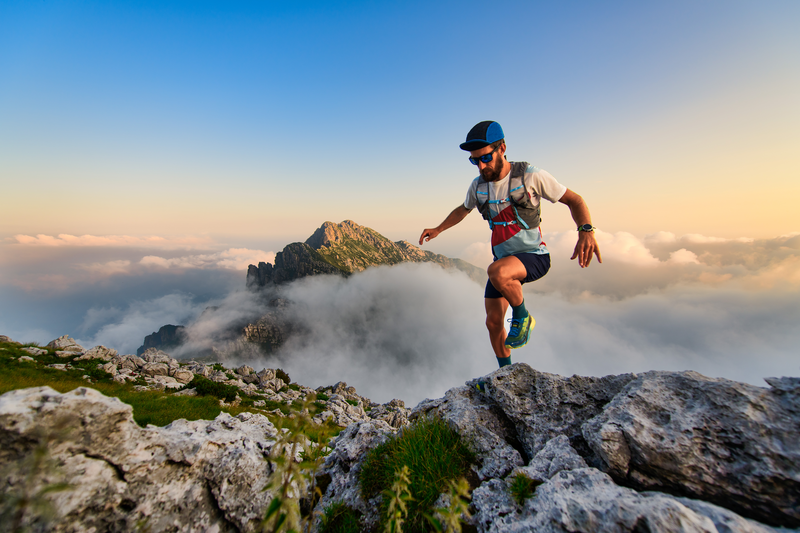
95% of researchers rate our articles as excellent or good
Learn more about the work of our research integrity team to safeguard the quality of each article we publish.
Find out more
ORIGINAL RESEARCH article
Front. Psychiatry , 18 July 2024
Sec. Molecular Psychiatry
Volume 15 - 2024 | https://doi.org/10.3389/fpsyt.2024.1377403
Introduction: Alcohol consumption can induce a neuroinflammatory response and contribute to the progression of neurodegeneration. However, its association with Parkinson’s disease (PD), the second most common neurodegenerative disorder, remains undetermined. Recent studies suggest that the glycoprotein non-metastatic melanoma protein B (GPNMB) is a potential biomarker for PD. We evaluated the association of rs199347, a variant of the GPNMB gene, with alcohol consumption and methylation upstream of GPNMB.
Methods: We retrieved genetic and DNA methylation data obtained from participants enrolled in the Taiwan Biobank (TWB) between 2008 and 2016. After excluding individuals with incomplete or missing information about potential PD risk factors, we included 1,357 participants in our final analyses. We used multiple linear regression to assess the association of GPNMB rs199347 and chronic alcohol consumption (and other potential risk factors) with GPNMB cg17274742 methylation.
Results: There was no difference between the distribution of GPNMB rs199347 genotypes between chronic alcohol consumers and the other study participants. A significant interaction was observed between the GPNMB rs199347 variant and alcohol consumption (p = 0.0102) concerning cg17274742 methylation. Compared to non-chronic alcohol consumers with the AA genotype, alcohol drinkers with the rs199347 GG genotype had significantly lower levels (hypomethylation) of cg17274742 (p = 0.0187).
Conclusion: Alcohol consumption among individuals with the rs199347 GG genotype was associated with lower levels of cg17274742 methylation, which could increase expression of the GPNMB gene, an important neuroinflammatory-related risk gene for PD.
Parkinson’s disease (PD) is the second most common neurodegenerative disorder, affecting >1% of the worldwide population ≥65 years of age, and is characterized primarily by slow movement, tremors, and rigidity (1, 2). The etiology of PD remains unknown, although it is believed to result from a combination of genetic, environmental, and behavioral factors by progressive loss of dopaminergic neurons in the substantia nigra and accumulation of α-synuclein within Lewy bodies (3–5). Pathophysiologically, PD has been associated with α-synuclein misfolding and aggregation, mitochondrial dysfunction, impairment of protein clearance, neuroinflammation, and oxidative stress (6–8). Epigenetic modifications, which can connect environment or behavior factors with the genetic changes underlying disease (9, 10), have been shown to modulate the immune systems of patients with PD (11–22).
Glycoprotein non-metastatic melanoma protein B (GPNMB), a type I transmembrane protein involved in immune cell maturation and activation (23), has been shown to be a promising biomarker of PD risk (24–27). GPNMB is highly expressed in macrophages and microglia, which play a significant role in neuroinflammation, and was shown to be upregulated in disease-associated microglia in neurodegenerative animal models (28, 29). A prominent single-nucleotide polymorphism (SNP) of GPNMB, rs199347, plays a significant role in modulating systemic immune response, especially neuroinflammation (21–26, 30). The rs199347 variant genotype and minor allele involves a guanine instead of the reference alanine (31) and has been identified as highly associated with PD risk (24). Recently, GPNMB was shown to promote the toxic aggregation of the alpha-synuclein protein in substantia nigra, which is believed to contribute to neurodegeneration, and could be a potential target for PD treatment (24, 32). Furthermore, recent proteomic analyses of cerebrospinal fluid have identified GPNMB as a primary causal protein in PD emphasizing its role in the disease’s heterogeneity and causality (33). Notably, studies involving animal models have demonstrated that overexpression of GPNMB can mitigate degeneration of dopaminergic neurons and provide anti-neuroinflammatory benefits (34, 35).The differences among the rs199347 genotypes (AA, AG, and GG) are significantly associated with GPNMB expression in the brain as well as whole blood (24, 25, 27, 36–39). Moreover, large, integrated GWAS on methylation data from brain samples of patients with PD found that the association between GPNMB and PD could be regulated by DNA methylation (25, 27, 39). Indeed, hypomethylation at cg17274742, which is proximal to the 7p15 chromosomal region in which GPNMB is located, is associated with increased GPNMB expression in PD patients (25, 27).
Alcohol consumption can affect epigenetic modification and gene expression (40–42), and associated DNA methylation changes have been observed in both the peripheral and central nervous systems (43, 44). Chronic alcohol consumption can trigger neuroinflammation resulting in central nervous system injury and possibly neurodegeneration (45–48). High alcohol consumption has been shown to increase GPNMB levels (49, 50) suggesting an involvement with PD. However, the connection between alcohol drinking and PD remains poorly understood, and epidemiological studies show contradictory data (51–55). Whether PD is caused by chronic alcohol consumption remains unclear (53–56), although chronic alcohol consumption has been found to drive biological mechanisms with significant changes to DNA methylation in serum and brain tissues (40, 41, 43, 44).
To date, no study has evaluated the role of alcohol intake on the expression of GPNMB, and specifically the PD-associated GPNMB SNP rs199347, and DNA methylation. Identifying methylation patterns associated with alcohol consumption and GPNMB expression could help elucidate the influence of alcohol consumption on the risk of PD.
Data were obtained from the Taiwan Biobank (TWB), an ongoing prospective cohort study of more than 150,000 participants. The TWB contains demographic and whole-genome sequencing data of Taiwanese (99% Han Chinese) without cancer aged 20 to 70 years, with lifestyle information captured through individual interviews (57, 58).
We enrolled all 2,352 individuals aged 20–70 years in the TWB with DNA methylation data. Using previously published epidemiology studies (59–63), we developed a list of potential risk factors for PD as variables for consideration: sex, age, body mass index, cigarette smoking, alcohol drinking, exercise, coffee drinking, uric acid levels, and hypertension. We excluded 995 individuals with incomplete or missing information, and the remaining 1,357 participants with complete information were included in our final analysis.
This study was approved by the Institutional Review Board of Chung Shan Medical University Hospital (CS1–20009).
The TWB assessed DNA methylation from whole blood using the Infinium MethylationEPIC BeadChip Kit (Illumina Inc, San Diego, CA, USA) (64–66). Cell-type heterogeneity was adjusted using the Reference-Free Adjustment for Cell-Type composition (ReFACTor) method (67). Methylation levels were quantified using beta values (0–1), which roughly correspond to the percent methylation at a particular site.
Genotyping was performed at Academia Sinica in Taiwan using a customized Axiom Genome-Wide Array Plate (Affymetrix, Santa Clara, CA, USA), and SNP information was obtained after imputation methods (68). All SNPs with minor allele frequency (MAF) <1% were excluded.
Chronic alcohol consumption was defined as drinking more than 150 ml of alcohol-containing beverage(s) per week for ≥6 months prior to enrollment in the TWB. Body mass index (BMI) was calculated (kg/m2) and categorized as underweight (BMI < 18.5), normal weight (18.5 ≤ BMI < 24), overweight (24 ≤ BMI < 27), and obese (BMI ≥ 27). Current cigarette smokers were those who smoked continuously for ≥6 months, and former smokers were those who previously smoked but quit for ≥6 months. Participants who exercised were those reporting a regular habit of exercising ≥30 min each three times per week. Hypertension was classified by patients self-reporting a diagnosis to a physician.
We used multiple linear regression to evaluate the association of GPNMB rs199347 and alcohol drinking with GPNMB cg17274742 methylation, as well as the interaction between GPNMB rs199347 and alcohol drinking. The differences between variables were calculated using a t-test for continuous variables and a chi-square test for categorical variables. Statistical significance was defined as α < 0.05. All analyses were conducted using PLINK version 1.9 beta (69) and SAS version 9.4 (SAS Institute Inc, Cary, NC, USA).
Overall, 1,357 participants comprising 648 men and 709 women were included in the study (Table 1). The mean GPNMB cg17274742 methylation levels (beta values) for individuals with chronic alcohol consumption was 0.9484 ± 0.0009 (mean ± standard error), compared to 0.9484 ± 0.0003 for those who did not chronically consume alcohol. For the non-chronic group, the number (percentage) of participants with the GPNMB rs199347 AA, AG, and GG genotypes was 633 (51.72%), 511 (41.75%), 80 (6.54%), respectively. For the chronic alcohol-drinking group, the number (percentage) with the GPNMB rs199347 AA, AG, and GG genotypes were 66 (49.62%), 53 (39.85%), and 14 (10.53%), respectively. The methylation levels and genotype distribution did not differ significantly between the chronic alcohol consumers and the other study participants.
The GPNMB rs199347 variant and alcohol consumption was not significantly associated with the methylation of cg17274742 (Table 2). However, men had significantly lower levels of cg17274742 methylation compared to women (β = −0.00302, p = 0.0169), while hypertensive patients had significantly higher levels of cg17274742 methylation compared to non-hypertensive patients (β = 0.00208, p = 0.0171).
GPNMB rs199347 variant and alcohol drinking had a significant interaction (p = 0.0102) with the methylation of cg17274742 (Table 3). After stratifying by alcohol consumption, rs199347 was not significantly associated with the methylation of cg17274742 in the control group. However, compared to the rs199347 AA genotype, the GG genotype was significantly associated with lower levels of methylation at cg17274742 (β = −0.00635, p = 0.0366).
After combining the rs199347 genotypes and alcohol consumption (Table 4), cg17274742 was significantly hypomethylated among chronic alcohol drinkers with the rs199347 GG genotype compared to the control group with the AA genotype (β= −0.00654, p = 0.0187).
Using data from a large, national prospective cohort study, we identified an association between cg17274742 methylation status, the GPNMB rs199347 polymorphism, and alcohol consumption. Our findings suggest that carriers of the rs199347 GG genotype and those who chronically consume alcohol have significantly more cg17274742 hypomethylation, which may result in increased GPNMB expression. This is the first study to find a relationship between a specific GPNMB polymorphism and alcohol-associated methylation changes suggesting that a lifestyle change, particularly a reduction in alcohol consumption for carriers of the rs199347 GG genotype, could reduce GPNMB expression, which is highly correlated with the risk of PD.
Recent studies highlight the importance and benefits of involving diverse and multiethnic populations in genetic studies (70, 71), including more accurately representing the risks of genetically associated diseases in different populations (72). Most published GWAS and methylomic studies involving the GPNMB rs199347 variant were conducted in majority-white study populations (36–39, 73, 74). Our study uses information obtained from the Taiwan Biobank (TWB), the largest biobank in East Asia with dense SNP array data, and leverages its high-coverage whole-genome sequencing and DNA methylation data in a population of Han Chinese (58). Notably, the rs199347 genotype frequencies observed in our study (A = 0.72, G = 0.28) are roughly equivalent to those reported by the Allele Frequency Aggregator Project in East Asia (A = 0.716, G = 0.283), which is different from the frequencies reported in white individuals (A = 0.59, G = 0.41) (31). While the TWB provides a robust dataset for understanding genetic associations in the Han Chinese population, it is crucial to discuss the generalizability of our findings beyond this group. The genetic and lifestyle diversity across different populations may impact the observed associations. Therefore, further studies are needed to explore how genetic differences between populations might influence the relationship between the GPNMB rs199347 variant, alcohol consumption, and Parkinson’s disease risk. Addressing these potential impacts would enhance the broader applicability of our findings and contribute to a more comprehensive understanding of genetic risk factors across diverse populations.
A novel integrative approach has been developed to align expression quantitative trait loci (eQTL) with genome-wide association study (GWAS) signals in Parkinson’s disease (PD), utilizing an updated PD GWAS dataset. This strategy highlighted the methylation site cg17274742 within the GPNMB gene, a site of interest for Coloc analysis, which investigates common causal variants shared between eQTL and GWAS data. Methylation at this specific locus not only affects gene expression but also influences splicing activities at the GPNMB/NUPL2 locus facilitated by robust protein–protein interactions. These interactions may connect to genes associated with either Mendelian or sporadic forms of PD (25). The presence of PD-associated variants at this methylation site reveals critical molecular pathways influencing the pathogenesis of PD, thereby emphasizing the significant role of both genetic and epigenetic factors in its development. In our study, we found that males had more cg17274742 hypomethylation, which may increase GPNMB expression. After correcting for age, the prevalence of PD in men is approximately 1.4 times higher than that in women (75), although a 2014 meta-analysis suggests that this difference is evident only in the 50- to 59-year age group (59). Additionally, we found that hypertension was associated with cg17274742 hypermethylation, which could decrease GPNMB expression and potentially reduce PD risk (24, 36). Published evidence connecting hypertension and PD diagnosis is contradictory (76–81), and different effects of hypertension have been observed in white versus Asian populations (76–79). Moreover, some antihypertensive medications may contribute to PD risk; inhibitors of the renin–angiotensin–aldosterone system may delay proinflammatory effects, and alpha-1-adrenergic receptor antagonists can enhance glycolysis and could reduce PD risk (82–87).
Chronic alcohol consumption can damage the central nervous system (45), and a recent translational study suggests a strong correlation between alcohol use disorder and the inflammatory response in the brain (45). Chronic alcohol consumption can trigger pro-inflammatory cytokines by activating peripheral macrophages and microglia in the central nervous system, which may alter the neuroinflammatory state in the brain (46, 47). Previous studies have noted the importance of different biological processes and functions of GPNMB, including cell differentiation and development, inflammation and immune response, progression, and neurodegeneration deterioration (23). Additionally, the Genotype-Tissue Expression (GTEx) project has identified that the GPNMB rs199347 variant significantly influences gene expression in various brain tissues, including the basal ganglia, cerebellum, and frontal cortex (88, 89). These regions are closely linked to neural mechanisms, particularly the dopaminergic pathways, which are crucial in the pathophysiology of PD (3, 6). The impact of this variant on these brain regions underscores its potential role in the clinical manifestations of PD. Our finding suggests that lifestyle changes, particularly a reduction in alcohol consumption for carriers of the rs199347 GG genotype, could modulate GPNMB expression, thereby identifying a potentially modifiable risk factor in Parkinson’s disease.
Our study was limited by the uncertainty around the amount of alcohol consumption. The TWB survey quantifies alcohol consumption in milliliters (ml), not milligrams (mg), and is therefore difficult to standardize across the study population. Additionally, data on alcohol consumption were self-reported and are subject to recall bias. Another limitation of our investigation concerns our inability to comprehensively account for all potential confounding variables, including dietary habits, lifestyle choices, and environmental exposures. Nevertheless, we mitigated this by controlling for a range of well-documented risk and protective factors, such as age, body mass index (BMI), smoking status, alcohol consumption, coffee intake, serum uric acid levels, and hypertension, all of which have been extensively studied in PD epidemiological research.
Ultimately, our results provide insight into the genetic and lifestyle factors associated with GPNMB expression, which is a potential biomarker and therapeutic target of PD. Future experiments using animal models or human cell lines to examine the underlying mechanisms behind this potential neuroinflammatory association in the central nervous system are warranted.
In summary, we found that having the GG genotype of GPNMB rs199347 with drinking habits decreases GPNMB methylation among Taiwan Biobank participants. These results provide information on the genetic and lifestyle factors that contribute to the expression, which is a PD potential biomarker and therapeutic target of PD, and could be used as a reference for experimental, longitudinal, or intervention studies evaluating the disease and its associated variables and mechanisms.
The access to and use of the Taiwan Biobank data in the present work was approved by the Ethics and Governance Council (EGC) of Taiwan Biobank (approval number: TWBR10907-05) and the Institutional Review Board (IRB) of National Health Research Institutes, Taiwan (approval number: EC1090402-E). The data collection of Taiwan Biobank was approved by the Ethics and Governance Council (EGC) of Taiwan Biobank and the Department of Health and Welfare, Taiwan (Wei-Shu-I-Tzu NO.1010267471). The data utilized in this study are sourced from the Taiwan Biobank, accessible at (https://www.twbiobank.org.tw/). Due to restrictions, the data are not publicly available and were used under license specifically for this study. However, these data can be obtained from the corresponding author, Yung-Po Liaw, upon reasonable request and with permission from the Taiwan Biobank.
Our study involves patients enrolled in the Taiwan Biobank (TWB). TWB was approved by the Institutional Review Board on Biomedical Science Research/IRB-BM, Academia Sinica and by the Ethics and Governance Council of Taiwan Biobank, Taiwan. Written informed consent was obtained before data collection from each participant in accordance with institutional requirements and the principles of the Declaration of Helsinki. This study was approved by the Institutional Review Board of the Chung Shan Medical University Hospital (CS1–20009). Participants in the Taiwan Biobank provided their written informed consent during enrollment. All methods were performed according to the relevant guidelines and regulations.
Y-CC: Conceptualization, Investigation, Validation, Visualization, Writing – original draft. Y-CL: Methodology, Validation, Writing – review & editing. ON: Investigation, Methodology, Supervision, Validation, Writing – review & editing. C-HH: Data curation, Formal analysis, Methodology, Writing – review & editing. J-HZ: Data curation, Formal analysis, Methodology, Writing – review & editing. S-LW: Conceptualization, Investigation, Validation, Writing – review & editing. Y-PL: Conceptualization, Funding acquisition, Investigation, Resources, Validation, Writing – review & editing.
The author(s) declare financial support was received for the research, authorship, and/or publication of this article. This work was funded by the Ministry of Science and Technology (MOST 109–2121-M-040–002; 109–2811-M-040–500; 110–2811-M-040–001; 110- 2121-M-040–002; 111–2811-M-040–001; 111–2121-M-040–002; 111–2811-M- 040–001).
English language and grammar review was provided by David K. Edwards V, PhD, CMPP (Madison, WI, USA).
The authors declare that the research was conducted in the absence of any commercial or financial relationships that could be construed as a potential conflict of interest.
All claims expressed in this article are solely those of the authors and do not necessarily represent those of their affiliated organizations, or those of the publisher, the editors and the reviewers. Any product that may be evaluated in this article, or claim that may be made by its manufacturer, is not guaranteed or endorsed by the publisher.
1. Bergman H, Deuschl G. Pathophysiology of Parkinson's disease: from clinical neurology to basic neuroscience and back. Mov Disord. (2002) 17 Suppl 3:S28–40. doi: 10.1002/(ISSN)1531-8257
2. Postuma RB, Berg D, Stern M, Poewe W, Olanow CW, Oertel W, et al. MDS clinical diagnostic criteria for Parkinson's disease. Mov Disord. (2015) 30:1591–601. doi: 10.1002/mds.26424
3. Balestrino R, Schapira AHV. Parkinson disease. Eur J Neurol. (2020) 27:27–42. doi: 10.1111/ene.14108
4. Alam M, Schmidt WJ. Rotenone destroys dopaminergic neurons and induces parkinsonian symptoms in rats. Behav Brain Res. (2002) 136:317–24. doi: 10.1016/S0166-4328(02)00180-8
5. Battaglia S, Nazzi C, Thayer JF. Genetic differences associated with dopamine and serotonin release mediate fear-induced bradycardia in the human brain. Trans Psychiatry. (2024) 14:24. doi: 10.1038/s41398-024-02737-x
6. Jankovic J, Tan EK. Parkinson's disease: etiopathogenesis and treatment. J Neurol Neurosurg Psychiatry. (2020) 91:795–808. doi: 10.1136/jnnp-2019-322338
7. Surguchov A, Surguchev A. Synucleins: new data on misfolding, aggregation and role in diseases. Biomedicines. (2022) 10:3241. doi: 10.3390/biomedicines10123241
8. Tanaka M, Szabó Á, Vécsei L, Giménez-Llort L. Emerging translational research in neurological and psychiatric diseases: from in vitro to in vivo models. Int J Mol Sci. (2023) 24:15739. doi: 10.3390/ijms242115739
9. Jirtle RL, Skinner MK. Environmental epigenomics and disease susceptibility. Nat Rev Genet. (2007) 8:253–62. doi: 10.1038/nrg2045
10. Tanaka M, Szabó Á, Vécsei L. Preclinical modeling in depression and anxiety: Current challenges and future research directions. Adv Clin Exp Med. (2023) 32:505–9. doi: 10.17219/acem/165944
11. Scheiblich H, Bousset L, Schwartz S, Griep A, Latz E, Melki R, et al. Microglial NLRP3 inflammasome activation upon TLR2 and TLR5 ligation by distinct α-synuclein assemblies. J Immunol. (2021) 207:2143–54. doi: 10.4049/jimmunol.2100035
12. Tremblay M-E, Cookson MR, Civiero L. Glial phagocytic clearance in Parkinson’s disease. Mol Neurodegen. (2019) 14:16. doi: 10.1186/s13024-019-0314-8
13. Grozdanov V, Bousset L, Hoffmeister M, Bliederhaeuser C, Meier C, Madiona K, et al. Increased immune activation by pathologic α-synuclein in Parkinson's disease. Ann Neurol. (2019) 86:593–606. doi: 10.1002/ana.25557
14. Tronel C, Largeau B, Santiago Ribeiro MJ, Guilloteau D, Dupont AC, Arlicot N. Molecular targets for PET imaging of activated microglia: the current situation and future expectations. Int J Mol Sci. (2017) 18. doi: 10.3390/ijms18040802
15. Joers V, Tansey MG, Mulas G, Carta AR. Microglial phenotypes in Parkinson's disease and animal models of the disease. Prog Neurobiol. (2017) 155:57–75. doi: 10.1016/j.pneurobio.2016.04.006
16. Grozdanov V, Bliederhaeuser C, Ruf WP, Roth V, Fundel-Clemens K, Zondler L, et al. Inflammatory dysregulation of blood monocytes in Parkinson's disease patients. Acta Neuropathol. (2014) 128:651–63. doi: 10.1007/s00401-014-1345-4
17. Sanchez-Guajardo V, Barnum CJ, Tansey MG, Romero-Ramos M. Neuroimmunological processes in Parkinson's disease and their relation to α-synuclein: microglia as the referee between neuronal processes and peripheral immunity. ASN Neuro. (2013) 5:113–39. doi: 10.1042/AN20120066
18. Brochard V, Combadière B, Prigent A, Laouar Y, Perrin A, Beray-Berthat V, et al. Infiltration of CD4+ lymphocytes into the brain contributes to neurodegeneration in a mouse model of Parkinson disease. J Clin Invest. (2009) 119:182–92. doi: 10.1172/JCI36470
19. Gerhard A, Pavese N, Hotton G, Turkheimer F, Es M, Hammers A, et al. In vivo imaging of microglial activation with [11C](R)-PK11195 PET in idiopathic Parkinson's disease. Neurobiol Dis. (2006) 21:404–12. doi: 10.1016/j.nbd.2005.08.002
20. Fiszer U, Mix E, Fredrikson S, Kostulas V, Link H. Parkinson's disease and immunological abnormalities: increase of HLA-DR expression on monocytes in cerebrospinal fluid and of CD45RO+ T cells in peripheral blood. Acta Neurol Scand. (1994) 90:160–6. doi: 10.1111/(ISSN)1600-0404
21. Smajić S, Prada-Medina CA, Landoulsi Z, Ghelfi J, Delcambre S, Dietrich C, et al. Single-cell sequencing of human midbrain reveals glial activation and a Parkinson-specific neuronal state. Brain. (2022) 145:964–78. doi: 10.1093/brain/awab446
22. Tansey MG, Wallings RL, Houser MC, Herrick MK, Keating CE, Joers V. Inflammation and immune dysfunction in Parkinson disease. Nat Rev Immunol. (2022) 22:657–73. doi: 10.1038/s41577-022-00684-6
23. Saade M, Araujo de Souza G, Scavone C, Kinoshita PF. The role of GPNMB in inflammation. Front Immunol. (2021) 12:674739. doi: 10.3389/fimmu.2021.674739
24. Diaz-Ortiz ME, Seo Y, Posavi M, Cordon MC, Clark E, Jain N, et al. GPNMB confers risk for Parkinson's disease through interaction with alpha-synuclein. Science. (2022) 377:eabk0637. doi: 10.1126/science.abk0637
25. Kia DA, Zhang D, Guelfi S, Manzoni C, Hubbard L, Reynolds RH, et al. Identification of candidate Parkinson disease genes by integrating genome-wide association study, expression, and epigenetic data sets. JAMA Neurol. (2021) 78:464. doi: 10.1001/jamaneurol.2020.5257
26. Zhou S, Tian Y, Song X, Xiong J, Cheng G. Brain proteome-wide and transcriptome-wide association studies, Bayesian colocalization and Mendelian randomization analyses revealed causal genes of Parkinson's disease. J Gerontol A Biol Sci Med Sci. (2022). doi: 10.1093/gerona/glac245
27. International Parkinson's Disease Genomics C, Wellcome Trust Case Control C. A two-stage meta-analysis identifies several new loci for Parkinson's disease. PloS Genet. (2011) 7:e1002142. doi: 10.1371/journal.pgen.1002142
28. Hüttenrauch M, Ogorek I, Klafki H, Otto M, Stadelmann C, Weggen S, et al. Glycoprotein NMB: a novel Alzheimer’s disease associated marker expressed in a subset of activated microglia. Acta Neuropathol Commun. (2018) 6. doi: 10.1186/s40478-018-0612-3
29. Krasemann S, Madore C, Cialic R, Baufeld C, Calcagno N, Fatimy RE, et al. The TREM2-APOE pathway drives the transcriptional phenotype of dysfunctional microglia in neurodegenerative diseases. Immunity. (2017) 47:566–581.e9. doi: 10.1016/j.immuni.2017.08.008
30. Török N, Maszlag-Török R, Molnár K, Szolnoki Z, Somogyvári F, Boda K, et al. Single nucleotide polymorphisms of indoleamine 2,3-dioxygenase 1 influenced the age onset of Parkinson's disease. FBL. (2022) 27. doi: 10.31083/j.fbl2709265
31. Sayers EW, Bolton EE, Brister JR, Canese K, Chan J, Comeau DC, et al. Database resources of the national center for biotechnology information. Nucleic Acids Res. (2022) 50:D20–d26. doi: 10.1093/nar/gkab1112
32. Moloney EB, Moskites A, Ferrari EJ, Isacson O, Hallett PJ. The glycoprotein GPNMB is selectively elevated in the substantia nigra of Parkinson's disease patients and increases after lysosomal stress. Neurobiol Dis. (2018) 120:1–11. doi: 10.1016/j.nbd.2018.08.013
33. Kaiser S, Zhang L, Mollenhauer B, Jacob J, Longerich S, Del-Aguila J, et al. A proteogenomic view of Parkinson’s disease causality and heterogeneity. NPJ Parkinson's Dis. (2023) 9:24. doi: 10.1038/s41531-023-00461-9
34. Budge KM, Neal ML, Richardson JR, Safadi FF. Transgenic overexpression of GPNMB protects against MPTP-induced neurodegeneration. Mol Neurobiol. (2020) 57:2920–33. doi: 10.1007/s12035-020-01921-6
35. Neal ML, Boyle AM, Budge KM, Safadi FF, Richardson JR. The glycoprotein GPNMB attenuates astrocyte inflammatory responses through the CD44 receptor. J Neuroinflamm. (2018) 15:73. doi: 10.1186/s12974-018-1100-1
36. Murthy MN, Blauwendraat C, Guelfi S, Hardy J, Lewis PA, Trabzuni D. Increased brain expression of GPNMB is associated with genome wide significant risk for Parkinson's disease on chromosome 7p15.3. Neurogenetics. (2017) 18:121–33. doi: 10.1007/s10048-017-0514-8
37. Iwaki H, Blauwendraat C, Leonard HL, Liu G, Maple-Grødem J, Corvol J-C, et al. Genetic risk of Parkinson disease and progression:: An analysis of 13 longitudinal cohorts. Neurol Genet. (2019) 5:e348. doi: 10.1212/NXG.0000000000000348
38. Chang D, Nalls MA, Hallgrímsdóttir IB, Hunkapiller J, van der Brug M, Cai F, et al. A meta-analysis of genome-wide association studies identifies 17 new Parkinson's disease risk loci. Nat Genet. (2017) 49:1511–6. doi: 10.1038/ng.3955
39. Nalls MA, Pankratz N, Lill CM, Do CB, Hernandez DG, Saa M, et al. Large-scale meta-analysis of genome-wide association data identifies six new risk loci for Parkinson's disease. Nat Genet. (2014) 46:989–93. doi: 10.1038/ng.3043
40. Wilson LE, Xu Z, Harlid S, White AJ, Troester MA, Sandler DP, et al. Alcohol and DNA methylation: an epigenome-wide association study in blood and normal breast tissue. Am J Epidemiol. (2019) 188:1055–65. doi: 10.1093/aje/kwz032
41. Liu C, Marioni RE, Hedman ÅK, Pfeiffer L, Tsai P-C, Reynolds LM, et al. A DNA methylation biomarker of alcohol consumption. Mol Psychiatry. (2018) 23:422–33. doi: 10.1038/mp.2016.192
43. Philibert RA, Plume JM, Gibbons FX, Brody GH, Beach SR. The impact of recent alcohol use on genome wide DNA methylation signatures. Front Genet. (2012) 3:54. doi: 10.3389/fgene.2012.00054
44. Manzardo AM, Henkhaus RS, Butler MG. Global DNA promoter methylation in frontal cortex of alcoholics and controls. Gene. (2012) 498:5–12. doi: 10.1016/j.gene.2012.01.096
45. Lanquetin A, Leclercq S, de Timary P, Segobin S, Naveau M, Coulbault L, et al. Role of inflammation in alcohol-related brain abnormalities: a translational study. Brain Commun. (2021) 3:fcab154. doi: 10.1093/braincomms/fcab154
46. Lowe PP, Morel C, Ambade A, Iracheta-Vellve A, Kwiatkowski E, Satishchandran A, et al. Chronic alcohol-induced neuroinflammation involves CCR2/5-dependent peripheral macrophage infiltration and microglia alterations. J Neuroinflamm. (2020) 17:296. doi: 10.1186/s12974-020-01972-5
47. Orio L, Alen F, Pavon FJ, Serrano A, Garcia-Bueno B. Oleoylethanolamide, neuroinflammation, and alcohol abuse. Front Mol Neurosci. (2018) 11:490. doi: 10.3389/fnmol.2018.00490
48. Szabo G, Lippai D. Chapter Eleven - Converging Actions of Alcohol on Liver and Brain Immune Signaling. In: Cui C, Shurtleff D, Harris RA, editors. International Review of Neurobiology. SAN DIEGO, CA: Academic Press (2014). p. 359–80.
49. Harris PS, Michel CR, Yun Y, McGinnis CD, Assiri MA, Ahmadi AR, et al. Proteomic analysis of alcohol-associated hepatitis reveals glycoprotein NMB (GPNMB) as a novel hepatic and serum biomarker. Alcohol. (2022) 99:35–48. doi: 10.1016/j.alcohol.2021.11.005
50. Hamada K, Ferguson LB, Mayfield RD, Krishnan HR, Maienschein-Cline M, Lasek AW. Binge-like ethanol drinking activates anaplastic lymphoma kinase signaling and increases the expression of STAT3 target genes in the mouse hippocampus and prefrontal cortex. Genes Brain Behav. (2021):e12729. doi: 10.1111/gbb.12729
51. Shao C, Wang X, Wang P, Tang H, He J, Wu N. Parkinson's disease risk and alcohol intake: A systematic review and dose-response meta-analysis of prospective studies. Front Nutr. (2021) 8:709846. doi: 10.3389/fnut.2021.709846
52. Peters S, Gallo V, Vineis P, Middleton LT, Forsgren L, Sacerdote C, et al. Alcohol consumption and risk of Parkinson's disease: data from a large prospective European cohort. Mov Disord. (2020) 35:1258–63. doi: 10.1002/mds.28039
53. Kim IY, Yang TO, Heath AK, Simpson RF, Reeves GK, Green J, et al. Alcohol intake and Parkinson's disease risk in the million women study. Mov Disord. (2020) 35:443–9. doi: 10.1002/mds.27933
54. Bettiol SS, Rose TC, Hughes CJ, Smith LA. Alcohol consumption and Parkinson's disease risk: A review of recent findings. J Parkinsons Dis. (2015) 5:425–42. doi: 10.3233/JPD-150533
55. Fukushima W, Miyake Y, Tanaka K, Sasaki S, Kiyohara C, Tsuboi Y, et al. Alcohol drinking and risk of Parkinson's disease: a case-control study in Japan. BMC Neurol. (2010) 10:111. doi: 10.1186/1471-2377-10-111
56. Eriksson AK, Löfving S, Callaghan RC, Allebeck P. Alcohol use disorders and risk of Parkinson's disease: findings from a Swedish national cohort study 1972-2008. BMC Neurol. (2013) 13:190. doi: 10.1186/1471-2377-13-190
57. Chen CH, Yang JH, Chiang CWK, Hsiung C-N, Wu P-E, Chang L-C, et al. Population structure of Han Chinese in the modern Taiwanese population based on 10,000 participants in the Taiwan Biobank project. Hum Mol Genet. (2016) 25:5321–31. doi: 10.1093/hmg/ddw346
58. Wei C-Y, Yang J-H, Yeh E-C, Tsai M-F, Kao H-J, Lo C-Z, et al. Genetic profiles of 103,106 individuals in the Taiwan Biobank provide insights into the health and history of Han Chinese. NPJ Genom Med. (2021) 6. doi: 10.1038/s41525-021-00178-9
59. Pringsheim T, Jette N, Frolkis A, Steeves TD. The prevalence of Parkinson's disease: a systematic review and meta-analysis. Mov Disord. (2014) 29:1583–90. doi: 10.1002/mds.25945
60. Noyce AJ, Bestwick JP, Silveira-Moriyama L, Hawkes CH, Giovannoni G, Lees AJ, et al. Meta-analysis of early nonmotor features and risk factors for Parkinson disease. Ann Neurol. (2012) 72:893–901. doi: 10.1002/ana.23687
61. Liu R, Guo X, Park Y, Huang X, Sinha R, Freedman ND, et al. Caffeine intake, smoking, and risk of Parkinson disease in men and women. Am J Epidemiol. (2012) 175:1200–7. doi: 10.1093/aje/kwr451
62. Chen H, Huang X, Guo X, Mailman RB, Park Y, Kamel F, et al. Smoking duration, intensity, and risk of Parkinson disease. Neurology. (2010) 74:878–84. doi: 10.1212/WNL.0b013e3181d55f38
63. Quik M. Smoking, nicotine and Parkinson's disease. Trends Neurosci. (2004) 27:561–8. doi: 10.1016/j.tins.2004.06.008
64. Du P, Zhang X, Huang CC, Jafari N, Kibbe WA, Hou L, et al. Comparison of Beta-value and M-value methods for quantifying methylation levels by microarray analysis. BMC Bioinf. (2010) 11:587. doi: 10.1186/1471-2105-11-587
65. Shen L, Kondo Y, Guo Y, Zhang J, Zhang L, Ahmed S, et al. Genome-wide profiling of DNA methylation reveals a class of normally methylated cpG island promoters. PloS Genet. (2007) 3:e181. doi: 10.1371/journal.pgen.0030181
66. Irizarry RA, Ladd-Acosta C, Carvalho B, Wu H, Brandenburg SA, Jeddeloh JA, et al. Comprehensive high-throughput arrays for relative methylation (CHARM). Genome Res. (2008) 18:780–90. doi: 10.1101/gr.7301508
67. Rahmani E, Zaitlen N, Baran Y, Eng C, Hu D, Galanter J, et al. Sparse PCA corrects for cell type heterogeneity in epigenome-wide association studies. Nat Methods. (2016) 13:443–5. doi: 10.1038/nmeth.3809
68. Shi S, Yuan N, Yang M, Du Z, Wang J, Sheng X, et al. Comprehensive assessment of genotype imputation performance. Hum Hered. (2018) 83:107–16. doi: 10.1159/000489758
69. Chang CC, Chow CC, Tellier LC, Vattikuti S, Purcell SM, Lee JJ. Second-generation PLINK: rising to the challenge of larger and richer datasets. Gigascience. (2015) 4:7. doi: 10.1186/s13742-015-0047-8
70. Graham SE, Clarke SL, Wu K-HH, Kanoni S, Zajac GJM, Ramdas S, et al. The power of genetic diversity in genome-wide association studies of lipids. Nature. (2021) 600:675–9. doi: 10.1038/s41586-021-04064-3
71. Popejoy AB, Fullerton SM. Genomics is failing on diversity. Nature. (2016) 538:161–4. doi: 10.1038/538161a
72. Sirugo G, Williams SM, Tishkoff SA. The missing diversity in human genetic studies. Cell. (2019) 177:26–31. doi: 10.1016/j.cell.2019.02.048
73. Prasad R, Jho EH. A concise review of human brain methylome during aging and neurodegenerative diseases. BMB Rep. (2019) 52:577–88. doi: 10.5483/BMBRep.2019.52.10.215
74. Langmyhr M, Henriksen SP, Cappelletti C, van de Berg WDJ, Pihlstrøm L, Toft M. Allele-specific expression of Parkinson's disease susceptibility genes in human brain. Sci Rep. (2021) 11:504. doi: 10.1038/s41598-020-79990-9
75. Collaborators GBDPsD. Global, regional, and national burden of Parkinson's disease, 1990-2016: a systematic analysis for the Global Burden of Disease Study 2016. Lancet Neurol. (2018) 17:939–53. doi: 10.1016/S1474-4422(18)30295-3
76. Ng Y-F, Ng E, Lim E-W, Prakash KM, Tan LCS, Tan E-K. Case-control study of hypertension and Parkinson’s disease. NPJ Parkinson's Dis. (2021) 7:63. doi: 10.1038/s41531-021-00202-w
77. Chen J, Zhang C, Wu Y, Zhang D. Association between hypertension and the risk of Parkinson’s disease: A meta-analysis of analytical studies. Neuroepidemiology. (2019) 52:181–92. doi: 10.1159/000496977
78. Hou L, Li Q, Jiang L, Qiu H, Geng C, Hong J-S, et al. Hypertension and diagnosis of Parkinson's disease: A meta-analysis of cohort studies. Front Neurol. (2018) 9:162. doi: 10.3389/fneur.2018.00162
79. Qiu C, Hu G, Kivipelto M, Laatikainen T, Antikainen R, Fratiglioni L, et al. Association of blood pressure and hypertension with the risk of Parkinson disease. Hypertension. (2011) 57:1094–100. doi: 10.1161/HYPERTENSIONAHA.111.171249
80. Paganini-Hill A. Risk factors for Parkinson's disease: the leisure world cohort study. Neuroepidemiology. (2001) 20:118–24. doi: 10.1159/000054770
81. McCann SJ, LeCouteur DG, Green AC, Brayne C, Johnson AG, Chan D, et al. The epidemiology of Parkinson's disease in an Australian population. Neuroepidemiology. (1998) 17:310–7. doi: 10.1159/000026185
82. Simmering JE, Welsh MJ, Schultz J, Narayanan NS. Use of glycolysis-enhancing drugs and risk of Parkinson's disease. Mov Disord. (2022) 37:2210–6. doi: 10.1002/mds.29184
83. Lin HC, Tseng YF, Shen AL, Chao JC, Hsu CY, Lin HL. Association of angiotensin receptor blockers with incident Parkinson disease in patients with hypertension: A retrospective cohort study. Am J Med. (2022) 135:1001–7. doi: 10.1016/j.amjmed.2022.04.029
84. Jo Y, Kim S, Ye BS, Lee E, Yu YM. Protective effect of renin-angiotensin system inhibitors on Parkinson's disease: A nationwide cohort study. Front Pharmacol. (2022) 13:837890. doi: 10.3389/fphar.2022.837890
85. Simmering JE, Welsh MJ, Liu L, Narayanan NS, Pottegård A. Association of glycolysis-enhancing α-1 blockers with risk of developing Parkinson disease. JAMA Neurol. (2021) 78:407–13. doi: 10.1001/jamaneurol.2020.5157
86. Cai R, Zhang Y, Simmering JE, Schultz JL, Li Y, Fernandez-Carasa I, et al. Enhancing glycolysis attenuates Parkinson’s disease progression in models and clinical databases. J Clin Invest. (2019) 129:4539–49. doi: 10.1172/JCI129987
87. Lee Y-C, Lin C-H, Wu R-M, Lin J-W, Chang C-H, Lai M-S. Antihypertensive agents and risk of Parkinson's disease: A nationwide cohort study. PloS One. (2014) 9:e98961. doi: 10.1371/journal.pone.0098961
88. Aguet F, Anand S, Ardlie KG, Gabriel S, Getz GA, Graubert A, et al. The GTEx Consortium atlas of genetic regulatory effects across human tissues. Science. (2020) 369:1318–30. doi: 10.1126/science.aaz1776
Keywords: Parkinson disease, DNA methylation, epigenesis, genetic, alcohol-related disorders, GPNMB
Citation: Chen Y-C, Liaw Y-C, Nfor ON, Hsiao C-H, Zhong J-H, Wu S-L and Liaw Y-P (2024) Epigenetic associations of GPNMB rs199347 variant with alcohol consumption in Parkinson’s disease. Front. Psychiatry 15:1377403. doi: 10.3389/fpsyt.2024.1377403
Received: 29 January 2024; Accepted: 27 June 2024;
Published: 18 July 2024.
Edited by:
Mason Tsai, National Chung Hsing University, TaiwanReviewed by:
Masaru Tanaka, University of Szeged (ELKH-SZTE), HungaryCopyright © 2024 Chen, Liaw, Nfor, Hsiao, Zhong, Wu and Liaw. This is an open-access article distributed under the terms of the Creative Commons Attribution License (CC BY). The use, distribution or reproduction in other forums is permitted, provided the original author(s) and the copyright owner(s) are credited and that the original publication in this journal is cited, in accordance with accepted academic practice. No use, distribution or reproduction is permitted which does not comply with these terms.
*Correspondence: Shey-Lin Wu, d3VzaGV5bGluQGdtYWlsLmNvbQ==; Yung-Po Liaw, bGlhd3lwQGNzbXUuZWR1LnR3
Disclaimer: All claims expressed in this article are solely those of the authors and do not necessarily represent those of their affiliated organizations, or those of the publisher, the editors and the reviewers. Any product that may be evaluated in this article or claim that may be made by its manufacturer is not guaranteed or endorsed by the publisher.
Research integrity at Frontiers
Learn more about the work of our research integrity team to safeguard the quality of each article we publish.