- 1Defense Advanced Research Projects Agency (DARPA), Biological Technologies Office (BTO), Arlington, VA, United States
- 2U.S. Army DEVCOM Chemical and Biological Center, Aberdeen, MD, United States
- 3Neuroscience Institute, Allegheny Health Network, Pittsburgh, PA, United States
The rapid rise in deaths since 2012 due to opioid poisoning is correlated with the proliferation of potent synthetic opioid agonists such as fentanyl, acrylfentanyl, and carfentanil. The efficacy of frontline antidotes such as naloxone in reversing such poisoning events has been questioned, and the possibility of naloxone-resistant synthetic opioids has been raised. In this manuscript, we applied in vitro techniques to establish the median effective inhibitory concentrations for fentanyl, acrylfentanyl, and carfentanil and subsequently evaluate naloxone’s ability to reverse agonist–receptor interactions.
Introduction
Synthetic mu-opioid receptor (MOR) agonists derived from the 4-anilinopiperidine scaffold are responsible for the exponential increase in opioid overdose deaths and poisoning (1–7). In the past several years, progress toward stemming the tide of death has been reduced due to the increasing potency of synthetic opioids and limited healthcare access (8–10). These compounds are relatively straightforward to prepare from available precursors and are used either as adulterants to other opioids or directly used (11–14). Multiple factors that contribute to the lethality of synthetic opioids are their a) rapid onset, b) high potencies (20 to 2000 times greater than morphine), and c) relatively long pharmacokinetics compared to frontline antidotes (15–21). These factors have led to critical questioning of the potency and efficacy of the frontline antidote naloxone to reverse synthetic opioid poisoning (22–26).
Two major mechanisms postulating the limitations of standard naloxone antidote dosing, in the event of synthetic opioid poisoning, have been put forward. The renarcotization theory states that the pharmacokinetic mismatch between naloxone and synthetic opioids causes a single naloxone dose to be insufficient at sustained overdose reversal (27–33). Naloxone resistance is a theorized phenomenon where naloxone has a limited and dulled capacity to reverse synthetic opioid agonist poisoning (22, 34). The idea of naloxone resistance was first put forward by Maryanoff and coworkers in 1982 and has since found reference in both popular press and scientific literature (35). The competing theory of renarcotization being the source of naloxone’s limitations stems from the metabolic mismatch between naloxone and synthetic opioids (36–42). Naloxone is, compared to synthetic opioids, relatively hydrophilic and is rapidly metabolized by the UGT2B7 glucuronidase enzyme leading to the production of the brain-impenetrable naloxone-3-glucuronide (43, 44). In contrast, synthetic opioids are passively absorbed by adipose tissue due to inherent hydrophobicity and, upon naloxone’s metabolism, are able to agonize the MOR, resulting in renarcotization (17, 45–48). In this manuscript, we sought to evaluate two potent synthetic MOR agonists both postulated to have potential naloxone resistance, resulting in the need for new countermeasures and antidotes for these compounds.
To achieve our aims, an in vitro method using a commercially validated CHO-K1 cell line expressing human MOR was employed to directly determine a median effective concentration (EC50) for fentanyl, carfentanil, and acrylfentanyl (Figure 1). Median inhibitory concentrations (IC50) for fentanyl, carfentanil, and acrylfentanyl after competition with naloxone were also generated to assess the reversibility of the receptor–ligand interaction.
Materials and methods
Chemicals
The LANCE Ultra cAMP assay point kit and 384-well ProxiPlate were purchased from Perkin Elmer (Shelton, CT, USA). The LANCE Ultra kit consisted of cAMP Standard (50 µM), Eu-cAMP tracer (ULight™-anti-cAMP), cAMP Detection Buffer, and BSA Stabilizer. Carfentanil was synthesized at the U.S. Army DEVCOM Chemical Biological Center (CBC) (APG, MD, USA), and purity was verified by 13C and 1H NMR. [d-Ala2,NMe-Phe4,Gly-ol5]-Enkephalin (DAMGO) was purchased from Tocris Bioscience (Park Ellisville, MO, USA). Hanks’ Balanced Salt Solution (HBSS) 1X, 4-(2-hydroxyethyl)-1-piperazineethanesulfonic acid (HEPES) 1M, Versene Solution, and Geneticin were procured from Life Technologies (Grand Island, NY, USA). Dimethyl sulfoxide (DMSO), 3-isobutyl-1-methylxanthine (IBMX), and forskolin were procured from Sigma-Aldrich (St. Louis, MO, USA). Dulbecco’s Phosphate-Buffered Saline (DPBS)/Modified Buffer and Ham’s F-12 Media were procured from HyClone Laboratories, Inc. (Logan, UT, USA). Fetal bovine serum (FBS) was procured from Mediatech, Inc. (Manassas, VA, USA). Fentanyl citrate was procured from Mallinckrodt Pharmaceuticals (St. Louis, MO, USA). Acrylfentanyl HCl was procured from Cayman Chemical (Ann Arbor, MI, USA).
Cell line
ValiScreen CHO-K1 cells expressing human MOR (ES-542-C) were purchased from Perkin Elmer, Inc. (Waltham, MA, USA). The cells were kept frozen in liquid nitrogen storage (vapor phase) until they were cultured. The cells were grown in accordance with product literature provided by Perkin Elmer. The cell cultures were split when they reached ~60%–80% confluence, and no cells were used past passage 10. Cells were used for opioid assay when they met the requirements described in the product literature (i.e., 60%–80% confluence). Before use, cellular solutions used in plating were counted on a Vi-CELL XR hemocytometer (Beckman Coulter Life Sciences, Indianapolis, IN, USA). The cells were plated at a concentration of 2.0 × 105 cells/mL.
Incubation and standard solutions
Standard solutions of fentanyl, carfentanil, acrylfentanyl, and naloxone (10 mM) were made in DMSO and stored until use in a freezer at −20°C. A standard solution of DAMGO (1.95 mM) was made in sterile water. Working solutions of fentanyl, carfentanil, acrylfentanyl, naloxone, and DAMGO (500 µM) were prepared immediately before the assay was performed in a fresh stimulation buffer. Stimulation buffer, forskolin dilutions, and cAMP standards were made, as needed, in accordance with the Lance Ultra cAMP assay protocol immediately before the assay was performed.
For the competition assay to assess naloxone requirements, each agonist’s EC90 was co-incubated in each well concurrently with naloxone concentrations ranging from 100 µM to 10 fM in full log intervals. A zero-naloxone concentration control was also included.
Statistical analysis
Median effective concentrations of DAMGO, fentanyl, carfentanil, and acrylfentanyl were calculated using GraphPad Prism v9.0.1 (GraphPad Software, Inc., La Jolla, CA, USA). Data were first normalized to the minimal and maximal responses of the control compound, DAMGO, and then non-linear regression was fit to each dose–response profile using the three-parameter [agonist] vs. normalized response function within the software. Ordinary one-way ANOVA tests using multiple comparisons were then conducted to assess the statistical significance of the EC50 values. EC90 values were then calculated similarly for each of the agonists for use in the competition assay that followed.
Median inhibitory concentrations of naloxone were then calculated for challenges with the EC90 values of DAMGO, fentanyl, carfentanil, and acrylfentanyl. This was performed similarly to the EC50 determination study with the exception that for this set of experiments, the non-linear regression function used was a three-parameter [inhibitor] vs. normalized response. Ordinary one-way ANOVA tests using multiple comparisons were similarly conducted to assess the statistical significance of the IC50 values against each of their challenge doses.
Results and discussion
Agonist dose–response
To establish baseline agonist–receptor activation, we generated full dose–response curves for DAMGO, fentanyl, carfentanil, and acrylfentanyl, and we determined EC50, EC90, and relative potency to fentanyl (Figure 2). By using a functional cAMP response cell model to determine agonist potential, we are able to directly ascertain drug potency as compared to pure receptor-radio-ligand binding assays, which may not reflect the true potency of agonists. Our studies were able to determine the compounds’ relative potency to fentanyl (Table 1) and several interesting points based on the EC50 values. Contrary to the initial literature report by Maryanoff, which found acrylfentanyl to have a potency greater than that of fentanyl, we found that acrylfentanyl had only ~50% of the potency of fentanyl. In contrast, we determined that carfentanil’s potency was nearly 100× that of fentanyl. The determined EC50 and EC90 values enable naloxone reversal assays.
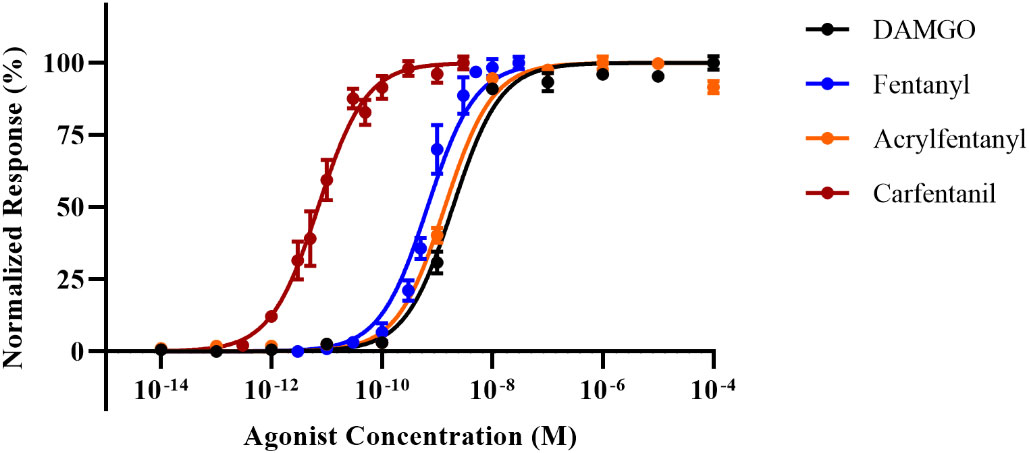
Figure 2 Dose–response curves for [d-Ala2,NMe-Phe4,Gly-ol5]-enkephalin (DAMGO), fentanyl, carfentanil, and acrylfentanyl. Data points plotted as mean ± standard error of the mean (SEM).
Antagonist performance of naloxone
In order to directly ascertain naloxone’s ability to reverse the agonist activity of the synthetic opioids, cells were stimulated with an EC90 of each compound, and a dose–response curve with naloxone was generated against each compound. The EC50 and EC90 values (Table 1) were determined in the agonist assay for fentanyl and acrylfentanyl. Cells were incubated with EC90 of DAMGO, fentanyl, carfentanil, or acrylfentanyl and log-dosed concentrations of naloxone. The experimental design allowed for the determination of the concentration of naloxone (antagonist) required to reverse an EC90 dose of a synthetic opioid (agonist). By maintaining an EC90 of agonist in each well and varying the concertation of naloxone, backward-S dose–response curves (Figure 3) were generated, and the IC50 values could be calculated for naloxone against the respective synthetic opioids (Table 2).
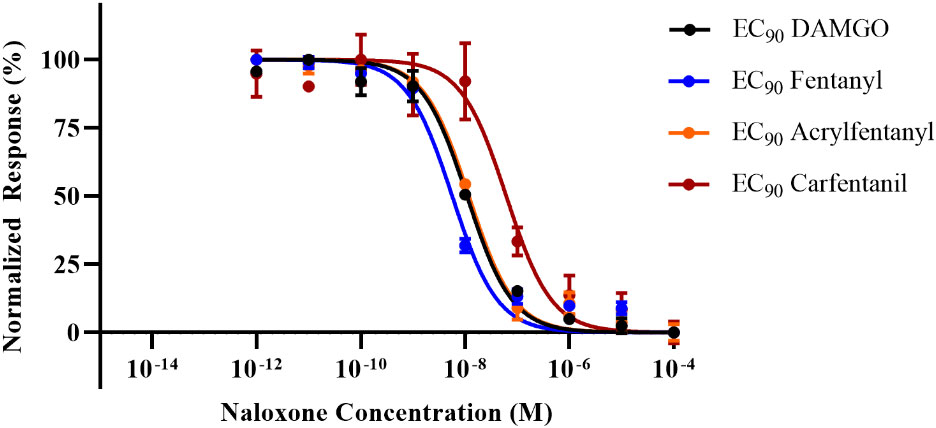
Figure 3 Inhibition dose–response curves for naloxone against challenges of the EC90 for each agonist. Data points plotted as mean ± SEM.
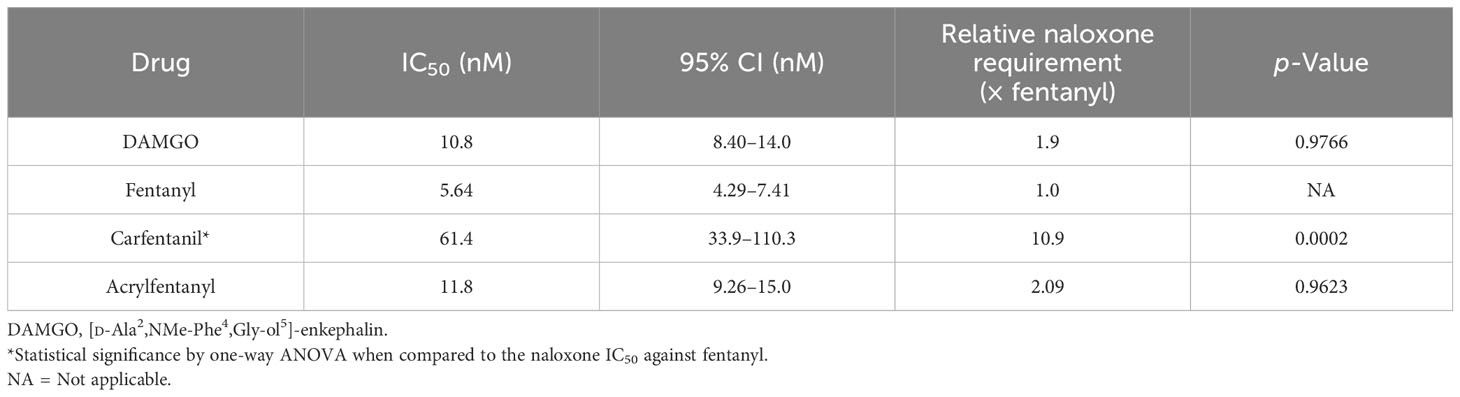
Table 2 IC50 values for naloxone when challenged with EC90 values of DAMGO, fentanyl, and acrylfentanyl.
While the results of the agonist reversal assay clearly demonstrate that the agonist activities of acrylfentanyl and carfentanil can both be reversed with naloxone, several interesting phenomena were observed. The first observation was that although acrylfentanyl had approximately one-half the potency of fentanyl, this compound required nearly double the amount of naloxone to reverse its agonist activity vs. fentanyl. Carfentanil, however, demonstrated potency roughly 100× that of fentanyl and required a significantly greater concentration of naloxone in order to antagonize a challenge of its EC90. Further, this demonstrates that reports of naloxone resistance need to be verified scientifically, as carfentanil appears to require more naloxone in order to reverse its effects, but this is not due to any detection of irreversible binding, but rather in this test, the system appears to be due to affinity for the receptor and functional agonistic potency.
While this study aims to equivocally characterize the receptor–ligand interaction of fentanyl-class opioid agonists at the MOR, it does not provide guidance for dosing requirements in instances of treatment of opioid overdose. This study merely aims to provide evidence that the interaction between a ligand, acrylfentanyl, and its primary target receptor is indeed a reversible interaction and thus able to be reversed in proper conditions, contrary to what has been reported in mass media. In agreement with our approach and conclusions, studies performed in humans with radiolabeled carfentanil also demonstrate rapid distribution of naloxone to the brain and subsequent displacement of [11C]-carfentanil shortly after administration followed by rapid redistribution of naloxone out of the central nervous system (CNS). The lower receptor affinity of naloxone enables its displacement from the MOR by carfentanil, practically demonstrating how naloxone resistance may occur in living systems (40). These data support our hypothesis that carfentanil, despite its heroic potency at the MOR, is able to be displaced even by nominal dosing of naloxone. Similarly, the authors of this study acknowledge that their work, too, does not dictate dosing requirements of naloxone or any other opioid antagonist based on the amount of agonist administered; their work merely demonstrates that the agonist is not irreversibly bound to the receptor but happens to be an incredibly potent agonist, functionally, although it is fully reversible at the molecular level.
Conclusions
In this study, we applied an in vitro live cell receptor activation assay method to determine EC50 values for synthetic MOR agonists. These values were then used to determine the amount of naloxone required to inhibit agonist activity. This straightforward approach allowed for the direct determination that naloxone is capable of reversing the agonist effects of even the most potent of known synthetic opioids (carfentanil). Nevertheless, our data also indicate that the amount of naloxone needed to reverse the agonist activity of synthetic opioids varies compared to fentanyl. Our data show that naloxone is an effective inhibitor of synthetic opioids. Our future studies aim to translate these results to an in vivo animal model of synthetic opioid poisoning to determine the amount of naloxone needed to successfully reverse fentanyl toxicity vs. acrylfentanyl and carfentanil. Finally, this study highlights the need for a longer-acting MOR antagonist that can be sufficiently dosed at levels that do not induce precipitated opioid withdrawal yet can still reverse the most potent synthetic opioids.
Data availability statement
The original contributions presented in the study are included in the article/supplementary material. Further inquiries can be directed to the corresponding author.
Author contributions
MF: Investigation, Methodology, Writing – original draft, Writing – review & editing, Conceptualization, Data curation, Formal analysis, Project administration, Resources, Software, Supervision, Validation, Visualization. TM: Data curation, Formal analysis, Investigation, Writing – original draft. BC: Writing – original draft, Writing – review & editing. SA: Writing – original draft, Writing – review & editing, Funding acquisition, Investigation, Methodology.
Funding
The author(s) declare financial support was received for the research, authorship, and/or publication of this article. The Feasel group gratefully acknowledges the DEVCOM Chemical Biological Center’s (formerly Edgewood Chemical Biological Center) Section 219 Seedling Program, and Dr. Augustus Way Fountain, III, who leads this intramural research program. The Averick group gratefully acknowledges the National Institute of Health CounterACT program and the National Institute of Drug and Alcohol Abuse (DA050565-01).
Conflict of interest
The authors declare that the research was conducted in the absence of any commercial or financial relationships that could be construed as a potential conflict of interest.
Publisher’s note
All claims expressed in this article are solely those of the authors and do not necessarily represent those of their affiliated organizations, or those of the publisher, the editors and the reviewers. Any product that may be evaluated in this article, or claim that may be made by its manufacturer, is not guaranteed or endorsed by the publisher.
References
1. Armenian P, Vo KT, Barr-Walker J, Lynch KL. Fentanyl, fentanyl analogs and novel synthetic opioids: A comprehensive review. Neuropharmacology. (2018) 134:121–32. doi: 10.1016/j.neuropharm.2017.10.016
2. Ujváry I, Jorge R, Christie R, Le Ruez T, Danielsson HV, Kronstrand R, et al. Acryloylfentanyl, a recently emerged new psychoactive substance: a comprehensive review. Forensic Toxicol. (2017) 35:232–43. doi: 10.1007/s11419-017-0367-8.
3. Frank RG, Pollack HA. Addressing the fentanyl threat to public health. New Engl J Med. (2017) 376:605–7. doi: 10.1056/NEJMp1615145.
4. Ruzycki S, Yarema M. Fentanyl misuse. Can Med Assoc J. (2016) 188:673–3. doi: 10.1503/cmaj.151378.
5. Krinsky CS, Lathrop SL, Crossey M, Baker G, Zumwalt R. A toxicology-based review of fentanyl-related deaths in new Mexico (1986–2007). Am J Forensic Med Pathol. (2011) 32:347–51. doi: 10.1097/PAF.0b013e31822ad269.
6. Burns SM, Cunningham CW, Mercer SL. DARK classics in chemical neuroscience: fentanyl. ACS Chem Neurosci. (2018) 9:2428–37. doi: 10.1021/acschemneuro.8b00174.
7. Hayes KL, Lieberman M. Assessment of two brands of fentanyl test strips with 251 synthetic opioids reveals "blind spots" in detection capabilities. Harm Reduct J. (2023) 20:175. doi: 10.1186/s12954-023-00911-w.
8. Stephenson J. CDC warns of surge in drug overdose deaths during COVID-19. JAMA Health Forum. (2021) 2:e210001. doi: 10.1001/jamahealthforum.2021.0001
9. Niles JK, Gudin J, Radcliff J, Kaufman HW. The opioid epidemic within the COVID-19 pandemic: drug testing in 2020. Population Health Manage. (2021) 24:S–43-S-51. doi: 10.1089/pop.2020.0230
10. Kuehn BM. Accelerated overdose deaths linked with COVID-19. Jama. (2021) 325:523. doi: 10.1001/jama.2021.0074.
11. Qin Y, Ni L, Shi J, Zhu Z, Shi S, Lam A-l, et al. Synthesis and biological evaluation of fentanyl analogues modified at phenyl groups with alkyls. ACS Chem Neurosci. (2018) 10:201–8. doi: 10.1021/acschemneuro.8b00363.
12. Lahmann M, Valdez CA, Leif RN, Mayer BP. An efficient, optimized synthesis of fentanyl and related analogs. PloS One. (2014) 9:e108250. doi: 10.1371/journal.pone.0108250.
13. Girón R.o., Abalo R, Goicoechea C, Martıín MI, Callado LF, Cano C, et al. Synthesis and opioid activity of new fentanyl analogs. Life Sci. (2002) 71:1023–34. doi: 10.1016/S0024-3205(02)01798-8.
14. Suh Y-G, Cho K-H, Shin D-Y. Total synthesis of fentanyl. Arch Pharmacal Res. (1998) 21:70–2. doi: 10.1007/BF03216756.
15. Uddayasankar U, Lee C, Oleschuk C, Eschun G, Ariano RE. The pharmacokinetics and pharmacodynamics of carfentanil after recreational exposure: A case report. Pharmacother: J Hum Pharmacol Drug Ther. (2018) 38:e41–5. doi: 10.1002/phar.2117
16. Bell A, Bennett AS, Jones TS, Doe-Simkins M, Williams LD. Amount of naloxone used to reverse opioid overdoses outside of medical practice in a city with increasing illicitly manufactured fentanyl in illicit drug supply. Subst Abuse. (2018) 40:52–5. doi: 10.1080/08897077.2018.1449053.
17. Kuip EJM, Zandvliet ML, Koolen SLW, Mathijssen RHJ, van der Rijt CCD. A review of factors explaining variability in fentanyl pharmacokinetics; focus on implications for cancer patients. Br J Clin Pharmacol. (2017) 83:294–313. doi: 10.1111/bcp.13129.
18. Lindsay CD, Riches JR, Roughley N, Timperley CM. CHAPTER 8. Chemical defence against fentanyls. In: Franz Worek JJ, Thiermann H, editors. Chemical Warfare Toxicology Volume 2. Management of Poisoning Royal Society of Chemistry, Cambridge UK (2016). p. 259–313. doi: 10.1039/9781782628071
19. Lötsch J, Walter C, Parnham MJ, Oertel BG, Geisslinger G. Pharmacokinetics of non-intravenous formulations of fentanyl. Clin Pharmacokinet. (2012) 52:23–36. doi: 10.1007/s40262-012-0016-7
20. Moran TS, Feasel MG. In Vitro Screening of Opioid Antagonist Effectiveness. Techinical Report. Edgewood Chemical Biological Center, USA (2017).
21. Jeffery MM, Stevens M, D'Onofrio G, Melnick ER. Fentanyl-associated overdose deaths outside the hospital. N Engl J Med. (2023) 389:87–8. doi: 10.1056/NEJMc2304991
22. Torralva R, Janowsky A. Noradrenergic mechanisms in fentanyl-mediated rapid death explain failure of naloxone in the opioid crisis. J Pharmacol Exp Ther. (2019) 371:453–75. doi: 10.1124/jpet.119.258566.
23. Dietze P, Jauncey M, Salmon A, Mohebbi M, Latimer J, van Beek I, et al. Effect of intranasal vs intramuscular naloxone on opioid overdose. JAMA Network Open. (2019) 2:e1914977. doi: 10.1001/jamanetworkopen.2019.14977
24. Fairbairn N, Coffin PO, Walley AY. Naloxone for heroin, prescription opioid, and illicitly made fentanyl overdoses: Challenges and innovations responding to a dynamic epidemic. Int J Drug Policy. (2017) 46:172–9. doi: 10.1016/j.drugpo.2017.06.005
25. Zuckerman M, Weisberg SN, Boyer EW. Pitfalls of intranasal naloxone. Prehospital Emergency Care. (2014) 18:550–4. doi: 10.3109/10903127.2014.896961.
26. Amaducci A, Aldy K, Campleman SL, Li S, Meyn A, Abston S, et al. Naloxone use in novel potent opioid and fentanyl overdoses in emergency department patients. JAMA Netw Open. (2023) 6:e2331264. doi: 10.1001/jamanetworkopen.2023.31264
27. Madison CA, Arora M, Kumar MNVR, Eitan S. Novel oral nanoparticle formulation of sustained release naloxone with mild withdrawal symptoms in mice. ACS Chem Neurosci. (2020) 11:1955–64. doi: 10.1021/acschemneuro.0c00141.
28. Kassick AJ, Allen HN, Yerneni SS, Pary F, Kovaliov M, Cheng C, et al. Covalent poly(lactic acid) nanoparticles for the sustained delivery of naloxone. ACS Appl Bio Materials. (2019) 2:3418–28. doi: 10.1021/acsabm.9b00380.
29. Rzasa Lynn R, Galinkin JL. Naloxone dosage for opioid reversal: current evidence and clinical implications. Ther Adv Drug Saf. (2017) 9:63–88. doi: 10.1177/2042098617744161.
30. Dahan A, Aarts L, Smith TW. Incidence, reversal, and prevention of opioid-induced respiratory depression. Anesthesiology. (2010) 112:226–38. doi: 10.1097/ALN.0b013e3181c38c25.
31. Kassick AJ, Treat A, Tomycz N, Feasel MG, Kolber BJ, Averick S. Design, synthesis, and biological evaluation of C(6)-difluoromethylenated epoxymorphinan Mu opioid receptor antagonists. RSC Med Chem. (2022) 13:175–82. doi: 10.1039/D1MD00285F.
32. Kassick AJ, Wu M, Luengas D, Ebqa'ai M, Tharika Nirmani LP, Tomycz N, et al. Covalently loaded naloxone nanoparticles as a long-acting medical countermeasure to opioid poisoning. ACS Pharmacol Transl Sci. (2021) 4:1654–64. doi: 10.1021/acsptsci.1c00168.
33. Lewter LA, Johnson MC, Treat AC, Kassick AJ, Averick S, Kolber BJ. Slow-sustained delivery of naloxone reduces typical naloxone-induced precipitated opioid withdrawal effects in male morphine-dependent mice. J Neurosci Res. (2022) 100:339–52. doi: 10.1002/jnr.24627.
34. Quintas LEM, Moss RB, Pryor MM, Baillie R, Kudrycki K, Friedrich C, et al. Higher naloxone dosing in a quantitative systems pharmacology model that predicts naloxone-fentanyl competition at the opioid mu receptor level. PloS One. (2020) 15:e0234683. doi: 10.1371/journal.pone.0234683.
35. Maryanoff BE, Simon EJ, Gioannini T, Gorissen H. Potential affinity labels for the opiate receptor based on fentanyl and related compounds. J Med Chem. (2002) 25:913–9. doi: 10.1021/jm00350a006.
36. Wilde M, Pichini S, Pacifici R, Tagliabracci A, Busardò FP, Auwärter V, et al. Metabolic pathways and potencies of new fentanyl analogs. Front Pharmacol. (2019) 10. doi: 10.3389/fphar.2019.00238
37. Feasel MG, Wohlfarth A, Nilles JM, Pang S, Kristovich RL, Huestis MA. Metabolism of carfentanil, an ultra-potent opioid, in human liver microsomes and human hepatocytes by high-resolution mass spectrometry. AAPS J. (2016) 18:1489–99. doi: 10.1208/s12248-016-9963-5.
38. Holmquist GL. Opioid metabolism and effects of cytochrome P450. Pain Med. (2009) 10:S20–9. doi: 10.1111/j.1526-4637.2009.00596.x
39. Poklis A. Fentanyl: A review for clinical and analytical toxicologists. J Toxicol: Clin Toxicol. (2008) 33:439–47. doi: 10.3109/15563659509013752.
40. Johansson J, Hirvonen J, Lovró Z, Ekblad L, Kaasinen V, Rajasilta O, et al. Intranasal naloxone rapidly occupies brain mu-opioid receptors in human subjects. Neuropsychopharmacology. (2019) 44:1667–73. doi: 10.1038/s41386-019-0368-x.
41. Tylleskar I, Skulberg AK, Skarra S, Nilsen T, Dale O. Pharmacodynamics and arteriovenous difference of intravenous naloxone in healthy volunteers exposed to remifentanil. Eur J Clin Pharmacol. (2018) 74:1547–53. doi: 10.1007/s00228-018-2545-y.
42. McDonald R, Lorch U, Woodward J, Bosse B, Dooner H, Mundin G, et al. Pharmacokinetics of concentrated naloxone nasal spray for opioid overdose reversal: Phase I healthy volunteer study. Addiction. (2018) 113:484–93. doi: 10.1111/add.14033
43. Seo K-A, Kim H-J, Jeong ES, Abdalla N, Choi C-S, Kim D-H, et al. In vitro assay of six UDP-glucuronosyltransferase isoforms in human liver microsomes, using cocktails of probe substrates and liquid chromatography–tandem mass spectrometry. Drug Metab Disposition. (2014) 42:1803–10. doi: 10.1124/dmd.114.058818
44. Di Marco A, D'Antoni M, Attaccalite S, Carotenuto P, Laufer R. Determination of drug glucuronidation and udp-glucuronosyltransferase selectivity using a 96-well radiometric assay. Drug Metab Disposition. (2005) 33:812–9. doi: 10.1124/dmd.105.004333.
45. Leen JLS, Juurlink DN. Carfentanil: a narrative review of its pharmacology and public health concerns. Can J Anesthesia/Journal canadien d'anesthésie. (2019) 66:414–21. doi: 10.1007/s12630-019-01294-y.
46. Gholami N, Farnaghi F, Saberi M, Zamani N, McDonald R, Hassanian-Moghaddam H. A study of the effectiveness of naltrexone in preventing recurrence of methadone poisoning in opioid-naive children. Drug Alcohol Depend. (2021) 219:108425. doi: 10.1016/j.drugalcdep.2020.108425.
47. Raleigh MD, Baruffaldi F, Peterson SJ, Le Naour M, Harmon TM, Vigliaturo JR, et al. and protects against fentanyl-induced effects in mice and rats. J Pharmacol Exp Ther. (2019) 368:282–91. doi: 10.1124/jpet.118.253674.
Keywords: opioid, fentanyl, overdose, naloxone, in vitro
Citation: Feasel MG, Moran TS, Cheng BC and Averick S (2024) Are carfentanil and acrylfentanyl naloxone resistant? Front. Psychiatry 15:1359851. doi: 10.3389/fpsyt.2024.1359851
Received: 22 December 2023; Accepted: 25 January 2024;
Published: 20 February 2024.
Edited by:
Phil Skolnick, Indivior, Inc., United StatesReviewed by:
Mary G. Hornick, Roosevelt University, United StatesMaryam Akhgari, Iranian Legal Medicine Organization, Iran
Copyright © 2024 Feasel, Moran, Cheng and Averick. This is an open-access article distributed under the terms of the Creative Commons Attribution License (CC BY). The use, distribution or reproduction in other forums is permitted, provided the original author(s) and the copyright owner(s) are credited and that the original publication in this journal is cited, in accordance with accepted academic practice. No use, distribution or reproduction is permitted which does not comply with these terms.
*Correspondence: Saadyah Averick, U2FhZHlhaC5BdmVyaWNrQGFobi5vcmc=