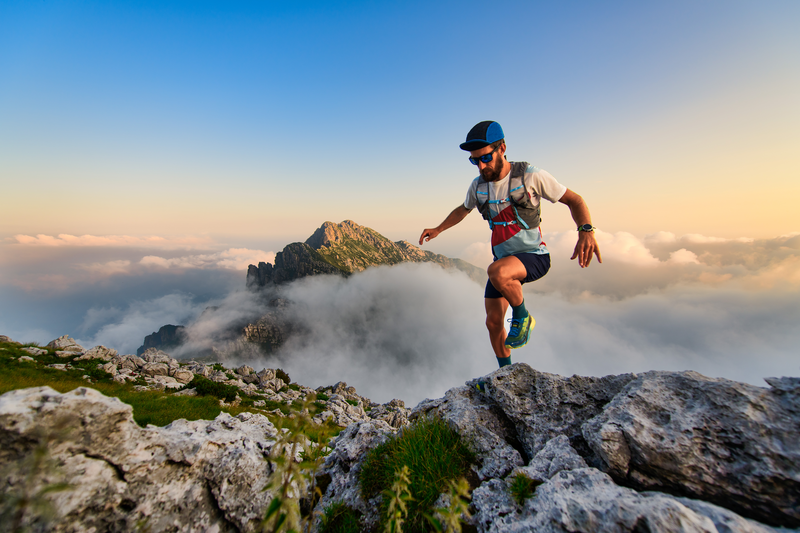
94% of researchers rate our articles as excellent or good
Learn more about the work of our research integrity team to safeguard the quality of each article we publish.
Find out more
ORIGINAL RESEARCH article
Front. Psychiatry , 04 March 2024
Sec. Mood Disorders
Volume 15 - 2024 | https://doi.org/10.3389/fpsyt.2024.1364786
Objectives: Major Depressive Disorder (MDD) is significantly influenced by childhood trauma (CT), affecting brain anatomy and functionality. Despite the unique disease trajectory in MDD patients with CT, the underlying neurobiological mechanisms remain unclear. Our objective is to investigate CT’s impact on the white matter structure of the brain in patients with MDD.
Methods: This research employed tract-based spatial statistics (TBSS) to detect variations between groups in Fractional Anisotropy (FA) throughout the whole brain in 71 medication-free MDD patients and 97 HCs. Participants filled out the Childhood Trauma Questionnaire (CTQ) and assessments for depression and anxiety symptoms. The relationship between FA and CTQ scores was explored with partial correlation analysis, adjusting for factors such as age, gender, educational background, and length of illness.
Results: Compared to HCs, the MDD group showed decreased FA values in the right posterior limb of the internal capsule (PLIC), the inferior fronto-occipital fasciculus (IFOF), and bilateral superior longitudinal fasciculus (SLF). Simple effects analysis revealed that compared to HC-CT, the MDD-CT group demonstrated decreased FA values in right PLIC, IFOF, and bilateral SLF. The MDD-nCT group showed decreased FA values in right PLIC and IFOF compared to HC-nCT. The total scores and subscale scores of CTQ were negatively correlated with the FA in the right SLF.
Conclusion: The right SLF may potentially be influenced by CT during the brain development of individuals with MDD. These results enhance our knowledge of the role of the SLF in the pathophysiology of MDD and the neurobiological mechanisms by which CT influences MDD.
Major Depressive Disorder (MDD) is a mental illness linked to severe consequences, including impaired social and occupational functioning, substance dependence, and suicide. Its core symptoms are persistent depressive mood and loss of interest (1). Globally, MDD impacts around 16% of individuals and stands as a primary contributor to disease burden (2, 3). The neurobiological mechanisms of MDD are not fully elucidated, and exploring these mechanisms and finding effective treatments are crucial.
It is well-known that the onset of MDD has many potential factors, with childhood trauma (CT) being the known risk factor (4). Increasing evidence suggests that people with a past of CT are more susceptible to psychiatric illnesses, including MDD (5), bipolar disorder, and post-traumatic stress disorder (6). Studies indicate that 46% of individuals diagnosed with MDD have experienced CT (7). Moreover, CT encompasses various forms such as emotional abuse, physical abuse, emotional neglect, and physical neglect; various types of CT may further elevate the risk of adverse outcomes later in life, like how physical abuse might affect an adult’s responsiveness to drug treatment (8). The impact of CT on MDD has been validated, and clinical research has shown that MDD patients with a background of CT exhibit higher suicide risks, increased severity of depressive symptoms, and functional deficits (9). Plentiful research indicates that CT is connected with immune, endocrine, and epigenetic activities, along with alterations in brain anatomy and functionality (10, 11). Despite the unique disease trajectory of MDD patients with CT, its neurobiological mechanisms remain unclear.
With the advancement of neuroimaging technologies, MRI studies suggest that healthy individuals with a history of CT show widespread cortical and subcortical morphological changes. Studies involving gray matter regions have found an association between CT experience and lower volumes of the hippocampus and amygdala (12–14). Beyond gray matter regions, several studies have reported specific white matter(WM) changes in individuals with an experience of CT, including the inferior longitudinal fasciculus, uncinate fasciculus, thalamic radiation, corona radiata, longitudinal fasciculi, cingulum, and corpus callosum (15–17). Notably, there is an anatomical overlap between the specific WM change areas in MDD patients and those mentioned above. This overlap might validate the close connection between CT and MDD, though it might also suggest a need to discern whether these changes are attributable to CT or MDD. Therefore, studying the impact of CT on MDD patients is necessary.
Many studies have proven that MDD patients with an experience of CT exhibit specific functional changes in the brain (18–21). However, only a few studies have explored the morphological changes in the brains of MDD patients with CT. These structural MRI study results are inconsistent (22, 23), with some finding changes and others not, possibly due to heterogeneity in study subjects (such as age, medication, duration of illness) and methodological differences in previous research. The effect of antidepressants on WM is unclear, as most past studies included medicated patients, making it difficult to determine whether these results are confounded by medication effects. Moreover, chronic or extended-duration illnesses may result in more pronounced neuroimaging alterations, and drug-naive patients with a shorter illness duration might help us better understand the early onset of the disease. Additionally, many studies merely demonstrate the impact of CT on the WM structure of the brain in MDD patients through correlation analysis (24, 25). Our article will refine the grouping based on CT and, on this basis, further investigate the effects of CT on participants and its interaction with the MDD diagnosis.
Diffusion Tensor Imaging (DTI) is among the most commonly employed techniques to assess the integrity of MDD brain WM, helping us better explore the disease’s underlying biological mechanisms and improve consensus on diagnosis and treatment (26). Fractional anisotropy (FA) is particularly responsive to alterations in microstructure and is often used to examine WM structural connectivity in MDD (27). Impaired WM integrity might be one of the most important pathogenic mechanisms in depression with CT, and it’s crucial to explore which fiber tracts are affected and whether WM structural changes mediate the relationship between CT and emotional/cognitive functional impairment. Among the primary analysis methods in DTI, region of interest (ROI) analysis and voxel-based morphometry present clear drawbacks: ROI analysis might restrict results to ‘regions of interest’, potentially overlooking other significant brain regions; voxel-based morphometry faces issues like registration, smoothing, and other MRI data processing problems (28). Tract-Based Spatial Statistics (TBSS) can comprehensively explore all brain regions associated with changes related to CT and is more sensitive, objective, and interpretable in DTI data analysis.
In this study, the TBSS method will be used to explore how CT and MDD affect WM structural changes and whether there is an interaction between them in medication-free MDD individuals versus comparable HCs. Additionally, we examined whether CT affects mood-related aspects by mediating changes in WM. Participants’ levels of depression and anxiety were assessed using depression and anxiety scales. Based on this, we further investigated the correlation between WM structure and the severity of depression, anxiety symptoms, and the extent of CT. We hypothesize that CT may have long-term and sustained impacts, associated with structural changes in the brains of adult patients with MDD.
This research encompassed 71 drug-naive MDD patients and 97 healthy controls (HCs), matched for gender, age, and educational level. MDD patients were sourced from the outpatient psychiatry department of Renmin Hospital of Wuhan University between April 2021 and September 2023. HCs with no history of psychiatric disorders were recruited from the community through advertisements. Diagnoses of MDD were made by psychiatrists through the Structured Clinical Interview for DSM-V (SCID). Entry requirements for the MDD group included: (1) fulfilling DSM-V standards for a major depressive episode; (2) first-episode, treatment-naive for psychotropic drugs or psychotherapy; (3) aged between 18-55 years; (4) Han Chinese ethnic;(5) right-handed; (6) ≥9 years of education. HCs fulfilled these conditions: (1) absence of significant psychiatric conditions or a family history of major mental disorders; (2) aged 18-55 years; (3) right-handed. Exclusion criteria were: (1) a record of other DSM-V Axis I psychiatric issues; (2) past or present organic brain disease, brain trauma, or cranial surgery; (3) history of substance abuse or dependence; (4) contraindications for MRI scanning; (5) pregnant or lactating women. On the day of scanning, all participants’ depression and anxiety levels were evaluated using the 24-item Hamilton Depression Rating Scale (HAMD-24) and the Hamilton Anxiety Rating Scale (HAMA).
Demographic and clinical characteristics of all participants were collected. The Childhood Trauma Questionnaire (CTQ) in its Chinese variant was utilized to evaluate participants’ childhood experiences (29). This questionnaire comprises 28 items assessing CT across five subscales: Emotional Neglect (EN), Physical Neglect (PN), Sexual Abuse (SA), Emotional Abuse (EA), and Physical Abuse (PA). Thresholds for moderate to severe trauma on each subscale were: PN≥10, PA≥10, SA≥8, EN≥15, EA≥13. In our research, participants with scores exceeding any of the thresholds were considered to have been exposed to moderate or severe CT, while those scoring below were considered to have none or mild CT. Participants were divided into four groups: MDD patients with moderate or severe CT (MDD-CT), MDD patients with none or mild CT(MDD-nCT), HC with moderate/severe CT(HC-CT), and HC with none or mild CT(HC-nCT). All recruited participants gave informed consent for this clinical research, which received approval from the Ethics Committee of Renmin Hospital of Wuhan University.
MRI data for all participants were collected using a 3.0T GE Signa HDx MRI scanner at Renmin Hospital of Wuhan University. Participants were instructed to lie quietly in a supine position on the scanner, with head movements minimized by padding. T1-weighted structural images were captured using the subsequent parameters: repetition time; 8.5 ms; flip angle, 12° echo time, 3.2 ms; slice thickness, 1.0 mm; gap, 0.0 mm; field of view, 256 mm × 256 mm; matrix, 256 × 256; voxel size, 1.0 mm × 1.0 mm × 1.0 mm; 176 slices. Diffusion tensor images were acquired by employing spin echoplanar imaging (EPI) sequences with these parameters: repetition time, 2000 ms; flip angle, 90°; echo time, 30 ms; voxel size, 3.4 mm × 3.4 mm × 4.0 mm; slice thickness, 4.0 mm; slice gap, 0 mm; FOV = 220 mm × 220 mm; matrix size, 64 × 64; 36 slices.
TBSS is a method for exploratory analysis that relies on image registration and analysis of the entire brain at the voxel level. All DTI images were processed using functional MR imaging of the brain (FMRIB) Software Library (FSL) (http://www.fmrib.ox.au.uk/fsl).
The preprocessing procedure for TBSS in neuroimaging involves several key steps to prepare diffusion data for analysis. Initially, high-quality DTI data is acquired, which is then corrected for motion and eddy current distortions. Following this, brain extraction tools, like FSL’s BET, are used to remove non-brain structures from the DTI images. Subsequently, diffusion measures such as FA are computed.
After generating FA images for each subject, they were aligned to the FMRIB58_FA template and transformed into the MNI standard space through affine registration. This process produced standard space versions of individual FA images, which were then averaged to form a mean FA image. A threshold of 0.2 for FA was set to omit voxels with low FA values. This resulted in a mean FA skeleton representing the center of all WM tracts. Finally, all individual standard space FA images were mapped onto the mean FA skeleton. Then we create a 4D file composed of all individual FA skeletons. Fslmaths, a utility command line from FSL, was frequently utilized to delineate exact regions of interest where notable differences between patient and HCs were present.
Demographic data of MDD patients and HCs, including age, gender, age, gender, education, illness duration and family history, depression level, anxiety level, CTQ scores, and imaging data, were compared. Categorical data were presented as proportions, and continuous variables as means and standard deviations. Shapiro-Wilkes test for normality and Levene’s test were used to assess the distribution and homogeneity of variances using SPSS 27.0. Gender family history differences were assessed using Chi-square tests. Demographic and behavioral differences in the two groups were assessed via independent sample t-tests or Mann-Whitney U tests (when the distributions were not Gaussian). One-way ANOVA tests with Bonferroni-test or Welch-ANOVA with Games-Howell post-tests (for unequal variances) were employed to compare these variables among the four groups.
For imaging data, two-way between-subjects ANOVA was employed to analyze data from the four groups, using FSL Randomize tool with 5000 permutations with the threshold-free cluster enhancement option (TFCE). Using this to examine the effects of diagnosis and CT, and to determine if there is an interaction between diagnosis and CT.
To examine whether clinical characteristics of MDD patients were related to their WM integrity, partial correlation analysis was conducted while adjusting for age, gender, education, and illness duration. The correlation between the average FA values and the patients’ levels of depression, anxiety, total CTQ scores, and subscale scores were assessed. Multiple comparisons were performed using a False discovery rate (FDR) < 0.05.
To enhance the robustness of our research findings, we conducted a sensitivity analysis. This involved reanalyzing the sample after excluding individuals with a family history of mental disorders.
Comprehensive demographic and clinical information are provided in Table 1. No statistically notable variations were observed in age, gender, or educational level across the groups. All intergroup comparisons were performed using Bonferroni correction for multiple comparisons. No statistically significant disparity in illness duration was observed between the MDD-CT and MDD-nCT groups.
A notable main effect of CT on anxiety levels was noted, suggesting that participants with CT exhibited more severe anxiety symptoms compared to those with none or mild CT. Additionally, the diagnosis of MDD demonstrated an important main effect on both depression and anxiety levels, with individuals in the MDD group exhibiting significantly higher levels of both compared to the healthy control group. No significant effects of CT on depression levels or CT × diagnosis interaction were observed (refer to Table 2).
Figure 1 and Table 3 illustrates regions where notable disparities in FA values were observed between the MDD and HCs. The brain areas showing decreased FA values in the MDD group included the right posterior limb of the internal capsule (PLIC), inferior fronto-occipital fasciculus (IFOF), and bilateral superior longitudinal fasciculus (SLF), totaling five clusters.
Figure 1 Group differences of TBSS analysis. Blue regions represent tracts with decreased FA in the MDD group compared with the HC group. (A-E) Represent five distinct cluster regions where significant differences between MDD and HCs were observed. MDD, major depressive disorders; HC, Healthy Controls; TBSS, tract-based spatial statistics; FA, fractional anisotropy; L, left hemisphere; R, right hemisphere; SLF, superior longitudinal fasciculus; PLIC, posterior limb of the internal capsule; IFOF, inferior fronto-occipital fasciculus.
Table 3 Areas of reduced white matter FA in MDD patients compared with HC (p < 0.05, TFCE-corrected).
As demonstrated in Table 4, a notable main effect of diagnosis on WM regions FA was noted. No significant effects of CT on WM regions FA or CT × diagnosis interaction were observed.
After simple effects analysis, as demonstrated in Figure 2 and Table 5, the MDD-CT group showed notably lower FA values in the right PLIC (p<0.001), IFOF (p<0.001), and bilateral SLF (p<0.001) compared to the HC-CT group. Compared to the HC-nCT group, the MDD-nCT group showed lower FA values in the right PLIC and IFOF (p<0.001). No WM regions with increased FA values were found in the MDD group compared to HCs. No significant brain region differences were found between the MDD-CT group and the MDD-nCT group.
Figure 2 (A–C) Represent three distinct cluster regions where significant differences between MDD-CT and HC-CT were observed following simple effects analysis. (D) Represents a distinct cluster region where significant differences between MDD-nCT and HC-nCT were observed following simple effects analysis. Blue regions represent tracts with decreased FA (MDD-CT vs HC-CT and MDD-nCT vs HC-nCT). MDD, major depressive disorders; HC, Healthy Controls; CT, childhood trauma; FA, fractional anisotropy; L, left hemisphere; R, right hemisphere; SLF, superior longitudinal fasciculus; PLIC, posterior limb of the internal capsule; IFOF, inferior fronto-occipital fasciculus.
Partial correlation analysis between FA values and CTQ subscale scores, as well as total scores, are presented in Figure 3 and Table 6. In the MDD group, Right SLF showed a negative correlation with EA (r=-0.397, p=0.017), PA (r=-0.370, p=0.017), PN (r=-0.356, p=0.018), and total score (r=-0.476, p=0.002). No significant correlations were found between the right PLIC, right IFOF, left SLF and CTQ subscale or total scores in the MDD group. There were also no notable correlations between the average FA values of each cluster and scores on the HAMD-24 and HAMA scales in the MDD group.
Figure 3 Scatter plots of partial correlation between FA regions and the target scales in MDD group. (A) Negative partial correlation between FA of right SLF and CTQ-EA score. (B) Negative partial correlation between FA of right SLF and CTQ-PA score. (C) Negative partial correlation between FA of right SLF and CTQ-PN score. (D) Negative partial correlation between FA of right SLF and CTQ-Total score. All correlations showed in this figure were constructed after controlling age, gender, years of education, and disease duration. MDD, major depressive disorder; FA, fractional anisotropy; CTQ, Childhood Trauma Questionnaire; CTQ-EA, emotional abuse subscale of childhood trauma questionnaire; CTQ-PA, physical abuse subscale of childhood trauma questionnaire; CTQ-PN, physical neglect subscale of childhood trauma questionnaire; CTQ-Total, Childhood Trauma Questionnaire total score; SLF, superior longitudinal fasciculus.
We excluded five individuals with a family history of mental disorders and employed the same analytical methods. The results of the sensitivity analysis remained robustly consistent with the original analysis findings (refer to Tables 7, 8).
Table 7 Sensitivity analysis: Diagnosis and childhood trauma effect on white matter FA. (p < 0.05, TFCE-corrected).
Table 8 Sensitivity analysis: areas of reduced white matter FA in MDD patients compared with HC (p < 0.05, TFCE-corrected).
This study aimed to identify neuroimaging evidence of structural brain changes in MDD patients who have experienced CT. We examined the effect of CT on MDD and the associations between CT, brain structural changes, and MDD. Our findings indicate a considerable main effect of diagnosis, with the MDD group exhibiting notable structural differences in the right IFOF, PLIC, and bilateral SLF compared to the HC group. CT did not show a significant main effect. Simple effects analysis revealed notable differences between the MDD-CT and HC-CT groups in the right IFOF, PLIC, and bilateral SLF, while the MDD-nCT and HC-nCT groups differed only in the right IFOF and PLIC. However, there were no interactive effects of CT × diagnosis. Moreover, a pronounced main effect of CT on anxiety symptoms was observed, with the CT group displaying heightened anxiety symptoms relative to the nCT group. Lastly, in the patient group, the right SLF was negatively correlated with EA, PA, PN, and total scores. This study is the first to comprehensively explore WM structural changes in drug-naive first-episode MDD patients who have experienced CT using the TBSS method.
Initially, our study found a reduction in FA in the right IFOF and PLIC in the MDD group compared to HCs. Our findings are supported by several MRI studies (30–32). Reduced FA is considered indicative of decreased WM organization, reduced axonal density, and myelination (33). Changes in these regions might be involved in the pathophysiology of depression. However, these differential changes were unrelated to the presence of CT. There were no significant differences in brain regions between the CT and nCT groups. This might indicate that the reduction in FA in the right IFOF and PLIC might be a specific change in the WM regions of MDD patients, independent of CT. These results differ from previous studies related to CT (15–17, 34, 35). However, Lim et al.’s study found that specific WM changes in the CT group were rectified in the subgroup analysis of unmedicated participants (34). Many studies have shown that medication can impact brain structure (30, 36). Therefore, the difference in our findings might be attributed to the inclusion of unmedicated participants. The IFOF, one of the longest association tracts in the human brain, links the occipital, frontal, and temporal lobes, and plays a role in emotional evaluation and visual perception regulation (37). The internal capsule, comprising multiple fiber tracts, connects the posterior limb with the parietal, temporal, occipital, and sensorimotor brain regions (38), and is associated with brain reward and self-stimulation processes (39). Structural changes in the IFOF and PLIC might be involved in the neurobiological mechanisms of MDD. We did not find a relationship between the IFOF, PLIC, and CT, which warrants further exploration in future research.
Secondly, we noted a marked decrease in FA in the bilateral SLF in the MDD group compared to HCs. Our study revealed structural changes in the SLF only between the MDD-CT and HC-CT groups, with no significant differences between MDD-nCT and HC-nCT groups. The SLF is an extensive fiber tract connecting almost all cortical areas of the lateral hemisphere, especially the frontal lobe with other cognitive or executive function brain regions. The SLF is associated with various cognitive and sensory functions, including language processing, attention control, working memory, executive function, visual and spatial processing (40, 41). Our findings in the SLF only validate prior research on MDD, suggesting that changes in SLF structure could be closely linked to the pathogenesis and progression of MDD. However, our study offers new insights regarding CT: the specific brain structural changes in MDD patients appear to be unrelated to CT, which differs from previous studies (15, 24, 42). We speculate that this might be due to related studies treating CT or MDD as isolated factors rather than examining them in conjunction. Additionally, clinical heterogeneity, including medication status, illness duration, and sample size, needs to be considered. These factors might collectively mediate the heterogeneity observed in our results. It is worth noting that CT and MDD are not entirely independent factors; CT, as a significant risk factor, has been proven to be involved in the development of MDD (43). Secondly, CT is an influencing factor in the pathogenesis of MDD but does not play a decisive role, which might partially explain the results we obtained after comparing the four groups. Finally, the significant differences between brain regions in MDD-CT and MDD-nCT might be due to differing levels of resilience among individuals with CT. Evidence suggests that CT can have varying impacts on an individual’s resilience (44), so those with experiences of CT might develop different outcomes (depression or non-depression) based on their resilience.
Although we did not identify significant brain structural changes related to CT experiences, our correlational analysis revealed a significant association between CT and FA values, as well as clinical symptoms. Our study found a notable main effect of CT on levels of anxiety. This suggests that CT might affect anxiety symptoms in both depressed and non-depressed individuals, with this impact being persistent and latent. Early life traumatic experiences might lead to higher levels of anxiety in adulthood. Our findings are supported by previous research indicating that CT experiences increase symptoms of depression, anxiety, and negative life event appraisal in adults (45). Furthermore, our exploration of the correlation between CT and WM fiber bundle FA values revealed negative correlations in the SLF with all trauma types except for the SA and EN. This suggests that CT may have a latent impact on the progression of MDD in patients. Adverse experiences in childhood, processed through individual sensory perceptions such as vision and hearing, could potentially affect the SLF, as it is involved in the processing of visual and auditory information. Consequently, we speculate that brain regions responsible for information processing may be susceptible to the effects of CT. This is supported by related research, which has found that CT predominantly affects several brain regions involved in cognitive and executive functions and information processing, such as areas for visual and auditory perception and language comprehension (46). Our findings of the correlation between CTQ scores and FA values in the SLF may indicate that CT subtly influences the developmental process of the SLF in the brains of MDD patients, thereby impairing cognitive and emotional functions and leading to more severe clinical symptoms.
Previous research has suggested that specific trauma types might have more severe impacts on individual brain structures: emotional neglect (35, 47), physical neglect (16, 35, 47), emotional abuse (8, 24), physical abuse (8, 13, 24), and sexual abuse (8, 13, 24). Our findings that EA, PA, PN and total scores predict a decrease in regional FA in the SLF suggests that the right SLF is susceptible to early adverse experiences. Future studies may concentrate on the association between different trauma types and the SLF. Moreover, the left SLF is not significantly correlated with CT scores. These differential results in the bilateral SLF suggest that we need to consider the effects of laterality. A recent study focusing on the SLF proposed that the SLF might play different roles in different hemispheres: the left SLF has a greater involvement in processing language, while the right SLF is closely related to visual spatial processing (41). A previous study supporting this view suggested that the right SLF facilitates rapid, prioritized visual-spatial processing in the right hemisphere (48). The greater correlation of the right SLF with CT might indicate a more substantial impact of CT experiences on individual visual processing-related brain regions. Additionally, in most individuals, the left hemisphere is notably less dominant in processing negative emotions (49). Therefore, CT might have a more widespread impact on the right hemisphere, known for its dominance in emotional processing, by affecting emotion regulation-related brain regions. Integrating these results, we speculate that CT experiences might subtly influence the right SLF in MDD patients, potentially altering functional networks. This alteration could impact the emotional and cognitive regulation functions of patients, potentially forming the basis for psychiatric symptoms. While these conclusions need further confirmation in future research, our findings might provide new insights into how and to what extent CT may influence the pathogenesis and progression of MDD.
Our study included drug-naive first-episode MDD patients and healthy individuals, and divided the patient and control groups into different subgroups based on CT experiences to explore the separate and interactive effects of CT and MDD diagnosis on individual brain WM fiber bundles. Our research provides new insights into the impact of CT experiences on MDD patients. However, this study has some limitations. Firstly, participants who experienced SA were quite rare in our study, which might limit our ability to detect brain changes related to this subscale. The reason for this phenomenon might be that many participants are unwilling to disclose. Future research should identify SA experiences or specifically study the impact of SA experiences on individual brain WM structure. Additionally, as the MDD-nCT patient group and HC-CT group had relatively small sample sizes, these results should be analyzed and interpreted cautiously, and future studies should include more samples. Then, our study focused solely on the FA. Although FA is widely used due to its sensitivity to microstructural changes (50), other DTI metrics such as mean diffusivity, radial diffusivity, and axial diffusivity also provide unique and complementary information about brain tissue characteristics. Future research could benefit from an integrated analysis incorporating these DTI metrics, which could lead to more comprehensive results. Lastly, the CTQ is a retrospective questionnaire, which may be subject to recall bias. Although previous research has shown that self-recollection can provide reliable information (51, 52), in MDD patients, due to their more negative self-evaluation and cognition, recollections of childhood experiences might be more negative. Future research can avoid this flaw through prospective studies or structured interviews.
This study explored the specific changes in brain WM structure in drug-naive first-episode MDD patients who have experienced CT. MDD individuals exhibited reduced FA in the right IFOF, PLIC, and bilateral SLF compared to HCs. Notably, the right SLF showed a significant reduction in FA and negative correlation with CT. Our findings suggest that the brain development of MDD patients might be latently influenced by CT, potentially linked to individual differences in resilience, with the SLF playing a pivotal role. These findings help us understand the role of the SLF in the pathophysiology of MDD and the neurobiological mechanisms by which CT influences MDD.
The raw data supporting the conclusions of this article will be made available by the authors, without undue reservation.
The studies involving human participants were reviewed and approved by the Ethics Committee of the Renmin Hospital of Wuhan University (WDRY2020-K236). The studies were conducted in accordance with the local legislation and institutional requirements. The participants provided their written informed consent to participate in this study. Written informed consent was obtained from the individual(s) for the publication of any potentially identifiable images or data included in this article.
HZ: Conceptualization, Data curation, Formal analysis, Investigation, Methodology, Validation, Visualization, Writing – original draft, Writing – review & editing. BR: Conceptualization, Data curation, Formal analysis, Investigation, Methodology, Validation, Writing – review & editing. GG: Conceptualization, Data curation, Investigation, Methodology, Validation, Writing – review & editing. MZ: Data curation, Investigation, Methodology, Validation, Writing – review & editing. JH: Data curation, Investigation, Validation, Writing – review & editing. NT: Data curation, Investigation, Resources, Writing – review & editing. LB: Data curation, Investigation, Resources, Writing – review & editing. LX: Conceptualization, Methodology, Resources, Supervision, Validation, Writing – review & editing. GW: Conceptualization, Funding acquisition, Project administration, Resources, Supervision, Validation, Writing – review & editing.
The author(s) declare that financial support was received for the research, authorship, and/or publication of this article. This study was supported by the National Natural Science Foundation of China (No.81871072 and No.82071523) and the Medical Science Advancement Program of Wuhan University (No. TFLC2018001). Design of this study was supported by the Key Research and Development Program of Hubei Province (2020BCA064).
The authors declare that the research was conducted in the absence of any commercial or financial relationships that could be construed as a potential conflict of interest.
All claims expressed in this article are solely those of the authors and do not necessarily represent those of their affiliated organizations, or those of the publisher, the editors and the reviewers. Any product that may be evaluated in this article, or claim that may be made by its manufacturer, is not guaranteed or endorsed by the publisher.
1. Marx W, Penninx BWJH, Solmi M, Furukawa TA, Firth J, Carvalho AF, et al. Major depressive disorder. Nat Rev Dis Primers. (2023) 9:44. doi: 10.1038/s41572-023-00454-1
2. Whiteford HA, Degenhardt L, Rehm J, Baxter AJ, Ferrari AJ, Erskine HE, et al. Global burden of disease attributable to mental and substance use disorders: findings from the Global Burden of Disease Study 2010. Lancet. (2013) 382:1575–86. doi: 10.1016/S0140-6736(13)61611-6
3. Kessler RC, Bromet EJ. The epidemiology of depression across cultures. Annu Rev Public Health. (2013) 34:119–38. doi: 10.1146/annurev-publhealth-031912-114409
4. Scheuer S, Wiggert N, Brückl TM, Awaloff Y, Uhr M, Lucae S, et al. Childhood abuse and depression in adulthood: The mediating role of allostatic load. Psychoneuroendocrinology. (2018) 94:134–42. doi: 10.1016/j.psyneuen.2018.04.020
5. Gardner MJ, Thomas HJ, Erskine HE. The association between five forms of child maltreatment and depressive and anxiety disorders: A systematic review and meta-analysis. Child Abuse Negl. (2019) 96:104082. doi: 10.1016/j.chiabu.2019.104082
6. Kisely S, Abajobir AA, Mills R, Strathearn L, Clavarino A, Najman JM. Child maltreatment and mental health problems in adulthood: birth cohort study. Br J Psychiatry. (2018) 213:698–703. doi: 10.1192/bjp.2018.207
7. Lippard ETC, Nemeroff CB. The devastating clinical consequences of child abuse and neglect: increased disease vulnerability and poor treatment response in mood disorders. Am J Psychiatry. (2020) 177:20–36. doi: 10.1176/appi.ajp.2019.19010020
8. Williams LM, Debattista C, Duchemin A-M, Schatzberg AF, Nemeroff CB. Childhood trauma predicts antidepressant response in adults with major depression: data from the randomized international study to predict optimized treatment for depression. Transl Psychiatry. (2016) 6:e799. doi: 10.1038/tp.2016.61
9. Medeiros GC, Prueitt WL, Minhajuddin A, Patel SS, Czysz AH, Furman JL, et al. Childhood maltreatment and impact on clinical features of major depression in adults. Psychiatry Res. (2020) 293:113412. doi: 10.1016/j.psychres.2020.113412
10. Cohen-Woods S, Fisher HL, Ahmetspahic D, Douroudis K, Stacey D, Hosang GM, et al. Interaction between childhood maltreatment on immunogenetic risk in depression: Discovery and replication in clinical case-control samples. Brain Behav Immun. (2018) 67:203–10. doi: 10.1016/j.bbi.2017.08.023
11. Teicher MH, Samson JA, Anderson CM, Ohashi K. The effects of childhood maltreatment on brain structure, function and connectivity. Nat Rev Neurosci. (2016) 17:652–66. doi: 10.1038/nrn.2016.111
12. Weissman DG, Lambert HK, Rodman AM, Peverill M, Sheridan MA, McLaughlin KA. Reduced hippocampal and amygdala volume as a mechanism underlying stress sensitization to depression following childhood trauma. Depress Anxiety. (2020) 37:916–25. doi: 10.1002/da.23062
13. Nogovitsyn N, Addington J, Souza R, Placsko TJ, Stowkowy J, Wang J, et al. Childhood trauma and amygdala nuclei volumes in youth at risk for mental illness. Psychol Med. (2022) 52:1192–9. doi: 10.1017/S0033291720003177
14. Riem MME, Alink LRA, Out D, Van Ijzendoorn MH, Bakermans-Kranenburg MJ. Beating the brain about abuse: Empirical and meta-analytic studies of the association between maltreatment and hippocampal volume across childhood and adolescence. Dev Psychopathol. (2015) 27:507–20. doi: 10.1017/S0954579415000127
15. McCarthy-Jones S, Oestreich LKL, Lyall AE, Kikinis Z, Newell DT, Savadjiev P, et al. Childhood adversity associated with white matter alteration in the corpus callosum, corona radiata, and uncinate fasciculus of psychiatrically healthy adults. Brain Imaging Behav. (2018) 12:449–58. doi: 10.1007/s11682-017-9703-1
16. Tendolkar I, Mårtensson J, Kühn S, Klumpers F, Fernández G. Physical neglect during childhood alters white matter connectivity in healthy young males. Hum Brain Mapp. (2018) 39:1283–90. doi: 10.1002/hbm.23916
17. He J, Zhong X, Cheng C, Dong D, Zhang B, Wang X, et al. Characteristics of white matter structural connectivity in healthy adults with childhood maltreatment. Eur J Psychotraumatol. (2023) 14:2179278. doi: 10.1080/20008066.2023.2179278
18. Wu Z, Luo Q, Wu H, Wu Z, Zheng Y, Yang Y, et al. Amplitude of low-frequency oscillations in major depressive disorder with childhood trauma. Front Psychiatry. (2020) 11:596337. doi: 10.3389/fpsyt.2020.596337
19. Wang X, Liu Q, Fan J, Gao F, Xia J, Liu X, et al. Decreased functional coupling within default mode network in major depressive disorder with childhood trauma. J Psychiatr Res. (2022) 154:61–70. doi: 10.1016/j.jpsychires.2022.07.051
20. Yu M, Linn KA, Shinohara RT, Oathes DJ, Cook PA, Duprat R, et al. Childhood trauma history is linked to abnormal brain connectivity in major depression. Proc Natl Acad Sci U.S.A. (2019) 116:8582–90. doi: 10.1073/pnas.1900801116
21. Xu Z, Zhang J, Wang D, Wang T, Zhang S, Ren X, et al. Altered brain function in drug-naïve major depressive disorder patients with early-life maltreatment: A resting-state fMRI study. Front Psychiatry. (2019) 10:255. doi: 10.3389/fpsyt.2019.00255
22. Kim SY, An SJ, Han JH, Kang Y, Bae EB, Tae W-S, et al. Childhood abuse and cortical gray matter volume in patients with major depressive disorder. Psychiatry Res. (2023) 319:114990. doi: 10.1016/j.psychres.2022.114990
23. Liu Y, Zhang J, Zhang M, Li X, Li K, Wang B, et al. Abnormal brain gray matter volume in patients with major depressive disorder: Associated with childhood trauma? J Affect Disord. (2022) 308:562–8. doi: 10.1016/j.jad.2022.04.083
24. Kang W, Kang Y, Kim A, Kim H, Han K-M, Ham B-J. Gray and white matter abnormalities in major depressive disorder patients and its associations with childhood adversity. J Affect Disord. (2023) 330:16–23. doi: 10.1016/j.jad.2023.02.145
25. Poletti S, Aggio V, Brioschi S, Bollettini I, Falini A, Colombo C, et al. Impact of early and recent stress on white matter microstructure in major depressive disorder. J Affect Disord. (2018) 225:289–97. doi: 10.1016/j.jad.2017.08.017
26. Dillon DG, Gonenc A, Belleau E, Pizzagalli DA. Depression is associated with dimensional and categorical effects on white matter pathways. Depress Anxiety. (2018) 35:440–7. doi: 10.1002/da.22734
27. Ahn S, Lee S-K. Diffusion tensor imaging: exploring the motor networks and clinical applications. Korean J Radiol. (2011) 12:651–61. doi: 10.3348/kjr.2011.12.6.651
28. Van Hecke W, Leemans A, Emsell L. DTI analysis Methods: Voxel-Based Analysis. In: Van Hecke W, Emsell L, Sunaert S, editors. Diffusion Tensor Imaging. Springer New York, New York, NY (2016). p. 183–203. doi: 10.1007/978-1-4939-3118-7_10
29. He J, Zhong X, Gao Y, Xiong G, Yao S. Psychometric properties of the Chinese version of the Childhood Trauma Questionnaire-Short Form (CTQ-SF) among undergraduates and depressive patients. Child Abuse Negl. (2019) 91:102–8. doi: 10.1016/j.chiabu.2019.03.009
30. van Velzen LS, Kelly S, Isaev D, Aleman A, Aftanas LI, Bauer J, et al. White matter disturbances in major depressive disorder: a coordinated analysis across 20 international cohorts in the ENIGMA MDD working group. Mol Psychiatry. (2020) 25:1511–25. doi: 10.1038/s41380-019-0477-2
31. Liu X, Watanabe K, Kakeda S, Yoshimura R, Abe O, Ide S, et al. Relationship between white matter integrity and serum cortisol levels in drug-naive patients with major depressive disorder: diffusion tensor imaging study using tract-based spatial statistics. Br J Psychiatry. (2016) 208:585–90. doi: 10.1192/bjp.bp.114.155689
32. Sugimoto K, Kakeda S, Watanabe K, Katsuki A, Ueda I, Igata N, et al. Relationship between white matter integrity and serum inflammatory cytokine levels in drug-naive patients with major depressive disorder: diffusion tensor imaging study using tract-based spatial statistics. Transl Psychiatry. (2018) 8:141. doi: 10.1038/s41398-018-0174-y
33. Beaulieu C. The basis of anisotropic water diffusion in the nervous system - a technical review. NMR BioMed. (2002) 15:435–55. doi: 10.1002/nbm.782
34. Lim L, Howells H, Radua J, Rubia K. Aberrant structural connectivity in childhood maltreatment: A meta-analysis. Neurosci Biobehav Rev. (2020) 116:406–14. doi: 10.1016/j.neubiorev.2020.07.004
35. Monteleone AM, Monteleone P, Esposito F, Prinster A, Ruzzi V, Canna A, et al. The effects of childhood maltreatment on brain structure in adults with eating disorders. World J Biol Psychiatry. (2019) 20:301–9. doi: 10.1080/15622975.2017.1395071
36. Voineskos AN, Mulsant BH, Dickie EW, Neufeld NH, Rothschild AJ, Whyte EM, et al. Effects of antipsychotic medication on brain structure in patients with major depressive disorder and psychotic features: neuroimaging findings in the context of a randomized placebo-controlled clinical trial. JAMA Psychiatry. (2020) 77:674–83. doi: 10.1001/jamapsychiatry.2020.0036
37. Catani M, Thiebaut de Schotten M. A diffusion tensor imaging tractography atlas for virtual in vivo dissections. Cortex. (2008) 44:1105–32. doi: 10.1016/j.cortex.2008.05.004
38. Zarei M, Johansen-Berg H, Jenkinson M, Ciccarelli O, Thompson AJ, Matthews PM. Two-dimensional population map of cortical connections in the human internal capsule. J Magn Reson Imaging. (2007) 25:48–54. doi: 10.1002/jmri.20810
39. St Onge JR, Stopper CM, Zahm DS, Floresco SB. Separate prefrontal-subcortical circuits mediate different components of risk-based decision making. J Neurosci. (2012) 32:2886–99. doi: 10.1523/JNEUROSCI.5625-11.2012
40. Nakajima R, Kinoshita M, Shinohara H, Nakada M. The superior longitudinal fascicle: reconsidering the fronto-parietal neural network based on anatomy and function. Brain Imaging Behav. (2020) 14:2817–30. doi: 10.1007/s11682-019-00187-4
41. Janelle F, Iorio-Morin C, D’amour S, Fortin D. Superior longitudinal fasciculus: A review of the anatomical descriptions with functional correlates. Front Neurol. (2022) 13:794618. doi: 10.3389/fneur.2022.794618
42. Lim L, Hart H, Howells H, Mehta MA, Simmons A, Mirza K, et al. Altered white matter connectivity in young people exposed to childhood abuse: a tract-based spatial statistics (TBSS) and tractography study. J Psychiatry Neurosci. (2019) 44:E11–20. doi: 10.1503/jpn.170241
43. Mandelli L, Petrelli C, Serretti A. The role of specific early trauma in adult depression: A meta-analysis of published literature. Childhood trauma and adult depression. Eur Psychiatry. (2015) 30:665–80. doi: 10.1016/j.eurpsy.2015.04.007
44. Park JY, Lee CW, Jang Y, Lee W, Yu H, Yoon J, et al. Relationship between childhood trauma and resilience in patients with mood disorders. J Affect Disord. (2023) 323:162–70. doi: 10.1016/j.jad.2022.11.003
45. Uchida Y, Takahashi T, Katayama S, Masuya J, Ichiki M, Tanabe H, et al. Influence of trait anxiety, child maltreatment, and adulthood life events on depressive symptoms. Neuropsychiatr Dis Treat. (2018) 14:3279–87. doi: 10.2147/NDT.S182783
46. Cai J, Li J, Liu D, Gao S, Zhao Y, Zhang J, et al. Long-term effects of childhood trauma subtypes on adult brain function. Brain Behav. (2023) 13:e2981. doi: 10.1002/brb3.2981
47. Tatham EL, Ramasubbu R, Gaxiola-Valdez I, Cortese F, Clark D, Goodyear B, et al. White matter integrity in major depressive disorder: Implications of childhood trauma, 5-HTTLPR and BDNF polymorphisms. Psychiatry Res Neuroimaging. (2016) 253:15–25. doi: 10.1016/j.pscychresns.2016.04.014
48. Thiebaut de Schotten M, Dell’Acqua F, Forkel SJ, Simmons A, Vergani F, Murphy DGM, et al. A lateralized brain network for visuospatial attention. Nat Neurosci. (2011) 14:1245–6. doi: 10.1038/nn.2905
49. Schiffer F, Teicher MH, Anderson C, Tomoda A, Polcari A, Navalta CP, et al. Determination of hemispheric emotional valence in individual subjects: a new approach with research and therapeutic implications. Behav Brain Funct. (2007) 3:13. doi: 10.1186/1744-9081-3-13
50. Figley CR, Uddin MN, Wong K, Kornelsen J, Puig J, Figley TD. Potential pitfalls of using fractional anisotropy, axial diffusivity, and radial diffusivity as biomarkers of cerebral white matter microstructure. Front Neurosci. (2021) 15:799576. doi: 10.3389/fnins.2021.799576
51. Danese A, Widom CS. Objective and subjective experiences of child maltreatment and their relationships with psychopathology. Nat Hum Behav. (2020) 4:811–8. doi: 10.1038/s41562-020-0880-3
Keywords: major depressive disorder, childhood trauma, diffusion tensor imaging(DTI), fractional anisotropy, tract-based spatial statistics(TBSS)
Citation: Zhao H, Rong B, Gao G, Zhou M, Huang J, Tu N, Bu L, Xiao L and Wang G (2024) Alterations in the white matter structure of major depressive disorder patients and their link to childhood trauma. Front. Psychiatry 15:1364786. doi: 10.3389/fpsyt.2024.1364786
Received: 03 January 2024; Accepted: 19 February 2024;
Published: 04 March 2024.
Edited by:
Panagiotis Ferentinos, National and Kapodistrian University of Athens, GreeceReviewed by:
Foteini Christidi, National and Kapodistrian University of Athens, GreeceCopyright © 2024 Zhao, Rong, Gao, Zhou, Huang, Tu, Bu, Xiao and Wang. This is an open-access article distributed under the terms of the Creative Commons Attribution License (CC BY). The use, distribution or reproduction in other forums is permitted, provided the original author(s) and the copyright owner(s) are credited and that the original publication in this journal is cited, in accordance with accepted academic practice. No use, distribution or reproduction is permitted which does not comply with these terms.
*Correspondence: Gaohua Wang, d2doNjQwMkB3aHUuZWR1LmNu; Ling Xiao, bGluZ3hpYW94aWFvQHdodS5lZHUuY24=
Disclaimer: All claims expressed in this article are solely those of the authors and do not necessarily represent those of their affiliated organizations, or those of the publisher, the editors and the reviewers. Any product that may be evaluated in this article or claim that may be made by its manufacturer is not guaranteed or endorsed by the publisher.
Research integrity at Frontiers
Learn more about the work of our research integrity team to safeguard the quality of each article we publish.