlying such coupling of receptor activity are presently unknown but may either involve direct receptor interaction or a circuit based mechanism. Further studies will be required to evaluate the exact nature of this linked receptor response.
The findings in this report are subject to at least three limitations. First, this study was conducted in males since we have previously shown that adolescent nicotine enhancement of cocaine self-administration is seen in both males and females (32, 33). However, further studies will be required to determine whether this is also the case for fluoxetine in females. Second, pretreatment with nicotine does not fully capture the myriad of other psychoactive constituents that are present in tobacco leaves, combustible tobacco products, or e-cigarette vaping liquids, and further investigations are needed in order to characterize how these other psychoactive constituents interact with nicotine and fluoxetine. Finally, since adolescent and adult rats received pretreatment for 4 consecutive days and behavioral testing was conducted the following day, our study is limited to the short-term effects of drug exposure and acute pharmacological interventions. Additional studies exploring the long-term consequences of drug exposure during adolescence could better inform the effectiveness of pharmacological interventions on behavior later in adulthood.
With increasing evidence that aberrant activation of 5-HT systems during adolescence triggers lasting changes in neuronal signaling, use of drugs such as tobacco, e-cigarettes, and antidepressants may permanently alter the course of adolescent brain maturation and neurotransmitter signaling. Although teen smoking is declining, teen vaping has increased greatly in recent years (6, 7) and has been suggested to be a gateway to future tobacco use (61, 62). We have now shown that nicotine disrupts normative limbic development and sensitizes the adolescent brain to DA drugs such as cocaine. Thus, as with tobacco, e-cigarettes may prime behavioral susceptibility to drugs of abuse in teenagers with lifelong consequences for mental health.
Treatments for mental health disorders in teens also pose serious safety concerns. Even though fluoxetine is FDA approved to treat teen depression, the majority of testing regarding its effectiveness and safety has been conducted in adults (63, 64). In addition, fluoxetine’s antidepressant effects require chronic administration in order to be therapeutic, suggesting postsynaptic neuroadaptations in 5-HT receptor signaling (65). However, we have demonstrated that changes in adolescent behavior after nicotine or fluoxetine exposure can be blocked with an acute treatment of a 5-HT1AR antagonist or partial agonist.
Prior studies have shown that 5-HT1AR antagonists are safe for clinical use. The delayed antidepressant effects of SSRIs can be reduced by co-treatment of pindolol, a nonselective beta blocker with 5-HT1AR antagonist activity (66, 67). The 5-HT1AR partial agonist busipirone – which has long been used to treat anxiety – also demonstrates clinical efficacy when given in combination with SSRIs (68). Recently, vilazodone, which has combined SSRI and 5-HT1AR partial agonist properties, has been approved for treatment of major depressive disorder in adults (69). Since we have shown that 5-HT1AR antagonists and partial agonists attenuate adverse behavioral responses to antidepressants in adolescents, treatment for teen depression should take into consideration potential drug therapies with 5-HT1AR antagonist activity to improve antidepressant efficacy, particularly during the initial period when adolescents are most vulnerable to the confounding effects of SSRI treatment. Furthermore, this body of work indicates that 5-HT1ARs are also viable drug targets for the treatment of adverse health consequences after adolescent exposure to tobacco, e-cigarettes, and antidepressants.
The raw data supporting the conclusions of this article will be made available by the authors, without undue reservation.
The animal study was approved by Institutional Animal Care and Use Committee (IACUC). The study was conducted in accordance with the local legislation and institutional requirements.
MY: Conceptualization, Formal analysis, Methodology, Project administration, Visualization, Writing – original draft, Writing – review & editing. FL: Conceptualization, Funding acquisition, Methodology, Resources, Supervision, Writing – original draft, Writing – review & editing.
The author(s) declare financial support was received for the research, authorship, and/or publication of this article. The study was funded by the National Institutes of Health, National Institute on Drug Abuse (NIH DA 21267) to investigate mechanisms of vulnerability to drugs of abuse.
The authors thank Drs. Sandra Loughlin and James Belluzzi for providing their subject-matter expertise and feedback. The authors also thank Michelle Cano for editing and project management support.
The authors declare that the research was conducted in the absence of any commercial or financial relationships that could be construed as a potential conflict of interest.
All claims expressed in this article are solely those of the authors and do not necessarily represent those of their affiliated organizations, or those of the publisher, the editors and the reviewers. Any product that may be evaluated in this article, or claim that may be made by its manufacturer, is not guaranteed or endorsed by the publisher.
The Supplementary Material for this article can be found online at: https://www.frontiersin.org/articles/10.3389/fpsyt.2024.1380123/full#supplementary-material
Supplementary Table 1 | Regional 5-HT1A receptor activity after nicotine or fluoxetine pretreatment in adolescent and adult brain slices. [35S]GTPγS binding is expressed (Mean +/- SEM) as % of basal. Asterisks (*) denote significant differences from saline. n = 9-11/ group.
Supplementary Figure 1 | Experimental timeline. Adolescents are represented in red, and adults are in black.
Supplementary Figure 2 | Representative autoradiograms for 8-OH-DPAT-stimulated [35S]GTPγS binding. Sample images were taken from adult (P90) brain slices after saline pretreatment.
Supplementary Figure 3 | Nicotine and fluoxetine pretreatments did not alter cocaine self-administration during adulthood. There were no significant differences in responses between pretreatment groups during cocaine self-administration in adult rats. n = 8-10/group.
Supplementary Figure 4 | Nicotine and fluoxetine pretreatments did not alter sucrose self-administration during adolescence. There were no significant differences in responses between pretreatment groups during sucrose self-administration in adolescent rats. n = 6/group.
1. O’Donnell P. Adolescent maturation of cortical dopamine. Neurotox Res. (2010) 18:306–12. doi: 10.1007/s12640-010-9157-3
2. Wahlstrom D, Collins P, White T, Luciana M. Developmental changes in dopamine neurotransmission in adolescence: behavioral implications and issues in assessment. Brain Cognit. (2010) 72:146–59. doi: 10.1016/j.bandc.2009.10.013
3. Yuan M, Cross SJ, Loughlin SE, Leslie FM. Nicotine and the adolescent brain. J Physiol. (2015) 593:1469–7793.x. doi: 10.1113/JP270492
4. Kessler RC, Berglund P, Demler O, Jin R, Merikangas KR, Walters EE. Lifetime prevalence and age-of-onset distributions of DSM-IV disorders in the National Comorbidity Survey Replication. Arch Gen Psychiatry. (2005) 62:593–602. doi: 10.1001/archpsyc.62.6.593
5. Crews F, He J, Hodge C. Adolescent cortical development: a critical period of vulnerability for addiction. Pharmacol Biochem Behav. (2007) 86:189–99. doi: 10.1016/j.pbb.2006.12.001
6. Cullen KA, Ambrose BK, Gentzke AS, Apelberg BJ, Jamal A, King BA. Notes from the field: use of electronic cigarettes and any tobacco product among middle and high school students — United states, 2011–2018. MMWR. Morbidity Mortality Weekly Rep. (2018) 67:1276–7. doi: 10.15585/mmwr.mm6745a5
7. Miech R, Johnston L, O’Malley PM, Bachman JG, Patrick ME. Adolescent vaping and nicotine use in 2017–2018 — U.S. National estimates. New Engl J Med. (2019) 380:192–3. doi: 10.1056/NEJMc1814130
8. Azagba S, Manzione L, Shan L, King J. Trends in smoking behaviors among US adolescent cigarette smokers. Pediatrics. (2020) 145. doi: 10.1542/peds.2019-3047
9. Leventhal AM, Strong DR, Kirkpatrick MG, Unger JB, Sussman S, Riggs NR, et al. Association of electronic cigarette use with initiation of combustible tobacco product smoking in early adolescence. Jama. (2015) 314:700. doi: 10.1001/jama.2015.8950
10. SAMHSA Substance Abuse and Mental Health Services Administration. Results from the 2010 national survey on drug use and health: summary of national findings. N series H-41. Rockville (MD: U.S. Department of Health and Human Services, Substance Abuse and Mental Health Services Administration (2011).
11. Kandel DB, Yamaguchi K, Chen K. Stages of progression in drug involvement from adolescence to adulthood: further evidence for the gateway theory. J Stud Alcohol. (1992) 53:447–57. doi: 10.15288/jsa.1992.53.447
12. Lai S, Lai H, Page JB, McCoy CB. The association between cigarette smoking and drug abuse in the United States. J Addict Dis. (2000) 19:11–24. doi: 10.1300/J069v19n04_02
13. Degenhardt L, Dierker L, Chiu WT, Medina-Mora ME, Neumark Y, Sampson N, et al. Evaluating the drug use "gateway" theory using cross-national data: consistency and associations of the order of initiation of drug use among participants in the WHO World Mental Health Surveys. Drug Alcohol Depend. (2010) 108:84–97. doi: 10.1016/j.drugalcdep.2009.12.001
14. Mccabe SE, West BT, Veliz P, Boyd CJ. E-cigarette use, cigarette smoking, dual use, and problem behaviors among U.S. Adolescents: results from a national survey. J Adolesc Health. (2017) 61:155–62. doi: 10.1016/j.jadohealth.2017.02.004
15. McKenzie M, Olsson CA, Jorm AF, Romaniuk H, Patton GC. Association of adolescent symptoms of depression and anxiety with daily smoking and nicotine dependence in young adulthood: findings from a 10-year longitudinal study. Addiction. (2010) 105:1652–9. doi: 10.1111/j.1360-0443.2010.03002.x
16. Walkup JT. Antidepressant efficacy for depression in children and adolescents: industry- and NIMH-funded studies. Am J Psychiatry (2017) 174(5):430–7. doi: 10.1176/appi.ajp.2017.16091059
17. March J, Silva S, Petrycki S, Curry J, Wells K, Fairbank J, et al. Fluoxetine, cognitive-behavioral therapy, and their combination for adolescents with depression: Treatment for Adolescents With Depression Study (TADS) randomized controlled trial. JAMA. (2004) 292:807–20. doi: 10.1001/jama.292.7.807
18. Whittington CJ, Kendall T, Fonagy P, Cottrell D, Cotgrove A, Boddington E. Selective serotonin reuptake inhibitors in childhood depression: systematic review of published versus unpublished data. Lancet. (2004) 363:1341–5. doi: 10.1016/S0140-6736(04)16043-1
19. Usala T, Clavenna A, Zuddas A, Bonati M. Randomised controlled trials of selective serotonin reuptake inhibitors in treating depression in children and adolescents: a systematic review and meta-analysis. Eur Neuropsychopharmacol. (2008) 18:62–73. doi: 10.1016/j.euroneuro.2007.06.001
20. Spear LP. The adolescent brain and age-related behavioral manifestations. Neurosci Biobehav Rev. (2000) 24:417–63. doi: 10.1016/S0149-7634(00)00014-2
21. Karanges EA, Kashem MA, Sarker R, Ahmed EU, Ahmed S, van Nieuwenhuijzen P, et al. Hippocampal protein expression is differentially affected by chronic paroxetine treatment in adolescent and adult rats: a possible mechanism of "paradoxical" antidepressant responses in young persons. Front Pharmacol. (2013) 4:86. doi: 10.3389/fphar.2013.00086
22. Hodes GE, Yang L, Van Kooy J, Santollo J, Shors TJ. Prozac during puberty: distinctive effects on neurogenesis as a function of age and sex. Neuroscience. (2009) 163:609–17. doi: 10.1016/j.neuroscience.2009.06.057
23. Vastola BJ, Douglas LA, Varlinskaya EI, Spear LP. Nicotine-induced conditioned place preference in adolescent and adult rats. Physiol Behav. (2002) 77:107–14. doi: 10.1016/S0031-9384(02)00818-1
24. Belluzzi JD, Lee AG, Oliff HS, Leslie FM. Age-dependent effects of nicotine on locomotor activity and conditioned place preference in rats. Psychopharmacol (Berl). (2004) 174:389–95. doi: 10.1007/s00213-003-1758-6
25. Shram MJ, Funk D, Li Z, Lê AD. Periadolescent and adult rats respond differently in tests measuring the rewarding and aversive effects of nicotine. Psychopharmacol (Berl). (2006) 186:201–8. doi: 10.1007/s00213-006-0373-8
26. Brielmaier JM, McDonald CG, Smith RF. Immediate and long-term behavioral effects of a single nicotine injection in adolescent and adult rats. Neurotoxicol Teratol. (2007) 29:74–80. doi: 10.1016/j.ntt.2006.09.023
27. Kota D, Martin BR, Robinson SE, Damaj MI. Nicotine dependence and reward differ between adolescent and adult male mice. J Pharmacol Exp Ther. (2007) 322:399–407. doi: 10.1124/jpet.107.121616
28. Torres OV, Tejeda HA, Natividad LA, O'Dell LE. Enhanced vulnerability to the rewarding effects of nicotine during the adolescent period of development. Pharmacol Biochem Behav. (2008) 90:658–63. doi: 10.1016/j.pbb.2008.05.009
29. Adriani W, Macri S, Pacifici R, Laviola G. Peculiar vulnerability to nicotine oral self-administration in mice during early adolescence. Neuropsychopharmacology. (2002) 27:212–24. doi: 10.1016/S0893-133X(02)00295-6
30. O’Dell LE, Bruijnzeel AW, Smith RT, Parsons LH, Merves ML, Goldberger BA, et al. Diminished nicotine withdrawal in adolescent rats: implications for vulnerability to addiction. Psychopharmacol (Berl). (2006) 186:612–9. doi: 10.1007/s00213-006-0383-6
31. Shram MJ, Siu EC, Li Z, Tyndale RF, Le AD. Interactions between age and the aversive effects of nicotine withdrawal under mecamylamine-precipitated and spontaneous conditions in male Wistar rats. Psychopharmacol (Berl). (2008) 198:181–90. doi: 10.1007/s00213-008-1115-x
32. Linker KE, Gad M, Tawadrous P, Cano M, Green KN, Wood MA, et al. Microglial activation increases cocaine self-administration following adolescent nicotine exposure. Nat Commun. (2020) 11:306. doi: 10.1038/s41467-019-14173-3
33. Dao JM, McQuown SC, Loughlin SE, Belluzzi JD, Leslie FM. Nicotine alters limbic function in adolescent rat by a 5-HT1A receptor mechanism. Neuropsychopharmacology. (2011) 36:1319–31. doi: 10.1038/npp.2011.8
34. McQuown SC, Belluzzi JD, Leslie FM. Low dose nicotine treatment during early adolescence increases subsequent cocaine reward. Neurotoxicol Teratol. (2007) 29:66–73. doi: 10.1016/j.ntt.2006.10.012
35. McQuown SC, Dao JM, Belluzzi JD, Leslie FM. Age-dependent effects of low-dose nicotine treatment on cocaine-induced behavioral plasticity in rats. Psychopharmacol (Berl). (2009) 207:143–52. doi: 10.1007/s00213-009-1642-0
36. Mojica CY, Dao JM, Yuan M, Loughlin SE, Leslie FM. Nicotine modulation of adolescent dopamine receptor signaling and hypothalamic peptide response. Neuropharmacology. (2014) 77:285–93. doi: 10.1016/j.neuropharm.2013.10.012
37. Alajaji M, Lazenka MF, Kota D, Wise LE, Younis RM, Carroll FI, et al. Early adolescent nicotine exposure affects later-life cocaine reward in mice. Neuropharmacology. (2016) 105:308–17. doi: 10.1016/j.neuropharm.2016.01.032
38. Hensler JG, Advani T, Monteggia LM. Regulation of serotonin-1A receptor function in inducible brain-derived neurotrophic factor knockout mice after administration of corticosterone. Biol Psychiatry. (2007) 62:521–9. doi: 10.1016/j.biopsych.2006.10.015
39. Broide RS, Robertson RT, Leslie FM. Regulation of alpha7 nicotinic acetylcholine receptors in the developing rat somatosensory cortex by thalamocortical afferents. J Neurosci. (1996) 16:2956–71. doi: 10.1523/JNEUROSCI.16-09-02956.1996
40. Spear LP. Adolescent alcohol exposure: are there separable vulnerable periods within adolescence? Physiol Behav. (2015) 148:122–30. doi: 10.1016/j.physbeh.2015.01.027
41. Dinopoulos A, Dori I, Parnavelas JG. The serotonin innervation of the basal forebrain shows a transient phase during development. Brain Res Dev Brain Res. (1997) 99:38–52. doi: 10.1016/S0165-3806(96)00198-8
42. Dori IE, Dinopoulos A, Parnavelas JG. The development of the synaptic organization of the serotonergic system differs in brain areas with different functions. Exp Neurol. (1998) 154:113–25. doi: 10.1006/exnr.1998.6937
43. Rubenstein JLR. Development of serotonergic neurons and their projections. Biol Psychiatry. (1998) 44:145–50. doi: 10.1016/S0006-3223(98)00133-4
44. Bang SJ, Commons KG. Age-dependent effects of initial exposure to nicotine on serotonin neurons. Neuroscience. (2011) 179:1–8. doi: 10.1016/j.neuroscience.2011.01.032
45. Hoyer D, Hannon JP, Martin GR. Molecular, pharmacological and functional diversity of 5-HT receptors. Pharmacol Biochem Behav. (2002) 71:533–54. doi: 10.1016/S0091-3057(01)00746-8
46. Millan MJ, Rivet JM, Canton H, Lejeune F, Gobert A, Widdowson P, et al. S 15535: a highly selective benzodioxopiperazine 5-HT1A receptor ligand which acts as an agonist and an antagonist at presynaptic and postsynaptic sites respectively. Eur J Pharmacol. (1993) 230:99–102. doi: 10.1016/0014-2999(93)90416-F
47. Klomp A, Vaclavu L, Meerhoff GF, Reneman L, Lucassen PJ. Effects of chronic fluoxetine treatment on neurogenesis and tryptophan hydroxylase expression in adolescent and adult rats. PloS One. (2014) 9:e97603. doi: 10.1371/journal.pone.0097603
48. Chudasama Y, Robbins TW. Dissociable contributions of the orbitofrontal and infralimbic cortex to pavlovian autoshaping and discrimination reversal learning: further evidence for the functional heterogeneity of the rodent frontal cortex. J Neurosci. (2003) 23:8771–80. doi: 10.1523/JNEUROSCI.23-25-08771.2003
49. Killcross S, Coutureau E. Coordination of actions and habits in the medial prefrontal cortex of rats. Cereb Cortex. (2003) 13:400–8. doi: 10.1093/cercor/13.4.400
50. Marinho ALZ, Vila-Verde C, Fogaca MV, Guimarães FS. Effects of intra-infralimbic prefrontal cortex injections of cannabidiol in the modulation of emotional behaviors in rats: contribution of 5HT1A receptors and stressful experiences. Behav Brain Res. (2015) 286:49–56. doi: 10.1016/j.bbr.2015.02.023
51. Parfitt DB, Newman SW. Fos-immunoreactivity within the extended amygdala is correlated with the onset of sexual satiety. Horm Behav. (1998) 34:17–29. doi: 10.1006/hbeh.1998.1459
52. Newman SW. The medial extended amygdala in male reproductive behavior. A node in the mammalian social behavior network. Ann N Y Acad Sci. (1999) 877:242–57. doi: 10.1111/j.1749-6632.1999.tb09271.x
53. de Paula BB, Leite-Panissi CRA. Distinct effect of 5-HT1A and 5-HT2A receptors in the medial nucleus of the amygdala on tonic immobility behavior. Brain Res. (2016) 1643:152–8. doi: 10.1016/j.brainres.2016.04.073
54. Dori I, Dinopoulos A, Blue ME, Parnavelas JG. Regional differences in the ontogeny of the serotonergic projection to the cerebral cortex. Exp Neurol. (1996) 138:1–14. doi: 10.1006/exnr.1996.0041
55. Muellbacher W, Ziemann U, Boroojerdi B, Cohen L, Hallett M. Role of the human motor cortex in rapid motor learning. Exp Brain Res. (2001) 136:431–8. doi: 10.1007/s002210000614
56. Antonelli T, Fuxe K, Tomasini MC, Bartoszyk GD, Seyfried CA, Tanganelli S, et al. Effects of sarizotan on the corticostriatal glutamate pathways. Synapse (New York N.Y.). (2005) 58:193–9. doi: 10.1002/syn.20195
57. Ostock CY, Dupre KB, Jaunarajs KL, Walters H, George J, Krolewski D, et al. Role of the primary motor cortex in L-Dopa-induced dyskinesia and its modulation by 5-HT1A receptor stimulation. Neuropharmacology. (2011) 61:753–60. doi: 10.1016/j.neuropharm.2011.05.021
58. Frantz KJ, Van Hartesveldt C. The locomotor effects of quinpirole in rats depend on age and gender. Pharmacol Biochem Behav. (1999) 64:821–6. doi: 10.1016/S0091-3057(99)00162-8
59. Caine SB, Thomsen M, Gabriel KI, Berkowitz JS, Gold LH, Koob GF, et al. Lack of self-administration of cocaine in dopamine D1 receptor knock-out mice. J Neurosci. (2007) 27:13140–50. doi: 10.1523/JNEUROSCI.2284-07.2007
60. Caine SB, Negus SS, Mello NK, Patel S, Bristow L, Kulagowski J, et al. Role of dopamine D2-like receptors in cocaine self-administration: studies with D2 receptor mutant mice and novel D2 receptor antagonists. J Neurosci. (2002) 22:2977–88. doi: 10.1523/JNEUROSCI.22-07-02977.2002
61. Soneji S, Barrington-Trimis JL, Wills TA, Leventhal AM, Unger JB, Gibson LA, et al. Association between initial use of e-cigarettes and subsequent cigarette smoking among adolescents and young adults. JAMA Pediatr. (2017) 171:788. doi: 10.1001/jamapediatrics.2017.1488
62. Barrington-Trimis JL, Yang Z, Schiff S, Unger J, Cruz TB, Urman R, et al. E-cigarette product characteristics and subsequent frequency of cigarette smoking. Pediatrics. (2020) 145. doi: 10.1542/peds.2019-1652
63. Coyle JT, Pine DS, Charney DS, Lewis L, Nemeroff CB, Carlson GA, et al. Depression and bipolar support alliance consensus statement on the unmet needs in diagnosis and treatment of mood disorders in children and adolescents. J Am Acad Child Adolesc Psychiatry. (2003) 42:1494–503. doi: 10.1097/00004583-200312000-00017
64. Olivier JD, Blom T, Arentsen T, Homberg JR. The age-dependent effects of selective serotonin reuptake inhibitors in humans and rodents: A review. Prog Neuropsychopharmacol Biol Psychiatry. (2011) 35:1400–8. doi: 10.1016/j.pnpbp.2010.09.013
65. Racagni G, Popoli M. Cellular and molecular mechanisms in the long-term action of antidepressants. Dialogues Clin Neurosci. (2008) 10:385–400. doi: 10.31887/DCNS.2008.10.4/gracagni
66. Artigas F, Perez V, Alvarez E. Pindolol induces a rapid improvement of depressed patients treated with serotonin reuptake inhibitors. Arch Gen Psychiatry. (1994) 51:248–51. doi: 10.1001/archpsyc.1994.03950030084009
67. Blier P, Bergeron R. Effectiveness of pindolol with selected antidepressant drugs in the treatment of major depression. J Clin Psychopharmacol. (1995) 15:217–22. doi: 10.1097/00004714-199506000-00011
68. Appelberg BG, Syvalahti EK, Koskinen TE, Mehtonen OP, Muhonen TT, Naukkarinen HH. Patients with severe depression may benefit from buspirone augmentation of selective serotonin reuptake inhibitors: results from a placebo-controlled, randomized, double-blind, placebo wash-in study. J Clin Psychiatry. (2001) 62:448–52. doi: 10.4088/JCP.v62n0608
Keywords: adolescence, age-dependent, nicotine, fluoxetine, cocaine, behavioral acquisition, self-administration
Citation: Yuan M and Leslie FM (2024) Nicotine and fluoxetine alter adolescent dopamine-mediated behaviors via 5-HT1A receptor activation. Front. Psychiatry 15:1380123. doi: 10.3389/fpsyt.2024.1380123
Received: 01 February 2024; Accepted: 09 May 2024;
Published: 11 June 2024.
Edited by:
Rosana Camarini, University of São Paulo, BrazilReviewed by:
Ian Alfredo Mendez, The University of Texas at El Paso, United StatesCopyright © 2024 Yuan and Leslie. This is an open-access article distributed under the terms of the Creative Commons Attribution License (CC BY). The use, distribution or reproduction in other forums is permitted, provided the original author(s) and the copyright owner(s) are credited and that the original publication in this journal is cited, in accordance with accepted academic practice. No use, distribution or reproduction is permitted which does not comply with these terms.
*Correspondence: Menglu Yuan, eXVhbm1AdWNpLmVkdQ==
Disclaimer: All claims expressed in this article are solely those of the authors and do not necessarily represent those of their affiliated organizations, or those of the publisher, the editors and the reviewers. Any product that may be evaluated in this article or claim that may be made by its manufacturer is not guaranteed or endorsed by the publisher.
Research integrity at Frontiers
Learn more about the work of our research integrity team to safeguard the quality of each article we publish.