- 1Key Laboratory for Biorheological Science and Technology of Ministry of Education, National and Local Joint Engineering Laboratory for Vascular Implant, Bioengineering College of Chongqing University, Chongqing, China
- 2Chongqing University Cancer Hospital, Chongqing, China
- 3Chongqing Key Laboratory of Nano/Micro Composite Materials and Devices, Chongqing University of Science and Technology, Chongqing, China
- 4JinFeng Laboratory, Chongqing, China
- 5Plants for Human Health Institute, Department of Food, Bioprocessing and Nutrition Sciences, North Carolina State University, Kannapolis, NC, United States
- 6Department of Biology, The University of Haripur, Haripur, Pakistan
Depression is considered a multifaceted and intricate mental disorder of growing concern due to its significant impact on global health issues. The human gut microbiota, also known as the “second brain,” has an important role in the CNS by regulating it through chemical, immunological, hormonal, and neurological processes. Various studies have found a significant bidirectional link between the brain and the gut, emphasizing the onset of depression therapies. The biological and molecular processes underlying depression and microbiota are required, as the bidirectional association may represent a novel study. However, profound insights into the stratification and diversity of the gut microbiota are still uncommon. This article investigates the emerging evidence of a bacterial relationship between the gut and the brain’s neurological system and its potential pathogenicity and relevance. The interplay of microbiota, immune system, nervous system neurotransmitter synthesis, and neuroplasticity transitions is also widely studied. The consequences of stress, dietary fibers, probiotics, prebiotics, and antibiotics on the GB axis are being studied. Multiple studies revealed the processes underlying this axis and led to the development of effective microbiota-based drugs for both prevention and treatment. Therefore, the results support the hypothesis that gut microbiota influences depression and provide a promising area of research for an improved knowledge of the etiology of the disease and future therapies.
1 Introduction
Depression represents a frequent psychiatric disorder hampered by social stigma, a lack of efficient therapies, and a lack of adequate mental health facilities. It is characterized by persistent poor mood and lasts for at least two weeks formally defined as depression (1–3). Nearly 280 million human beings globally (21%) suffer from depression, although this disorder is commonly misdiagnosed and untreated for a variety of reasons (4). Numerous internal and environmental variables contribute to major depression, which affects millions of people every year (5). It is believed that the 400-1000 bacterial species that live in the gut of humans affect the brain’s nervous system. The human gut which is populated by 1014 microbes ten times more than human cells is an important part of the microbiota ecology (6). A growing amount of interest is being placed on the beneficial relationship between intestinal bacteria and their host that affects cell hormone release, which has an impact on host metabolism and the development of metabolic diseases (7, 8). In developed nations, about one out of every five individuals has experienced a depressive disorder at some point in their lives. Moreover, depressive conditions tend to be more distinct in nearly one-third of economically poor nations (9, 10).
Although the origins of the disease are still unknown, several concepts have been put out to explain the etiology of its underlying mechanisms. Selective serotonin reuptake inhibitors (SSRIs) and serotonin-noradrenaline reuptake inhibitors (SNRIs) are extensively employed as antidepressants. These medications were developed by the monoamine hypothesis, which may be attributed to a deficiency in monoamine neurotransmitters (4, 11). In 2016, the World Health Organization (WHO) provided an estimate of 785,000 suicides taking place annually, resulting in a suicide rate of 10.6 suicides per 100,000 individuals (12). Notably, significant depression may be present in up to 60% of these instances (11). It may be able to expand the range of treatments and preventive therapies for patients suffering from major depression disorder (MDD) by better understanding the role of the gut microbes and the interaction between antidepressants and the axis of the gut-brain. Recent breakthroughs in MDD treatment emphasize the importance of intestinal microbes as a study domain (13, 14). Based on evidence from twin, family, and epidemiological studies, environmental factors and their interplay with hereditary factors have a significant impact on MDD (15, 16). Figure 1 illustrates the efficacy of probiotics, particularly butyrate-producing bacteria, to alleviate the physiological causes of MDD while also structurally modulating the intestinal flora. This treatment method is used to treat MDD disorders (4, 17, 18). New hypotheses regarding the development of depression have emerged, including the changes in the hypothalamus-pituitary-adrenal (HPA) axis, which is associated with the stress response, as well as the dysfunction in neurogenesis and neuroplasticity, which involves brain-derived neurotrophic factors (BDNF) (19).
Several different factors may contribute to depression such as stress, poor nutrition, insufficient exercise, obesity, chronic low-grade inflammation, smoking, atopic diseases, insufficient sleep, poor cleanliness, problems with dental care, and nutritional deficiencies, especially vitamin D insufficiency (20, 21). The study of the therapeutic effects of gut microbes on behavioral and cognitive function will lead to innovations in depression treatment (22). The human microbiome is now well understood by researchers because of significant advances in sequencing technology. This knowledge found the significance of symbiotic microbes in the body, particularly the gut microbiome (GM), for maintaining homeostasis (23, 24). This may contain 10–100 trillion symbiotic microbes, including bacteria, viruses, fungi, protozoans, and archaeal (25), most of these are intestinal bacteria, which belong to the phyla Firmicutes and Bacteroidetes (26, 27). Gut bacteria play a crucial role in maintaining host health through essential metabolic processes, supporting, and developing the immune system, and providing defense against pathogen invasions (28–30). Numerous neurological and psychiatric disorders, including conditions such as autism spectrum disorder, anxiety, and depression, have been associated with dysbiosis.
The Microbiota-Gut-Brain Axis (MGBA) theory has advanced the most current depression hypothesis. (2, 31). The contribution of the MGB axis avenues in depression has become well-established as shown in Figure 2 (32). Depression-related physiological dysbiosis is thought to involve deficient metabolites and neural networks, lower growth of neurons, reduced neural plasticity, and brain inflammation (33–36). Current research indicates that the MGBA exerts either a direct or an indirect influence on MDD via the gut microbiota. This concept acts as a significant impetus for discovering mechanistic studies and exploring microbial biomarkers within the realm of depression (4, 37, 38). Prebiotics facilitate the proliferation of healthy probiotic bacteria and enhance the gut-brain link, have the potential to increase the influence of probiotics on the adult neurometabolism (39). Recent studies suggest that post-biotics might also influence the activity of the Brain-Gut-Microbiota (BGM) axis (40). This review aims to offer a comprehensive perspective on the interplay between colonic microbiota and depressive disorders, with a specific focus on elucidating the association of intestinal microbial relation with depression progression. Moreover, it explores the therapeutic possibilities of probiotics, prebiotics, and related factors in addressing depression.
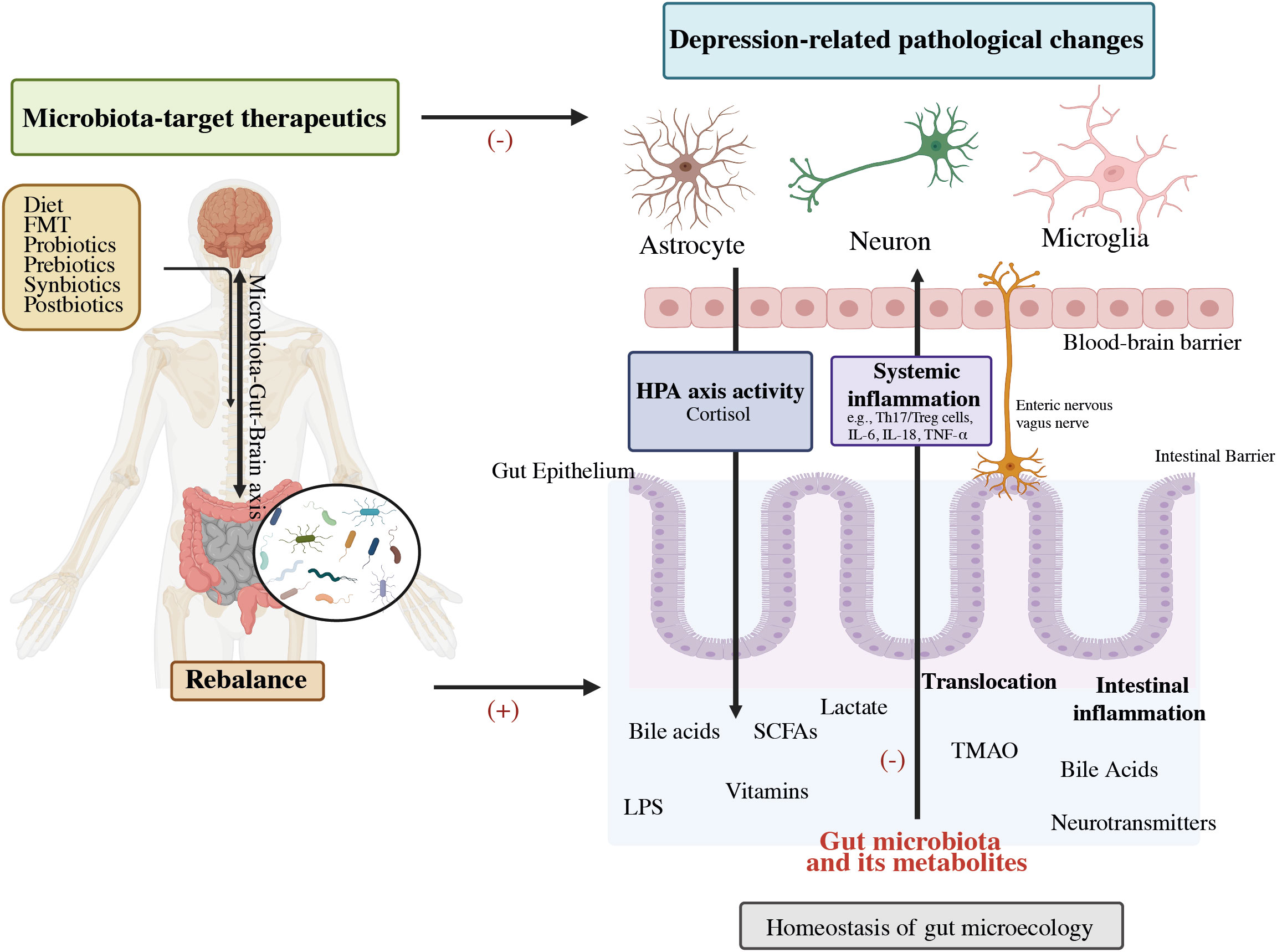
Figure 2 Potential role of the gut-brain-microbiota axis in depression pathophysiology and its therapeutics. FMT, Fecal microbiota transplantation; HPA, Hypothalamic–pituitary–adrenal; SCFAs, Short-chain fatty acids; LPS, Lipopolysaccharide; TMAO, Trimethylamine-N-oxide; IL-6, Interleukin-6; IL-1β, Interleukin-1β; TNF-α, Tumor necrosis factor-alpha (32).
2 Microbiota-gut-brain axis and depression
The MGB axis concept has arisen in the psychiatric field, as to how microbiome contributes to human homeostasis (41). The microbiota-gut-brain axis shows an association between the brain and the billions of bacteria in the intestinal tract (42). The human GI tract contains up to 1014 microbe cells, which comprise the microbiota (43), which is an umbrella term for all microorganisms living on or in the human body (44). About 75% of the microbiome is made up of the two main taxa Bacteroidetes and Firmicutes (45), and Proteobacteria, Actinobacteria, Fusobacteria, and Verrucomicrobia are found in comparatively small amounts (46). Recent investigations have revealed the digestive microbial tract has an enormous impact on human brain processes through the gut-brain axis (47). In particular, MDD patients have an increased Bacteroidetes/Firmicutes ratio, which is considered an improvement of the genus Bacteroides and a depletion of the genus Blautia, Faecalibacterium, and Coprococcus (48–50). Further, Eggerthella levels were consistently higher and Sutterella levels were consistently lower in people with MDD (49, 51). The ability of the microbiota to affect CNS activities bidirectionally through the vagus nervous system (VNS) is mainly mediated by the neurological (ANS), hormonal (HPA axis), and immunological (cytokine and chemokine) processes, all of which are interrelated (52). Exposure to biological contaminants may promote intestinal dysbiosis, which disrupts the GBA’s regular activity and contributes to psychological conditions such as anxiety, stress, depression, and mental disorders as illustrated in Figure 3 (54).
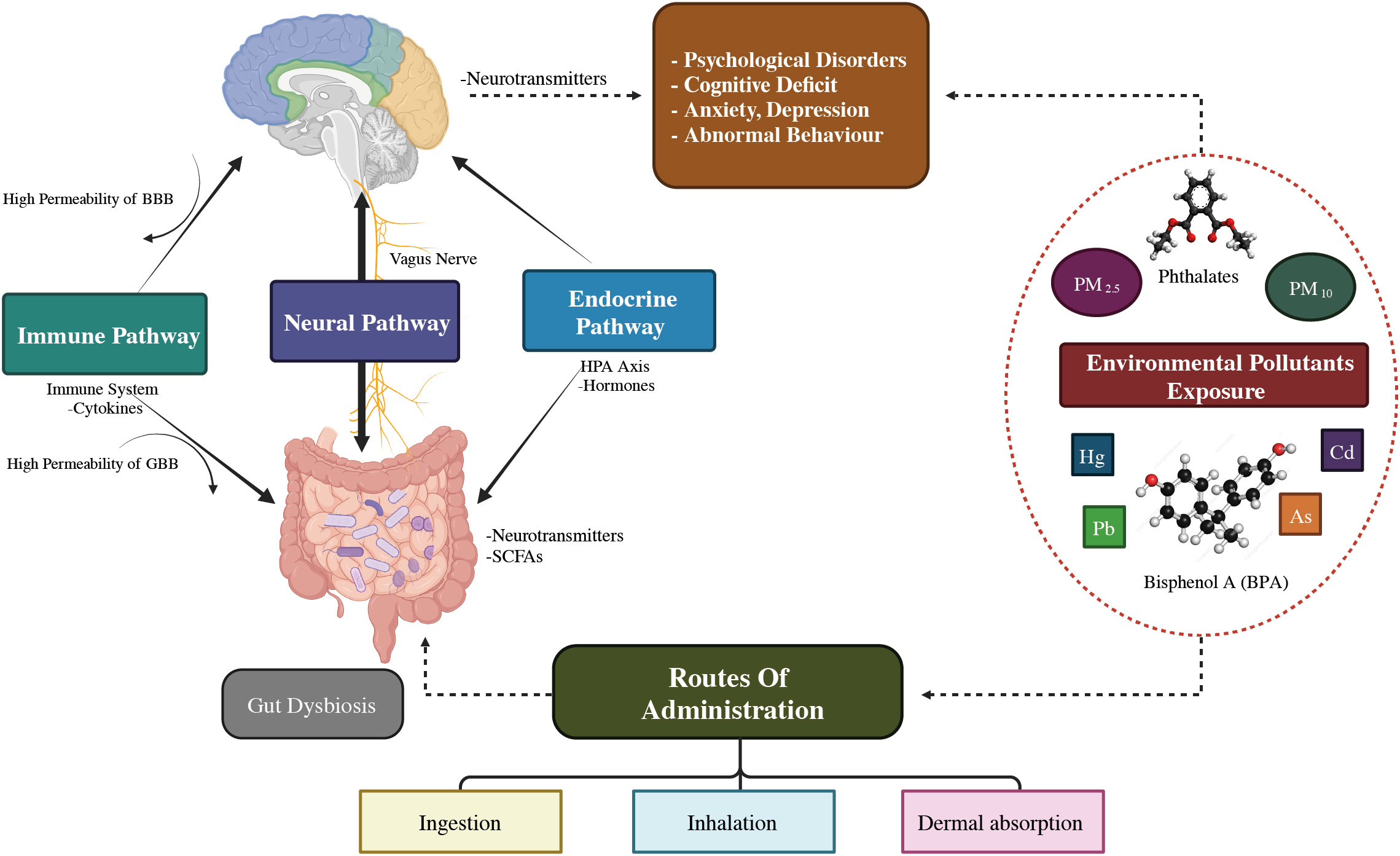
Figure 3 The illustrative demonstration of possible bidirectional linkages that regulate the microbiota-gut-brain (MGB) axis. BBB, Blood–Brain barrier; GBB, Gut–Blood barrier; HPA axis, Hypothalamus–Pituitary–Adrenal axis; SCFAs, Short chain fatty acids; Cd, Cadmium; As, Arsenic; O3, Ozone; BPA, Bisphenol A; PM, Particulate matter; ↑: increased/higher (53).
Moreover, increased systematic translocation of intestinal metabolites, bacterial particulates, or even microbiota through the damaged intestinal tract (the “leaky gut”) heightens systemic inflammatory reactions such as Th17/Treg disorder, interleukin-6, IL-1, and tumor necrosis factors alpha that are being implicated in the etiology of depression. The enteric nervous system (ENS), also known as the “second brain,” is linked to the formation of brain disorders (55). By affecting gut secretion, immune defenses, motility, and permeability, aberrant ENS action caused by intestinal disorders exacerbates depression-related clinical modifications. The stimulation of the vagus nerve was initially approved in clinical studies for treatment-resistant depression. Bacterial cells, such as lipopolysaccharides (LPS) and peripheral inflammatory mediators enter the brain via BBB, causing neural inflammation, and subsequently induce neuropathological changes, such as synaptic defects, degeneration, abnormal neurons, and brain chemicals discharge which contribute to the development of depressive disorders. Excess cortisol promotes gut disease as part of the brain-gut axis by altering the integrity of intestinal barriers and inflammatory responses, leading to a leaky gut; this pathway is an important aspect of the MGB axis in depression. Other signal transduction systems and metabolic pathways involved in the MGB-based cause of depression include the endocannabinoid system, CAMKII-CREB and MAPK signaling, and glycerophospholipid metabolism. These bidirectional gut-brain communication routes form a complex network of systems, and their interconnections confounded studies into the mechanisms behind the microbial modulation of depressive disease. Ultimately, the MGB axis is responsible for the systemic diffusion of several hormones and metabolites into the bloodstream (56–59).
3 Composition of gut bacteria and the GBA
The composition of gut microbial communities varies among individuals, and approximately 70% of species-level phylogenetic types are distinct to each person (60). Several studies have found a link between dysbiosis and depression by comparing alterations in the gut microbial profile of sufferers of MDD to healthy persons, notably in terms of the variety of bacteria and relative number of specific microbial taxa as shown in Figure 4. In Depression, Firmicutes (primarily the genera Clostridium, Enterococcus, Lactobacillus, and Faecalibacterium) and Bacteroidetes (particularly the species Bacteroides and Prevotella) account for over 90% of the adult gut microbiota (61). Proteobacteria, Actinobacteria, Fusobacteria, and Verrucomicrobia are some of the other phyla found in MDD patients. In MDD patients with MDD, there was a rise in Eggerthella and a decrease in Sutterella (62). The initial phases of psychosis appear to be caused by a dysregulated fungal-bacteria interaction, and gut fungal dysbiosis has been associated with depression (63). The microbiota also includes eukaryotes such as yeasts and protists, viruses, and a few archaea species (Methanobrevibactersmithii) (64, 65). Studies on animals have shown that a gut microbe imbalance alters neurological chemistry, metabolic status, and brain activity (66). By overexpressing tight junction proteins and maintaining the blood-brain barrier (BBB) integrity, short-chain fatty acids (SCFA) can penetrate the BBB via monocarboxylate transporters (MCTs) (67). The SCFAs acetate (C2), propionate (C3), and butyrate (C4), produced by various microbial species in the gut are the most prevalent anions or metabolites in the gut which have a substantial role in psychiatric diseases (68, 69), in both direct and indirect ways (67). Modifications in neurotransmitter levels by modulatory pathways such as the kynurenine pathway, and also changes in accessibility and effects of SCFAs in the brain, may have an impact on BDNF activities such as neural survival and CNS development (70).
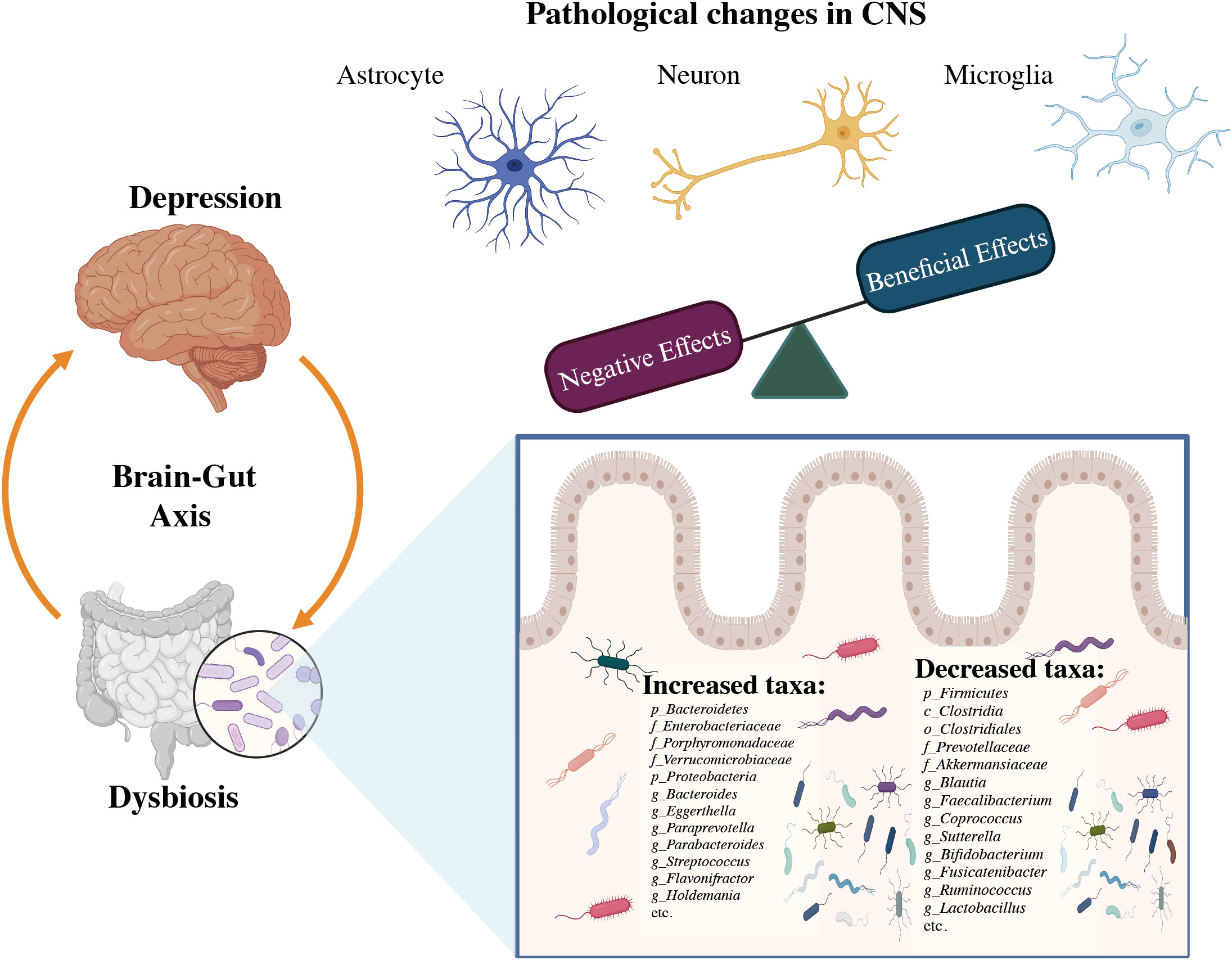
Figure 4 The association between dysbiosis and brain infectious modifications during the course of depression (32).
The SCFAs also influence the secretion of intestinal hormones such as peptide tyrosine (PYY), cholecystokinin (CCK), and glucagon-like peptide-1 (GLP-1) from gut mucosal enteroendocrine cells that express free fatty асid receptors (FFARs) (71, 72). Blood-borne PYY and GLP;-1 enter the cerebellum of rodents and have major effects on neurotransmitters and behavior. Daily exposure to a wide range of environmental pollutants alters our gut microbes, inhibits bidirectional GBA, and may result in the development of psychiatric diseases (53). Even so, it was demonstrated that the primary mechanisms by which psychobiotics exert their effects include HPA axis regulation, immune response and inflammation modulation, and neurohormone and neurotransmitter synthesis as depicted in Figure 5 (70).
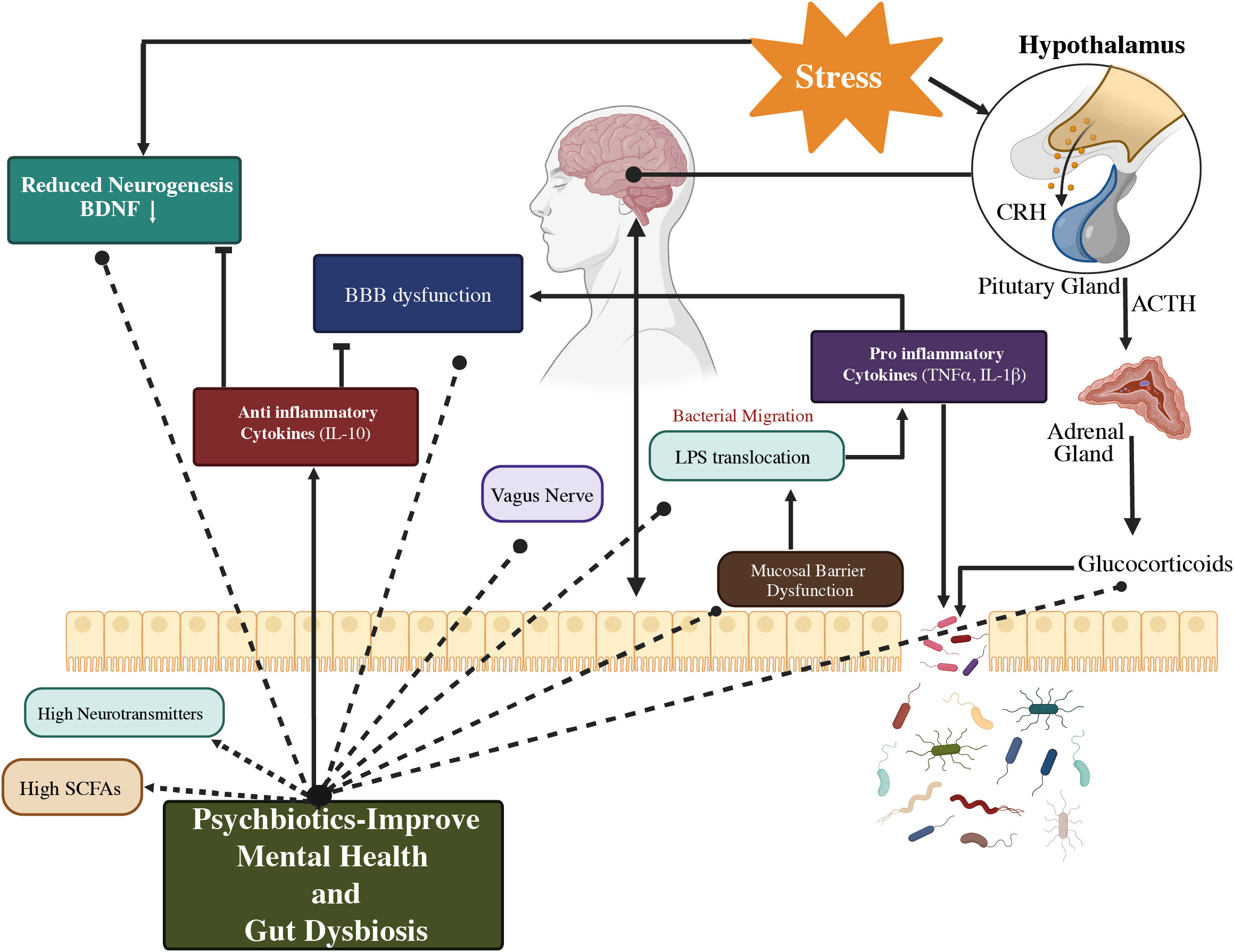
Figure 5 A representation of a proposed method of action for psychobiotics, involving intestinal microbiota modification. Psychobiotics treat psychological conditions by lowering inflammation, repairing gut permeability, restoring BBB integrity, modifying neurotransmitters, regulating the HPA axis, and increasing SCFA levels. BBB, Blood–Brain barrier; HPA axis, Hypothalamus–Pituitary–Adrenal axis; CRH, Corticotrophin-releasing hormone; ACTH, Adrenocorticotropic hormone; SCFAs, Short-chain fatty acids; IL-10, Interleukin-10; TNF α, Tumor necrosis factor α; BDNF, Brain-derived neurotrophic factor; LPS, Lipopolysaccharides; ↑: higher/increased (53).
The pathophysiology of various mental diseases, including anxiety and MDD, is linked to gut microbiota dysbiosis (73, 74). It was shown that some pathogens and enterotypes have significant contributors to induce mental disorders. A total of six microbial taxa were found to have adverse interactions, whereas Hungatella and Fusicatenibacter showed favorable associations, with Butyricicoccus, Clostridium, Desulfovibrio piger, Parabacteroides merdae, and Hungatella showing positive correlations, with the depressive phenome. Patients with mood disorders consistently had lower levels of Faecalibacterium and greater levels of Actinobacteria and Enterobacteriaceae (75). A second enterotype found a decrease in high-density lipoprotein cholesterol (HDLc) and an increase in the plasma atherogenic index (76), and the results reveal that certain microbes are possibly associated with psychological disorders and their manifestation (77). Potential advances in treatments and medical biomarkers may result from an improved comprehension of the functions played by different enterotypes and pathogens in mental health issues (51).
Moreover, several risk factors were linked to the pathophysiology of intestinal dysbiosis, antibiotic usage was shown to both transiently and permanently alter the composition of the gut microbiome (78, 79). Obesity, high-fat diets, and high-sugar diets have all been associated with reproducible gut microbiome modifications (80–82). It is also thought that exposure to environmental pollutants have a role in the development of gut dysbiosis at different stages of life (83, 84). In addition, related to gut dysbiosis include xenobiotics, such as exposure to pesticides and heavy metals, as well as social stressors (50, 85). The interaction is facilitated by the microbiota, but a dysbiotic biota can lead to brain inflammation and psychopathologies as depicted in Figure 6 (86, 87).
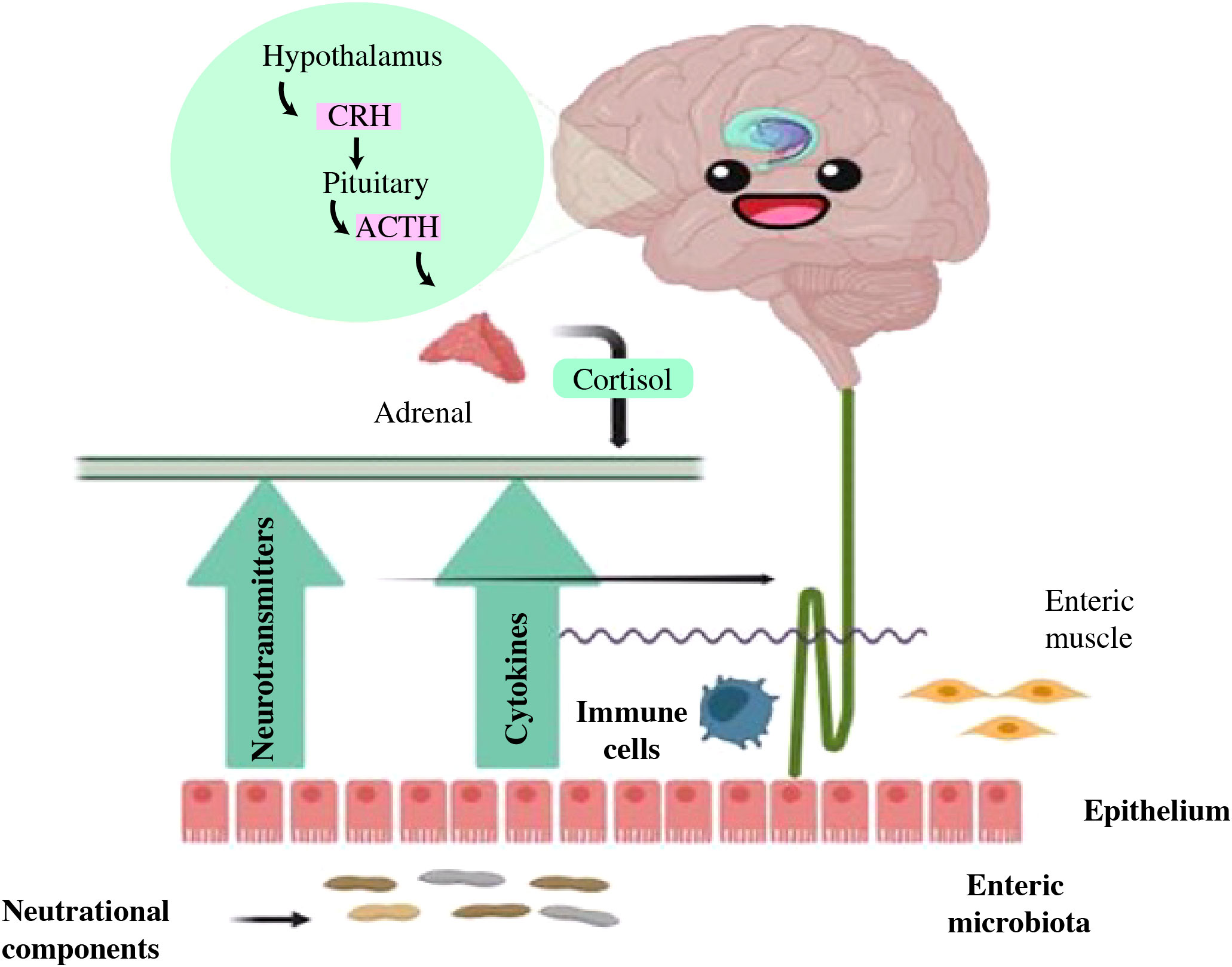
Figure 6 The reciprocal interaction between gut microbiota and the brain. Gut microbiota may modulate brain function and development through immune signaling (anti-inflammatory cytokines, chemokines, and immune cells), endocrine, and neural pathways. The brain may influence the gut through neurotransmitters that impact immune function and through alterations in cortisol levels, intestinal motility, and permeability. Nutritional components may exert effects on each of these communication pathways (86).
Both clinical and preclinical research has found the influence of bacterial alterations on depression concerning inflammatory bowel diseases (IBD). Over 20 percent of individuals with IBD experience depression and difficulty sleeping. Interventions targeted at reducing inflammation have become increasingly popular among both IBD patients and healthy individuals since inflammation impacts brain tissue and their cognitive abilities (88). Individual’s distinct intestinal bacteria are determined by their surroundings, developmental stage, and genetic profile (89). The etiology of depression is often linked to the intestinal MGB axis, and it overlaps with the pathways that contribute to the progress of IBD and obesity (90). Studies conducted on individuals exhibiting depression symptoms showed that using probiotic supplements may have minor antidepressant properties (91). The gut’s reciprocal association with the neural network affects the formation of local inflammatory damage and psychophysiological sensitivity in IBD individuals (92). Depressive disorders and increased stress sensitivity are often associated with IBD, which might impact the progress and severity of the disorder. In general, the gut-mood correlations in this instance of IBD have been confirmed by the medical investigations (93).
The gut microbiota has evolved more pathogenically over time due to succession and within-host evolution, which may have caused unique microbial traits in MDD patients of different ages (48, 94, 95). Many studies explored age and gender as treatment variables for depression. Globally, women are prone than men to experience depression-related disorders (96). The probability of mood disorders decreases with age, and the difference in frequency between young men and women vanishes in individuals over 75. It was found that the hyperlink between depression and married life varies with age and gender. The risk rates for depression were lower for widowed, single, or divorced women compared to married individuals (97). In 2019, 4.2% of individuals identified with moderate symptoms, 11.5% had mild symptoms, and 2.8% of adults reported having profound signs of depression. The demographic of 18 to 29 years old comprised the highest number of adults with signs of depression (21.0%), followed by 45 to 64 years old (18.4%), 65 and older (18.4%), and 30-44 years old (16.8%). Women were more likely than men to experience moderate, severe, or mild manifestations of depression (98). Studies found an association between markers of quality of life (QoL) and certain intestinal microbes (Faecalibacterium and Coprococcus) associated with depression (99, 100).
The term “quality of life” encompasses a broad range of elements, including living conditions, opinions, social contacts, mental health, and degree of independence. It was used as a predictor of both the efficacy of therapy and depression relapse. The QoL level is similarly lower in euthymic depressed people, and this can be impacted by mental abilities, clinical symptoms, and social factors. A few studies have looked at the QOL of euthymic individuals with MDD and depression (101). After considering antidepressant effects, it was discovered that depression correlates with a depletion of the number of Dialister and Coprococcus spp. (102). Moreover, bacterial production of the dopamine metabolite (3,4-dihydroxyphenylacetic acid) is strongly linked with mental QoL, while γ-aminobutyric acid is associated with clinical depression. They provide evidence suggesting the intestinal microbes and QoL variables have mental health implications (93). Furthermore, animal studies demonstrate that the microbiota in the gut can alter the neurological aspects of depression (103), such as low-grade immune activation (104), the HPA activity (105), altered tryptophan metabolism (106), and neurotrophic factors (107, 108).
Some research indicates that transplanting the microbiome from one animal (either stressed or fat) to another control animal can significantly alter anxiety-like behaviors, which are a common comorbidity of depression (109). Different lactobacillus and bifidobacterial species have been found in animal models to influence depression and stress-related behavior (110–112). Recently, an abundance of experimental research was conducted, especially on animals, to discover how the gut microbiota influences GBA (27). Prebiotic and probiotic use may benefit mood and anxiety aspects, alter HPA function, and alter brain activity, according to a growing body of research conducted on healthy individuals (113, 114).
Moreover, bacteriophages help the microbiota of bacterial species perform their functions by increasing the range of the colon microbial alleles and traits (115). It demonstrates that MDD patients have changed the bacteriophage population, particularly in the phylum Caudovirales (48). Prior reviews covered the part Candida albicans plays in the pathogenesis of psychological syndromes (116), owing to the intricacy of interactions between bacterial and nonbacterial gut microbiota. Antibiotic usage, persistent stress, and a poor diet are other factors that impact gut flora and raise the risk of depression (117, 118). Multiple studies are being conducted on the intestinal microbes of developed countries; however, there is a dearth of research on indigenous tribes that are not subjected to similar stresses and situations as modern developed nations. Recent studies have highlighted the potential link between microbiota and mental disorders in such groups. It was revealed that MDD individuals have altered β, and α-diversity as well as a reduction in butyrate-producing bacteria. Initial studies suggest that the microbiome may be utilized to predict treatment outcomes and diagnose psychological problems. Moreover, clinical study outcomes support the use of probiotics as an adjunct in the MDD treatment, and determine the efficacy of psychobiotic therapy in aboriginal tribes with psychiatric conditions (119, 120). However, the induction of the gut bacteria via prebiotics, probiotics, or antibiotics, and specific FMT-based studies, is an effective therapeutic or preventative measure to counteract behavioral and cognitive problems, and these may be useful to supplement the action of drugs for the management of brain disorders (121).
3.1 Antibiotic use in neurological disorders and potential side effects
The first known case of antibiotic-induced depression was reported in 2010, and it may be a contributing factor to anxiety and depression. A well-known antibiotic minocycline has demonstrated promising results in treating depression in both humans and animals (122–124). The monoamine theory of depression led to the development of both tricyclic antidepressants (TCAs) and serotonin-specific reuptake inhibitors (SSRIs), which both increase central monoamine levels. This concept is still being studied in great detail. Several newer antidepressants, such as the SSRI, and fluoxetine have antibacterial activity to treat depression (125, 126). The improper use of antibiotics can significantly affect the diversity of bacterial species, potentially leading to both short and long-term shifts in the composition of intestinal bacterial flora. This can subsequently result in dysbiosis and disruptions in intestinal function (69). The field of administration of antibiotics is a significant issue, as seen in Table 1 (127–129). Based on a detrimental clinical study, the administration of beta-lactam intravenously, comprising ampicillin, sulbactam, and cefazolin, not only disrupts the balance of bacteria but also affects the synthesis of powerful metabolites. Horizontal gene transfer (HGT) and antibiotic resistance genes both exist. Since probiotics, commensal, and opportunistic infections can survive against antimicrobial drugs by passing resistance genes, this has several therapeutic consequences. Although gene therapy and personalized drugs could treat antimicrobial resistance, probiotic-induced resistance requires significant interventional study to uncover the host-specific antibiotic-resistant genes (130). Antibiotics can alter the gut microbiota, and microbes can also modify the drugs, altering their chemistry and leading to the development of drug resistance.
3.2 Chronic stress
The term “Stress” identified by Hans Selye, defined it as the body’s entire reaction to demands placed on it. He identified the three different stages of the body’s stress response including alarm, resistance, and weariness. He also separated stress into two types such as acute (short-term) and chronic (long-term), depending on how long the stressful event lasted (131, 132). Stress is a prevalent risk factor for over 75% of both physical and mental health conditions, contributing to elevated morbidity and mortality rates (133). Chronic mild stress (CMS) is widely cited in the literature as a substantial predictor of having a severe depressive episode (1, 134). Historically, the attention was mostly on traditional pathways, the HPA axis, and the sympathetic nervous system that link stress to disease. Inflammation has come to be a key mechanism behind the adverse consequences of anxiety (135–139). Depressed has also been associated with disruptions in the immune system, particularly the reduction of natural killer cell activity. Furthermore, preclinical models indicate a link between depression and prolonged stress. In such models, persistent corticosterone (CORT) treatment, exposure to erratic moderate stressors, and social defeat are standard techniques for producing depressive-like behaviors in animals. These actions comprise feeling helpless and anhedonia, two hallmarks of depression symptoms (140, 141). Interleukin (IL)-1b and TNF-alpha are two inflammatory markers that have significantly risen in animal models as a result of increased stress hormone production (142). Individuals suspected of depression usually have higher serum or plasma concentrations of several cytokines, including TNF-α and its soluble receptor TNFR2. This data is derived from a meta-analytic investigation that concentrated on the main cytokines examined. Conversely, individuals with depression appear to have lower IFN-α levels than healthy controls. These findings highlight the intricate link between the immune system and depression, suggesting that inflammation may play a role in the development of depressive symptoms (143–147). The higher levels of C-reactive protein (CRP), a widely employed indicator of inflammation, were found to be predictive of the onset of depressive symptoms (148, 149). More study is required to fully comprehend the potential link between depression and inflammation. Remarkably, a few studies indicate that inflammation may not be high in the early stages of MDD, which may indicate a poor prognosis for the first antidepressant medication. The differences in the duration or chronicity of the MDD may account for these disparities in inflammatory markers (150, 151).
3.3 Poor diet
Nutritional psychiatry is an emerging field of science that focuses on the relationship between dietary and mental health, brain function, and mental disorders (152). Understanding how diet and nutrition affect these pathways may provide important new information on effective MDD treatment strategies. One particularly effective method that has shown promise is the Mediterranean diet (MD), which may help improve the clinical outcomes for depressed patients (153). A study suggests that eating habits and dietary quality may affect the mental health of adolescents (154). Numerous studies have shown that poorer quality diets, such as the Western diet, can lower Lactobacillus levels, reduce the richness of the entire microbial community, and be associated with depression risk susceptibility (155). Twelve epidemiological studies were conducted to examine any possible links between trends in children’s and adults’ mental health and the quality of their diet (154). The intake of folate, zinc, and magnesium appears to be negatively connected with depressive disease, which means that a larger intake of these nutrients is linked to a decreased risk of depression. Because of the opposite link between omega-3 fatty acids and anxiety disorders, a higher intake of omega-3 fatty acids corresponds to a decreased risk of developing anxiety disorders (156). Therefore, dietary interventions are emerging as highly promising avenues of exploration in the realm of MDD. It is attributed to the presence of certain nutrients such as omega-3 fats, vitamins, polyphenols, and cocaine, as well as foodstuffs such as fish, nuts, seeds, vegetables, fruits, coffee/tea, and fermented goods, which are currently being studied. As demonstrated in Table 2, dietary supplements such as S-Adenosylmethionine, acetylcarnitine, creatine, and amino acids have been investigated (157, 158). The study of eating habits and the broader nutritional understanding is still a potential field of investigation. Interventions that use the Greek diet show substantial advantages to those suffering from profound depression. However, further research is needed to confirm their value and completely understand the multifaceted relationship between nutrition and the MDD (159, 160).
4 Gut microbiota-derived metabolites and depression
Bacterial compounds found in the gut can enter the bloodstream affect brain regions and can interact with nearby gut components like hormonal cells or neurons, which can subsequently send signals to the brain (161). Numerous bioactive chemicals derived from gut microbes have been shown through experiments to affect the brain through direct or indirect methods. These processes can exacerbate neurological disorders or neuropathology when they become imbalanced (162). Numerous microbial species that may produce neurotransmitters, signaling chemicals, or metabolize their precursors into various substances can be found in the human microbiome (163–165), during food fermentation and bacterially altered host molecules including bile acids (BAs) (166), tryptophan metabolites, and SCFAs with CNS effects are also produced by the gut bacteria. The metabolites from the gut microbiota are a double-edged sword in depression. (167).
4.1 Short-chain fatty acids
Small organic molecules referred to as SCFAs are readily engaged in the large intestine and produced through anaerobic fermentation, primarily in the cecum and colon, from dietary carbohydrates that are typically indigestible by our bodies (168). Although there are conflicting reports of how SCFAs affect behavior, they are believed to have a role in digestive, immunological, and molecular processes. Acetate, butyrate, and propionate have been demonstrated to lessen the symptoms of depression in rats (169). According to Kelly et al. (170), transplanting the fecal microbiota of MDD patients into sterile rats led to an increase in fecal SCFA levels when compared to controls and sodium butyrate improved depressed behavior in rats. Bacterial butyrate has been demonstrated to control the tyrosine hydroxylase gene’s expression, which in turn controls the production of dopamine, adrenaline, and noradrenaline by suggesting to have antidepressant properties (24, 171). The SCFAs were shown their treatment had antidepressant benefits by reducing the permeability and the HPA axis, particularly butyrate (168, 169). The MGBA has suggested that acetic, propionic, and caproic acids may have an impact on when depression symptoms appear. For example, acetate helps to defend against enteropathogenic infections and strengthens the intestinal barrier (172, 173). The isovaleric acid was found to have a causal link between signs of depression and blood cortisol levels and appears to inhibit neurotransmitter release in the synaptic cleft (174). Certainly, SCFAs have been observed to enhance the production of anorexigenic neuropeptides and alter the concentrations of neurotransmitters such as glutamine, glutamate, and GABA in the hypothalamus. The tryptophan 5-hydroxylase 1, crucial for the synthesis of serotonin (5-HT), and tyrosine hydroxylase, a rate-limiting enzyme in the production of dopamine, adrenaline, and noradrenaline, are both positively regulated by SCFAs (175, 176).
4.2 Bile acid
Bile acids are naturally occurring chemicals produced in the liver. In mice, bile acids can breach the BBB by activating the nuclear receptor farnesoid X receptor (FXR) in the ileum. This activation leads to the production of fibroblast growth factor 19 (FGF19) or its equivalent, fibroblast growth factor 15 (FGF15), which can suppress the HPA axis. This suppression of the HPA axis can have a protective effect against depression (71, 177, 178). It suggests that elevated BA levels can serve as a type of depression defense. Turicibacterales, Turicibacteraceae, and Turicibacter were associated with the increase in BAs production (32). At present, the precise mechanisms through which secondary bile acids influence the development of MDD remain unclear. Additionally, it is uncertain whether certain antidepressant medications, like paroxetine, a selective SSRI, have an impact on the gut microbiota. To gain a comprehensive understanding of the role of secondary BAs in MDD and the potential effects of antidepressant medications on the gut microbiota and BA synthesis, further research is necessary. This ongoing investigation is crucial for elucidating the complex interplay between these factors and their relevance to the management and treatment of MDD.
4.3 Trimethylamine N-oxide (TMAO)
Trimethylamine-N-oxide (TMAO), a tiny chemical compound produced by gut bacteria, has lately been linked to postoperative cognitive and neurodegenerative impairment (179). Foods containing phosphatidylcholine and L-carnitine are included in diets such as eggs, red meat, cheese, and sea salt fish. The gut microbiota converts these nutrients into trimethylamine (TMA), which is then oxidized by the hepatic FMO-1 and FMO-3 enzyme family members (180). Therefore, a variety of influencing variables, including diet, the gut microbiota, liver function, and the GBB, which controls the permeability of molecules produced from the gut into the bloodstream, affect serum TMAO levels (181, 182). Recently, it was demonstrated that variations in the TMAO concentration produced by gut bacteria are similarly associated with depression (183). The Firmicutes phylum contains the majority of the bacteria that produce TMA, including some Actinobacteria and Proteobacteria as well as Clostridium cluster XIVa and Eubacterium strains (184). Elevated production of TMA and its subsequent conversion to TMAO is associated with the potential to accumulate in various tissues, exerting effects on multiple organs and systems within the body. This phenomenon, notably affecting the cardiovascular system and the liver, demonstrates an adverse correlation with gut dysbiosis (185, 186). Prior research found an association between TMAO levels and the severity of depressive symptoms in both males and females. Overall, further study is needed to understand how the gut microbiota contributes to the neurocognitive advantages of TMA/TMAO in people with MDD. Other microbiota-derived metabolites linked to MDD pathophysiology include LPS, lactate, vitamins, aromatic amino acids, and tryptophan metabolites (187, 188).
5 Epigenetic-modulated inheritance and depression
The estimated familial risk of depression is approximately 35%, compared to the 65–70% hereditary rates for bipolar disorder and schizophrenia (189, 190). Recently, over 80 replicable loci linked to MDD were found by a genome-wide association study (GWAS), each of having a negligible impact (191, 192). Besides, only a very small portion of the overall inherited risk remains defined by the major depressive polygenic risk rating determined by these genetic locations (191). It was suggested that many gene variations that cause genetic risk are still unknown and other factors are strongly linked to the chance of developing MDD and other anxiety-related diseases, as shown by epidemiological research (193–195). It is becoming more widely recognized that variations in epigenetic modification lead to aberrant neuroendocrine responses, loss of neuroplasticity, neurotransmission, and dysfunction of neuroglia, all of which have been associated with the etiology of MDD and stresses such as anxieties and post-traumatic stress disorder (PTSD) (196, 197). Modifications in monoamine neurotransmitter function, altered stress-responsive mechanisms, and brain inflammation are all regulated by genetics. Moreover, epigenetic biomarkers have a role in the identification and treatment of MDD. Knowing the complex etiology and varied hallmarks of MDD may be enhanced by examining epigenetic changes, which could provide novel avenues for treatment (198, 199).
Although MDD supports their usage, the field of etiology research is still evolving by substituting peripheral epigenetic changes for brain activity. Nonetheless, it could be useful in developing biomarkers for disease and the efficacy of a treatment (200). Depression and anxiety may occur as a result of external variables such as parental stress, childhood trauma, and traumatic events in adults (201). Such variables may interact with genes through gene-by-environment interactions or direct epigenetic regulation. These processes include modifications to DNA, non-coding RNA, the structure of chromatin, histones, and so on (202). Several cytogenetic structural modifications associated with MDD are primarily unknown (203, 204). The m6A-methylated RNA has been identified by MeRIP-Seq methods offering a novel avenue for studying the transcriptome-wide expression of m6A in mental disorders. When combined with the widely used next-generation RNA sequencing methods, such research methods should enable an increased understanding of the possible pathways underlying depression (205).
There is evidence from new studies that brain chromatin receives metabolic signals (206). The regulation of glycolytic genes is regulated by lactate-dependent histone lactylation (H4K12a) in microglia, which results in microglia dysfunction and mental retardation (207). Further investigation is required to examine the role that metabolism-dependent genetic mechanisms play in the development of depression and whether these mechanisms can have effects similar to those of antidepressants. Moreover, several studies have revealed an association between genetics, the gut flora’s metabolites, and the intestinal microbiome, highlighting the influence of the microbiota on the synthesis of non-coding RNA (208, 209)., With the advent of novel mutagenic techniques such as Transcription Activator-Like Effector Nucleases (TALENs) and Clustered Regularly Interspaced Short Palindromic Repeats (CRISPR-Cas9), diseases related to the real induction of epigenetic loci at known stress-related DNA locations can be investigated (210, 211)., These advances allow us to focus on specific genes of the brain, and periods with epigenetic regulators to find out whether regulation of epigenetic changes at specific loci causes a distinct mental disorder (212).
6 The gut microbiota’s clinical application potential in MDD
By reducing neural inflammation and brain degeneration, probiotic, prebiotic, and fecal microbiota transplant administration may be a useful treatment for gut dysbiosis and enhance cognitive impairment (213, 214). To maintain a healthy balance, the activity of the gut bacteria can be controlled by diet, the use of drugs that encourage the growth of the microbiota, and the incorporation of microbes to nutrients (215). Figure 7 depicts current approaches for modifying gut flora, with a focus on the health advantages offered by integrated synbiotics and immobile probiotics (216).
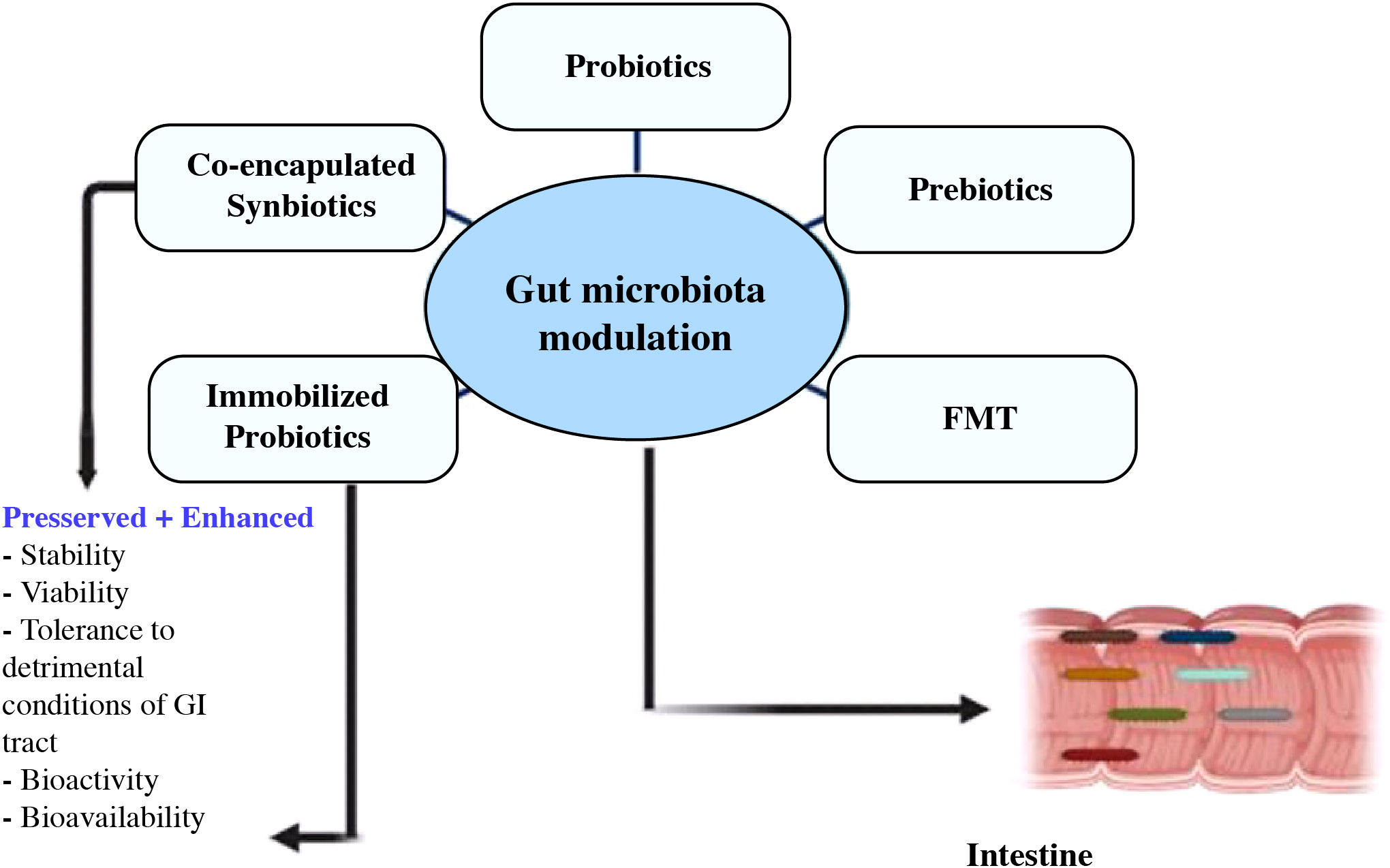
Figure 7 Utilizing modern techniques, co-encapsulated synbiotics and immobilized probiotics offer benefits in the targeted control of the gut flora (216).
6.1 Prebiotic
The International Scientific Association for Probiotics and Prebiotics (ISAPP) has modified the definition of prebiotics to “a substrate that is selectively utilized by host microorganisms conferring a health benefit” and has expertise in microbiology, nutrition, and clinical research(217). The original prebiotic definition of chemicals based on carbohydrates was expanded to include non-carbohydrate substances such as PUFAs, polyphenols, etc. Prebiotics like inulin and short-chain carbohydrates work as a substrate for naturally occurring colonic bacteria like Bifidobacterium and Lactobacillus. Prebiotic use results in an increase in healthy bacteria in the gut microbiome, which benefits health (218).Prebiotics are classified as functional foods in the scientific literature due to their significance in enhancing health and avoiding infection (219). It includes the factors that promote the growth of beneficial microbes and the positive impact their products and metabolites have on health indexes. In addition to phenol, inulin, and chemicals from vegetables, herbs, and plants, fructooligosaccharides (FOS), galactooligosaccharides (GOS), and inulin are the most explored prebiotics and the prebiotics with the most evidence in the treatment of depression (220). The fructo-oligosaccharides and B-immunogalacto-oligosaccharides promoted the development of helpful bacteria like B. longum and decreased stress-induced activation of the HPA axis (221). However the anxiety and depressive-like behavior caused by LPS has been demonstrated to decrease after receiving B-immunogalacto-oligosaccharide (222). The prebiotics do not directly affect the body, but they do indirectly improve host health by encouraging the development of probiotics. As a result, prebiotics and probiotics are frequently used in the treatment of depression (223).
6.2 Probiotics
The name “probiotic” is derived from the Greek word “pro bios,” which means “for life,” and the positive impact of lactic acid fermentation products on human health has ancient origins (224). Both transient and native bacteria can be found in the human digestive system. The majority of native bacteria are a component of the commensal microbiota, even though some of them have been classified as potentially harmful or health-promoting. Bifidobacterium and Lactobacillus genera are the most common strains and potential sources of probiotics, and some of these organisms have powerful anti-inflammatory properties (225). Probiotics maintain the integrity of the gut barrier’s integrated function and reduce intestinal permeability, which lowers endotoxin levels (226, 227). Recent research suggests that the probiotic lactobacillus rhamnosusHN001 enhances psychological health in clinical populations (228). In 1910, Phillips’ study (229) published the first account in the literature of the beneficial benefits of probiotics in the treatment of depression. Miyaoka and colleagues examined the use of Clostridium butyricum (CBM588) as an additional therapy in patients with MDD who were resistant to treatment (230).The majority of probiotic regimens for the treatment of depression are based on Lactobacillus spp. and Bifidobacterium spp., according to a prior thorough investigation that found 178 species and subspecies of probiotics that can attenuate depression (231). Several probiotic species, including L. helveticus, L. rhamnosus, B. longum, and B. breve CCFM1025, are effective in treating clinical depression (Table 3) (232). A probiotic treatment dramatically raised the amount of Lactobacillus and reversed immunological alterations while reducing anxiety and depressive-like behaviors (233). Majeed and colleagues found a notable improvement in the symptoms of depression and IBS in patients treated with Bacillus Coagulans MTCC 5856 (234). The evidence suggests that Lactobacillus plantarum DR7 may offer benefits in terms of alleviating symptoms and improving psychological ratings. This particular strain of Lactobacillus is being investigated for its potential positive impact on various aspects of mental health and well-being (235).
6.3 Dietary interventions
Diet has been shown to have a significant effect on mood disorders and also on the composition and functionality of the gut microbiome (236, 237). There is an association between diet and depression, but because studies have been contradictory, the cause and effect of the association are still unclear. The Mediterranean diet (MD) (159, 160, 238), the Japanese diet (239), and the Norwegian diet (240) are just a few examples of “healthy” dietary patterns that are known for their abundance of vegetables, fruits, cereals, nuts, seeds, and pulses as well as their moderate amounts of dairy, eggs, and fish and unsaturated fats for treating depression. The MD diet which is abundant in nutritional polyphenols and fibers and has anti-inflammatory traits, is widely believed to be good for human wellness. Evidence shows that MD diet is linked to increased quality of life, a lower risk of developing metabolic disorders including diabetes and obesity, a lower risk of developing cancer and heart disease, and an improvement in mental health (241). The prevalence of depression is greater in those who follow a “Western” dietary pattern, which includes sugary and fatty meals, refined grains, fried and processed foods, red meat, high-fat dairy products, and low intake of fruits and vegetables (242, 243). The correlative investigations of healthy individuals revealed that eating “healthy” diets with lots of fruits and vegetables, moderate amounts of dairy, eggs, and fish, and unsaturated fats results in a decreased prevalence of depression (244). The association between depressive disorders and relative intake of carbohydrates is studied using DNA data from over 400,000 individuals. A 16% rise in calories derived from glucose was shown to be related to a 58% reduction in the rate of depression; the protective effect involves significant association (245).
Moreover, in France identified a link between bad nutrition and depression incidence, it was most likely due to reverse causation, with depression raising the likelihood of poor eating habits (246). Consuming meals high in sugar and saturated fats is prompted by depression symptoms, which cause gut dysbiosis and exacerbate depressive symptoms. It should come as no surprise that the Western diet, which is heavy in fat and sugar, raises intestinal permeability and pro-inflammatory signaling (247). However, a diet high in fiber, for instance, should be taken into consideration as adjuvant therapy for MDD since it stimulates the synthesis of immunomodulatory substances, such as SCFAs. In fact, Bifidobacterium, a species that was found in higher concentrations in the blood of MDD patients who consumed fermented milk products (248, 249). However, nutritional interventions should be used cautiously for the medical management of significant depressive disorders; they ought to be paired with other therapies such as behavioral therapy, medication for depression, and habitual modifications.
6.4 Synbiotics and postbiotics
A synergic combination of probiotics and prebiotics is referred to as a synbiotic, and prebiotics specifically have a role in promoting probiotic colonization of the gut (250). In a randomized experiment, the probiotic combination of B. lactis, B. bifidum, L. acidophilus, and B. longum with the synbiotics FOS, GOS, and inulin decreased depression and raised blood levels of a brain-derived neurotrophic factor in depressed patients compared to controls (251). According to this study, probiotics alone did not significantly reduce depressive symptoms as much as synbiotics did. Stated differently, despite the fact that the term “postbiotics” has been utilized in the literature for over two decades, there has only been a notable surge in research on its effects during the last five years (252). Postbiotics have therapeutic benefits that are comparable to those of probiotics in that they maintain the integrity of the epithelial barrier function, restore the variety and composition of the microbiota, manage immune reactions, and modulate signaling along the gut-brain axis (253). This presents a cutting-edge microbial treatment strategy for depression. According to studies, regulating the gut-brain axis protected mice against Salmonella-induced depressive-like behavior by pretreatment with heat-killed probiotic L. plantarum-derived Postbiotics, notably their metabolites (254). Some bacterial metabolites, including SCFAs and BAs have postbiotics properties. Sodium butyrate was administered orally to mice to cure depressive-like behaviors and to repair changes in gut barrier integrity and microbiota composition brought on by the drug paclitaxel(255). Similar to probiotics, Postbiotics may exert health benefits through similar mechanisms, such as improved epithelial barrier function, host-microbiota modulation, immune response modulation, systemic metabolism modulation, and nervous system signaling (256, 257).
6.5 Fecal microbiota transplantation
To balances the recipient’s unbalanced gut microbiota, FMT, which first emerged in China in the 4th century, entails injecting a healthy donor’s fecal solution into the recipient’s intestine (256). While only a few of the conditions that have been shown to benefit from FMT therapies include IBS, ulcerative colitis, and recurrent and refractory Clostridium difficile infection (258–260). Due to the postulated importance of the MGB axis in the pathophysiology of depression, the potential use of FMT as a therapeutic strategy to treat neuropsychiatric diseases (261). The GBA’s vagus nerve may be a crucial signaling mechanism that controls the antidepressant properties of FMT (170). Pre-clinical research has therefore demonstrated the contagiousness of gut microbiota-driven behaviors (64). FMT administration slowly decreased signs of depression in patients with diarrhea-predominant irritable bowel disorder, regardless of intestinal disease remission. These optimistic clinical outcomes were validated in individuals with concurrent IBS-D, stress, and depression by a randomized controlled study (RCT) (262). Despite the lack of research on FMT from healthy donors to depressed recipients, the potential for FMT to be beneficial in treating MDD stems from the transmissibility of behaviors linked to microbiota (263, 264). When developing medications related to FMT, it is crucial to maximize safety while considering both immediate and long-term adverse effects (265). In rodents, FMT-induced changes in the microbial makeup of the gut can modify behavior and have an impact on rodent models of psychiatric diseases (266). There have been no clinical trials on the use of FMT to treat depression. Similarly, FMT may provide antidepressant microbiota to depressed people, and anxiety is transmissible via FMT in mice (263). By using FMT, one may supplement harmful gut bacteria with healthy ones. So, it is possible to anticipate advantages in the immunological conditions used to treat neuropsychiatric disorders (267, 268). Even yet, more study is required before FMT may be used to treat depression. It’s fascinating to note that the clinical improvement caused by FMT was accompanied by an increase in the diversity of gut bacteria and a decrease in GI symptoms (269).
7 Conclusion, limitations, and future thrust
7.1 Conclusion
The maintenance of human health is significantly influenced by the gut microbiota, and research on the microbiome has advanced significantly, most notably in understanding how it functions biologically in depression. Examining whether gut microbiota and depression are related would allow researchers to address several significant concerns and potentially helpful treatments. To better understand the mechanisms of these disorders related to therapy, further research in the future is required on what causes the development of dysbiosis and depression, as well as drug-microbiota interactions. The gut flora and MDD have been associated in most clinical studies and experimental data about the microbiome’s involvement in the disorder. Research questions like “How does MGB signaling affect brain development?” have also been examined using stool samples. To develop a disease-based strain resource database, more research is required to identify the specific functions of the strains and elucidate the underlying mechanisms governing the gut-brain axis. This will need to identify the pathogenic and beneficial strains implicated in depression through the use of high-throughput sequencing, multi-omics approaches, and microbial culture technology. As adjuvant methods to boost coverage alongside the primary therapy, new probiotics (psychobiotics) or pharmabiotics containing bacteria that produce SCFAs and neuroactive metabolites are being researched. These bacteria inhibit normal depressive stress along the whole MGB axis. More studies and data, especially from randomized controlled trials, are needed to demonstrate the synergistic effects of integrative therapy such as diet and exercise on patients. Isotope monitoring, modified and CRISPR-edited strains, and other emerging methodological tools are assisting in the improvement of microbiota-based therapy methods for depression.
7.2 Limitations
An emerging body of evidence explores the role of intestinal microbiota in depression which presents challenging avenues where it is essential to admit various limitations inherent to this field of study. One of the illustrious challenges lies in constituting directionality and causality in the gut-brain axis, as the bidirectional exchange of information between these systems makes it complex to specifically determine whether the modulations in the microbiota composition are the main cause or consequence of depression disorder. Heterogeneity across the methods of different studies which include alterations in sample collection, data analysis, and sequencing techniques adds a further layer of complexity in drawing appropriate conclusions. Additionally, the diversity of microbiota and its sensitivity to an individual’s lifestyle, genetics, and geographical location further complexes the generalizability of observations. Available research studies are lacking in deep mechanistic insights which left the gaps in interpreting the molecular and cellular pathways interlinking the microbiome to depression symptoms. Clinical and preclinical trials demand microbiome-targeted strategies which should necessarily be based on rigorous, widespread, large-scale, and long-term experiments to further validate the safety and efficacy. Thus, while the role of the intestinal microbiome in depression disorder presents an entrancing exploration avenue, these limitations underline the necessity of moderate interpretation and comprehensive research.
7.3 Future thrust
The future interests of research into the complex association between the intestinal microbiome and depression promise a perspective shift in understanding and management of this intricate disorder. Overcoming current research limitations, future studies are braced to untangle the precise pathways governing the bidirectional exchange of information at the gut-brain axis. By rendering cutting-edge techniques that involve metagenomics and metabolomics, researchers can instantly identify particular microbial species and their linked metabolites which share critical roles in influencing neural mechanisms and behavior. Longitudinal probing can track microbiota dynamics before, during, and after depression events consequently will help in illuminating the causal relationship, potentially leading towards the determination of early diagnostic markers. Integration of a multitude of omics data such as genomics, microbiomics, and metabolomics, with individual clinical and preclinical profiling, will help in the development of tailored management strategies for individuals susceptible to microbiome-associated depression. Artificial intelligence (AI) tools and machine learning algorithms will enable the extraction of complex patterns from intricate datasets, disclosing novel correlations and directing the identification of potential targeted therapeutic measures. Incorporating research-based findings into effective intervention renders a potential frontier. Microbiome-targeted therapeutic interventions such as prebiotics, probiotics, and fecal microbiota can be promising as an adjunctive treatment for depression disorder. However robust preclinical and clinical trials are necessary to optimize these strategies and establish their efficacy and safety profiles. Moreover, investigations into the roles of diet in altering the gut-brain axis render a specific research avenue to promote mental health via nutritional strategies.
Author contributions
AL: Writing – original draft. MH: Writing – review & editing, Supervision. AH: Writing – review & editing. MU: Methodology, Writing – review & editing. LZ: Writing – review & editing. MR: Writing – review & editing. AD: Writing – review & editing. KU: Writing – review & editing. WW: Writing – review & editing. GW: Funding acquisition, Supervision, Writing – review & editing.
Funding
The author(s) declare financial support was received for the research, authorship, and/or publication of this article. This work was supported by grants from the National Natural Science Foundation of China (12032007, 31971242); the Science and Technology Innovation Project of JinFeng Laboratory, Chongqing, China (jfkyjf202203001); Natural Science Fund Surface Project at Chongqing University of Science and Technology (CSTB2023NSCQ-MSX1060) and Chongqing Traditional Chinese Medicine Innovation Team Construction Project. We are also thankful for the First Batch of Key Disciplines on Public Health in Chongqing and the Public Experiment Centre of State Bio industrial Base (Chongqing), China.
Conflict of interest
The authors declare that the research was conducted in the absence of any commercial or financial relationships that could be construed as a potential conflict of interest.
Publisher’s note
All claims expressed in this article are solely those of the authors and do not necessarily represent those of their affiliated organizations, or those of the publisher, the editors and the reviewers. Any product that may be evaluated in this article, or claim that may be made by its manufacturer, is not guaranteed or endorsed by the publisher.
References
1. Kavoor AR. COVID-19 in people with mental illness: challenges and vulnerabilities. Asian J Psychiatry (2020) 51:102051. doi: 10.1016/j.ajp.2020.102051
2. Chang L, Wei Y, Hashimoto K. Brain–gut–microbiota axis in depression: A historical overview and future directions. Brain Res Bull (2022) 182:44–56. doi: 10.1016/j.brainresbull.2022.02.004
3. Irum N, Afzal T, Faraz MH, Aslam Z, Rasheed F. The role of gut microbiota in depression: an analysis of the gut-brain axis. Front Behav Neurosci (2023) 17:1185522. doi: 10.3389/fnbeh.2023.1185522
4. Suda K, Matsuda K. How microbes affect depression: underlying mechanisms via the Gut–brain axis and the modulating role of probiotics. Int J Mol Sci (2022) 23:1172. doi: 10.3390/ijms23031172
5. Nyassi S, Abdi YA, Minto J, Osman F. “Helping mentally ill, a reward both in this life and after”: A qualitative study among community health professionals in Somaliland. Community Ment Health J (2023), 1–13.
6. Zhang Y-J, Li S, Gan R-Y, Zhou T, Xu D-P, Li H-B. Impacts of gut bacteria on human health and diseases. Int J Mol Sci (2015) 16:7493–519. doi: 10.3390/ijms16047493
7. Sherman MP, Zaghouani H, Niklas V. Gut microbiota, the immune system, and diet influence the neonatal gut–brain axis. Pediatr Res (2015) 77:127–35. doi: 10.1038/pr.2014.161
8. Martin AM, Sun EW, Rogers GB, Keating DJ. The influence of the gut microbiome on host metabolism through the regulation of gut hormone release. Front Physiol (2019) 10:428. doi: 10.3389/fphys.2019.00428
9. Olfson M, Marcus SC. National patterns in antidepressant medication treatment. Arch Gen Psychiatry (2009) 66:848–56. doi: 10.1001/archgenpsychiatry.2009.81
10. Alduhishy M. The overprescription of antidepressants and its impact on the elderly in Australia. Trends Psychiatry Psychother (2018) 40:241–3. doi: 10.1590/2237-6089-2016-0077
11. Yohn CN, Gergues MM, Samuels BA. The role of 5-HT receptors in depression. Mol Brain (2017) 10:1–12. doi: 10.1186/s13041-017-0306-y
12. Turecki G, Brent DA, Gunnell D, O’connor RC, Oquendo MA, Pirkis J, et al. Suicide and suicide risk. Nat Rev Dis Primers (2019) 5:74. doi: 10.1038/s41572-019-0121-0
13. Siopi E, Chevalier G, Katsimpardi L, Saha S, Bigot M, Moigneu C, et al. Changes in gut microbiota by chronic stress impair the efficacy of fluoxetine. Cell Rep (2020) 30:3682–3690. e3686. doi: 10.1016/j.celrep.2020.02.099
14. Łoniewski I, Misera A, Skonieczna-Żydecka K, Kaczmarczyk M, Kaźmierczak-Siedlecka K, Misiak B, et al. Major depressive disorder and gut microbiota–association not causation. A scoping review. Prog Neuropsychopharmacol Biol Psychiatry (2021) 106:110111. doi: 10.1016/j.pnpbp.2020.110111
15. Klengel T, Binder EB. Gene—environment interactions in major depressive disorder. Can J Psychiatry (2013) 58:76–83. doi: 10.1177/070674371305800203
16. Bowes L, Joinson C, Wolke D, Lewis G. Peer victimisation during adolescence and its impact on depression in early adulthood: prospective cohort study in the United Kingdom. bmj (2015) 350. doi: 10.1136/bmj.h2469
17. Gao J, Zhao L, Cheng Y, Lei W, Wang Y, Liu X, et al. Probiotics for the treatment of depression and its comorbidities: A systemic review. Front Cell Infect Microbiol (2023) 13:1167116. doi: 10.3389/fcimb.2023.1167116
18. Johnson D, Letchumanan V, Thum CC, Thurairajasingam S, Lee L-H. A microbial-based approach to mental health: the potential of probiotics in the treatment of depression. Nutrients (2023) 15:1382. doi: 10.3390/nu15061382
19. Corrigan M, O’rourke AM, Moran B, Fletcher JM, Harkin A. Inflammation in the pathogenesis of depression: a disorder of neuroimmune origin. Neuronal Signaling (2023) 7. doi: 10.1042/NS20220054
20. Berk M, Williams LJ, Jacka FN, O’neil A, Pasco JA, Moylan S, et al. So depression is an inflammatory disease, but where does the inflammation come from? BMC Med (2013) 11:1–16. doi: 10.1186/1741-7015-11-200
21. Jokela M, Virtanen M, Batty GD, Kivimäki M. Inflammation and specific symptoms of depression. JAMA Psychiatry (2016) 73:87–8. doi: 10.1001/jamapsychiatry.2015.1977
22. Liu S, Guo R, Liu F, Yuan Q, Yu Y, Ren F. Gut microbiota regulates depression-like behavior in rats through the neuroendocrine-immune-mitochondrial pathway. Neuropsychiatr Dis Treat (2020), 859–69. doi: 10.2147/NDT.S243551
23. Cani PD. Human gut microbiome: hopes, threats and promises. Gut (2018) 67:1716–25. doi: 10.1136/gutjnl-2018-316723
24. Sorbara MT, Pamer EG. Microbiome-based therapeutics. Nat Rev Microbiol (2022) 20:365–80. doi: 10.1038/s41579-021-00667-9
25. Peroni DG, Nuzzi G, Trambusti I, Di Cicco ME, Comberiati P. Microbiome composition and its impact on the development of allergic diseases. Front Immunol (2020) 11:700. doi: 10.3389/fimmu.2020.00700
26. Mahowald MA, Rey FE, Seedorf H, Turnbaugh PJ, Fulton RS, Wollam A, et al. Characterizing a model human gut microbiota composed of members of its two dominant bacterial phyla. Proc Natl Acad Sci (2009) 106:5859–64. doi: 10.1073/pnas.0901529106
27. Ursell LK, Metcalf JL, Parfrey LW, Knight R. Defining the human microbiome. Nutr Rev (2012) 70:S38–44. doi: 10.1111/j.1753-4887.2012.00493.x
28. Pickard JM, Zeng MY, Caruso R, Núñez G. Gut microbiota: Role in pathogen colonization, immune responses, and inflammatory disease. Immunol Rev (2017) 279:70–89. doi: 10.1111/imr.12567
29. Ventura M, O’toole PW, De Vos WM, Van Sinderen D. Selected aspects of the human gut microbiota. Cell Mol Life Sci (2018) 75:81–2. doi: 10.1007/s00018-017-2669-8
30. Vernocchi P, Del Chierico F, Putignani L. Gut microbiota metabolism and interaction with food components. Int J Mol Sci (2020) 21:3688. doi: 10.3390/ijms21103688
31. Kim IB, Park S-C, Kim Y-K. Microbiota-Gut-Brain Axis in Major Depression: A New Therapeutic Approach. In: Neuroinflammation, Gut-Brain Axis and Immunity in Neuropsychiatric Disorders. Springer (2023). p. 209–24.
32. Liu L, Wang H, Chen X, Zhang Y, Zhang H, Xie P. Gut microbiota and its metabolites in depression: from pathogenesis to treatment. EBioMedicine (2023) 90:104527. doi: 10.1016/j.ebiom.2023.104527
33. Troubat R, Barone P, Leman S, Desmidt T, Cressant A, Atanasova B, et al. Neuroinflammation and depression: A review. Eur J Neurosci (2021) 53:151–71. doi: 10.1111/ejn.14720
34. Tartt AN, Mariani MB, Hen R, Mann JJ, Boldrini M. Dysregulation of adult hippocampal neuroplasticity in major depression: pathogenesis and therapeutic implications. Mol Psychiatry (2022) 27:2689–99. doi: 10.1038/s41380-022-01520-y
35. Zhang Z, Huang P, Li S, Liu Z, Zhang J, Liu Z. Neural mechanisms underlying the processing of emotional stimuli in individuals with depression: An ALE meta-analysis study. Psychiatry Res (2022) 313:114598. doi: 10.1016/j.psychres.2022.114598
36. Zaidi S, Ali K, Khan AU. It’s all relative: analyzing microbiome compositions, its significance, pathogenesis and microbiota derived biofilms: Challenges and opportunities for disease intervention. Arch Microbiol (2023) 205:257. doi: 10.1007/s00203-023-03589-7
37. Vickers NJ. Animal communication: when i’m calling you, will you answer too? Curr Biol (2017) 27:R713–5. doi: 10.1016/j.cub.2017.05.064
38. Averina OV, Zorkina YA, Yunes RA, Kovtun AS, Ushakova VM, Morozova AY, et al. Bacterial metabolites of human gut microbiota correlating with depression. Int J Mol Sci (2020) 21:9234. doi: 10.3390/ijms21239234
39. Sarkar A, Lehto SM, Harty S, Dinan TG, Cryan JF, Burnet PW. Psychobiotics and the manipulation of bacteria–gut–brain signals. Trends Neurosci (2016) 39:763–81. doi: 10.1016/j.tins.2016.09.002
40. Chudzik A, Orzyłowska A, Rola R, Stanisz GJ. Probiotics, prebiotics and postbiotics on mitigation of depression symptoms: Modulation of the brain–gut–microbiome axis. Biomolecules (2021) 11:1000. doi: 10.3390/biom11071000
41. Cryan JF, O’mahony SM. The microbiome-gut-brain axis: from bowel to behavior. Neurogastroenterol Motil (2011) 23:187–92. doi: 10.1111/j.1365-2982.2010.01664.x
42. Cryan JF, O’riordan KJ, Cowan CS, Sandhu KV, Bastiaanssen TF, Boehme M, et al. The microbiota-gut-brain axis. Physiol Rev (2019).
43. Montanari M, Imbriani P, Bonsi P, Martella G, Peppe A. Beyond the microbiota: understanding the role of the enteric nervous system in Parkinson’s disease from mice to human. Biomedicines (2023) 11:1560. doi: 10.3390/biomedicines11061560
44. Sekirov I, Russell SL, Antunes LCM, Finlay BB. Gut microbiota in health and disease. Physiol Rev (2010). doi: 10.1152/physrev.00045.2009
45. Foster JA, Neufeld K-a. Gut–brain axis: how the microbiome influences anxiety and depression. Trends Neurosci (2013) 36:305–12. doi: 10.1016/j.tins.2013.01.005
46. Karlsson FH, Ussery DW, Nielsen J, Nookaew I. A closer look at bacteroides: phylogenetic relationship and genomic implications of a life in the human gut. Microbial Ecol (2011) 61:473–85. doi: 10.1007/s00248-010-9796-1
47. Arzani M, Jahromi SR, Ghorbani Z, Vahabizad F, Martelletti P, Ghaemi A, et al. Gut-brain axis and migraine headache: a comprehensive review. J headache Pain (2020) 21:1–12. doi: 10.1186/s10194-020-1078-9
48. Yang J, Zheng P, Li Y, Wu J, Tan X, Zhou J, et al. Landscapes of bacterial and metabolic signatures and their interaction in major depressive disorders. Sci Adv (2020) 6:eaba8555. doi: 10.1126/sciadv.aba8555
49. Nikolova VL, Smith MR, Hall LJ, Cleare AJ, Stone JM, Young AH. Perturbations in gut microbiota composition in psychiatric disorders: a review and meta-analysis. JAMA Psychiatry (2021) 78:1343–54. doi: 10.1001/jamapsychiatry.2021.2573
50. Hernández-Mendoza A, González-Córdova AF, Martínez-Porchas M. Influence of probiotics on the animal gut microbiota and their impact on the bioavailability of toxic agents: an opinion paper. Front Nutr (2022) 9:870162. doi: 10.3389/fnut.2022.870162
51. Liu L, Wang H, Zhang H, Chen X, Zhang Y, Wu J, et al. Toward a deeper understanding of gut microbiome in depression: the promise of clinical applicability. Adv Sci (Weinh) (2022) 9:e2203707. doi: 10.1002/advs.202203707
52. Carabotti M, Scirocco A, Maselli MA, Severi C. The gut-brain axis: interactions between enteric microbiota, central and enteric nervous systems. Ann Gastroenterol (2015) 28:203–9.
53. Singh S, Sharma P, Pal N, Kumawat M, Shubham S, Sarma DK, et al. Impact of environmental pollutants on gut microbiome and mental health via the gut-brain axis. Microorganisms (2022) 10. doi: 10.3390/microorganisms10071457
54. Hattori N, Yamashiro Y. The gut-brain axis. Ann Nutr Metab (2021) 77:1–3. doi: 10.1159/000512226
55. Wang W, Qi F, Wipf D, Cai C, Yu T, Li Y, et al. Sparse bayesian learning for end-to-end EEG decoding. IEEE Trans Pattern Anal Mach Intelligence. (2023). doi: 10.1109/TPAMI.2023.3299568
56. Alvarez-Mon MA, Gómez AM, Orozco A, Lahera G, Sosa MD, Diaz D, et al. Abnormal distribution and function of circulating monocytes and enhanced bacterial translocation in major depressive disorder. Front Psychiatry (2019) 10:812. doi: 10.3389/fpsyt.2019.00812
57. Alvarez-Mon MA, Gómez-Lahoz AM, Orozco A, Lahera G, Diaz D, Ortega MA, et al. Expansion of CD4 T lymphocytes expressing interleukin 17 and tumor necrosis factor in patients with major depressive disorder. J Personal Med (2021) 11:220. doi: 10.3390/jpm11030220
58. Alvarez-Mon MA, Gomez-Lahoz AM, Orozco A, Lahera G, Sosa-Reina MD, Diaz D, et al. Blunted expansion of regulatory T lymphocytes is associated with increased bacterial translocation in patients with major depressive disorder. Front Psychiatry (2021) 11:591962. doi: 10.3389/fpsyt.2020.591962
59. Wu Y, Zheng Y, Wang X, Tang P, Guo W, Ma H, et al. Ginseng-containing sijunzi decoction ameliorates ulcerative colitis by orchestrating gut homeostasis in microbial modulation and intestinal barrier integrity. Am J Chin Med (2023) 51:677–99. doi: 10.1142/S0192415X23500325
60. Ley RE, Bäckhed F, Turnbaugh P, Lozupone CA, Knight RD, Gordon JI. Obesity alters gut microbial ecology. Proc Natl Acad Sci (2005) 102:11070–5. doi: 10.1073/pnas.0504978102
61. Stojanov S, Berlec A, Štrukelj B. The influence of probiotics on the firmicutes/bacteroidetes ratio in the treatment of obesity and inflammatory bowel disease. Microorganisms (2020) 8:1715. doi: 10.3390/microorganisms8111715
62. Yang C, Xu J, Xu X, Xu W, Tong B, Wang S, et al. Characteristics of gut microbiota in patients with metabolic associated fatty liver disease. Sci Rep (2023) 13:9988. doi: 10.1038/s41598-023-37163-4
63. Jiang HY, Pan LY, Zhang X, Zhang Z, Zhou YY, Ruan B. Altered gut bacterial–fungal interkingdom networks in patients with current depressive episode. Brain Behav (2020) 10:e01677. doi: 10.1002/brb3.1677
64. McMillan A. Utilizing Untargeted Metabolomics to Characterize Microbial Communities and Identify Biomarkers of an Unhealthy State. The University of Western Ontario (Canada (2016).
65. Abenavoli L, Scarpellini E, Colica C, Boccuto L, Salehi B, Sharifi-Rad J, et al. Gut microbiota and obesity: a role for probiotics. Nutrients (2019) 11:2690. doi: 10.3390/nu11112690
66. Balaguer-Trias J, Deepika D, Schuhmacher M, Kumar V. Impact of contaminants on microbiota: linking the gut–brain axis with neurotoxicity. Int J Environ Res Public Health (2022) 19:1368. doi: 10.3390/ijerph19031368
67. Silva YP, Bernardi A, Frozza RL. The role of short-chain fatty acids from gut microbiota in gut-brain communication. Front Endocrinol (2020) 11:25. doi: 10.3389/fendo.2020.00025
68. Morrison DJ, Preston T. Formation of short chain fatty acids by the gut microbiota and their impact on human metabolism. Gut Microbes (2016) 7:189–200. doi: 10.1080/19490976.2015.1134082
69. Portincasa P, Bonfrate L, Vacca M, De Angelis M, Farella I, Lanza E, et al. Gut microbiota and short chain fatty acids: implications in glucose homeostasis. Int J Mol Sci (2022) 23:1105. doi: 10.3390/ijms23031105
70. Del Toro-Barbosa M, Hurtado-Romero A, Garcia-Amezquita LE, García-Cayuela T. Psychobiotics: mechanisms of action, evaluation methods and effectiveness in applications with food products. Nutrients (2020) 12:3896. doi: 10.3390/nu12123896
71. Perry RJ, Lee S, Ma L, Zhang D, Schlessinger J, Shulman GI. FGF1 and FGF19 reverse diabetes by suppression of the hypothalamic–pituitary–adrenal axis. Nat Commun (2015) 6:6980. doi: 10.1038/ncomms7980
72. Psichas A, Sleeth M, Murphy K, Brooks L, Bewick G, Hanyaloglu A, et al. The short chain fatty acid propionate stimulates GLP-1 and PYY secretion via free fatty acid receptor 2 in rodents. Int J Obes (2015) 39:424–9. doi: 10.1038/ijo.2014.153
73. Strati F, Cavalieri D, Albanese D, De Felice C, Donati C, Hayek J, et al. Altered gut microbiota in Rett syndrome. Microbiome (2016) 4:1–15. doi: 10.1186/s40168-016-0185-y
74. Jacka FN, O’neil A, Opie R, Itsiopoulos C, Cotton S, Mohebbi M, et al. A randomised controlled trial of dietary improvement for adults with major depression (the ‘SMILES’trial). BMC Med (2017) 15:1–13. doi: 10.1186/s12916-017-0791-y
75. Maes M, Vasupanrajit A, Jirakran K, Klomkliew P, Chanchaem P, Tunvirachaisakul C, et al. Adverse childhood experiences and reoccurrence of illness impact the gut microbiome, which affects suicidal behaviours and the phenome of major depression: towards enterotypic phenotypes. Acta Neuropsychiatr (2023) 35:328–45. doi: 10.1017/neu.2023.21
76. Zainal NH, Newman MG. Prospective network analysis of proinflammatory proteins, lipid markers, and depression components in midlife community women. Psychol Med (2023) 53:5267–78. doi: 10.1017/S003329172200232X
77. Brydges CR, Bhattacharyya S, Dehkordi SM, Milaneschi Y, Penninx B, Jansen R, et al. Metabolomic and inflammatory signatures of symptom dimensions in major depression. Brain Behav Immun (2022) 102:42–52. doi: 10.1016/j.bbi.2022.02.003
78. Jakobsson HE, Jernberg C, Andersson AF, Sjölund-Karlsson M, Jansson JK, Engstrand L. Short-term antibiotic treatment has differing long-term impacts on the human throat and gut microbiome. PloS One (2010) 5:e9836. doi: 10.1371/journal.pone.0009836
79. Degruttola AK, Low D, Mizoguchi A, Mizoguchi E. Current understanding of dysbiosis in disease in human and animal models. Inflammatory bowel Dis (2016) 22:1137–50. doi: 10.1097/MIB.0000000000000750
80. Bisanz JE, Upadhyay V, Turnbaugh JA, Ly K, Turnbaugh PJ. Meta-analysis reveals reproducible gut microbiome alterations in response to a high-fat diet. Cell Host Microbe (2019) 26:265–272. e264. doi: 10.1016/j.chom.2019.06.013
81. Ju M, Liu Y, Li M, Cheng M, Zhang Y, Deng G, et al. Baicalin improves intestinal microecology and abnormal metabolism induced by high-fat diet. Eur J Pharmacol (2019) 857:172457. doi: 10.1016/j.ejphar.2019.172457
82. Fan S, Chen S, Lin L. Research progress of gut microbiota and obesity caused by high-fat diet. Front Cell Infect Microbiol (2023) 13:1139800. doi: 10.3389/fcimb.2023.1139800
83. Rodríguez JM, Murphy K, Stanton C, Ross RP, Kober OI, Juge N, et al. The composition of the gut microbiota throughout life, with an emphasis on early life. Microbial Ecol Health Dis (2015) 26:26050. doi: 10.3402/mehd.v26.26050
84. Cong X, Xu W, Romisher R, Poveda S, Forte S, Starkweather A, et al. Focus: Microbiome: Gut microbiome and infant health: Brain-gut-microbiota axis and host genetic factors. Yale J Biol Med (2016) 89:299.
85. Tsiaoussis J, Antoniou MN, Koliarakis I, Mesnage R, Vardavas CI, Izotov BN, et al. Effects of single and combined toxic exposures on the gut microbiome: Current knowledge and future directions. Toxicol Lett (2019) 312:72–97. doi: 10.1016/j.toxlet.2019.04.014
86. Keunen K, Van Elburg RM, Van Bel F, Benders MJ. Impact of nutrition on brain development and its neuroprotective implications following preterm birth. Pediatr Res (2015) 77:148–55. doi: 10.1038/pr.2014.171
87. Clapp M, Aurora N, Herrera L, Bhatia M, Wilen E, Wakefield S. Gut microbiota’s effect on mental health: The gut-brain axis. Clinics Pract (2017) 7:987. doi: 10.4081/cp.2017.987
88. Moulton CD, Pavlidis P, Norton C, Norton S, Pariante C, Hayee B, et al. Depressive symptoms in inflammatory bowel disease: an extraintestinal manifestation of inflammation? Clin Exp Immunol (2019) 197:308–18. doi: 10.1111/cei.13276
89. Limbana T, Khan F, Eskander N. Gut microbiome and depression: how microbes affect the way we think. Cureus (2020) 12:e9966. doi: 10.7759/cureus.9966
90. Radford-Smith DE, Anthony DC. Prebiotic and probiotic modulation of the microbiota–gut–brain axis in depression. Nutrients (2023) 15:1880. doi: 10.3390/nu15081880
91. Nunes PPB. Evidence from clinical studies of the nutrological modulation of the gut microbiota in inflammatory bowel diseases: a systematic review. Int J Nutrol (2023) 16. doi: 10.54448/ijn23204
92. Dong Z, Xie Q, Shen X, Hao Y, Li J, Xu H, et al. Strain-level structure of gut microbiome associated with the cognitive function in major depressive disorder. (2022). doi: 10.21203/rs.3.rs-1796347/v1
93. Villa T, Sánchez-Pérez A. The gut microbiome affects human mood and behavior. Dev Biol Prokaryotes Lower Eukaryotes (2021), 541–65. doi: 10.1007/978-3-030-77595-7_22
94. Chen J-J, He S, Fang L, Wang B, Bai S-J, Xie J, et al. Age-specific differential changes on gut microbiota composition in patients with major depressive disorder. Aging (Albany NY) (2020) 12:2764.
95. Martino C, Dilmore AH, Burcham ZM, Metcalf JL, Jeste D, Knight R. Microbiota succession throughout life from the cradle to the grave. Nat Rev Microbiol (2022) 20:707–20. doi: 10.1038/s41579-022-00768-z
96. Todeva-Radneva A, Kandilarova S, Paunova R, Stoyanov D, Zdravkova T, Sladky R. Functional connectivity of the anterior cingulate cortex and the right anterior insula differentiates between major depressive disorder, bipolar disorder and healthy controls. Biomedicines (2023) 11:1608. doi: 10.3390/biomedicines11061608
97. Anguzu R, Nagavally S, Dawson AZ, Walker RJ, Egede LE. Age and gender differences in trends and impact of depression on quality of life in the United States 2008 to 2016. Women’s Health Issues (2021) 31:353–65. doi: 10.1016/j.whi.2021.02.005
98. Thapar A, Eyre O, Patel V, Brent D. Depression in young people. Lancet (2022) 400:617–31. doi: 10.1016/S0140-6736(22)01012-1
99. Stower H. Depression linked to the microbiome. Nat Med (2019) 25:358–8. doi: 10.1038/s41591-019-0396-4
100. Valles-Colomer M, Falony G, Darzi Y, Tigchelaar EF, Wang J, Tito RY, et al. The neuroactive potential of the human gut microbiota in quality of life and depression. Nat Microbiol (2019) 4:623–32. doi: 10.1038/s41564-018-0337-x
101. Bo Q, Tian L, Li F, Mao Z, Wang Z, Ma X, et al. Quality of life in euthymic patients with unipolar major depressive disorder and bipolar disorder. Neuropsychiatr Dis Treat (2019), 1649–57. doi: 10.2147/NDT.S201567
102. Rhee SJ, Kim H, Lee Y, Lee HJ, Park CHK, Yang J, et al. The association between serum microbial DNA composition and symptoms of depression and anxiety in mood disorders. Sci Rep (2021) 11:13987. doi: 10.1038/s41598-021-93112-z
103. Park A, Collins J, Blennerhassett P, Ghia J, Verdu E, Bercik P, et al. Altered colonic function and microbiota profile in a mouse model of chronic depression. Neurogastroenterol Motil (2013) 25:733–e575. doi: 10.1111/nmo.12153
104. Bailey MT, Dowd SE, Galley JD, Hufnagle AR, Allen RG, Lyte M. Exposure to a social stressor alters the structure of the intestinal microbiota: implications for stressor-induced immunomodulation. Brain behavior Immun (2011) 25:397–407. doi: 10.1016/j.bbi.2010.10.023
105. Sudo N, Chida Y, Aiba Y, Sonoda J, Oyama N, Yu XN, et al. Postnatal microbial colonization programs the hypothalamic–pituitary–adrenal system for stress response in mice. J Physiol (2004) 558:263–75. doi: 10.1113/jphysiol.2004.063388
106. Clarke G, Grenham S, Scully P, Fitzgerald P, Moloney R, Shanahan F, et al. The microbiome-gut-brain axis during early life regulates the hippocampal serotonergic system in a sex-dependent manner. Mol Psychiatry (2013) 18:666–73. doi: 10.1038/mp.2012.77
107. Ogbonnaya ES, Clarke G, Shanahan F, Dinan TG, Cryan JF, O’leary OF. Adult hippocampal neurogenesis is regulated by the microbiome. Biol Psychiatry (2015) 78:e7–9. doi: 10.1016/j.biopsych.2014.12.023
108. Yano JM, Yu K, Donaldson GP, Shastri GG, Ann P, Ma L, et al. Indigenous bacteria from the gut microbiota regulate host serotonin biosynthesis. Cell (2015) 161:264–76. doi: 10.1016/j.cell.2015.02.047
109. Bruce-Keller AJ, Salbaum JM, Luo M, Blanchard Iv E, Taylor CM, Welsh DA, et al. Obese-type gut microbiota induce neurobehavioral changes in the absence of obesity. Biol Psychiatry (2015) 77:607–15. doi: 10.1016/j.biopsych.2014.07.012
110. Bravo JA, Forsythe P, Chew MV, Escaravage E, Savignac HM, Dinan TG, et al. Ingestion of Lactobacillus strain regulates emotional behavior and central GABA receptor expression in a mouse via the vagus nerve. Proc Natl Acad Sci (2011) 108:16050–5. doi: 10.1073/pnas.1102999108
111. Messaoudi M, Lalonde R, Violle N, Javelot H, Desor D, Nejdi A, et al. Assessment of psychotropic-like properties of a probiotic formulation (Lactobacillus helveticus R0052 and Bifidobacterium longum R0175) in rats and human subjects. Br J Nutr (2011) 105:755–64. doi: 10.1017/S0007114510004319
112. Savignac H, Tramullas M, Kiely B, Dinan T, Cryan J. Bifidobacteria modulate cognitive processes in an anxious mouse strain. Behav Brain Res (2015) 287:59–72. doi: 10.1016/j.bbr.2015.02.044
113. Naseribafrouei A, Hestad K, Avershina E, Sekelja M, Linløkken A, Wilson R, et al. Correlation between the human fecal microbiota and depression. Neurogastroenterol Motil (2014) 26:1155–62. doi: 10.1111/nmo.12378
114. Jiang H, Ling Z, Zhang Y, Mao H, Ma Z, Yin Y, et al. Altered fecal microbiota composition in patients with major depressive disorder. Brain behavior Immun (2015) 48:186–94. doi: 10.1016/j.bbi.2015.03.016
115. Shkoporov AN, Turkington CJ, Hill C. Mutualistic interplay between bacteriophages and bacteria in the human gut. Nat Rev Microbiol (2022) 20:737–49. doi: 10.1038/s41579-022-00755-4
116. Talapko J, Juzbašić M, Matijević T, Pustijanac E, Bekić S, Kotris I, et al. Candida albicans—the virulence factors and clinical manifestations of infection. J Fungi (2021) 7:79. doi: 10.3390/jof7020079
117. Ng KM, Ferreyra JA, Higginbottom SK, Lynch JB, Kashyap PC, Gopinath S, et al. Microbiota-liberated host sugars facilitate post-antibiotic expansion of enteric pathogens. Nature (2013) 502:96–9. doi: 10.1038/nature12503
118. Roca-Saavedra P, Mendez-Vilabrille V, Miranda JM, Nebot C, Cardelle-Cobas A, Franco CM, et al. Food additives, contaminants and other minor components: Effects on human gut microbiota—A review. J Physiol Biochem (2018) 74:69–83. doi: 10.1007/s13105-017-0564-2
119. Shanahan F, Ghosh TS, Molloy MG, O’toole PW. The nonindustrialised microbiome in a modern world. Clin Sci (2022) 136:1683–90. doi: 10.1042/CS20220203
120. Vishnempet Shridhar S, Beghini F, Alexander M, Brito IL, Christakis NA. Environmental, socioeconomic, and health factors associated with gut microbiome species and strains in isolated Honduras villages. medRxiv (2023) 2004:23288404.
121. Zhao J, Zhang Q, Cheng W, Dai Q, Wei Z, Guo M, et al. Heart–gut microbiota communication determines the severity of cardiac injury after myocardial ischaemia/reperfusion. Cardiovasc Res (2023), cvad023. doi: 10.1093/cvr/cvad023
122. Garrido-Mesa N, Zarzuelo A, Gálvez J. Minocycline: far beyond an antibiotic. Br J Pharmacol (2013) 169:337–52. doi: 10.1111/bph.12139
123. Kuo P-H, Chung Y-CE. Moody microbiome: Challenges and chances. J Formosan Med Assoc (2019) 118:S42–54. doi: 10.1016/j.jfma.2018.09.004
124. Nettis MA. Minocycline in Major Depressive Disorder: and overview with considerations on treatment-resistance and comparisons with other psychiatric disorders. Brain behavior immunity-health (2021) 17:100335. doi: 10.1016/j.bbih.2021.100335
125. Cussotto S, Clarke G, Dinan TG, Cryan JF. Psychotropic drugs and the microbiome. Microbes Mind (2021) 32:113–33. doi: 10.1159/000510423
126. Dinan K, Dinan T. Antibiotics and mental health: The good, the bad and the ugly. J Internal Med (2022) 292:858–69. doi: 10.1111/joim.13543
127. Freitas AC, Chaban B, Bocking A, Rocco M, Yang S, Hill JE, et al. The vaginal microbiome of pregnant women is less rich and diverse, with lower prevalence of Mollicutes, compared to non-pregnant women. Sci Rep (2017) 7:9212. doi: 10.1038/s41598-017-07790-9
128. Braye K, Foureur M, De Waal K, Jones M, Putt E, Ferguson J. Group B streptococcal screening, intrapartum antibiotic prophylaxis, and neonatal early-onset infection rates in an Australian local health district: 2006-2016. PloS One (2019) 14:e0214295. doi: 10.1371/journal.pone.0214295
129. Patangia DV, Anthony Ryan C, Dempsey E, Paul Ross R, Stanton C. Impact of antibiotics on the human microbiome and consequences for host health. MicrobiologyOpen (2022) 11:e1260. doi: 10.1002/mbo3.1260
130. Daniali M, Nikfar S, Abdollahi M. Antibiotic resistance propagation through probiotics. Expert Opin Drug Metab Toxicol (2020) 16:1207–15. doi: 10.1080/17425255.2020.1825682
131. Godoy LD, Rossignoli MT, Delfino-Pereira P, Garcia-Cairasco N, De Lima Umeoka EH. A comprehensive overview on stress neurobiology: basic concepts and clinical implications. Front Behav Neurosci (2018) 12:127. doi: 10.3389/fnbeh.2018.00127
132. Tan SY, Yip A. Hans Selye, (1907–1982): Founder of the stress theory. Singapore Med J (2018) 59:170. doi: 10.11622/smedj.2018043
133. Cohen S, Janicki-Deverts D, Miller GE. Psychological stress and disease. Jama (2007) 298:1685–7. doi: 10.1001/jama.298.14.1685
134. Alexopoulos GS. Mechanisms and treatment of late-life depression. Trans Psychiatry (2019) 9:188. doi: 10.1038/s41398-019-0514-6
135. Rohleder N. Stimulation of systemic low-grade inflammation by psychosocial stress. Psychosomatic Med (2014) 76:181–9. doi: 10.1097/PSY.0000000000000049
136. Furman D, Campisi J, Verdin E, Carrera-Bastos P, Targ S, Franceschi C, et al. Chronic inflammation in the etiology of disease across the life span. Nat Med (2019) 25:1822–32. doi: 10.1038/s41591-019-0675-0
137. Hantsoo L, Kornfield S, Anguera MC, Epperson CN. Inflammation: a proposed intermediary between maternal stress and offspring neuropsychiatric risk. Biol Psychiatry (2019) 85:97–106. doi: 10.1016/j.biopsych.2018.08.018
138. Rohleder N. Stress and inflammation–The need to address the gap in the transition between acute and chronic stress effects. Psychoneuroendocrinology (2019) 105:164–71. doi: 10.1016/j.psyneuen.2019.02.021
139. Viljoen M. Low-grade systemic inflammation and the workplace. Work (2021) 69:903–15. doi: 10.3233/WOR-213523
140. Krishnan V, Nestler EJ. Animal models of depression: molecular perspectives. Mol Funct Models neuropsychiatry (2011), 121–47. doi: 10.1007/7854_2010_108
141. Planchez B, Surget A, Belzung C. Animal models of major depression: drawbacks and challenges. J Neural Transm (2019) 126:1383–408. doi: 10.1007/s00702-019-02084-y
142. Kubera M, Obuchowicz E, Goehler L, Brzeszcz J, Maes M. In animal models, psychosocial stress-induced (neuro) inflammation, apoptosis and reduced neurogenesis are associated to the onset of depression. Prog Neuropsychopharmacol Biol Psychiatry (2011) 35:744–59. doi: 10.1016/j.pnpbp.2010.08.026
143. Rink L, Kruse A, Haase H, Rink L, Kruse A, Haase H. Die Regulation des Immunsystems und immunprivilegierte Organe. Immunol für Einsteiger (2012), 119–42. doi: 10.1007/978-3-8274-2440-2_7
144. Breitbart W, Rosenfeld B, Tobias K, Pessin H, Ku GY, Yuan J, et al. Depression, cytokines, and pancreatic cancer. Psycho-oncology (2014) 23:339–45. doi: 10.1002/pon.3422
145. Leonard WJ, Wan C-K. IL-21 signaling in immunity. F1000Research (2016) 5. doi: 10.12688/f1000research.7634.1
146. Caraci F, Spampinato SF, Morgese MG, Tascedda F, Salluzzo MG, Giambirtone MC, et al. Neurobiological links between depression and AD: the role of TGF-β1 signaling as a new pharmacological target. Pharmacol Res (2018) 130:374–84. doi: 10.1016/j.phrs.2018.02.007
147. Himmerich H, Patsalos O, Lichtblau N, Ibrahim MA, Dalton B. Cytokine research in depression: principles, challenges, and open questions. Front Psychiatry (2019) 10:30. doi: 10.3389/fpsyt.2019.00030
148. Valkanova V, Ebmeier KP, Allan CL. CRP, IL-6 and depression: a systematic review and meta-analysis of longitudinal studies. J Affect Disord (2013) 150:736–44. doi: 10.1016/j.jad.2013.06.004
149. Horn SR, Long MM, Nelson BW, Allen NB, Fisher PA, Byrne ML. Replication and reproducibility issues in the relationship between C-reactive protein and depression: A systematic review and focused meta-analysis. Brain behavior Immun (2018) 73:85–114. doi: 10.1016/j.bbi.2018.06.016
150. Anderson G, Berk M, Dean O, Moylan S, Maes M. Role of immune-inflammatory and oxidative and nitrosative stress pathways in the etiology of depression: therapeutic implications. CNS Drugs (2014) 28:1–10. doi: 10.1007/s40263-013-0119-1
151. Vogelzangs N, Beekman AT, Van Reedt Dortland AK, Schoevers RA, Giltay EJ, De Jonge P, et al. Inflammatory and metabolic dysregulation and the 2-year course of depressive disorders in antidepressant users. Neuropsychopharmacology (2014) 39:1624–34. doi: 10.1038/npp.2014.9
152. Jacka FN. Nutritional psychiatry: where to next? EBioMedicine (2017) 17:24–9. doi: 10.1016/j.ebiom.2017.02.020
153. Jacka FN, Maes M, Pasco JA, Williams LJ, Berk M. Nutrient intakes and the common mental disorders in women. J Affect Disord (2012) 141:79–85. doi: 10.1016/j.jad.2012.02.018
154. O’neil A, Quirk SE, Housden S, Brennan SL, Williams LJ, Pasco JA, et al. Relationship between diet and mental health in children and adolescents: a systematic review. Am J Public Health (2014) 104:e31–42. doi: 10.2105/AJPH.2014.302110
155. Bear TL, Dalziel JE, Coad J, Roy NC, Butts CA, Gopal PK. The role of the gut microbiota in dietary interventions for depression and anxiety. Adv Nutr (2020) 11:890–907. doi: 10.1093/advances/nmaa016
156. Su K-P, Matsuoka Y, Pae C-U. Omega-3 polyunsaturated fatty acids in prevention of mood and anxiety disorders. Clin Psychopharmacol Neurosci (2015) 13:129. doi: 10.9758/cpn.2015.13.2.129
157. Ekinci GN, Sanlier N. The relationship between nutrition and depression in the life process: A mini-review. Exp Gerontol (2022), 112072.
158. Ortega MA, Fraile-Martínez Ó, García-Montero C, Alvarez-Mon MA, Lahera G, Monserrat J, et al. Biological role of nutrients, food and dietary patterns in the prevention and clinical management of major depressive disorder. Nutrients (2022) 14:3099. doi: 10.3390/nu14153099
159. Dominguez LJ, Di Bella G, Veronese N, Barbagallo M. Impact of Mediterranean diet on chronic non-communicable diseases and longevity. Nutrients (2021) 13:2028. doi: 10.3390/nu13062028
160. Ventriglio A, Sancassiani F, Contu MP, Latorre M, Di Salvatore M, Fornaro M, et al. Erratum: mediterranean diet and its benefits on health and mental health: A literature review. Clin Pract Epidemiol Ment Health: CP EMH (2021) 17:9–9. doi: 10.2174/1745017902117010009
161. Chen Y, Xu J, Chen Y. Regulation of neurotransmitters by the gut microbiota and effects on cognition in neurological disorders. Nutrients (2021) 13:2099.
162. Galland L. The gut microbiome and the brain. J med Food (2014) 17:1261–72. doi: 10.1089/jmf.2014.7000
163. Williams BB, Van Benschoten AH, Cimermancic P, Donia MS, Zimmermann M, Taketani M, et al. Discovery and characterization of gut microbiota decarboxylases that can produce the neurotransmitter tryptamine. Cell Host Microbe (2014) 16:495–503. doi: 10.1016/j.chom.2014.09.001
164. Strandwitz P. Neurotransmitter modulation by the gut microbiota. Brain Res (2018) 1693:128–33. doi: 10.1016/j.brainres.2018.03.015
165. Miri S, Yeo J, Abubaker S, Hammami R. Neuromicrobiology, an emerging neurometabolic facet of the gut microbiome? Front Microbiol (2023) 14:1098412. doi: 10.3389/fmicb.2023.1098412
166. Nicolas GR, Chang PV. Deciphering the chemical lexicon of host–gut microbiota interactions. Trends Pharmacol Sci (2019) 40:430–45. doi: 10.1016/j.tips.2019.04.006
167. Filosa S, Di Meo F, Crispi S. Polyphenols-gut microbiota interplay and brain neuromodulation. Neural regen Res (2018) 13:2055. doi: 10.4103/1673-5374.241429
168. Caspani G, Kennedy S, Foster JA, Swann J. Gut microbial metabolites in depression: understanding the biochemical mechanisms. Microbial Cell (2019) 6:454. doi: 10.15698/mic2019.10.693
169. Van De Wouw M, Boehme M, Lyte JM, Wiley N, Strain C, O’sullivan O, et al. Short-chain fatty acids: microbial metabolites that alleviate stress-induced brain–gut axis alterations. J Physiol (2018) 596:4923–44. doi: 10.1113/JP276431
170. Kelly JR, Borre Y, O’brien C, Patterson E, El Aidy S, Deane J, et al. Transferring the blues: depression-associated gut microbiota induces neurobehavioural changes in the rat. J Psychiatr Res (2016) 82:109–18. doi: 10.1016/j.jpsychires.2016.07.019
171. Gebrayel P, Nicco C, Al Khodor S, Bilinski J, Caselli E, Comelli EM, et al. Microbiota medicine: Towards clinical revolution. J Trans Med (2022) 20:1–20. doi: 10.1186/s12967-022-03296-9
172. Rogers G, Keating DJ, Young RL, Wong M-L, Licinio J, Wesselingh S. From gut dysbiosis to altered brain function and mental illness: mechanisms and pathways. Mol Psychiatry (2016) 21:738–48. doi: 10.1038/mp.2016.50
173. Jesulola E, Micalos P, Baguley IJ. Understanding the pathophysiology of depression: From monoamines to the neurogenesis hypothesis model-are we there yet? Behav Brain Res (2018) 341:79–90. doi: 10.1016/j.bbr.2017.12.025
174. Szczesniak O, Hestad KA, Hanssen JF, Rudi K. Isovaleric acid in stool correlates with human depression. Nutr Neurosci (2016) 19:279–83. doi: 10.1179/1476830515Y.0000000007
175. Sandrini S, Aldriwesh M, Alruways M, Freestone P. Microbial endocrinology: host–bacteria communication within the gut microbiome. J Endocrinol (2015) 225:R21–34. doi: 10.1530/JOE-14-0615
176. Tian P, O’riordan KJ, Lee Y-K, Wang G, Zhao J, Zhang H, et al. Towards a psychobiotic therapy for depression: Bifidobacterium breve CCFM1025 reverses chronic stress-induced depressive symptoms and gut microbial abnormalities in mice. Neurobiol Stress (2020) 12:100216. doi: 10.1016/j.ynstr.2020.100216
177. Stofan M, Guo GL. Bile acids and FXR: novel targets for liver diseases. Front Med (2020) 7:544. doi: 10.3389/fmed.2020.00544
178. Di Ciaula A, Bonfrate L, Baj J, Khalil M, Garruti G, Stellaard F, et al. Recent advances in the digestive, metabolic and therapeutic effects of farnesoid X receptor and fibroblast growth factor 19: from cholesterol to bile acid signaling. Nutrients (2022) 14:4950. doi: 10.3390/nu14234950
179. Vogt NM, Romano KA, Darst BF, Engelman CD, Johnson SC, Carlsson CM, et al. The gut microbiota-derived metabolite trimethylamine N-oxide is elevated in Alzheimer’s disease. Alzheimer’s Res Ther (2018) 10:1–8.
180. Tang WW, Wang Z, Levison BS, Koeth RA, Britt EB, Fu X, et al. Intestinal microbial metabolism of phosphatidylcholine and cardiovascular risk. New Engl J Med (2013) 368:1575–84. doi: 10.1056/NEJMoa1109400
181. Bennett BJ, De Aguiar Vallim TQ, Wang Z, Shih DM, Meng Y, Gregory J, et al. Trimethylamine-N-oxide, a metabolite associated with atherosclerosis, exhibits complex genetic and dietary regulation. Cell Metab (2013) 17:49–60. doi: 10.1016/j.cmet.2012.12.011
182. Ufnal M, Pham K. The gut-blood barrier permeability–a new marker in cardiovascular and metabolic diseases? Med Hypotheses (2017) 98:35–7. doi: 10.1016/j.mehy.2016.11.012
183. Zheng P, Wang Y, Chen L, Yang D, Meng H, Zhou D, et al. Identification and validation of urinary metabolite biomarkers for major depressive disorder. Mol Cell Proteomics (2013) 12:207–14. doi: 10.1074/mcp.M112.021816
184. Meinitzer S, Baranyi A, Holasek S, Schnedl WJ, Zelzer S, Mangge H, et al. Sex-specific associations of trimethylamine-N-oxide and zonulin with signs of depression in carbohydrate malabsorbers and nonmalabsorbers. Dis Markers (2020) 2020. doi: 10.1155/2020/7897240
185. Rath S, Heidrich B, Pieper DH, Vital M. Uncovering the trimethylamine-producing bacteria of the human gut microbiota. Microbiome (2017) 5:1–14. doi: 10.1186/s40168-017-0271-9
186. Arias N, Arboleya S, Allison J, Kaliszewska A, Higarza SG, Gueimonde M, et al. The relationship between choline bioavailability from diet, intestinal microbiota composition, and its modulation of human diseases. Nutrients (2020) 12:2340. doi: 10.3390/nu12082340
187. Lukić I, Getselter D, Koren O, Elliott E. Role of tryptophan in microbiota-induced depressive-like behavior: evidence from tryptophan depletion study. Front Behav Neurosci (2019) 13:123. doi: 10.3389/fnbeh.2019.00123
188. Ortega MA, Alvarez-Mon MA, García-Montero C, Fraile-Martinez O, Guijarro LG, Lahera G, et al. Gut microbiota metabolites in major depressive disorder—Deep insights into their pathophysiological role and potential translational applications. Metabolites (2022) 12:50. doi: 10.3390/metabo12010050
189. Flint J, Kendler KS. The genetics of major depression. Neuron (2014) 81:484–503. doi: 10.1016/j.neuron.2014.01.027
190. Baselmans BML, Yengo L, Van Rheenen W, Wray NR. Risk in relatives, heritability, SNP-based heritability, and genetic correlations in psychiatric disorders: A review. Biol Psychiatry (2021) 89:11–9. doi: 10.1016/j.biopsych.2020.05.034
191. Howard DM, Adams MJ, Clarke TK, Hafferty JD, Gibson J, Shirali M, et al. Genome-wide meta-analysis of depression identifies 102 independent variants and highlights the importance of the prefrontal brain regions. Nat Neurosci (2019) 22:343–52. doi: 10.1038/s41593-018-0326-7
192. Levey DF, Stein MB, Wendt FR, Pathak GA, Zhou H, Aslan M, et al. Bi-ancestral depression GWAS in the Million Veteran Program and meta-analysis in >1.2 million individuals highlight new therapeutic directions. Nat Neurosci (2021) 24:954–63. doi: 10.1038/s41593-021-00860-2
193. Monroe SM, Anderson SF, Harkness KL. Life stress and major depression: The mysteries of recurrences. Psychol Rev (2019) 126:791–816. doi: 10.1037/rev0000157
194. Danese A, Widom CS. Objective and subjective experiences of child maltreatment and their relationships with psychopathology. Nat Hum Behav (2020) 4:811–8. doi: 10.1038/s41562-020-0880-3
195. Schiele MA, Gottschalk MG, Domschke K. The applied implications of epigenetics in anxiety, affective and stress-related disorders - A review and synthesis on psychosocial stress, psychotherapy and prevention. Clin Psychol Rev (2020) 77:101830. doi: 10.1016/j.cpr.2020.101830
196. Yang R, Gautam A, Getnet D, Daigle BJ, Miller S, Misganaw B, et al. Epigenetic biotypes of post-traumatic stress disorder in war-zone exposed veteran and active duty males. Mol Psychiatry (2021) 26:4300–14. doi: 10.1038/s41380-020-00966-2
197. Tian H, Hu Z, Xu J, Wang C. The molecular pathophysiology of depression and the new therapeutics. MedComm (2020) (2022) 3:e156. doi: 10.1002/mco2.156
198. Otte C, Gold SM, Penninx BW, Pariante CM, Etkin A, Fava M, et al. Major depressive disorder. Nat Rev Dis Primers (2016) 2:16065. doi: 10.1038/nrdp.2016.65
200. Braun PR, Han S, Hing B, Nagahama Y, Gaul LN, Heinzman JT, et al. Genome-wide DNA methylation comparison between live human brain and peripheral tissues within individuals. Transl Psychiatry (2019) 9:47. doi: 10.1038/s41398-019-0376-y
201. He J, Dai W, Li Y, He L, Huang R. Frequency of depression-related symptoms in caregivers of patients with silicosis. J healthcare Eng (2019) 2019. doi: 10.1155/2019/6035920
202. Jiang S, Postovit L, Cattaneo A, Binder EB, Aitchison KJ. Epigenetic modifications in stress response genes associated with childhood trauma. Front Psychiatry (2019) 10:808. doi: 10.3389/fpsyt.2019.00808
203. Nagano T, Lubling Y, Stevens TJ, Schoenfelder S, Yaffe E, Dean W, et al. Single-cell Hi-C reveals cell-to-cell variability in chromosome structure. Nature (2013) 502:59–64. doi: 10.1038/nature12593
204. Flyamer IM, Gassler J, Imakaev M, Brandão HB, Ulianov SV, Abdennur N, et al. Single-nucleus Hi-C reveals unique chromatin reorganization at oocyte-to-zygote transition. Nature (2017) 544:110–4. doi: 10.1038/nature21711
205. Meng J, Lu Z, Liu H, Zhang L, Zhang S, Chen Y, et al. A protocol for RNA methylation differential analysis with MeRIP-Seq data and exomePeak R/Bioconductor package. Methods (2014) 69:274–81. doi: 10.1016/j.ymeth.2014.06.008
206. Mews P, Donahue G, Drake AM, Luczak V, Abel T, Berger SL. Acetyl-CoA synthetase regulates histone acetylation and hippocampal memory. Nature (2017) 546:381–6. doi: 10.1038/nature22405
207. Pan RY, He L, Zhang J, Liu X, Liao Y, Gao J, et al. Positive feedback regulation of microglial glucose metabolism by histone H4 lysine 12 lactylation in Alzheimer’s disease. Cell Metab (2022) 34:634–648.e636.
208. Gao Y, Zhou J, Qi H, Wei J, Yang Y, Yue J, et al. LncRNA lncLy6C induced by microbiota metabolite butyrate promotes differentiation of Ly6C(high) to Ly6C(int/neg) macrophages through lncLy6C/C/EBPβ/Nr4A1 axis. Cell Discovery (2020) 6:87. doi: 10.1038/s41421-020-00211-8
209. Zhou C, Rao X, Wang H, Zeng B, Yu Y, Chen J, et al. Hippocampus-specific regulation of long non-coding RNA and mRNA expression in germ-free mice. Funct Integr Genomics (2020) 20:355–65. doi: 10.1007/s10142-019-00716-w
210. Heller EA, Cates HM, Peña CJ, Sun H, Shao N, Feng J, et al. Locus-specific epigenetic remodeling controls addiction- and depression-related behaviors. Nat Neurosci (2014) 17:1720–7. doi: 10.1038/nn.3871
211. Heller EA, Hamilton PJ, Burek DD, Lombroso SI, Peña CJ, Neve RL, et al. Targeted epigenetic remodeling of the cdk5 gene in nucleus accumbens regulates cocaine- and stress-evoked behavior. J Neurosci (2016) 36:4690–7. doi: 10.1523/JNEUROSCI.0013-16.2016
212. Pulecio J, Verma N, Mejía-Ramírez E, Huangfu D, Raya A. CRISPR/cas9-based engineering of the epigenome. Cell Stem Cell (2017) 21:431–47. doi: 10.1016/j.stem.2017.09.006
213. Baldi S, Mundula T, Nannini G, Amedei A. Microbiota shaping—The effects of probiotics, prebiotics, and fecal microbiota transplant on cognitive functions: A systematic review. World J Gastroenterol (2021) 27:6715. doi: 10.3748/wjg.v27.i39.6715
214. Su Y-N, Wang M-J, Yang J-P, Wu X-L, Xia M, Bao M-H, et al. Effects of Yulin Tong Bu formula on modulating gut microbiota and fecal metabolite interactions in mice with polycystic ovary syndrome. Front Endocrinol (2023) 14:1122709. doi: 10.3389/fendo.2023.1122709
215. Grenham S, Clarke G, Cryan JF, Dinan TG. Brain–gut–microbe communication in health and disease. Front Physiol (2011) 2:94. doi: 10.3389/fphys.2011.00094
216. Kvakova M, Bertkova I, Stofilova J, Savidge TC. Co-encapsulated synbiotics and immobilized probiotics in human health and gut Microbiota modulation. Foods (2021) 10:1297. doi: 10.3390/foods10061297
217. Gibson GR, Hutkins R, Sanders ME, Prescott SL, Reimer RA, Salminen SJ, et al. Expert consensus document: The International Scientific Association for Probiotics and Prebiotics (ISAPP) consensus statement on the definition and scope of prebiotics. Nat Rev Gastroenterol Hepatol (2017) 14:491–502. doi: 10.1038/nrgastro.2017.75
218. Piccioni A, Covino M, Candelli M, Ojetti V, Capacci A, Gasbarrini A, et al. How do diet patterns, single foods, prebiotics and probiotics impact gut microbiota? Microbiol Res (2023) 14:390–408. doi: 10.3390/microbiolres14010030
219. Precup G, Pocol CB, Teleky B-E, Vodnar DC. Awareness, Knowledge, and Interest about Prebiotics—A Study among Romanian Consumers. Int J Environ Res Public Health (2022) 19:1208. doi: 10.3390/ijerph19031208
220. Burokas A, Arboleya S, Moloney RD, Peterson VL, Murphy K, Clarke G, et al. Targeting the microbiota-gut-brain axis: prebiotics have anxiolytic and antidepressant-like effects and reverse the impact of chronic stress in mice. Biol Psychiatry (2017) 82:472–87. doi: 10.1016/j.biopsych.2016.12.031
221. Dinan TG, Cryan JF. The microbiome-gut-brain axis in health and disease. Gastroenterol Clinics (2017) 46:77–89. doi: 10.1016/j.gtc.2016.09.007
222. Savignac HM, Couch Y, Stratford M, Bannerman DM, Tzortzis G, Anthony DC, et al. Prebiotic administration normalizes lipopolysaccharide (LPS)-induced anxiety and cortical 5-HT2A receptor and IL1-β levels in male mice. Brain behavior Immun (2016) 52:120–31. doi: 10.1016/j.bbi.2015.10.007
223. Zhang Q, Chen B, Zhang J, Dong J, Ma J, Zhang Y, et al. Effect of prebiotics, probiotics, synbiotics on depression: results from a meta-analysis. BMC Psychiatry (2023) 23:1–15. doi: 10.1186/s12888-023-04963-x
224. Gismondo M, Drago L, Lombardi A. Review of probiotics available to modify gastrointestinal flora. Int J antimicrobial Agents (1999) 12:287–92. doi: 10.1016/S0924-8579(99)00050-3
225. Isolauri E, Kirjavainen P, Salminen S. Probiotics: a role in the treatment of intestinal infection and inflammation? Gut (2002) 50:iii54–9. doi: 10.1136/gut.50.suppl_3.iii54
226. Kazemi A, Noorbala AA, Azam K, Eskandari MH, Djafarian K. Effect of probiotic and prebiotic vs placebo on psychological outcomes in patients with major depressive disorder: A randomized clinical trial. Clin Nutr (2019) 38:522–8. doi: 10.1016/j.clnu.2018.04.010
227. Zheng Y, Zhang Z, Tang P, Wu Y, Zhang A, Li D, et al. Probiotics fortify intestinal barrier function: a systematic review and meta-analysis of randomized trials. Front Immunol (2023) 14:1143548. doi: 10.3389/fimmu.2023.1143548
228. Slykerman R, Hood F, Wickens K, Thompson J, Barthow C, Murphy R, et al. Effect of Lactobacillus rhamnosus HN001 in pregnancy on postpartum symptoms of depression and anxiety: a randomised double-blind placebo-controlled trial. EBioMedicine (2017) 24:159–65. doi: 10.1016/j.ebiom.2017.09.013
229. Phillips JGP. The treatment of melancholia by the lactic acid bacillus. J Ment Sci (1910) 56:422–30. doi: 10.1192/bjp.56.234.422
230. Miyaoka T, Kanayama M, Wake R, Hashioka S, Hayashida M, Nagahama M, et al. Clostridium butyricum MIYAIRI 588 as adjunctive therapy for treatment-resistant major depressive disorder: a prospective open-label trial. Clin neuropharmacol (2018) 41:151–5. doi: 10.1097/WNF.0000000000000299
231. Varela-Trinidad GU, Domínguez-Díaz C, Solórzano-Castanedo K, Íñiguez-Gutiérrez L, Hernández-Flores TDJ, Fafutis-Morris M. Probiotics: Protecting our health from the gut. Microorganisms (2022) 10:1428. doi: 10.3390/microorganisms10071428
232. Akkasheh G, Kashani-Poor Z, Tajabadi-Ebrahimi M, Jafari P, Akbari H, Taghizadeh M, et al. Clinical and metabolic response to probiotic administration in patients with major depressive disorder: a randomized, double-blind, placebo-controlled trial. Nutrition (2016) 32:315–20. doi: 10.1016/j.nut.2015.09.003
233. Li N, Wang Q, Wang Y, Sun A, Lin Y, Jin Y, et al. Oral probiotics ameliorate the behavioral deficits induced by chronic mild stress in mice via the gut microbiota-inflammation axis. Front Behav Neurosci (2018) 12:266. doi: 10.3389/fnbeh.2018.00266
234. Majeed M, Nagabhushanam K, Arumugam S, Majeed S, Ali F. Bacillus coagulans MTCC 5856 for the management of major depression with irritable bowel syndrome: a randomised, double-blind, placebo controlled, multi-centre, pilot clinical study. Food Nutr Res (2018) 62. doi: 10.29219/fnr.v62.1218
235. Chong H, Yusoff N, Hor Y-Y, Lew L-C, Jaafar M, Choi S-B, et al. Lactobacillus plantarum DR7 alleviates stress and anxiety in adults: a randomised, double-blind, placebo-controlled study. Beneficial Microbes (2019) 10:355–73. doi: 10.3920/BM2018.0135
236. Sharma S, Fulton S. Diet-induced obesity promotes depressive-like behaviour that is associated with neural adaptations in brain reward circuitry. Int J Obes (2013) 37:382–9. doi: 10.1038/ijo.2012.48
237. David LA, Maurice CF, Carmody RN, Gootenberg DB, Button JE, Wolfe BE, et al. Diet rapidly and reproducibly alters the human gut microbiome. Nature (2014) 505:559–63. doi: 10.1038/nature12820
238. García-Montero C, Fraile-Martinez O, Gomez-Lahoz AM, Pekarek L, Castellanos AJ, Noguerales-Fraguas F, et al. Nutritional components in Western diet versus Mediterranean diet at the gut microbiota–immune system interplay. Implications for health and disease. Nutrients (2021) 13:699. doi: 10.3390/nu13020699
239. Suzuki T, Miyaki K, Tsutsumi A, Hashimoto H, Kawakami N, Takahashi M, et al. Japanese dietary pattern consistently relates to low depressive symptoms and it is modified by job strain and worksite supports. J Affect Disord (2013) 150:490–8. doi: 10.1016/j.jad.2013.04.044
240. Jacka FN, Mykletun A, Berk M, Bjelland I, Tell GS. The association between habitual diet quality and the common mental disorders in community-dwelling adults: the Hordaland Health study. Psychosomatic Med (2011) 73:483–90. doi: 10.1097/PSY.0b013e318222831a
241. Barber TM, Kabisch S, Pfeiffer AFH, Weickert MO. The effects of the mediterranean diet on health and gut microbiota. Nutrients (2023) 15. doi: 10.3390/nu15092150
242. Akbaraly TN, Brunner EJ, Ferrie JE, Marmot MG, Kivimaki M, Singh-Manoux A. Dietary pattern and depressive symptoms in middle age. Br J Psychiatry (2009) 195:408–13. doi: 10.1192/bjp.bp.108.058925
243. Ruusunen A, Lehto SM, Mursu J, Tolmunen T, Tuomainen T-P, Kauhanen J, et al. Dietary patterns are associated with the prevalence of elevated depressive symptoms and the risk of getting a hospital discharge diagnosis of depression in middle-aged or older Finnish men. J Affect Disord (2014) 159:1–6. doi: 10.1016/j.jad.2014.01.020
244. Molendijk M, Molero P, Sánchez-Pedreño FO, van der Does W, Martínez-González MA. Diet quality and depression risk: a systematic review and dose-response meta-analysis of prospective studies. J Affect Disord (2018) 226:346–54. doi: 10.1016/j.jad.2017.09.022
245. Seethaler B, Nguyen NK, Basrai M, Kiechle M, Walter J, Delzenne NM, et al. Short-chain fatty acids are key mediators of the favorable effects of the Mediterranean diet on intestinal barrier integrity: data from the randomized controlled LIBRE trial. Am J Clin Nutr (2022) 116:928–42. doi: 10.1093/ajcn/nqac175
246. Le Port A, Gueguen A, Kesse-Guyot E, Melchior M, Lemogne C, Nabi H, et al. Association between dietary patterns and depressive symptoms over time: a 10-year follow-up study of the GAZEL cohort. PloS One (2012) 7:e51593.
247. Martinez-Medina M, Denizot J, Dreux N, Robin F, Billard E, Bonnet R, et al. Western diet induces dysbiosis with increased E coli in CEABAC10 mice, alters host barrier function favouring AIEC colonisation. Gut (2014) 63:116–24. doi: 10.1136/gutjnl-2012-304119
248. Aizawa E, Tsuji H, Asahara T, Takahashi T, Teraishi T, Yoshida S, et al. Possible association of Bifidobacterium and Lactobacillus in the gut microbiota of patients with major depressive disorder. J Affect Disord (2016) 202:254–7. doi: 10.1016/j.jad.2016.05.038
249. Inserra A, Rogers GB, Licinio J, Wong ML. The microbiota-inflammasome hypothesis of major depression. Bioessays (2018) 40:1800027. doi: 10.1002/bies.201800027
250. Roy S, Dhaneshwar S. Role of prebiotics, probiotics, and synbiotics in management of inflammatory bowel disease: Current perspectives. World J Gastroenterol (2023) 29:2078. doi: 10.3748/wjg.v29.i14.2078
251. Haghighat N, Rajabi S, Mohammadshahi M. Effect of synbiotic and probiotic supplementation on serum brain-derived neurotrophic factor level, depression and anxiety symptoms in hemodialysis patients: a randomized, double-blinded, clinical trial. Nutr Neurosci (2021) 24:490–9. doi: 10.1080/1028415X.2019.1646975
252. Salminen S, Collado MC, Endo A, Hill C, Lebeer S, Quigley EM, et al. The International Scientific Association of Probiotics and Prebiotics (ISAPP) consensus statement on the definition and scope of postbiotics. Nat Rev Gastroenterol Hepatol (2021) 18:649–67. doi: 10.1038/s41575-021-00440-6
253. Taverniti V, Guglielmetti S. The immunomodulatory properties of probiotic microorganisms beyond their viability (ghost probiotics: proposal of paraprobiotic concept). Genes Nutr (2011) 6:261–74. doi: 10.1007/s12263-011-0218-x
254. Wu Y, Wang Y, Hu A, Shu X, Huang W, Liu J, et al. Lactobacillus plantarum-derived postbiotics prevent Salmonella-induced neurological dysfunctions by modulating gut–brain axis in mice. Front Nutr (2022) 9:946096. doi: 10.3389/fnut.2022.946096
255. Cristiano C, Cuozzo M, Coretti L, Liguori F, Cimmino F, Turco L, et al. Oral sodium butyrate supplementation ameliorates paclitaxel-induced behavioral and intestinal dysfunction. Biomed Pharmacother (2022) 153:113528. doi: 10.1016/j.biopha.2022.113528
256. Ney L-M, Wipplinger M, Grossmann M, Engert N, Wegner VD, Mosig AS. Short chain fatty acids: key regulators of the local and systemic immune response in inflammatory diseases and infections. Open Biol (2023) 13:230014. doi: 10.1098/rsob.230014
257. Pimentel TC, Cruz AG, Pereira E, Da Costa WKA, Da Silva Rocha R, De Souza Pedrosa GT, et al. Postbiotics: An overview of concepts, inactivation technologies, health effects, and driver trends. Trends Food Sci Technol (2023). doi: 10.1016/j.tifs.2023.06.009
258. Zhang F, Luo W, Shi Y, Fan Z, Ji G. Should we standardize the 1,700-year-old fecal microbiota transplantation? Am J Gastroenterol (2012) 107:1755–6. doi: 10.1038/ajg.2012.251
259. Bamba S, Nishida A, Imaeda H, Inatomi O, Sasaki M, Sugimoto M, et al. Successful treatment by fecal microbiota transplantation for Japanese patients with refractory Clostridium difficile infection: a prospective case series. J Microbiol Immunol Infect (2019) 52:663–6. doi: 10.1016/j.jmii.2017.08.027
260. Ianiro G, Segal JP, Mullish BH, Quraishi MN, Porcari S, Fabiani G, et al. Fecal microbiota transplantation in gastrointestinal and extraintestinal disorders. Future Microbiol (2020) 15:1173–83. doi: 10.2217/fmb-2020-0061
261. Hu B, Das P, Lv X, Shi M, Aa J, Wang K, et al. Effects of ‘healthy’fecal microbiota transplantation against the deterioration of depression in fawn-hooded rats. Msystems (2022) 7:e00218–00222.
262. Chen M, Liu M, Li C, Peng S, Li Y, Xu X, et al. Fecal microbiota transplantation effectively cures a patient with severe bleeding immune checkpoint inhibitor-associated colitis and a short review. Front Oncol (2022) 12:913217. doi: 10.3389/fonc.2022.913217
263. Doll JP, Vázquez-Castellanos JF, Schaub A-C, Schweinfurth N, Kettelhack C, Schneider E, et al. Fecal microbiota transplantation (FMT) as an adjunctive therapy for depression—case report. Front Psychiatry (2022) 13:815422. doi: 10.3389/fpsyt.2022.815422
264. Vasiliu O. Is fecal microbiota transplantation a useful therapeutic intervention for psychiatric disorders? A narrative review of clinical and preclinical evidence. Curr Med Res Opin (2023) 39:161–77. doi: 10.1080/03007995.2022.2124071
265. Rapoport EA, Baig M, Puli SR. Adverse events in fecal microbiota transplantation: a systematic review and meta-analysis. Ann Gastroenterol (2022) 35:150.
266. Schmidt EK, Torres-Espin A, Raposo PJ, Madsen KL, Kigerl KA, Popovich PG, et al. Fecal transplant prevents gut dysbiosis and anxiety-like behaviour after spinal cord injury in rats. PloS One (2020) 15:e0226128. doi: 10.1371/journal.pone.0226128
267. De Vasconcelos P, Lacerda JF. Hematopoietic stem cell transplantation for neurological disorders: A focus on inborn errors of metabolism. Front Cell Neurosci (2022) 16:895511. doi: 10.3389/fncel.2022.895511
268. Shan Y, Wang H, Yang Y, Wang J, Zhao W, Huang Y, et al. Evidence of a large current of transcranial alternating current stimulation directly to deep brain regions. Mol Psychiatry (2023) 1-9. doi: 10.1038/s41380-023-02150-8
Keywords: depressive disorder, gut microbiota, gut-brain axis (GBA), dysbiosis, metabolites, probiotics, prebiotics, FMT
Citation: Luqman A, He M, Hassan A, Ullah M, Zhang L, Rashid Khan M, Din AU, Ullah K, Wang W and Wang G (2024) Mood and microbes: a comprehensive review of intestinal microbiota’s impact on depression. Front. Psychiatry 15:1295766. doi: 10.3389/fpsyt.2024.1295766
Received: 17 September 2023; Accepted: 22 January 2024;
Published: 09 February 2024.
Edited by:
Kiran Veer Sandhu, University College Cork, IrelandReviewed by:
Hossein Dabiri, Shahid Beheshti University of Medical Sciences, IranSangyong Jung, CHA University, Republic of Korea
Padhmanand Sudhakar, Kumaraguru College of Technology, India
Copyright © 2024 Luqman, He, Hassan, Ullah, Zhang, Rashid Khan, Din, Ullah, Wang and Wang. This is an open-access article distributed under the terms of the Creative Commons Attribution License (CC BY). The use, distribution or reproduction in other forums is permitted, provided the original author(s) and the copyright owner(s) are credited and that the original publication in this journal is cited, in accordance with accepted academic practice. No use, distribution or reproduction is permitted which does not comply with these terms.
*Correspondence: Guixue Wang, d2FuZ2d4QGNxdS5lZHUuY24=; Wei Wang, YWJieXN0aW5hOThAMTYzLmNvbQ==
†These authors have contributed equally to this work