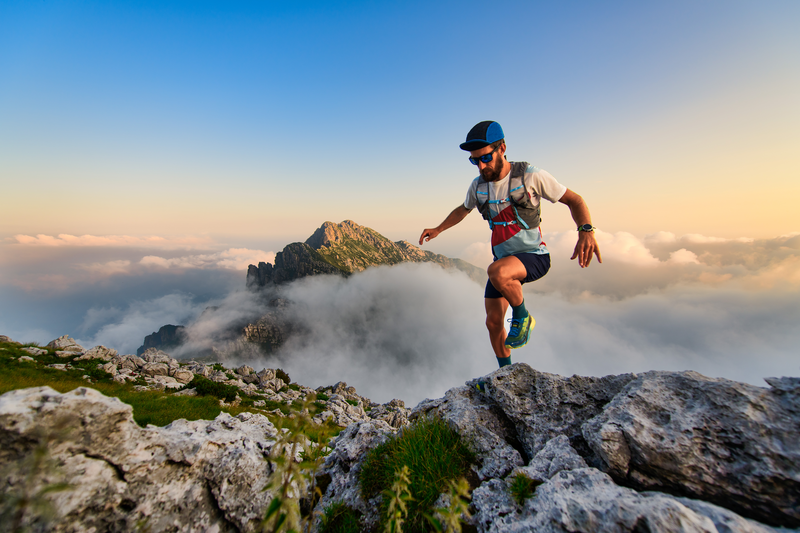
94% of researchers rate our articles as excellent or good
Learn more about the work of our research integrity team to safeguard the quality of each article we publish.
Find out more
MINI REVIEW article
Front. Psychiatry , 06 June 2024
Sec. Anxiety and Stress Disorders
Volume 15 - 2024 | https://doi.org/10.3389/fpsyt.2024.1356563
This article is part of the Research Topic The underlying mechanisms of stress related disorders and potential treatment maneuvers View all 8 articles
Introduction: Post-traumatic stress disorder (PTSD) is a psychiatric disorder triggered by exposure to a life-threatening or sexually violent traumatic event, and is characterized by symptoms involving intrusive re-experiencing, persistent avoidance of associated stimuli, emotional and cognitive disturbances, and hyperarousal for long periods after the trauma has occurred. These debilitating symptoms induce occupational and social impairments that contribute to a significant clinical burden for PTSD patients, and substantial socioeconomic costs, reaching approximately $20,000 dollars per individual with PTSD each year in the US. Despite increased translational research focus in the field of PTSD, the development of novel, effective pharmacotherapies for its treatment remains an important unmet clinical need.
Observations: In this review, we summarize the evidence implicating dysfunctional activity of the amygdala in the pathophysiology of PTSD. We identify the transient receptor potential canonical (TRPC) ion channels as promising drug targets given their distribution in the amygdala, and evidence from animal studies demonstrating their role in fear response modulation. We discuss the evidence-based pharmacotherapy and psychotherapy treatment approaches for PTSD.
Discussion: In view of the prevalence and economic burden associated with PTSD, further investigation is warranted into novel treatment approaches based on our knowledge of the involvement of brain circuitry and the role of the amygdala in PTSD, as well as the potential added value of combined pharmacotherapy and psychotherapy to better manage PTSD symptoms.
Post-traumatic stress disorder (PTSD) is a psychiatric condition characterized by a history of exposure to a life-threatening or sexually traumatic event leading to re-experiencing, avoidance, negative thoughts, and hyperarousal symptoms resulting in occupational and social function impairments (1). The development of novel and efficacious pharmacotherapies for this disorder represents an important unmet clinical need, as no new drugs have been approved by the US Food and Drug Administration (FDA) for PTSD treatment since sertraline (1999) and paroxetine (2000) (2–4). Approximately 58% of PTSD clinical trial participants still meet diagnostic criteria following cognitive behavioral therapy and only 32%–66% achieve a sufficient level of functioning (5, 6). In a meta-analysis of 66 randomized controlled trials assessing PTSD medication efficacy, 42% of SSRI-treated patients did not demonstrate symptom improvement, and varying efficacy and tolerability of current medications suggests further research is warranted (7). Despite remarkable progress in our understanding of the neurobiology of PTSD over the past 15 years (8), further investigation of the biological correlates and pathological mechanisms of PTSD symptoms are required to enhance diagnosis and treatment (9). Thus, considering PTSD prevalence and the significant economic and clinical burden associated with the disorder at a societal and individual level, uncovering the psychosocial and biological correlates of PTSD is essential in assessing the current treatment landscape and facilitating emerging therapy development.
Diagnosis of PTSD is based on exposure to actual or threatened death, serious injury, or sexual violence, according to the Diagnostic and Statistical Manual of Mental Disorders 5th Edition (DSM-5) (1). Traumatic exposures can vary and confer diverse levels of risk for PTSD depending on their nature and severity (10). Individuals with PTSD experience symptoms in 4 domains: intrusive re-experiencing, persistent avoidance behaviors, emotional and cognitive disturbances, and hyperarousal and reactivity, including difficulty sleeping and concentrating (1, 4, 11). Although remission can occur within months of onset in some cases, symptoms typically last for many years (10).
A systematic literature review of PTSD prevalence data in the United States from 2015 to 2019 reported that the 1-year prevalence of PTSD among the civilian population ranged from 2.3% to 9.1%, with a lifetime prevalence of 3.4%–26.9%, while for the military/veteran population, rates were higher at 6.7%–50.2% and 7.7%–17.0%, respectively (12). The large prevalence variation in military groups was attributed to heterogeneity in study design and study population (12). Despite increased prevalence, the military/veteran population only accounts for a small proportion of the PTSD population. There are double the number of men and 25 times the number of women with PTSD in the civilian population by comparison, highlighting that PTSD is not exclusive to veterans/military service members (13). Women in the US tend to have higher lifetime PTSD prevalence rates than men (8%–13% for women, 4%–6% for men), and 2018 estimates calculated that women accounted for 66.4% of the overall PTSD population in the US (12, 13). The societal impact of PTSD in 2018 was calculated to have an economic burden of $232.2 billion in the US, with costs of ~$20,000 per individual living with PTSD (13). A quality assessment of recent economic burden and cost-of-illness in PTSD studies reported that cost varied according to the type and severity of the associated trauma; sexual assault in women was associated with a high economic burden (14). As PTSD is underdiagnosed and undertreated (12, 13), its true economic burden likely exceeds these estimates.
Although up to 84% of the general population will experience a traumatic event (15), only a fraction develop PTSD, indicating that PTSD susceptibility varies depending on risk and resiliency factors including the nature and severity of the aversive event, sex, inter-individual differences in coping styles and genetics, and the neurodevelopmental period in which the trauma was experienced (16–20). The specific biological and environmental factors that determine susceptibility and resilience to PTSD pathology remain unclear (9), necessitating better understanding of the neurobiological processes that contribute to PTSD pathobiology.
The amygdala complex is situated in the temporal lobe of the brain and comprises several subcortical nuclei (21–23). It is has 2 main regions: the primitive centrocorticomedial complex and the basolateral complex (BLA) (21, 23). Functions of the amygdala include encoding fearful stimuli, allowing the brain to direct behavioral responses upon encountering such stimuli, as well as playing a role in fear generalization, arousal, and reward processing; thus, it has been widely implicated in PTSD pathophysiology (21, 24, 25).
The amygdala controls both innate fear, and acquired/learned fear, with the latter linked to escape or avoidance behaviors to previous noxious stimuli (22) which are associated with functional changes in the BLA (26). Considering the important role of these amygdala nuclei in fear conditioning, it follows that morphological and functional changes in these regions may explain the reinforcement of memories related to traumatic events in PTSD (27). Reports indicate that morphological and functional changes in the amygdala are evident, resulting in disrupted control of fear generalization, arousal, and reward processing in individuals with PTSD (21, 24, 25, 28, 29). Specifically, decreases in amygdala nuclei volumes have been reported to be associated with symptoms of PTSD (21, 24, 29). Reduced grey matter volume in limbic brain regions including the amygdala are correlated with sleep disturbances (insomnia and nightmares) in patients with PTSD (30), which may contribute to symptom severity (31, 32). Analysis of links between BLA and centrocorticomedial complex volumes with PTSD symptom severity in 47 young survivors from the 2011 Norwegian terror attack revealed an inverse relationship between symptom severity and amygdala nuclei volume 24–36 months post-trauma (21), supporting associations between these factors and PTSD. The conflicting reports of increased amygdala activity and reduced volumetric data is consistent with previous findings in PTSD (33), and may be partly explained by a lack of distinction between regional changes in specific amygdala nuclei and those of the whole amygdala (21). A recent scoping review of structural neuroimaging studies examining hippocampus and amygdala subregions in adults with PTSD reported that only 5 of the 21 eligible studies focused on volume changes in the amygdala, highlighting the anatomical complexity and methodological challenges of establishing structural changes in this brain region (34). Functional neuroimaging, alternatively, may hold greater promise in understanding the role of the amygdala in PTSD pathophysiology (35). Abnormal functional connectivity between the amygdala and other parts of the brain has been demonstrated in PTSD, with stronger connectivity between subregions of the amygdala, particularly the BLA, spanning to the prefrontal cortex (PFC) compared with healthy controls (25, 28, 35). The amygdala communicates the salience of the threat cues to the PFC, which in turn regulates emotional and behavioral fear responses to provide top-down emotional control (36). It is not surprising, therefore, that such connectivity is altered in a condition associated with enhanced fear responses. Furthermore, white matter changes in the ventromedial PFC that negatively correlated with symptom severity have been demonstrated in individuals with PTSD within 2 days of experiencing traffic-accident-induced trauma (37). Further support for BLA involvement in the regulation of fear memories and responses is provided by animal studies demonstrating that BLA neurons trigger hippocampal neurogenesis in fearful contexts (38), and also play an essential role in reinstating fear response to a previously extinguished fear memory (39); patients with PTSD often have spontaneous and regular traumatic memory retrieval (40).
PTSD symptoms develop not only from associative learning such as fear conditioning, where trauma-related stimuli trigger a fear response, but also from non-associative learning processes such as habituation or sensitization to aversive cues (27). These non-associative processes involve incremental escalations of defensive fear responses to aversive or harmful stimuli that can be responsible for pain (41). Individuals with PTSD are believed to be more sensitive to stimuli, to over-generalize fearful stimuli or events, and are unable to extinguish learned or conditioned fear (11). Sensitization to neutral stimuli is evident in patients with PTSD, for example, where the traumatic experience becomes associated with a previously neutral stimulus that has now acquired a threatening meaning (42).
Inflammatory biomarkers including cytokines and C-reactive protein have shown to positively correlate with anxiety symptoms and negatively correlate to functional connectivity between the lower amygdala and ventromedial PFC in women with anxiety and PTSD (43). This association between functional changes in amygdala-PFC connectivity and stressor-induced inflammation is also evident in healthy females that demonstrated greater neural activity in the left amygdala in response to negative social feedback that correlated with greater changes in IL-6 (44). Given that elevated serum levels of pro-inflammatory cytokines are commonly reported in individuals with PTSD (45), and that cytokines can cross the blood-brain barrier (46, 47), it is possible that trauma-induced inflammation may contribute to pathophysiological changes in stress-related brain areas such as the amygdala (48). These findings are well supported by clinical studies, which have shown an association between inflammation and PTSD symptoms (45). Animal studies have also shown that heightened inflammation impairs extinction of fear memory (49, 50), and administration of the interleukin-6 (IL-6) cytokines to the BLA prior to extinction training impairs fear extinction memory (51), suggesting inflammation can alter amygdala fear regulation.
Central to the brain circuitry underlying fear memory processing in disorders such as PTSD is the lateral amygdala, which is the input for stimuli during fear conditioning in animals (11, 22, 52, 53). Therefore, impairment of fear or extinction responses in individuals with PTSD may be indicative of diminished synaptic plasticity, particularly in pathways associated with the lateral amygdala where sensory input is initially processed (22, 52, 54, 55). This early synaptic plasticity in response to fear stimuli in the lateral amygdala is essential in signaling the presence of danger to other connected regions such as the PFC, hippocampus, and hypothalamus so that defensive behavioral and physiological stress responses can be readied for the fight/flight reaction (27, 56). Overall, the role of the amygdala in fear responses, and the dysfunctional activity and connectivity of this brain region in the fear conditioning response and impaired fear extinction in PTSD, is strongly supported (27, 36, 56).
To date, 7 types of transient receptor potential canonical (TRPC) ion channels have been identified (57, 58). TRPC channels are distributed in several brain areas, particularly the amygdala and hippocampus (22, 59), and are reported to be moderately expressed in rodent PFCs (60). TRPC channel activation leads to an influx of Na+ and Ca2+ ions causing both membrane depolarization and elevation of intracellular Ca2+, impacting significantly on cellular function and intracellular signaling (58). The diverse structural properties and distribution of the various TRPC channel types determine the different functions associated with their activation, but they can be broadly described as cellular sensors, due to their regulation of intracellular calcium (58). As they have diverse biological roles, TRPCs are considered potential drug targets for a broad range of disorders such as respiratory disorders, diabetes, cancer, as well as neurological and psychiatric diseases (61).
In the limbic regions, TRPC1, TRPC4, and TRPC5 are the most common, primarily within hippocampal pyramidal cells and the amygdala (62–64). TRPC4 and TRPC5 are homologous proteins that form ion channels involved in the regulation of neuronal growth, axon guidance, synaptic plasticity, and cellular excitability (60). Impairments in these cellular processes contribute to important brain functions, including working memory, hippocampal dysfunctions such as seizures, and fear responses (62, 64, 65). TRPC knockout mice (TRPC5−/−) exhibited diminished fear-related behaviors, indicating that TRPC5 plays a key role in fear responses in mice (22). A similar study in TRPC4−/− mice demonstrated diminished anxiety or innate fear relative to wild-type mice (59). Interestingly, these anxiolytic-like behaviors in TRPC4−/− mice were only present in stressful test conditions (bright versus dim lighting) suggesting the TRPC4 effects are more readily revealed in anxiety-provoking situations (59), a desirable effect in the treatment of PTSD. However, no differences in learned or conditioned fear responses were observed in these knockouts (59). A link between TRPC subtypes and neuroinflammation is also evident from animal studies. Transgenic mice with IL-10 deficiency in microglial cells that express elevated levels of pro-inflammatory cytokines also exhibited decreased TRPC4 and TRPC5 in the hippocampus, and decreased TRPC5 in the PFC and amygdala when compared to wild-type control (66, 67), suggesting a link between TRPC and neuroinflammation in stress-related brain areas. Considering the evidence implicating amygdala involvement in PTSD symptoms (24), the location of both TRPC4 and TRPC5 in the amygdala (22, 59, 60) and their link with local neuroinflammation (66, 67), in addition to the reduced fear and anxiety demonstrated in knockout mice (TRPC4−/− rodents and TRPC5−/− rodents) (22, 59), it is reasonable to hypothesize that dampening amygdala activation of the fear response by blocking these ion channels may reduce PTSD symptom severity.
Currently, both psychological and pharmacologic strategies are employed for PTSD management (1). Recommended forms of PTSD treatment include psychological strategies, shared decision-making, collaborative care, and trauma-focused therapy; both shared decision-making and collaborative care involve reviewing the relevant diagnosis, available treatment options, and use of decision aids to improve clinical outcomes (1). Manualized, trauma-focused therapies such as prolonged exposure therapy, eye movement desensitization and restructuring, cognitive processing therapy, and cognitive behavioral therapy have also been shown to reduce PTSD symptoms (1). Cognitive behavioral therapy is also effective in alleviating PTSD-associated insomnia (68). The efficacy of psychotherapies is strongly supported by current literature; however, access is often limited (69). Since the COVID-19 pandemic, internet-based delivery of treatments has increased and offers potential avenues to widen access and reduce costs (70). Challenges identified in clinical studies examining efficacy of these therapies include issues with engagement and early drop-out rates (71), which may be improved by facilitating access to technology-based delivery or with combined efficacious pharmacotherapy (70, 72). In recent years, non-invasive brain stimulation techniques, including repetitive transcranial magnetic stimulation (rTMS) and transcranial direct current stimulation (tDCS), have demonstrated benefits in PTSD treatment (73–76). Whereas tDCS involves introducing low-intensity electric currents through the skull, rTMS involves repeated and rapidly changing electric currents on the surface of the skull. Both rTMS and tDCS have been shown to modulate cortical excitability in the brain (76, 77), and can improve PTSD symptoms, either as a monotherapy or as a treatment enhancement strategy with minimal side effects (73, 74, 78). However, data relating to treatment effects in PTSD are limited, and studies to clarify the exact mechanisms of action of tDCS and rTMS are warranted (75, 78).
Current clinical guidelines recommend treatment with serotonergic antidepressants; sertraline, fluoxetine, venlafaxine, or paroxetine as monotherapy have shown the greatest benefit with 58% of SSRI-treated patients exhibiting symptom improvement across clinical trials compared with 35% of placebo-treated participants (1, 7). For many SSRI drugs, effects in the amygdala have been demonstrated (Table 1). However, only sertraline and paroxetine are currently approved by the FDA for PTSD. Other pharmacological treatments have also been investigated for treating sleep disturbances in PTSD and include adrenergic inhibiting agents (prazosin, doxazosin), clonidine, tricyclic antidepressants, serotonin and alpha-adrenoceptor antagonists (trazodone, nefazodone, mirtazapine, cyclobenzaprine), atypical antipsychotics, gamma-aminobutyric acid modulators (gabapentin, topiramate), and cyproheptadine (115, 116). Additionally, while combined pharmacotherapy and psychotherapy approaches are recommended, randomized controlled trials have rarely shown them to be more effective than monotherapy. Therefore, additional studies of novel medications for use as monotherapy or in combination with psychotherapy to manage PTSD symptoms are warranted. A summary of candidate treatments for PTSD, detailing evidence of efficacy from clinical trials, and effects on the amygdala are given in Table 1.
Table 1 A summary of candidate PTSD treatment effects in the amygdalaa.
Experimental adjunct treatments such as 3,4-methylenedioxymethamphetamine (MDMA), ketamine and psychedelics, for example psilocybin, have also been proposed for treating PTSD (117, 118). Recent randomized controlled trials in patients with chronic PTSD demonstrated that ketamine, an antagonist of the glutamate N-methyl-D-aspartate receptor, administered intravenously as either single or six repeated infusions over a 2-week period, resulted in clinically/significant improvements in PTSD symptoms compared with midazolam (98, 99). The benefits of ketamine in patients with PTSD and comorbid chronic pain or major depressive disorder (MDD) have also been demonstrated with comparable improvement in both PTSD and co-morbid symptoms (100, 101). However, a more recent study reported no significant effect of repeated ketamine administration over 4 weeks on PTSD symptoms (102). Similarly, varied results of ketamine on anxiety-like behaviors have been reported in animal models (119–122). Nevertheless, the magnitude of the effect on PTSD symptoms across other clinical trials, and the fact that esketamine is already FDA-approved for treating depression, suggest that the potential for ketamine as an alternative to SSRI treatments, or administered alongside psychotherapy, warrants further investigation in PTSD (118, 123). MDMA-assisted psychotherapy is currently in FDA Phase III clinical trials and is considered to facilitate psychotherapy by decreasing amygdala activation during therapeutic sessions, allowing participants to revisit distressing memories in a state of emotional security. Nevertheless, MDMA treatment has risks such as serotonergic depletion that can induce temporary states of anhedonia, lethargy, irritability, depression, anxiety, altered pain thresholds, and sleep disturbance, particularly in females (72, 124). Psychedelics and MDMA induce profound changes in perception, mood, and experiences of time and/or space, necessitating therapists to remain with patients while processing the experience and until the psychomimetic effects subside. As access to these treatments may be unavailable and/or cost prohibitive, newer treatments without such side effects or therapeutic burden are urgently needed.
In a Phase I clinical trial, BI 1358894 was well tolerated at doses of ≤200 mg, with a favorable pharmacokinetic (PK) profile in healthy Caucasian volunteers (125). Typically, TRPC4 and TRPC5 channels remain closed when not activated, which blocks calcium from entering the axon terminal, preventing the transmission of a neuronal signal and a physiological response (126, 127). When a stimulus is detected, TRPC4 and TRPC5 channels open, calcium enters the axon terminal and neuronal signals are transmitted, generating a physiological response (62, 126–128). Therefore, TRPC4/5 inhibition by BI 1358894 should reduce channel activation, preventing calcium influx, thereby dampening the related physiological responses (Figure 1).
Figure 1 TRPC 4/5 inhibitor mechanism of action. Inhibition of TRPC 4/5 prevents influx of cations including calcium (1) and reduces neuronal activity of the amygdala (2). Reduced amygdala activity normalizes cortico-limbic system signaling, improving behaviors associated with dysfunctional emotional processing (3). These effects are mediated through modulation of biological processes (4) that impact on various brain functions of the amygdala (5). TRPC, transient receptor potential canonical ion channel.
Further study of BI 1358894 in 73 patients with MDD treated with BI 1358894 100 mg, citalopram 20 mg, or a matched placebo tablet, found reduced activation in several brain regions involved in emotional processing on fMRI, including the amygdala in those treated with BI 1358894 (97). BI 1358894 induced signal reduction in bilateral amygdala, the left anterior insula, the anterior cingulate cortex, and the left dorsolateral PFC in response to negative faces or scenes, whereas the attenuating effect of citalopram was limited to right amygdala activity in response to negative facial expressions (97). This agent may be beneficial in the reduction of PTSD symptoms. Given the association between the amygdala and PTSD, modulating the amygdala response with a pharmacologic agent could lead to improved compliance and completion of trauma-focused psychotherapy, thus providing greater PTSD symptomology improvements.
Despite recent innovations in both psychological and pharmacologic interventions for PTSD, an unmet clinical need to improve treatment strategies remains (1). Given its significant prevalence and economic burden, novel treatment approaches are urgently needed to better manage symptoms.
LD: Conceptualization, Writing – review & editing. MH: Conceptualization, Writing – review & editing.
The author(s) declare financial support was received for the research, authorship, and/or publication of this article. Writing, editorial support, and formatting assistance were provided by Lieve Desbonnet, PhD, and Sharon Bryant, PhD, Fishawack Communications Ltd, part of Avalere Health, which was contracted and funded by Boehringer Ingelheim Pharmaceuticals Inc. (BIPI).
The authors meet the criteria for authorship as recommended by the International Committee of Medical Journal Editors. The authors did not receive payment related to the development of the manuscript.
MBH has received funding from Boehringer Ingelheim Pharmaceuticals Inc. (BIPI), the US Department of Defense and the US Department of Veteran Affairs. In the past 2 years, LLD has received consulting fees from Otsuka, Signant Health, and Boehringer Ingelheim; research funding or materials from the US Department of Veteran Affairs, Alkermes, Aptinyx, Tonix, US Department of Defense, Social Finance, and Westat; and honorarium from Clinical Care Options.
BIPI was given the opportunity to review the manuscript for medical and scientific accuracy as well as intellectual property considerations. To ensure independent interpretation of clinical study results and enable authors to fulfil their role and obligations under the ICMJE criteria, Boehringer Ingelheim grants all external authors access to clinical study data pertinent to the development of the publication.
All claims expressed in this article are solely those of the authors and do not necessarily represent those of their affiliated organizations, or those of the publisher, the editors and the reviewers. Any product that may be evaluated in this article, or claim that may be made by its manufacturer, is not guaranteed or endorsed by the publisher.
1. Schrader C, Ross A. A review of PTSD and current treatment strategies. Mo Med. (2021) 118:546–51.
2. Ehret M. Treatment of posttraumatic stress disorder: Focus on pharmacotherapy. Ment Health Clin. (2019) 9:373–82. doi: 10.9740/mhc.2019.11.373
3. FDA. (1991). Available online at: https://www.accessdata.fda.gov/drugsatfda_docs/label/2016/019839S74S86S87_20990S35S44S45lbl.pdf.
4. CenterWatch w. Paxil, Paxil CR (paroxetine hydrochloride) [CentreWatch database for clinical trial information] . Available online at: https://cms.centerwatch.com/directories/1067-fda-approved-drugs/listing/3992-paxil-paxil-cr-paroxetine-hydrochloride.
5. Morgan L. MDMA-assisted psychotherapy for people diagnosed with treatment-resistant PTSD: what it is and what it isn't. Ann Gen Psychiatry. (2020) 19:33. doi: 10.1186/s12991-020-00283-6
6. Schnyder U. Why new psychotherapies for posttraumatic stress disorder? Psychother Psychosom. (2005) 74:199–201. doi: 10.1159/000085142
7. Williams T, Phillips NJ, Stein DJ, Ipser JC. Pharmacotherapy for post traumatic stress disorder (PTSD). Cochrane Database Syst Rev. (2022) 3:Cd002795. doi: 10.1002/14651858.CD002795.pub3
8. Fenster RJ, Lebois LAM, Ressler KJ, Suh J. Brain circuit dysfunction in post-traumatic stress disorder: from mouse to man. Nat Rev Neurosci. (2018) 19:535–51. doi: 10.1038/s41583-018-0039-7
9. DePierro J, Lepow L, Feder A, Yehuda R. Translating molecular and neuroendocrine findings in posttraumatic stress disorder and resilience to novel therapies. Biol Psychiatry. (2019) 86:454–63. doi: 10.1016/j.biopsych.2019.07.009
10. Kessler RC, Aguilar-Gaxiola S, Alonso J, Benjet C, Bromet EJ, Cardoso G, et al. Trauma and PTSD in the WHO world mental health surveys. Eur J Psychotraumatol. (2017) 8:1353383. doi: 10.1080/20008198.2017.1353383
11. Mahan AL, Ressler KJ. Fear conditioning, synaptic plasticity and the amygdala: implications for posttraumatic stress disorder. Trends Neurosci. (2012) 35:24–35. doi: 10.1016/j.tins.2011.06.007
12. Schein J, Houle C, Urganus A, Cloutier M, Patterson-Lomba O, Wang Y, et al. Prevalence of post-traumatic stress disorder in the United States: a systematic literature review. Curr Med Res Opin. (2021) 37:2151–61. doi: 10.1080/03007995.2021.1978417
13. Davis LL, Schein J, Cloutier M, Gagnon-Sanschagrin P, Maitland J, Urganus A, et al. The economic burden of posttraumatic stress disorder in the United States from a societal perspective. J Clin Psychiatry. (2022) 83:21m14116. doi: 10.4088/JCP.21m14116
14. von der Warth R, Dams J, Grochtdreis T, König HH. Economic evaluations and cost analyses in posttraumatic stress disorder: a systematic review. Eur J Psychotraumatol. (2020) 11:1753940. doi: 10.1080/20008198.2020.1753940
15. Horn SR, Charney DS, Feder A. Understanding resilience: New approaches for preventing and treating PTSD. Exp Neurol. (2016) 284:119–32. doi: 10.1016/j.expneurol.2016.07.002
16. Nievergelt CM, Maihofer AX, Klengel T, Atkinson EG, Chen CY, Choi KW, et al. International meta-analysis of PTSD genome-wide association studies identifies sex- and ancestry-specific genetic risk loci. Nat Commun. (2019) 10:4558. doi: 10.1038/s41467-019-12576-w
17. Hodes GE, Epperson CN. Sex differences in vulnerability and resilience to stress across the life span. Biol Psychiatry. (2019) 86:421–32. doi: 10.1016/j.biopsych.2019.04.028
18. Adams J, Mrug S, Knight DC. Characteristics of child physical and sexual abuse as predictors of psychopathology. Child Abuse Negl. (2018) 86:167–77. doi: 10.1016/j.chiabu.2018.09.019
19. Wild J, Smith KV, Thompson E, Béar F, Lommen MJ, Ehlers A. A prospective study of pre-trauma risk factors for post-traumatic stress disorder and depression. Psychol Med. (2016) 46:2571–82. doi: 10.1017/S0033291716000532
20. Ebrahimi R, dennis p, Shroyer AL, Alvarez C, beckham j, Sumner J. Abstract 12929: Association of posttraumatic stress disorder with stroke in women veterans. Circulation. (2022) 146:A12929–A. doi: 10.1161/circ.146.suppl_1.12929
21. Ousdal OT, Milde AM, Hafstad GS, Hodneland E, Dyb G, Craven AR, et al. The association of PTSD symptom severity with amygdala nuclei volumes in traumatized youths. Trans Psychiatry. (2020) 10:288. doi: 10.1038/s41398-020-00974-4
22. Riccio A, Li Y, Moon J, Kim KS, Smith KS, Rudolph U, et al. Essential role for TRPC5 in amygdala function and fear-related behavior. Cell. (2009) 137:761–72. doi: 10.1016/j.cell.2009.03.039
24. Morey RA, Gold AL, LaBar KS, Beall SK, Brown VM, Haswell CC, et al. Amygdala volume changes in posttraumatic stress disorder in a large case-controlled veterans group. Arch Gen Psychiatry. (2012) 69:1169–78. doi: 10.1001/archgenpsychiatry.2012.50
25. Brown VM, LaBar KS, Haswell CC, Gold AL, McCarthy G, Morey RA. Altered resting-state functional connectivity of basolateral and centromedial amygdala complexes in posttraumatic stress disorder. Neuropsychopharmacology. (2014) 39:351–9. doi: 10.1038/npp.2013.197
26. Sah P. Fear, anxiety, and the amygdala. Neuron. (2017) 96:1–2. doi: 10.1016/j.neuron.2017.09.013
27. Careaga MBL, Girardi CEN, Suchecki D. Understanding posttraumatic stress disorder through fear conditioning, extinction and reconsolidation. Neurosci Biobehav Rev. (2016) 71:48–57. doi: 10.1016/j.neubiorev.2016.08.023
28. Liu T, Ke J, Qi R, Zhang L, Zhang Z, Xu Q, et al. Altered functional connectivity of the amygdala and its subregions in typhoon-related post-traumatic stress disorder. Brain Behav. (2021) 11:e01952. doi: 10.1002/brb3.1952
29. Del Casale A, Ferracuti S, Barbetti AS, Bargagna P, Zega P, Iannuccelli A, et al. Grey matter volume reductions of the left hippocampus and amygdala in PTSD: A coordinate-based meta-analysis of magnetic resonance imaging studies. Neuropsychobiology. (2022) 81:257–64. doi: 10.1159/000522003
30. Nardo D, Högberg G, Jonsson C, Jacobsson H, Hällström T, Pagani M. Neurobiology of sleep disturbances in PTSD patients and traumatized controls: MRI and SPECT findings. Front Psychiatry. (2015) 6:134. doi: 10.3389/fpsyt.2015.00134
31. Dolan M, Slavish DC, Weiss NH, Contractor AA. The role of emotion dysregulation in the relationship between sleep disturbances and PTSD symptom severity. J Nerv Ment Dis. (2023) 211:203–15. doi: 10.1097/NMD.0000000000001631
32. Schenker MT, Theoswin PM, Qian H, Jordan AS, Nicholas CL, Felmingham KL. Sleep and day-to-day PTSD symptom variability: an ecological momentary assessment and actigraphy monitored study in trauma-exposed young adults. Eur J Psychotraumatol. (2023) 14:2202058. doi: 10.1080/20008066.2023.2202058
33. Pietrzak RH, Averill LA, Abdallah CG, Neumeister A, Krystal JH, Levy I, et al. Amygdala-hippocampal volume and the phenotypic heterogeneity of posttraumatic stress disorder: a cross-sectional study. JAMA Psychiatry. (2015) 72:396–8. doi: 10.1001/jamapsychiatry.2014.2470
34. Ben-Zion Z, Korem N, Fine NB, Katz S, Siddhanta M, Funaro MC, et al. Structural neuroimaging of hippocampus and amygdala subregions in posttraumatic stress disorder: A scoping review. Biol Psychiatry Glob Open Sci. (2024) 4:120–34. doi: 10.1016/j.bpsgos.2023.07.001
35. Haris EM, Bryant RA, Williamson T, Korgaonkar MS. Functional connectivity of amygdala subnuclei in PTSD: a narrative review. Mol Psychiatry. (2023) 28:3581–94. doi: 10.1038/s41380-023-02291-w
36. Kredlow AM, Fenster RJ, Laurent ES, Ressler KJ, Phelps EA. Prefrontal cortex, amygdala, and threat processing: implications for PTSD. Neuropsychopharmacology. (2022) 47:247–59. doi: 10.1038/s41386-021-01155-7
37. Sun Y, Wang Z, Ding W, Wan J, Zhuang Z, Zhang Y, et al. Alterations in white matter microstructure as vulnerability factors and acquired signs of traffic accident-induced PTSD. PloS One. (2013) 8:e83473. doi: 10.1371/journal.pone.0083473
38. Kirby ED, Friedman AR, Covarrubias D, Ying C, Sun WG, Goosens KA, et al. Basolateral amygdala regulation of adult hippocampal neurogenesis and fear-related activation of newborn neurons. Mol Psychiatry. (2012) 17:527–36. doi: 10.1038/mp.2011.71
39. Lin HC, Tseng YC, Mao SC, Chen PS, Gean PW. GABAA receptor endocytosis in the basolateral amygdala is critical to the reinstatement of fear memory measured by fear-potentiated startle. J Neurosci. (2011) 31:8851–61. doi: 10.1523/JNEUROSCI.0979-11.2011
40. Kida S. Reconsolidation/destabilization, extinction and forgetting of fear memory as therapeutic targets for PTSD. Psychopharmacol (Berl). (2019) 236:49–57. doi: 10.1007/s00213-018-5086-2
41. Rahn EJ, Guzman-Karlsson MC, David Sweatt J. Cellular, molecular, and epigenetic mechanisms in non-associative conditioning: implications for pain and memory. Neurobiol Learn Mem. (2013) 105:133–50. doi: 10.1016/j.nlm.2013.06.008
42. Michael T, Ehlers A. Enhanced perceptual priming for neutral stimuli occurring in a traumatic context: two experimental investigations. Behav Res Ther. (2007) 45:341–58. doi: 10.1016/j.brat.2006.03.012
43. Mehta ND, Stevens JS, Li Z, Fani N, Gillespie CF, Ravi M, et al. Inflammation, amygdala-ventromedial prefrontal functional connectivity and symptoms of anxiety and PTSD in African American women recruited from an inner-city hospital: Preliminary results. Brain Behav Immun. (2022) 105:122–30. doi: 10.1016/j.bbi.2022.06.013
44. Muscatell KA, Dedovic K, Slavich GM, Jarcho MR, Breen EC, Bower JE, et al. Greater amygdala activity and dorsomedial prefrontal-amygdala coupling are associated with enhanced inflammatory responses to stress. Brain Behav Immun. (2015) 43:46–53. doi: 10.1016/j.bbi.2014.06.201
45. Hori H, Kim Y. Inflammation and post-traumatic stress disorder. Psychiatry Clin Neurosci. (2019) 73:143–53. doi: 10.1111/pcn.12820
46. Banks WA, Kastin AJ, Broadwell RD. Passage of cytokines across the blood-brain barrier. Neuroimmunomodulation. (1995) 2:241–8. doi: 10.1159/000097202
47. Banks WA. The blood-brain barrier in neuroimmunology: Tales of separation and assimilation. Brain Behav Immun. (2015) 44:1–8. doi: 10.1016/j.bbi.2014.08.007
48. Kim TD, Lee S, Yoon S. Inflammation in post-traumatic stress disorder (PTSD): A review of potential correlates of PTSD with a neurological perspective. Antioxidants (Basel Switzerland). (2020) 9:107. doi: 10.3390/antiox9020107
49. Young MB, Howell LL, Hopkins L, Moshfegh C, Yu Z, Clubb L, et al. A peripheral immune response to remembering trauma contributes to the maintenance of fear memory in mice. Psychoneuroendocrinology. (2018) 94:143–51. doi: 10.1016/j.psyneuen.2018.05.012
50. Yu Z, Fukushima H, Ono C, Sakai M, Kasahara Y, Kikuchi Y, et al. Microglial production of TNF-alpha is a key element of sustained fear memory. Brain Behav Immun. (2017) 59:313–21. doi: 10.1016/j.bbi.2016.08.011
51. Hao Y, Jing H, Bi Q, Zhang J, Qin L, Yang P. Intra-amygdala microinfusion of IL-6 impairs the auditory fear conditioning of rats via JAK/STAT activation. Behav Brain Res. (2014) 275:88–95. doi: 10.1016/j.bbr.2014.08.052
52. Blair HT, Schafe GE, Bauer EP, Rodrigues SM, LeDoux JE. Synaptic plasticity in the lateral amygdala: a cellular hypothesis of fear conditioning. Learn Mem. (2001) 8:229–42. doi: 10.1101/lm.30901
53. Parsons RG, Ressler KJ. Implications of memory modulation for post-traumatic stress and fear disorders. Nat Neurosci. (2013) 16:146–53. doi: 10.1038/nn.3296
54. Quirk GJ, Repa C, LeDoux JE. Fear conditioning enhances short-latency auditory responses of lateral amygdala neurons: parallel recordings in the freely behaving rat. Neuron. (1995) 15:1029–39. doi: 10.1016/0896-6273(95)90092-6
55. Tsvetkov E, Carlezon WA, Benes FM, Kandel ER, Bolshakov VY. Fear conditioning occludes LTP-induced presynaptic enhancement of synaptic transmission in the cortical pathway to the lateral amygdala. Neuron. (2002) 34:289–300. doi: 10.1016/S0896-6273(02)00645-1
56. Saha R, Kriebel M, Anunu R, Volkmer H, Richter-Levin G. Intra-amygdala metaplasticity modulation of fear extinction learning. Eur J Neurosci. (2022) 55:2455–63. doi: 10.1111/ejn.15080
57. Strübing C, Krapivinsky G, Krapivinsky L, Clapham DE. Formation of novel TRPC channels by complex subunit interactions in embryonic brain. J Biol Chem. (2003) 278:39014–9. doi: 10.1074/jbc.M306705200
58. Wang H, Cheng X, Tian J, Xiao Y, Tian T, Xu F, et al. TRPC channels: Structure, function, regulation and recent advances in small molecular probes. Pharmacol Ther. (2020) 209:107497. doi: 10.1016/j.pharmthera.2020.107497
59. Riccio A, Li Y, Tsvetkov E, Gapon S, Yao GL, Smith KS, et al. Decreased anxiety-like behavior and Galphaq/11-dependent responses in the amygdala of mice lacking TRPC4 channels. J Neurosci. (2014) 34:3653–67. doi: 10.1523/JNEUROSCI.2274-13.2014
60. Fowler MA, Sidiropoulou K, Ozkan ED, Phillips CW, Cooper DC. Corticolimbic expression of TRPC4 and TRPC5 channels in the rodent brain. PloS One. (2007) 2:e573. doi: 10.1371/journal.pone.0000573
61. Fallah HP, Ahuja E, Lin H, Qi J, He Q, Gao S, et al. A review on the role of TRP channels and their potential as drug targets_an insight into the TRP channel drug discovery methodologies. Front Pharmacol. (2022) 13:914499. doi: 10.3389/fphar.2022.914499
62. Gualdani R, Gailly P. How TRPC channels modulate hippocampal function. Int J Mol Sci. (2020) 21:3915. doi: 10.3390/ijms21113915
63. Chung YH, Sun Ahn H, Kim D, Hoon Shin D, Su Kim S, Yong Kim K, et al. Immunohistochemical study on the distribution of TRPC channels in the rat hippocampus. Brain Res. (2006) 1085:132–7. doi: 10.1016/j.brainres.2006.02.087
64. Bröker-Lai J, Kollewe A, Schindeldecker B, Pohle J, Nguyen Chi V, Mathar I, et al. Heteromeric channels formed by TRPC1, TRPC4 and TRPC5 define hippocampal synaptic transmission and working memory. EMBO J. (2017) 36:2770–89. doi: 10.15252/embj.201696369
65. Zheng F, Phelan KD. The role of canonical transient receptor potential channels in seizure and excitotoxicity. Cells. (2014) 3:288–303. doi: 10.3390/cells3020288
66. Huo S, Ren J, Ma Y, Ozathaley A, Yuan W, Ni H, et al. Upregulation of TRPC5 in hippocampal excitatory synapses improves memory impairment associated with neuroinflammation in microglia knockout IL-10 mice. J Neuroinflammation. (2021) 18:275. doi: 10.1186/s12974-021-02321-w
67. Yang L, Liu C, Li W, Ma Y, Huo S, Ozathaley A, et al. Depression-like behavior associated with E/I imbalance of mPFC and amygdala without TRPC channels in mice of knockout IL-10 from microglia. Brain Behav Immun. (2021) 97:68–78. doi: 10.1016/j.bbi.2021.06.015
68. Hertenstein E, Trinca E, Wunderlin M, Schneider CL, Züst MA, Fehér KD, et al. Cognitive behavioral therapy for insomnia in patients with mental disorders and comorbid insomnia: A systematic review and meta-analysis. Sleep Med Rev. (2022) 62:101597. doi: 10.1016/j.smrv.2022.101597
69. Lewis C, Roberts NP, Andrew M, Starling E, Bisson JI. Psychological therapies for post-traumatic stress disorder in adults: systematic review and meta-analysis. Eur J Psychotraumatol. (2020) 11:1729633. doi: 10.1080/20008198.2020.1729633
70. Lenferink LIM, Meyerbröker K, Boelen PA. PTSD treatment in times of COVID-19: A systematic review of the effects of online EMDR. Psychiatry Res. (2020) 293:113438. doi: 10.1016/j.psychres.2020.113438
71. Bisson JI, Roberts NP, Andrew M, Cooper R, Lewis C. Psychological therapies for chronic post-traumatic stress disorder (PTSD) in adults. Cochrane Database Syst Rev. (2013) 2013:Cd003388. doi: 10.1002/14651858
72. Inouye A, Wolfgang A. 3,4-Methylenedioxymethamphetamine (MDMA)-assisted therapy in Hawaii: A brief review. Cureus. (2022) 14:e26402. doi: 10.7759/cureus.26402
73. Marcolin K, da Cunha  BM, Yoneyama BC, Ribeiro TA. Effects of transcranial direct current stimulation (tDCS) in "Kiss nightclub fire" patients with post-traumatic stress disorder (PTSD): A phase II clinical trial. SAGE Open Med. (2023) 11:20503121231160953. doi: 10.1177/20503121231160953
74. Kan RLD, Zhang BBB, Zhang JJQ, Kranz GS. Non-invasive brain stimulation for posttraumatic stress disorder: a systematic review and meta-analysis. Trans Psychiatry. (2020) 10:168. doi: 10.1038/s41398-020-0851-5
75. Gouveia FV, Davidson B, Meng Y, Gidyk DC, Rabin JS, Ng E, et al. Treating post-traumatic stress disorder with neuromodulation therapies: transcranial magnetic stimulation, transcranial direct current stimulation, and deep brain stimulation. Neurotherapeutics. (2020) 17:1747–56. doi: 10.1007/s13311-020-00871-0
76. Makale MT, Abbasi S, Nybo C, Keifer J, Christman L, Fairchild JK, et al. Personalized repetitive transcranial magnetic stimulation (prtms®) for post-traumatic stress disorder (ptsd) in military combat veterans. Heliyon. (2023) 9:e18943. doi: 10.1016/j.heliyon.2023.e18943
77. Polanía R, Nitsche MA, Paulus W. Modulating functional connectivity patterns and topological functional organization of the human brain with transcranial direct current stimulation. Hum Brain Mapp. (2011) 32:1236–49. doi: 10.1002/hbm.21104
78. Cirillo P, Gold AK, Nardi AE, Ornelas AC, Nierenberg AA, Camprodon J, et al. Transcranial magnetic stimulation in anxiety and trauma-related disorders: A systematic review and meta-analysis. Brain Behav. (2019) 9:e01284. doi: 10.1002/brb3.1284
79. Schultebraucks K, Maslahati T, Wingenfeld K, Hellmann-Regen J, Kraft J, Kownatzki M, et al. Intranasal oxytocin administration impacts the acquisition and consolidation of trauma-associated memories: a double-blind randomized placebo-controlled experimental study in healthy women. Neuropsychopharmacology. (2022) 47:1046–54. doi: 10.1038/s41386-021-01247-4
80. Frijling JL. Preventing PTSD with oxytocin: effects of oxytocin administration on fear neurocircuitry and PTSD symptom development in recently trauma-exposed individuals. Eur J Psychotraumatol. (2017) 8:1302652. doi: 10.1080/20008198.2017.1302652
81. Sippel LM, Flanagan JC, Holtzheimer PE, Moran-Santa-Maria MM, Brady KT, Joseph JE. Effects of intranasal oxytocin on threat- and reward-related functional connectivity in men and women with and without childhood abuse-related PTSD. Psychiatry Res Neuroimaging. (2021) 317:111368. doi: 10.1016/j.pscychresns.2021.111368
82. Koch SB, van Zuiden M, Nawijn L, Frijling JL, Veltman DJ, Olff M. Intranasal oxytocin administration dampens amygdala reactivity towards emotional faces in male and female PTSD patients. Neuropsychopharmacology. (2016) 41:1495–504. doi: 10.1038/npp.2015.299
83. Kreuder AK, Scheele D, Schultz J, Hennig J, Marsh N, Dellert T, et al. Common and dissociable effects of oxytocin and lorazepam on the neurocircuitry of fear. Proc Natl Acad Sci U.S.A. (2020) 117:11781–7. doi: 10.1073/pnas.1920147117
84. Mignot E, Mayleben D, Fietze I, Leger D, Zammit G, Bassetti CLA, et al. Safety and efficacy of daridorexant in patients with insomnia disorder: results from two multicentre, randomised, double-blind, placebo-controlled, phase 3 trials. Lancet Neurol. (2022) 21:125–39. doi: 10.1016/S1474-4422(21)00436-1
85. Martenyi F, Brown EB, Zhang H, Prakash A, Koke SC. Fluoxetine versus placebo in posttraumatic stress disorder. J Clin Psychiatry. (2002) 63:199–206. doi: 10.4088/JCP.v63n0305
86. Gunduz-Cinar O, Flynn S, Brockway E, Kaugars K, Baldi R, Ramikie TS, et al. Fluoxetine facilitates fear extinction through amygdala endocannabinoids. Neuropsychopharmacology. (2016) 41:1598–609. doi: 10.1038/npp.2015.318
87. Yu YH, Ou CY, Shyu BC, Huang ACW. Basolateral amygdala but not medial prefrontal cortex contributes to chronic fluoxetine treatments for PTSD symptoms in mice. Behav Neurol. (2020) 2020:8875087. doi: 10.1155/2020/8875087
88. Ramaswamy S, Driscoll D, Reist C, Smith LM, Albers LJ, Rose J, et al. A double-blind, placebo-controlled randomized trial of vilazodone in the treatment of posttraumatic stress disorder and comorbid depression. Prim Care Companion CNS Disord. (2017) 19:17m02138. doi: 10.4088/PCC.17m02138
89. Marshall RD, Beebe KL, Oldham M, Zaninelli R. Efficacy and safety of paroxetine treatment for chronic PTSD: a fixed-dose, placebo-controlled study. Am J Psychiatry. (2001) 158:1982–8. doi: 10.1176/appi.ajp.158.12.1982
90. Tucker P, Zaninelli R, Yehuda R, Ruggiero L, Dillingham K, Pitts CD. Paroxetine in the treatment of chronic posttraumatic stress disorder: results of a placebo-controlled, flexible-dosage trial. J Clin Psychiatry. (2001) 62:860–8. doi: 10.4088/JCP.v62n1105
91. Szeszko PR, MacMillan S, McMeniman M, Lorch E, Madden R, Ivey J, et al. Amygdala volume reductions in pediatric patients with obsessive-compulsive disorder treated with paroxetine: preliminary findings. Neuropsychopharmacology. (2004) 29:826–32. doi: 10.1038/sj.npp.1300399
92. Brady K, Pearlstein T, Asnis GM, Baker D, Rothbaum B, Sikes CR, et al. Efficacy and safety of sertraline treatment of posttraumatic stress disorder: a randomized controlled trial. JAMA. (2000) 283:1837–44. doi: 10.1001/jama.283.14.1837
93. Davidson JR, Rothbaum BO, van der Kolk BA, Sikes CR, Farfel GM. Multicenter, double-blind comparison of sertraline and placebo in the treatment of posttraumatic stress disorder. Arch Gen Psychiatry. (2001) 58:485–92. doi: 10.1001/archpsyc.58.5.485
94. Zohar J, Amital D, Miodownik C, Kotler M, Bleich A, Lane RM, et al. Double-blind placebo-controlled pilot study of sertraline in military veterans with posttraumatic stress disorder. J Clin Psychopharmacol. (2002) 22:190–5. doi: 10.1097/00004714-200204000-00013
95. Victor TA, Furey ML, Fromm SJ, Ohman A, Drevets WC. Relationship between amygdala responses to masked faces and mood state and treatment in major depressive disorder. Arch Gen Psychiatry. (2010) 67:1128–38. doi: 10.1001/archgenpsychiatry.2010.144
96. Davidson J, Rothbaum BO, Tucker P, Asnis G, Benattia I, Musgnung JJ. Venlafaxine extended release in posttraumatic stress disorder: a sertraline- and placebo-controlled study. J Clin Psychopharmacol. (2006) 26:259–67. doi: 10.1097/01.jcp.0000222514.71390.c1
97. Grimm S, Keicher C, Paret C, Niedtfeld I, Beckmann C, Mennes M, et al. The effects of transient receptor potential cation channel inhibition by BI 1358894 on cortico-limbic brain reactivity to negative emotional stimuli in major depressive disorder. Eur Neuropsychopharmacol. (2022) 65:44–51. doi: 10.1016/j.euroneuro.2022.10.009
98. Feder A, Parides MK, Murrough JW, Perez AM, Morgan JE, Saxena S, et al. Efficacy of intravenous ketamine for treatment of chronic posttraumatic stress disorder: a randomized clinical trial. JAMA Psychiatry. (2014) 71:681–8. doi: 10.1001/jamapsychiatry.2014.62
99. Feder A, Costi S, Rutter SB, Collins AB, Govindarajulu U, Jha MK, et al. A randomized controlled trial of repeated ketamine administration for chronic posttraumatic stress disorder. Am J Psychiatry. (2021) 178:193–202. doi: 10.1176/appi.ajp.2020.20050596
100. Dadabayev AR, Joshi SA, Reda MH, Lake T, Hausman MS, Domino E, et al. Low dose ketamine infusion for comorbid posttraumatic stress disorder and chronic pain: A randomized double-blind clinical trial. Chronic Stress. (2020) 4:2470547020981670. doi: 10.1177/2470547020981670
101. Albott CS, Lim KO, Forbes MK, Erbes C, Tye SJ, Grabowski JG, et al. Efficacy, safety, and durability of repeated ketamine infusions for comorbid posttraumatic stress disorder and treatment-resistant depression. J Clin Psychiatry. (2018) 79:17m11634. doi: 10.4088/JCP.17m11634
102. Abdallah CG, Roache JD, Gueorguieva R, Averill LA, Young-McCaughan S, Shiroma PR, et al. Dose-related effects of ketamine for antidepressant-resistant symptoms of posttraumatic stress disorder in veterans and active duty military: a double-blind, randomized, placebo-controlled multi-center clinical trial. Neuropsychopharmacology. (2022) 47:1574–81. doi: 10.1038/s41386-022-01266-9
103. Loureiro JRA, Leaver A, Vasavada M, Sahib AK, Kubicki A, Joshi S, et al. Modulation of amygdala reactivity following rapidly acting interventions for major depression. Hum Brain Mapp. (2020) 41:1699–710. doi: 10.1002/hbm.24895
104. Mitchell JM, Bogenschutz M, Lilienstein A, Harrison C, Kleiman S, Parker-Guilbert K, et al. MDMA-assisted therapy for severe PTSD: a randomized, double-blind, placebo-controlled phase 3 study. Nat Med. (2021) 27:1025–33. doi: 10.1038/s41591-021-01336-3
105. Oehen P, Traber R, Widmer V, Schnyder U. A randomized, controlled pilot study of MDMA (± 3,4-Methylenedioxymethamphetamine)-assisted psychotherapy for treatment of resistant, chronic Post-Traumatic Stress Disorder (PTSD). J Psychopharmacol. (2013) 27:40–52. doi: 10.1177/0269881112464827
106. Young MB, Andero R, Ressler KJ, Howell LL. 3,4-Methylenedioxymethamphetamine facilitates fear extinction learning. Trans Psychiatry. (2015) 5:e634. doi: 10.1038/tp.2015.138
107. Ross S, Bossis A, Guss J, Agin-Liebes G, Malone T, Cohen B, et al. Rapid and sustained symptom reduction following psilocybin treatment for anxiety and depression in patients with life-threatening cancer: a randomized controlled trial. J Psychopharmacol. (2016) 30:1165–80. doi: 10.1177/0269881116675512
108. Davis AK, Barrett FS, May DG, Cosimano MP, Sepeda ND, Johnson MW, et al. Effects of psilocybin-assisted therapy on major depressive disorder: A randomized clinical trial. JAMA Psychiatry. (2021) 78:481–9. doi: 10.1001/jamapsychiatry.2020.3285
109. Barrett FS, Doss MK, Sepeda ND, Pekar JJ, Griffiths RR. Emotions and brain function are altered up to one month after a single high dose of psilocybin. Sci Rep. (2020) 10:2214. doi: 10.1038/s41598-020-59282-y
110. Roseman L, Demetriou L, Wall MB, Nutt DJ, Carhart-Harris RL. Increased amygdala responses to emotional faces after psilocybin for treatment-resistant depression. Neuropharmacology. (2018) 142:263–9. doi: 10.1016/j.neuropharm.2017.12.041
111. Kraehenmann R, Schmidt A, Friston K, Preller KH, Seifritz E, Vollenweider FX. The mixed serotonin receptor agonist psilocybin reduces threat-induced modulation of amygdala connectivity. NeuroImage Clin. (2016) 11:53–60. doi: 10.1016/j.nicl.2015.08.009
112. Just S, Chenard BL, Ceci A, Strassmaier T, Chong JA, Blair NT, et al. Treatment with HC-070, a potent inhibitor of TRPC4 and TRPC5, leads to anxiolytic and antidepressant effects in mice. PloS One. (2018) 13:e0191225. doi: 10.1371/journal.pone.0191225
113. Kar N. Cognitive behavioral therapy for the treatment of post-traumatic stress disorder: a review. Neuropsychiatr Dis Treat. (2011) 7:167–81. doi: 10.2147/NDT
114. Bryant RA, Felmingham K, Kemp A, Das P, Hughes G, Peduto A, et al. Amygdala and ventral anterior cingulate activation predicts treatment response to cognitive behaviour therapy for post-traumatic stress disorder. Psychol Med. (2008) 38:555–61. doi: 10.1017/S0033291707002231
115. Geldenhuys C, van den Heuvel LL, Steyn P, Seedat S. Pharmacological management of nightmares associated with posttraumatic stress disorder. CNS Drugs. (2022) 36:721–37. doi: 10.1007/s40263-022-00929-x
116. Lipinska G, Baldwin DS, Thomas KG. Pharmacology for sleep disturbance in PTSD. Hum psychopharmacology. (2016) 31:156–63. doi: 10.1002/hup.2522
117. Henner RL, Keshavan MS, Hill KP. Review of potential psychedelic treatments for PTSD. J Neurol Sci. (2022) 439:120302. doi: 10.1016/j.jns.2022.120302
118. Stein MB, Simon NM. Ketamine for PTSD: Well, isn't that special. Am J Psychiatry. (2021) 178:116–8. doi: 10.1176/appi.ajp.2020.20121677
119. Hayase T, Yamamoto Y, Yamamoto K. Behavioral effects of ketamine and toxic interactions with psychostimulants. BMC Neurosci. (2006) 7:25. doi: 10.1186/1471-2202-7-25
120. Li N, Liu RJ, Dwyer JM, Banasr M, Lee B, Son H, et al. Glutamate N-methyl-D-aspartate receptor antagonists rapidly reverse behavioral and synaptic deficits caused by chronic stress exposure. Biol Psychiatry. (2011) 69:754–61. doi: 10.1016/j.biopsych.2010.12.015
121. Sala N, Paoli C, Bonifacino T, Mingardi J, Schiavon E, La Via L, et al. Acute ketamine facilitates fear memory extinction in a rat model of PTSD along with restoring glutamatergic alterations and dendritic atrophy in the prefrontal cortex. Front Pharmacol. (2022) 13:759626. doi: 10.3389/fphar.2022.759626
122. Zhang LM, Zhou WW, Ji YJ, Li Y, Zhao N, Chen HX, et al. Anxiolytic effects of ketamine in animal models of posttraumatic stress disorder. Psychopharmacol (Berl). (2015) 232:663–72. doi: 10.1007/s00213-014-3697-9
123. Pradhan B, Mitrev L, Moaddell R, Wainer IW. d-Serine is a potential biomarker for clinical response in treatment of post-traumatic stress disorder using (R,S)-ketamine infusion and TIMBER psychotherapy: A pilot study. Biochim Biophys Acta Proteins Proteom. (2018) 1866:831–9. doi: 10.1016/j.bbapap.2018.03.006
124. Vizeli P, Liechti ME. Safety pharmacology of acute MDMA administration in healthy subjects. J Psychopharmacol. (2017) 31:576–88. doi: 10.1177/0269881117691569
125. Vestbo J, Papi A, Corradi M, Blazhko V, Montagna I, Francisco C, et al. Single inhaler extrafine triple therapy versus long-acting muscarinic antagonist therapy for chronic obstructive pulmonary disease (TRINITY): a double-blind, parallel group, randomised controlled trial. Lancet. (2017) 389:1919–29. doi: 10.1016/S0140-6736(17)30188-5
127. Kawamoto EM, Vivar C, Camandola S. Physiology and pathology of calcium signaling in the brain. Front Pharmacol. (2012) 3:61. doi: 10.3389/fphar.2012.00061
Keywords: post-traumatic stress disorder, PTSD, amygdala, pathophysiology, pharmacotherapy, transient receptor potential canonical ion channel
Citation: Davis LL and Hamner MB (2024) Post-traumatic stress disorder: the role of the amygdala and potential therapeutic interventions – a review. Front. Psychiatry 15:1356563. doi: 10.3389/fpsyt.2024.1356563
Received: 15 December 2023; Accepted: 16 May 2024;
Published: 06 June 2024.
Edited by:
Min Cai, Fourth Military Medical University, ChinaReviewed by:
Long Li, Chinese Academy of Sciences (CAS), ChinaCopyright © 2024 Davis and Hamner. This is an open-access article distributed under the terms of the Creative Commons Attribution License (CC BY). The use, distribution or reproduction in other forums is permitted, provided the original author(s) and the copyright owner(s) are credited and that the original publication in this journal is cited, in accordance with accepted academic practice. No use, distribution or reproduction is permitted which does not comply with these terms.
*Correspondence: Mark B. Hamner, aGFtbmVybWJAbXVzYy5lZHU=
Disclaimer: All claims expressed in this article are solely those of the authors and do not necessarily represent those of their affiliated organizations, or those of the publisher, the editors and the reviewers. Any product that may be evaluated in this article or claim that may be made by its manufacturer is not guaranteed or endorsed by the publisher.
Research integrity at Frontiers
Learn more about the work of our research integrity team to safeguard the quality of each article we publish.