- 1Laboratory for Traumatic Stress Studies and Center for Genetics and BioMedical Informatics Research, CAS Key Laboratory of Mental Health, Institute of Psychology, Chinese Academy of Sciences, Beijing, China
- 2Department of Psychology, University of Chinese Academy of Sciences, Beijing, China
- 3Academy of Psychology and Behavior, Tianjin Normal University, Tianjin, China
- 4People’s Hospital of Deyang City, Deyang, Sichuan, China
Background: Posttraumatic stress disorder (PTSD) and major depressive disorder (MDD) comorbidity occurs through exposure to trauma with genetic susceptibility. Neuropeptide-Y (NPY) and dopamine are neurotransmitters associated with anxiety and stress-related psychiatry through receptors. We attempted to explore the genetic association between two neurotransmitter receptor systems and the PTSD–MDD comorbidity.
Methods: Four groups were identified using latent profile analysis (LPA) to examine the patterns of PTSD and MDD comorbidity among survivors exposed to earthquake-related trauma: low symptoms, predominantly depression, predominantly PTSD, and PTSD–MDD comorbidity. NPY2R (rs4425326), NPY5R (rs11724320), DRD2 (rs1079597), and DRD3 (rs6280) were genotyped from 1,140 Chinese participants exposed to earthquake-related trauma. Main, gene–environment interaction (G × E), and gene–gene interaction (G × G) effects for low symptoms, predominantly depression, and predominantly PTSD were tested using a multinomial logistic model with PTSD–MDD comorbidity as a reference.
Results: The results demonstrated that compared to PTSD–MDD comorbidity, epistasis (G × G) NPY2R-DRD2 (rs4425326 × rs1079597) affects low symptoms (β = −0.66, OR = 0.52 [95% CI: 0.32–0.84], p = 0.008, pperm = 0.008) and predominantly PTSD (β = −0.56, OR = 0.57 [95% CI: 0.34–0.97], p = 0.037, pperm = 0.039), while NPY2R-DRD3 (rs4425326 × rs6280) impacts low symptoms (β = 0.82, OR = 2.27 [95% CI: 1.26–4.10], p = 0.006, pperm = 0.005) and predominantly depression (β = 1.08, R = 2.95 [95% CI: 1.55–5.62], p = 0.001, pperm = 0.001). The two G × G effects are independent.
Conclusion: NPY and dopamine receptor genes are related to the genetic etiology of PTSD–MDD comorbidity, whose specific mechanisms can be studied at multiple levels.
1 Introduction
According to a survey conducted across 24 countries by the World Mental Health Organization, approximately 70.4% of adults have experienced at least one traumatic event (1, 2). Subjects witnessing traumatic events have a critical risk of suffering from traumatic stress-related diseases, primarily posttraumatic stress disorder (PTSD) and major depressive disorder (MDD) (3, 4). Previous studies have observed that more than 50% of the adults diagnosed with PTSD have MDD simultaneously (5, 6). Those with PTSD–MDD comorbidity have more treatment burden, lower quality of life, and lower income than the ones with PTSD or MDD alone (6). The mechanism of PTSD–MDD comorbidity has been insufficiently explored. Some scholars believed that PTSD–MDD comorbidity was attributed to the diagnosis symptom overlap between the two (7). Several stable symptoms overlap between PTSD and MDD. These include diminished interest, sleep disturbance, and trouble concentrating in revising all Diagnostic and Statistical Manual of Mental Disorders (DSM) editions, with unclear evidence. Other scholars believed that PTSD–MDD comorbidity was a different manifestation of a common genetic structure depending on trauma susceptibility. Mundy, Hübel (8) identified that PTSD and MDD could share the genetic structure of trauma susceptibility through polygenic risk scores (PRS) analysis. Nievergelt, Maihofer (9) discovered significant positive correlations between PTSD and MDD genetic variants through PRS. Some researchers observed that the inheritance between PTSD and MDD was entirely related (r = 1) (10). A recent meta-analysis indicated that PTSD and MDD share multiple genomic loci through cross-trait (11). Various lines of evidence suggest that genetics may be necessary for PTSD–MDD comorbidity.
PTSD–MDD comorbidity is a complex psychiatric disorder with multiple neural and molecular pathways. Among them, neurotransmitter systems, neuropeptide Y (NPY) receptors, and dopamine play essential roles in mental disorders. Neuropeptide Y consists of 36 amino acids, is widely distributed, and expressed in the central nervous system (CNS), such as the prefrontal cortex, hippocampus, amygdala, etc., and is highly conserved across various species (12–14). The NPY system adapts to stress response (15–17). First, the HPA axis is activated to cope with a stressful situation when people experience a stressful or traumatic event. Afterward, NPY levels would increase in the brain to balance out an overactive stress response (18). The NPY system was dysregulated in PTSD and MDD patients (16), primarily manifested as a decrease. Such downtrend NPY consistently existed at transcription and protein levels in human and animal studies (19, 20). NPY activates biological functions primarily through G protein-coupled receptors, NPY1R, NPY2R, NPY4R, NPY5R, and NPY6R. Y2R and Y5R are essential in stress response adaptation and emotional occurrence (21–23). Animal and human studies have found that NPY2R was upregulated during PTSD and depression (19, 20). Anxiety and depression symptoms in mice were relieved after injecting Y2R antagonists (24), indicating that Y2R may be anxiety-active. Y5R could work with Y1R to inhibit stress-induced dendritic hypertrophy of basolateral amygdala (BLA) pyramidal output neurons, demonstrating anxiolytic and antidepressant effects (25). Therefore, Y2R and Y5R may have opposite roles in stress response adaptation. We conjecture that Y2R and Y5R could be associated with the etiological mechanism of PTSD–MDD comorbidity.
Dopamine is a catecholamine that plays the role of a neurotransmitter in the brain and involves stress adaptation (26). Studies have observed that dopamine was downregulated with reduced activity in MDD and PTSD patients (27, 28). Similar to NPY, dopamine functions through its receptors. There are two main types of receptors, D1-like and D2-like receptors, of which D1-like receptor activation is excitatory (such as D1 and D5 receptors). In contrast, D2-like receptor activation is inhibited (such as D2, D3, and D4 receptors) (29, 30). Chronic exposure to mild stress damages the mesolimbic dopamine circuit, an underlying neurobiological mechanism for anhedonia, a core MDD symptom (28, 31). Established animal studies identified that D2/D3 agonists, not D1 receptors, exert rapid antidepressant effects (32). Early human studies observed that D2/D3 receptor binding was higher in MDD brains than in controls (33). Additionally, D2/D3 agonists could decrease the fear response, indicating significant antidepressant effects in PTSD mice (34). Thus, D2/D3 receptors could be associated with the etiological mechanism behind PTSD. Therefore, we hypothesize that D2/D3 could also be related to PTSD–MDD comorbidity.
There is a complex interplay between NPY and dopamine systems, engaging in the physiological processes of stress adaptation and exerting an impact on the PTSD–MDD comorbidity. The study indicated that NPY neurons in the arcuate nucleus (ARC) extensively projected to the ventral tegmental area (VTA) and accumbens (NAc). They interacted with dopaminergic neural circuits and co-expressed with dopamine, participating in various physiological processes, including stress-induced anxiety (35). Several studies have indicated a positive correlation between NPY activity and dopamine release. NPY and Y5R agonists have been shown to facilitate dopamine release in the NAc and striatum. Y2R antagonists, on the other hand, attenuated NPY-induced dopamine release (36, 37). Furthermore, studies have found an antagonistic interplay between dopamine and NPY in depression and food intake. An increase in dopamine release reduced NPY mRNA and weakened the biological effects induced by NPY (35, 38). Therefore, the dysfunctional antagonistic interplay between NPY and the dopamine system would likely to be involved in the biological mechanisms of related mental disorders. Nevertheless, the precise mechanisms underlying the interaction between the dopamine and NPY systems remain elusive. Similar observations were discerned in relevant genetic studies as well. Genetic polymorphism within the NPY have been identified in association with both PTSD and MDD (39, 40). NPY2R gene polymorphism, which mediated the effects of NPY, have been found to be associated with stress-induced mental disorders such as MDD (41). The functional variation rs4425326 in NPY2R has been reported to be associated with substance abuse (42, 43). Additionally, differential expression of NPY5R has been identified in relation to fear learning in PTSD, and lower mRNA levels of NPY5R associated with elevated anxiety (44, 45). For the dopamine system, a recent meta-analysis revealed that genetic polymorphism within the DRD2 exhibited a similar trend in both PTSD and MDD (46). The DRD3 rs6280 has also been found to be associated with PTSD and MDD (47, 48). In addition to the direct effects of genetic variations, the environment is a crucial risk factor influencing the PTSD–MDD comorbidity. Studies indicated that genetic variations interacted with the environment to impact PTSD and MDD (49, 50). Therefore, it can be speculated that genetic variations in receptor genes, namely, NPY2R, NPY5R, DRD2, and DRD3, within the NPY and dopamine systems may directly influence the physiological responses of PTSD–MDD comorbidity or interact indirectly with the environment to impact these responses, but these remain to be explored.
Latent profile analysis (LPA) or latent class analysis (LCA) classifies potential categories for continuous or categorical indicators (51). Traditional factor analysis or disease diagnosis could ignore the differences in disease phenotypes exhibited by heterogeneous groups, decreasing the finding validity. In contrast, LPA considers individual heterogeneity and centers on individuals with potentially identical response patterns that are classified together. This could mitigate the adverse effects of potential individual heterogeneity. Several studies have used LPA to classify individuals with PTSD-related comorbidity. However, the phenotype of PTSD comorbidity with other psychiatric disorders can vary based on the trauma type (52). Contractor et al. (53) used LPA to determine the potential structure of PTSD–MDD comorbidity by analyzing the symptoms of 268 college students with potential trauma events (PTE). PTSD and depression symptoms were divided into three sub-categories: high severity, lower PTSD–higher depression, and higher PTSD–lower depression. The results indicated that PTSD and depression were parallel to each other. Armour et al. (54) conducted LPA on 283 Canadian veterans with PTSD and MDD comorbidity and isolated three similar patterns of comorbidity: high, moderate, and low symptoms of PTSD and MDD. Hruska et al. (55) conducted LPA on 249 motorcycle accident victims with combined PTSD, current MDD, and alcohol or other drug use disorders (MDD/AoDs) and delved into four categories: resilient, mild, moderate, and severe psychopathology. We cannot use these PTSD–MDD patterns directly due to the large sample variation and inconsistent classification results for discerning PTSD and MDD patterns. Therefore, LPA helps determine PTSD and MDD patterns in the current study.
We explored the mechanisms of NPY and dopamine receptors in PTSD–MDD comorbidity in the Chinese population exposed to earthquake-related trauma from a genetic perspective. We analyzed the gene main effects, gene × environment interaction effects (G × E), and gene × gene interaction effects (G × G) to decipher the genetic mechanism in PTSD–MDD comorbidity. The receptor genes NPY2R, NPY5R, DRD2, and DRD3 were selected as candidate genes. We recruited 1,140 participants from the 2008 Wenchuan earthquake survivors to determine their earthquake-related trauma, PTSD, and depressive symptoms. The participants were divided into four groups through LPA based on the measured PTSD and depressive symptoms. The four categories were low symptoms, predominantly depression, predominantly PTSD, and PTSD–MDD comorbidity.
2 Materials and methods
2.1 Participants and procedures
All the participants were recruited from people who had survived the 2008 Wenchuan magnitude 8.0 earthquake in Sichuan Province, China. We went to Hanwang District in Mianzhu City, the largest rebuilding community post-earthquake, in November 2013 to obtain the data. Our investigators included trained clinical psychologists, psychiatrists, psychotherapists, and postgraduate psychology students. Sampling procedures were detailed in our previous studies (23). The inclusion criteria were as follows (1): Each family selects one of the most suitable candidates to participate in our study with the family as a research unit (a person in the household born closest to the investigation date). (2) Participants must have experienced the Wenchuan earthquake first-hand. (3) Participants should be at least 16 years of age. (4) Participants should not have suffered from any previous mental disorders. Based on the inclusion criteria, we informed participants of the purpose of our study. We ensured that everyone understood our objectives and obtained their written informed consent. Later, we obtained self-reported questionnaire data from participants, amounting to 1,196 people.
Specialist nurses collected blood samples from these participants for subsequent genotyping. Among them, 24 participants refused to provide blood, 26 failed DNA extraction, and 6 failed genotyping. Finally, we obtained the data for 1,140 participants. The demographic data of participants are demonstrated in Table 1. The participants were between 16 and 73 years (M = 48.1, SD = 10.0), except for two minors (one aged 16 and one aged 17). The rest were adults, with 363 men (31.8%) and 777 women (68.2%), and approximately 99.6% were Han Chinese.
This study was conducted by the Declaration of Helsinki and received an ethical committee approval on 12 June 2013 (Institutional Review Board of the Institute of Psychology, Chinese Academy of Sciences, study registered under number H13010). All the participants understood the objectives and signed the written informed consent. The experimental methods were performed following the relevant guidelines.
2.2 Measurement
Demographic data were collected using our questionnaire, including the gender, age, marital status, education level, and ethnicity of the participants.
The environmental variable, earthquake-related trauma exposure, was assessed using a questionnaire with 10 questions (23): during the earthquake, (1) Were you trapped under the rubble? (2) Were you injured? (3) Were you disabled due to injuries? (4) Did you participate in rescue efforts? (5) Did you witness the death of someone? (6) Did you see mutilated bodies? (7) Did any family members die during the disaster? (8) Were any family members injured? (9) Did any friend or neighbor die during the disaster? (10) Did you lose your livelihood because of the disaster? Each question was scored at two points; 0 meant no experience, and 1 implied experience. Scores represented the level of earthquake-related trauma exposure. The total score ranged from 0 to 10; the higher the score, the more severe the trauma exposure.
PTSD diagnosis is inferred from the diagnostic criteria provided by the fifth edition of the Diagnostic and Statistical Manual of Mental Disorders [DSM-5; (56)]. The participant must have experienced at least one traumatic event, one intrusion symptom, one avoidance symptom, two negative alterations in cognition and mood, and two arousal symptoms lasting longer than 1 month. Participant PTSD symptoms were assessed by the DSM-5 PTSD checklist [PCL-5, (57)]. The questionnaire has 20 self-reported items with four dimensions: intrusion, avoidance, negative alterations in cognition and mood, and hyperarousal. Participants had to answer this questionnaire depending on their circumstances and the Wenchuan earthquake. Each item was scored on a Likert five-point scale from 0 (not at all) to 4 (extremely). The higher scores represented the severity of the corresponding symptoms. The final score was determined by adding item scores for each dimension. The original PCL-5 questionnaire indicated good reliability and validity (58). The revised Chinese version also possessed good reliability and validity in existing studies (59). Cronbach’s α was 0.95 in this study.
MDD was determined through the Center for Epidemiological Studies–Depression Scale (CES-D) developed by Radloff (60). The questionnaire had 20 self-reported items, evaluating the severity of depressive symptoms from four dimensions: positive affect, depressive affect, somatic complaints, and interpersonal problems. The four questions to evaluate positive affect were reverse score questions. Each item was scored on a Likert four-point scale from 0 (rare or none of the time/less than one day) to 3 (most or all the time/5 to 7 days). The higher scores were related to more severe depression. The final score was determined by adding item scores for each dimension. The original CES-D questionnaire indicated good psychometric properties (61). The Chinese version of CES-D also had solid reliability and validity (62). Cronbach’s α was 0.87 in our study.
2.3 SNP selection and genotyping
Four candidate genes, NPY2R, NPY5R, DRD2, and DRD3, and corresponding functionally significant SNPs were selected for our study. For NPY2R, rs4425326 was associated with alcohol dependence and withdrawal (43). For NPY5R, rs11724320 was related to panic disorder (63). For DRD2, rs1079597 has been widely reported to be correlated with addictive behaviors (64, 65). For DRD3, rs6280 was associated with impulsive behavior, addiction, and schizophrenia (66, 67).
Based on the DNA extraction standard protocol proposed by Garg (68), DNA was extracted from peripheral blood. The genotyping of rs4425326, rs11724320, rs1079597, and rs6280 was performed using a custom-by-design 2 × 48-Plex SNP scan™ Kit (Genesky Bio-technologies Inc., Shanghai, China) through double ligation and multiplex fluorescence polymerase chain reaction (PCR). Gene Mapper 4.1 (Applied Biosystems) software helped analyze the raw data following the fragment size of allele-specific ligation-PCR products. The genotype call rates exceeded 98%.
2.4 Statistical analysis
In our previous study, a detailed LPA for patterns of PTSD and depression symptoms has been reported (59). Specifically, using Mplus 7.0 maximum likelihood estimation with robust standard errors, an LPA was conducted on the T scores of eight dimensions, namely, four dimensions of PTSD symptoms (intrusion, avoidance, negative alterations in cognitions and mood, and hyperarousal) and four dimensions of depression symptoms (depressive affect, positive affect, somatic complaints, and interpersonal problems). Comprehensive evaluation of fit indices, parsimony, and interpretability was conducted to select the optimal class model. An LPA model that had a lower Bayesian Information Criterion (BIC), a lower Akaike Information Criterion (AIC), a higher entropy, a significant Lo-Mendell-Rubin likelihood ratio test (LMR LRT), a significant Lo-Mendell-Rubin adjusted likelihood ratio test (ALMR LRT), and a significant bootstrap likelihood ratio test (BLRT) would be the optimal class model. After analyzing, the four-class model exhibited a lower BIC and a lower AIC than the one-, two-, and three-class model, as well as a higher entropy than the five-class model. Simultaneously, the four-class model had significant LMR LRT, ALMR LRT, and BLRT. In accordance with this optimal class model, the collected phenotypic data were synthesized into four groups: low symptoms (n = 616, 53.9%), predominantly depression (n = 203, 18.2%), predominantly PTSD (n = 219, 18.9%), and PTSD–MDD comorbidity (n = 102, 9.0%).
We analyzed the variance of each SNP in demographic statistical variables (gender, age, marital status, and education) and earthquake-related trauma exposures using R 4.2.0 (https://www.r-project.org/) to classify the LPA information.
To test whether the main, G × E, and G × G effects among NPY2R, NPY5R, DRD2, and DRD3 genes were significantly different between PTSD–MDD comorbidity and other groups, multinomial logistic regression was conducted with adjustment for gender, age, marital status, and education level. The independent variables included SNPs, earthquake-related trauma, SNP × SNP, and SNP × earthquake-related trauma. In contrast, the dependent variables were different groups from LPA. In each SNP genotype, the major allele homozygous was coded as 0, heterozygous was coded as 1, and the minor allele homozygous coded as 2. The p-values of logistic regression were bidirectional in the analysis. We conducted a permutation test (number of permutations = 100,000) to correct the possible deviation caused by insufficient sample size and multiple comparisons. The results were reported as p < 0.05 and permutation p < 0.05.
3 Results
Based on the descriptive statistical analysis, the mean score of earthquake-related trauma was 3.46 (SD = 1.80, score range: 0–10). The mean total score of PTSD was 18.77 (SD = 13.46, score range: 1–77), and that of depression was 37.02 (SD = 8.63, score range: 20–68), with details in Table 2. The analysis of variance revealed that rs4425326, rs11724320, and rs1079597 showed no significant differences in gender, age, marital status, and education. Rs6280 possessed significant differences in age and education level (Supplementary Table S1). All the SNP genotypes conformed to the Hardy–Weinberg equilibrium in the all samples, female patients, and male patients, respectively (Supplementary Tables S2-S4).
The distribution of SNP genotype in each group is represented in Table 3. Multinomial logistic regression analysis of G × G interactions between PTSD–MDD comorbidity and other groups was significant. As described in Table 4, NPY2R-DRD2 (rs4425326 × rs1079597) and NPY2R-DRD3 (rs4425326 × rs6280) interaction effects were significant {(β = −0.66, OR = 0.52 [95% CI: 0.32–0.84], p = 0.008, pperm = 0.008) and (β = 0.82, OR = 2.27 [95% CI: 1.26–4.10], p = 0.006, pperm = 0.005)} when low symptoms were compared with PTSD–MDD comorbidity. When predominantly PTSD was compared with PTSD–MDD comorbidity, the NPY2R-DRD2 (rs4425326 × rs1079597) interaction effect was significant (β = −0.56, OR = 0.57 [95% CI: 0.34–0.97], p = 0.037, pperm = 0.039). The NPY2R-DRD3 (rs4425326 × rs6280) interaction effect was significant (β = 1.08, OR = 2.95 [95% CI: 1.55–5.62], p = 0.001, pperm = 0.001) when predominantly depression was compared with PTSD–MDD comorbidity.
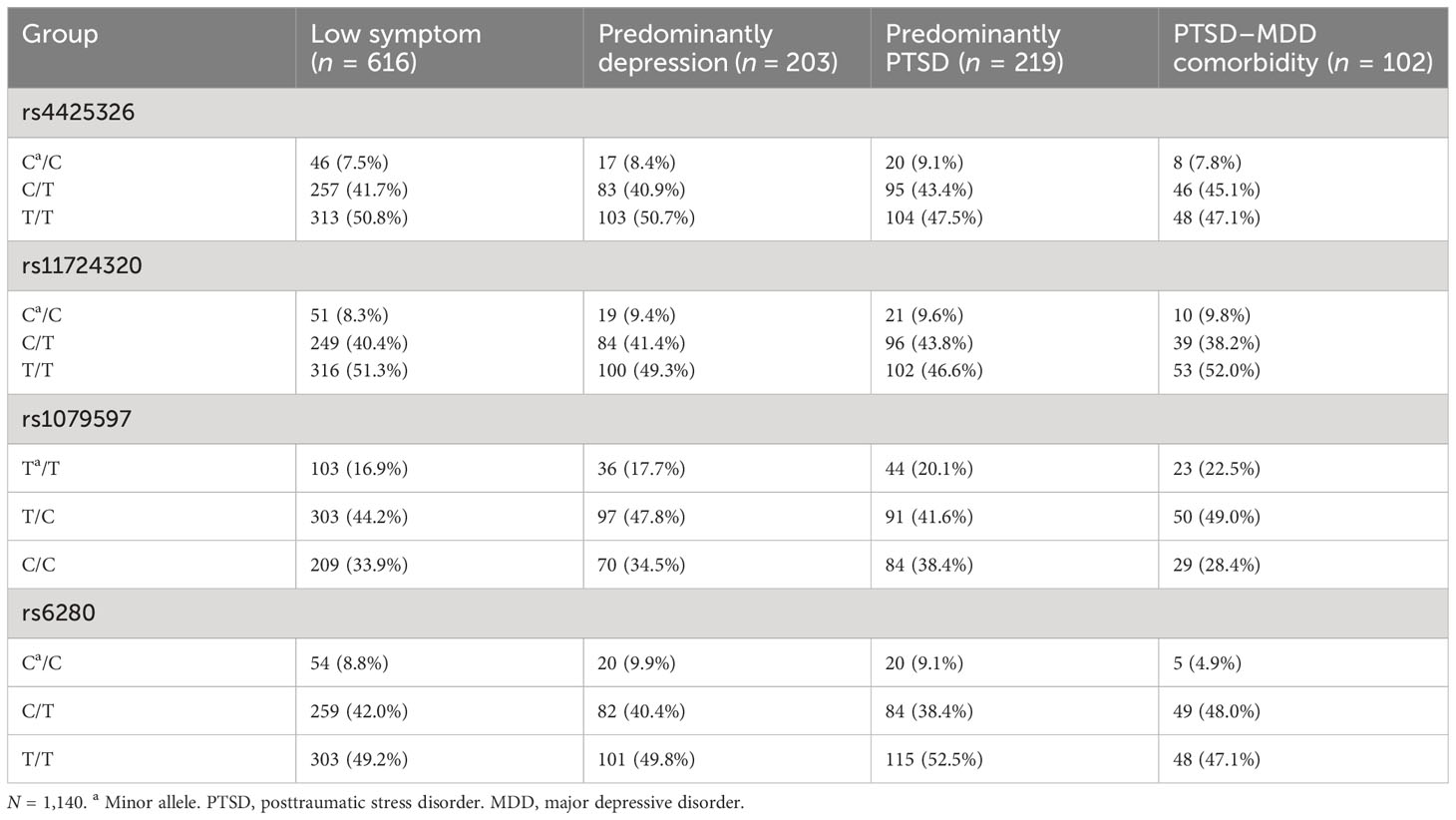
Table 3 Frequencies of different genotypes of each SNP in four groups divided by latent profile analysis.
After putting all the SNPs, G × E, and G × G into one regression model and PTSD–MDD comorbidity was set as the reference, the NPY2R-DRD2 of the rs4425326 × rs1079597 genotype interaction was significant when compared with low symptoms (β = −0.68, OR = 0.51 [95% CI: 0.31–0.83], p = 0.007, pperm = 0.007) and predominantly PTSD (β = −0.59, OR = 0.55 [95% CI: 0.33–0.94], p = 0.029, pperm = 0.032). Moreover, the NPY2R-DRD3 of the rs4425326 × rs6280 genotype interaction was significant when compared with low symptoms (β = 0.85, OR = 2.35 [95% CI: 1.27–4.30], p = 0.005, pperm = 0.005) and predominantly depression (β = 0.39, OR = 3.09 [95% CI: 1.59–6.03], p = 0.0008, pperm = 0.0009) (Supplementary Table S5). Therefore, the interactions of NPY2R-DRD2 and NPY2R × DRD3 were independent.
No SNPs significantly affected PTSD–MDD comorbidity and other groups (p > 0.05). Moreover, no significant G × E effects existed between PTSD–MDD comorbidity and other groups (p > 0.05) (Supplementary Table S6). Additionally, there were no other significant G × G effects (Supplementary Table S7).
4 Discussion
The current study performed gene main effects, gene–environment interaction, and gene–gene interaction analysis on NPY2R, NPY5R, DRD2, and DRD3 genotypes in Chinese exposed to earthquake-related trauma. The objective was to explore the potential genetic mechanism of NPY and dopamine receptors in PTSD–MDD comorbidity.
The results did not observe any significant gene main effects and gene–environment interaction. Consistent with previous genome-wide association study (GWAS) results, rs4425326, rs11724320, rs1079597, and rs6280 have not been found to be significantly associated with either PTSD or MDD in GWASs (9, 69). However, this does not necessarily mean that the genotypes of rs4425326, rs11724320, rs1079597, and rs6280 have no effect on PTSD–MDD comorbidity. It is possible that their impact on PTSD–MDD comorbidity is not achieved through a straightforward direct effect. In line with another discovery in the current study, two significant gene–gene interaction effects were identified. With PTSD–MDD comorbidity as a reference group, low symptoms, predominantly depression, and predominantly PTSD group were compared among the four phenotypic groups recommended by LPA. We found NPY2R × DRD2 and NPY2R × DRD3 interaction effects significantly, even considering main gene, gene–environment interaction, and gene–gene interaction effects. Therefore, the NPY and dopamine receptor genes do not affect PTSD–MDD comorbidity alone. Instead, they interact with the PTSD–MDD comorbidity mechanism. Consistent with previous findings by Rezitis (35), NPY could interact with the dopamine system in stress response. Specifically, the rs4425326 and rs1079597 allele genotypes interact to put individuals at risk for PTSD–MDD comorbidity compared to low symptoms. In contrast, rs4425326 and rs6280 allele genotypes interact to protect individuals from PTSD–MDD comorbidity. When predominantly PTSD was compared with PTSD–MDD comorbidity, the interaction between rs4425326 and rs1079597 alleles put individuals at risk of developing PTSD–MDD comorbidity. When predominantly depression was compared with PTSD–MDD comorbidity, the interaction between rs4425326 and rs6280 alleles protects individuals from PTSD–MDD comorbidity. These two gene–gene interactions existed after controlling for all gene main effects, gene–environment interaction, gene–gene interaction, earthquake-related trauma exposure, and demographic variables. Our findings established that NPY and dopamine receptors are associated with the genetic etiology of PTSD–MDD comorbidity. Moreover, genetics are involved in the etiology of PTSD–MDD comorbidity (5, 8, 10).
The NPY system is extensively involved in stress response, of which Y2R has been found to be anxiety-active. The rs4425326 polymorphism is in the exon of NPY2R, 0.2 Mb upstream on the 5′ end. SNPs in this region may potentially influence the transcriptional regulation of NPY2R, thereby affecting the expression of NPY2R (70). Studies have shown that Y2R was a presynaptic autoreceptor involved in the NPY negative feedback loop, thereby reducing NPY release to promote anxiety (15).
The dopamine system is widely recognized in psychiatry, manifested by an abnormal decrease of D2/D3 receptors (71). In fact, DRD2 and DRD3 shared 52% global homology, suggesting the potential functional similarities in their evolutionary processes, as both belong to the D2-like receptors (72). The rs1079597 is in the regulatory and structural coding regions of DRD2. It has been found to be associated with schizophrenia and addictive behaviors (64, 73). The C allele of this SNP was associated with a decreased density of D2 receptors in the individual’s striatum (74). Studies indicated that D2 receptor agonists had anxiolytic and antidepressant effects, alleviating symptoms of PTSD and MDD (34, 75). D2 receptor antagonists have been observed to induce emotional blunting and disrupt the motivational system (76). This phenomenon may be linked to emotional numbness in PTSD and anhedonia in MDD. Therefore, the rs1079597 may alter dopamine release by modulating the density of D2 receptors in the midbrain dopamine circuit, thus participating in the molecular mechanisms underlying the PTSD–MDD comorbidity. The rs6280 is in the exon of DRD3, and is a functional SNP that alters dopamine receptor binding affinity (77). Like rs1079597 in DRD2, rs6280 was also related to depression and addictive behaviors (48, 78). The rs6280 induced a substitution of serine with glycine at position 9 in the polypeptide product, leading to a substantial increase in the binding affinity of D3 receptors for dopamine (79). This modification facilitated dopamine release in the striatum, thereby contributing to the modulation of the stress adaptation.
The significant interaction between NPY2R and DRD2, as well as the interaction with DRD3, strongly indicates the involvement of the NPY and dopamine systems in PTSD–MDD comorbidity. The rs4425326 is implicated in potentially regulating the expression of NPY2R, while rs1079597 modulates D2 receptor density, and rs6280 alters the binding affinity of D3 receptors. Consequently, the interaction between the NPY and dopamine systems in PTSD–MDD comorbidity is likely mediated through the Y2R, D2, and D3 receptors. Y2R and D2 may interact within the amygdala that controls emotional memory, especially fear memory. The memory of traumatic events is a crucial cognitive function influencing PTSD and MDD. Studies have shown that Y2R and D2/D3 receptor activation all facilitated the extinction of fear memory in the amygdala (34, 80). Therefore, Y2R and D2/D3 may exert synergistic effects on the acquisition and extinction of fear memory. The interaction between Y2R and D3 is likely to take place when stress encounters. Studies have shown that when stress had just occurred, Y2R activated the NPY negative feedback loop to decrease NPY release while increasing dopamine release (15, 37). This increased dopamine release may be due to the high affinity of D3 modulated by the rs6280 that prompted VTA dopaminergic neurons to release more dopamine in response to stress (81). Therefore, Y2R and D3 may mobilize the organism in response to stress through synergistic effects. When stress disappeared, the persistent overactivation of Y2R and the compensatory reduction in dopamine within the VTA that failed to disappear may be one of the molecular mechanisms contributing to PTSD–MDD comorbidity. Previous studies have consistently identified a substantial elevation in expression of NPY2R mRNA and a decline in dopamine levels in PTSD and MDD (20, 28, 34). Nevertheless, the specific antagonistic or synergistic molecular mechanisms warrant meticulous experimental validation and exploration.
In addition, our study also explored the relationship between NPY5R and PTSD–MDD comorbidity. However, there are no significant results. NPY5R is not studied enough in PTSD–MDD comorbidity. There were conflicting results in the remaining studies, whose function could not be evaluated during stress adaptation. More research from multiple levels, such as genetics and neurobiology, should explore the future role of NPY and dopamine systems in stress adaptation.
There are several limitations to our study. First, after the LPA, the number of subjects in each group is small, challenging the result validity. In the future, more extensive sample studies should replicate our outcomes. Secondly, the self-reporting method may bring about biases for self-expectation. In the future, techniques such as clinical diagnosis or adding physiological indicators can improve the reliability and validity of the study. Third, the gender ratio in current samples is not balanced, and previous studies have observed an interaction between genetic variants and gender, especially in sexually dimorphic conditions (82). Therefore, further exploration should be conducted in samples with a balanced gender ratio, and the role of gender in PTSD–MDD comorbidity should be further investigated. Finally, our conclusions are limited to the Chinese population exposed to earthquake-related trauma. The same genetic variants may be different in other trauma types or populations. Therefore, the results should be interpreted and generalized with caution.
Overall, the current study identified NPY and dopamine receptor genes involved in the genetic etiology of PTSD–MDD comorbidity. There were significant interactions in two gene pairs, NPY2R-DRD2 and NPY2R-DRD3, providing a new etiological explanation of PTSD–MDD comorbidity. The findings expand the previous research on the anxiolytic and antidepressant effects of NPY and provide information on the dopamine system in stress-related disorders. Site-directed mutagenesis can be further employed to observe the effects of rs4425326, rs1079597, and rs6280 variations on methylation and gene expression in neural cell lines. Moreover, future studies should comprehensively explore the roles of the NPY and dopamine systems in PTSD–MDD comorbidity at multiple levels, taking into consideration the antagonistic, synergistic, or other interactive relationships between NPY and its receptors and dopamine and its receptors. Utilize gene knockout or gene overexpression animal experiments to explore effective genes and their underlying mechanisms. Inject agonists or antagonists to explore specific targets and mechanisms for promoting or alleviating PTSD–MDD comorbidity symptoms. Additionally, further genome-wide interaction analyses on a larger scale are imperative to comprehensively explore and substantiate the contribution of gene interactions to the PTSD–MDD comorbidity at the genome-wide level.
Data availability statement
The original contributions presented in the study are publicly available. This data can be found here: https://ngdc.cncb.ac.cn/ under the accession number GVM000614.
Ethics statement
The studies involving humans were approved by Institutional Review Board of the Institute of Psychology, Chinese Academy of Sciences. The studies were conducted in accordance with the local legislation and institutional requirements. Written informed consent for participation in this study was provided by the participants’ legal guardians/next of kin.
Author contributions
LX: Formal analysis, Investigation, Methodology, Writing – original draft, Writing – review & editing. JZ: Writing – review & editing. HY: Data curation, Writing – review & editing. CC: Data curation, Writing – review & editing. RF: Data curation, Writing – review & editing. PL: Data curation, Writing – review & editing. SL: Data curation, Writing – review & editing. BW: Writing – review & editing. KZ: Conceptualization, Formal analysis, Funding acquisition, Methodology, Project administration, Supervision, Writing – original draft, Writing – review & editing. LW: Conceptualization, Funding acquisition, Investigation, Methodology, Project administration, Supervision, Writing – review & editing.
Funding
The author(s) declare financial support was received for the research, authorship, and/or publication of this article. This work was supported by the National Natural Science Foundation of China (No. U21A20364, No. 31971020 and No. 82174237), the Key Project of the National Social Science Foundation of China (No. 20ZDA079), the Key Project of Research Base of Humanities and Social Sciences of Ministry of Education (No.16JJD190006), and the Scientific Foundation of Institute of Psychology, Chinese Academy of Sciences (No. E2CX4115CX).
Acknowledgments
We sincerely appreciate understanding and support of participants and their families.
Conflict of interest
The authors declare that the research was conducted in the absence of any commercial or financial relationships that could be construed as a potential conflict of interest.
Publisher’s note
All claims expressed in this article are solely those of the authors and do not necessarily represent those of their affiliated organizations, or those of the publisher, the editors and the reviewers. Any product that may be evaluated in this article, or claim that may be made by its manufacturer, is not guaranteed or endorsed by the publisher.
Supplementary material
The Supplementary Material for this article can be found online at: https://www.frontiersin.org/articles/10.3389/fpsyt.2024.1257911/full#supplementary-material
References
1. Kessler RC, Aguilar-Gaxiola S, Alonso J, Benjet C, Bromet EJ, Cardoso G, et al. Trauma and Ptsd in the who world mental health surveys. Eur J Psychotraumatol. (2017) 8:1353383. doi: 10.1080/20008198.2017.1353383
2. Benjet C, Bromet E, Karam EG, Kessler RC, McLaughlin KA, Ruscio AM, et al. The epidemiology of traumatic event exposure worldwide: results from the world mental health survey consortium. Psychol Med. (2016) 46:327–43. doi: 10.1017/s0033291715001981
3. Forman-Hoffman VL, Bose J, Batts KR, Glasheen C, Hirsch E, Karg RS, et al. Correlates of Lifetime Exposure to One or More Potentially Traumatic Events and Subsequent Posttraumatic Stress among Adults in the United States: Results from the Mental Health Surveillance Study, 2008-2012. Cbhsq Data Review. Rockville (MD: Substance Abuse and Mental Health Services Administration (US (2012) p. 1–49.
4. Hovens JG, Giltay EJ, Spinhoven P, van Hemert AM, Penninx BW. Impact of childhood life events and childhood trauma on the onset and recurrence of depressive and anxiety disorders. J Clin Psychiatry. (2015) 76:931–8. doi: 10.4088/JCP.14m09135
5. Koenen KC, Moffitt TE, Caspi A, Gregory A, Harrington H, Poulton R. The developmental mental-disorder histories of adults with posttraumatic stress disorder: A prospective longitudinal birth cohort study. J Abnorm Psychol. (2008) 117:460–6. doi: 10.1037/0021-843x.117.2.460
6. Caramanica K, Brackbill RM, Liao T, Stellman SD. Comorbidity of 9/11-related Ptsd and depression in the world trade center health registry 10-11 years postdisaster. J Trauma Stress. (2014) 27:680–8. doi: 10.1002/jts.21972
7. Flory JD, Yehuda R. Comorbidity between post-traumatic stress disorder and major depressive disorder: alternative explanations and treatment considerations. Dialogues Clin Neurosci. (2015) 17:141–50. doi: 10.31887/DCNS.2015.17.2/jflory
8. Mundy J, Hübel C, Gelernter J, Levey D, Murray RM, Skelton M, et al. Psychological trauma and the genetic overlap between posttraumatic stress disorder and major depressive disorder. Psychol Med. (2021) 52:1–10. doi: 10.1017/s0033291721000830
9. Nievergelt CM, Maihofer AX, Klengel T, Atkinson EG, Chen CY, Choi KW, et al. International meta-analysis of ptsd genome-wide association studies identifies sex- and ancestry-specific genetic risk loci. Nat Commun. (2019) 10:4558. doi: 10.1038/s41467-019-12576-w
10. Sartor CE, Grant JD, Lynskey MT, McCutcheon VV, Waldron M, Statham DJ, et al. Common heritable contributions to low-risk trauma, high-risk trauma, posttraumatic stress disorder, and major depression. Arch Gen Psychiatry. (2012) 69:293–9. doi: 10.1001/archgenpsychiatry.2011.1385
11. Zhang F, Rao S, Cao H, Zhang X, Wang Q, Xu Y, et al. Genetic evidence suggests posttraumatic stress disorder as a subtype of major depressive disorder. J Clin Invest. (2022) 132:e145942. doi: 10.1172/jci145942
12. Tatemoto K, Carlquist M, Mutt V. Neuropeptide Y–a novel brain peptide with structural similarities to peptide yy and pancreatic polypeptide. Nature. (1982) 296:659–60. doi: 10.1038/296659a0
13. Heilig M, Widerlöv E. Neurobiology and clinical aspects of neuropeptide Y. Crit Rev Neurobiol. (1995) 9:115–36.
14. Dumont Y, Quirion R. An overview of neuropeptide Y: pharmacology to molecular biology and receptor localization. Exs. (2006) 95):7–33. doi: 10.1007/3-7643-7417-9_2
15. Kautz M, Charney DS, Murrough JW. Neuropeptide Y, resilience, and Ptsd therapeutics. Neurosci Lett. (2017) 649:164–9. doi: 10.1016/j.neulet.2016.11.061
16. Tural U, Iosifescu DV. Neuropeptide Y in Ptsd, Mdd, and chronic stress: A systematic review and meta-analysis. J Neurosci Res. (2020) 98:950–63. doi: 10.1002/jnr.24589
17. Reichmann F, Holzer P. Neuropeptide Y: A stressful review. Neuropeptides. (2016) 55:99–109. doi: 10.1016/j.npep.2015.09.008
18. Nwokafor C, Serova LI, Sabban EL. Preclinical findings on the potential of intranasal neuropeptide Y for treating hyperarousal features of Ptsd. Ann N Y Acad Sci. (2019) 1455:149–59. doi: 10.1111/nyas.14172
19. Serova LI, Nwokafor C, Van Bockstaele EJ, Reyes BAS, Lin X, Sabban EL. Single prolonged stress Ptsd model triggers progressive severity of anxiety, altered gene expression in Locus coeruleus and hypothalamus and effected sensitivity to Npy. Eur Neuropsychopharmacol. (2019) 29:482–92. doi: 10.1016/j.euroneuro.2019.02.010
20. Sharma A, Ren X, Zhang H, Pandey GN. Effect of depression and suicidal behavior on neuropeptide Y (Npy) and its receptors in the adult human brain: A postmortem study. Prog Neuropsychopharmacol Biol Psychiatry. (2022) 112:110428. doi: 10.1016/j.pnpbp.2021.110428
21. Redrobe JP, Dumont Y, Herzog H, Quirion R. Neuropeptide Y (Npy) Y2 receptors mediate behaviour in two animal models of anxiety: evidence from Y2 receptor knockout mice. Behav Brain Res. (2003) 141:251–5. doi: 10.1016/s0166-4328(02)00374-1
22. Walker MW, Wolinsky TD, Jubian V, Chandrasena G, Zhong H, Huang X, et al. The novel neuropeptide Y Y5 receptor antagonist Lu Aa33810 [N-[[Trans-4-[(4,5-dihydro[1]Benzothiepino[5,4-D]Thiazol-2-yl)Amino]Cyclohexyl]Methyl]-methanesulfonamide] exerts anxiolytic- and antidepressant-like effects in rat models of stress sensitivity. J Pharmacol Exp Ther. (2009) 328:900–11. doi: 10.1124/jpet.108.144634
23. Liu P, Wang L, Cao C, Wang R, Zhang J, Zhang B, et al. The underlying dimensions of Dsm-5 posttraumatic stress disorder symptoms in an epidemiological sample of Chinese earthquake survivors. J Anxiety Disord. (2014) 28:345–51. doi: 10.1016/j.janxdis.2014.03.008
24. Bacchi F, Mathé AA, Jiménez P, Stasi L, Arban R, Gerrard P, et al. Anxiolytic-like effect of the selective neuropeptide Y Y2 receptor antagonist Biie0246 in the elevated plus-maze. Peptides. (2006) 27:3202–7. doi: 10.1016/j.peptides.2006.07.020
25. Michaelson SD, Miranda Tapia AP, McKinty A, Silveira Villarroel H, Mackay JP, Urban JH, et al. Contribution of Npy Y(5) receptors to the reversible structural remodeling of basolateral amygdala dendrites in male rats associated with Npy-mediated stress resilience. J Neurosci. (2020) 40:3231–49. doi: 10.1523/jneurosci.2621-19.2020
26. Cabib S, Puglisi-Allegra S. The mesoaccumbens dopamine in coping with stress. Neurosci Biobehav Rev. (2012) 36:79–89. doi: 10.1016/j.neubiorev.2011.04.012
27. Geracioti TD Jr., Jefferson-Wilson L, Strawn JR, Baker DG, Dashevsky BA, Horn PS, et al. Effect of traumatic imagery on cerebrospinal fluid dopamine and serotonin metabolites in posttraumatic stress disorder. J Psychiatr Res. (2013) 47:995–8. doi: 10.1016/j.jpsychires.2013.01.023
28. Belujon P, Grace AA. Dopamine system dysregulation in major depressive disorders. Int J Neuropsychopharmacol. (2017) 20:1036–46. doi: 10.1093/ijnp/pyx056
29. Floresco SB, Tse MT. Dopaminergic regulation of inhibitory and excitatory transmission in the basolateral amygdala-prefrontal cortical pathway. J Neurosci. (2007) 27:2045–57. doi: 10.1523/jneurosci.5474-06.2007
30. Delva NC, Stanwood GD. Dysregulation of brain dopamine systems in major depressive disorder. Exp Biol Med (Maywood). (2021) 246:1084–93. doi: 10.1177/1535370221991830
31. Moreines JL, Owrutsky ZL, Gagnon KG, Grace AA. Divergent effects of acute and repeated quetiapine treatment on dopamine neuron activity in normal vs. Chronic mild stress induced hypodopaminergic states. Transl Psychiatry. (2017) 7:1275. doi: 10.1038/s41398-017-0039-9
32. Li Y, Zhu ZR, Ou BC, Wang YQ, Tan ZB, Deng CM, et al. Dopamine D2/D3 but not dopamine D1 receptors are involved in the rapid antidepressant-like effects of ketamine in the forced swim test. Behav Brain Res. (2015) 279:100–5. doi: 10.1016/j.bbr.2014.11.016
33. Pare CM, Yeung DP, Price K, Stacey RS. 5-hydroxytryptamine, noradrenaline, and dopamine in brainstem, hypothalamus, and caudate nucleus of controls and of patients committing suicide by coal-gas poisoning. Lancet. (1969) 2:133–5. doi: 10.1016/s0140-6736(69)92442-8
34. Malikowska-Racia N, Sałat K, Nowaczyk A, Fijałkowski Ł, Popik P. Dopamine D2/D3 receptor agonists attenuate Ptsd-like symptoms in mice exposed to single prolonged stress. Neuropharmacology. (2019) 155:1–9. doi: 10.1016/j.neuropharm.2019.05.012
35. Rezitis J, Herzog H, Ip CK. Neuropeptide Y interaction with dopaminergic and serotonergic pathways: interlinked neurocircuits modulating hedonic eating behaviours. Prog Neuropsychopharmacol Biol Psychiatry. (2022) 113:110449. doi: 10.1016/j.pnpbp.2021.110449
36. Quarta D, Leslie CP, Carletti R, Valerio E, Caberlotto L. Central administration of Npy or an Npy-Y5 selective agonist increase in vivo extracellular monoamine levels in mesocorticolimbic projecting areas. Neuropharmacology. (2011) 60:328–35. doi: 10.1016/j.neuropharm.2010.09.016
37. Adewale AS, Macarthur H, Westfall TC. Neuropeptide Y-induced enhancement of the evoked release of newly synthesized dopamine in rat striatum: mediation by Y2 receptors. Neuropharmacology. (2007) 52:1396–402. doi: 10.1016/j.neuropharm.2007.01.018
38. Gu Z, Chu L, Han Y. Therapeutic effect of resveratrol on mice with depression. Exp Ther Med. (2019) 17:3061–4. doi: 10.3892/etm.2019.7311
39. Vollmer LL, Schmeltzer S, Schurdak J, Ahlbrand R, Rush J, Dolgas CM, et al. Neuropeptide Y impairs retrieval of extinguished fear and modulates excitability of neurons in the infralimbic prefrontal cortex. J Neurosci. (2016) 36:1306–15. doi: 10.1523/jneurosci.4955-13.2016
40. Wang Y, Yang Y, Hui L, Tie C, Li F, Xu ZQ, et al. A neuropeptide Y variant (Rs16139) associated with major depressive disorder in replicate samples from Chinese Han population. PloS One. (2013) 8:e57042. doi: 10.1371/journal.pone.0057042
41. Treutlein J, Strohmaier J, Frank J, Witt SH, Rietschel L, Forstner AJ, et al. Association between neuropeptide Y receptor Y2 promoter variant Rs6857715 and major depressive disorder. Psychiatr Genet. (2017) 27:34–7. doi: 10.1097/ypg.0000000000000149
42. Sato N, Kageyama S, Chen R, Suzuki M, Mori H, Tanioka F, et al. Association between neuropeptide Y receptor 2 polymorphism and the smoking behavior of elderly Japanese. J Hum Genet. (2010) 55:755–60. doi: 10.1038/jhg.2010.108
43. Wetherill L, Schuckit MA, Hesselbrock V, Xuei X, Liang T, Dick DM, et al. Neuropeptide Y receptor genes are associated with alcohol dependence, alcohol withdrawal phenotypes, and cocaine dependence. Alcohol Clin Exp Res. (2008) 32:2031–40. doi: 10.1111/j.1530-0277.2008.00790.x
44. McCullough KM, Daskalakis NP, Gafford G, Morrison FG, Ressler KJ. Cell-type-specific interrogation of Cea Drd2 neurons to identify targets for pharmacological modulation of fear extinction. Transl Psychiatry. (2018) 8:164. doi: 10.1038/s41398-018-0190-y
45. Roseboom PH, Nanda SA, Fox AS, Oler JA, Shackman AJ, Shelton SE, et al. Neuropeptide Y receptor gene expression in the primate amygdala predicts anxious temperament and brain metabolism. Biol Psychiatry. (2014) 76:850–7. doi: 10.1016/j.biopsych.2013.11.012
46. Zhang X, Han Y, Liu X, Chen J, Yuan Z, Wang Y. Assessment of genetic variants in D2 dopamine receptor (Drd2) gene as risk factors for post-traumatic stress disorder (Ptsd) and major depressive disorder (Mdd): A systematic review and meta-analysis. J Affect Disord. (2023) 328:312–23. doi: 10.1016/j.jad.2023.02.001
47. Wolf EJ, Mitchell KS, Logue MW, Baldwin CT, Reardon AF, Aiello A, et al. The dopamine D3 receptor gene and posttraumatic stress disorder. J Trauma Stress. (2014) 27:379–87. doi: 10.1002/jts.21937
48. Avinun R, Nevo A, Radtke SR, Brigidi BD, Hariri AR. Divergence of an association between depressive symptoms and a dopamine polygenic score in Caucasians and Asians. Eur Arch Psychiatry Clin Neurosci. (2020) 270:229–35. doi: 10.1007/s00406-019-01040-x
49. Auxéméry Y. [Posttraumatic stress disorder (Ptsd) as a consequence of the interaction between an individual genetic susceptibility, a traumatogenic event and a social context]. Encephale. (2012) 38:373–80. doi: 10.1016/j.encep.2011.12.003
50. Mandelli L, Serretti A. Gene environment interaction studies in depression and suicidal behavior: an update. Neurosci Biobehav Rev. (2013) 37:2375–97. doi: 10.1016/j.neubiorev.2013.07.011
51. McCutcheon A. Latent Class Analysis. California: Thousand Oaks (1987). Available at: https://methods.sagepub.com/book/latent-class-analysis.
52. DiMauro J, Carter S, Folk JB, Kashdan TB. A historical review of trauma-related diagnoses to reconsider the heterogeneity of Ptsd. J Anxiety Disord. (2014) 28:774–86. doi: 10.1016/j.janxdis.2014.09.002
53. Contractor AA, Roley-Roberts ME, Lagdon S, Armour C. Heterogeneity in patterns of dsm-5 posttraumatic stress disorder and depression symptoms: latent profile analyses. J Affect Disord. (2017) 212:17–24. doi: 10.1016/j.jad.2017.01.029
54. Armour C, Contractor A, Elhai JD, Stringer M, Lyle G, Forbes D, et al. Identifying latent profiles of posttraumatic stress and major depression symptoms in Canadian veterans: exploring differences across profiles in health related functioning. Psychiatry Res. (2015) 228:1–7. doi: 10.1016/j.psychres.2015.03.011
55. Hruska B, Irish LA, Pacella ML, Sledjeski EM, Delahanty DL. Ptsd symptom severity and psychiatric comorbidity in recent motor vehicle accident victims: A latent class analysis. J Anxiety Disord. (2014) 28:644–9. doi: 10.1016/j.janxdis.2014.06.009
56. American Psychiatric Association D, American Psychiatric Association. Diagnostic and statistical manual of mental disorders: DSM-5, Washington, DC: American psychiatric association Press (2013) 947 p.
57. Blevins CA, Weathers FW, Davis MT, Witte TK, Domino JL. The posttraumatic stress disorder checklist for Dsm-5 (Pcl-5): development and initial psychometric evaluation. J Trauma Stress. (2015) 28:489–98. doi: 10.1002/jts.22059
58. Wilkins KC, Lang AJ, Norman SB. Synthesis of the psychometric properties of the Ptsd checklist (Pcl) military, civilian, and specific versions. Depress Anxiety. (2011) 28:596–606. doi: 10.1002/da.20837
59. Cao X, Wang L, Cao C, Zhang J, Liu P, Zhang B, et al. Patterns of Dsm-5 posttraumatic stress disorder and depression symptoms in an epidemiological sample of Chinese earthquake survivors: A latent profile analysis. J Affect Disord. (2015) 186:58–65. doi: 10.1016/j.jad.2015.06.058
60. Radloff LS. The Ces-D scale:A self-report depression scale for research in the general population. Appl psychol Measurement. (1977) 1:385–401. doi: 10.1177/014662167700100306
61. Lewinsohn PM, Seeley JR, Roberts RE, Allen NB. Center for epidemiologic studies depression scale (Ces-D) as a screening instrument for depression among community-residing older adults. Psychol Aging. (1997) 12:277–87. doi: 10.1037//0882-7974.12.2.277
62. Chi I. Mental health of the old-old in Hong Kong. Clin Gerontologist: J Aging Ment Health. (1995) 15:31–44. doi: 10.1300/J018v15n03_04
63. Domschke K, Hohoff C, Jacob C, Maier W, Fritze J, Bandelow B, et al. Chromosome 4q31-34 panic disorder risk locus: association of neuropeptide Y Y5 receptor variants. Am J Med Genet B Neuropsychiatr Genet. (2008) 147b:510–6. doi: 10.1002/ajmg.b.30629
64. Abijo T, Blum K, Gondré-Lewis MC. Neuropharmacological and neurogenetic correlates of opioid use disorder (Oud) as a function of ethnicity: relevance to precision addiction medicine. Curr Neuropharmacol. (2020) 18:578–95. doi: 10.2174/1570159x17666191118125702
65. Zhang J, Yan P, Li Y, Cai X, Yang Z, Miao X, et al. A 35.8 kilobases haplotype spanning Ankk1 and Drd2 is associated with heroin dependence in Han Chinese males. Brain Res. (2018) 1688:54–64. doi: 10.1016/j.brainres.2018.03.017
66. Chukwueke CC, Kowalczyk WJ, Di Ciano P, Gendy M, Taylor R, Heishman SJ, et al. Exploring the role of the Ser9gly (Rs6280) dopamine D3 receptor polymorphism in nicotine reinforcement and cue-elicited craving. Sci Rep. (2020) 10:4085. doi: 10.1038/s41598-020-60940-4
67. M N, Patil AN, Pattanaik S, Kaur A, Banerjee D, Grover S. Abcb1 and Drd3 polymorphism as a response predicting biomarker and tool for pharmacogenetically guided clozapine dosing in Asian Indian treatment resistant schizophrenia patients. Asian J Psychiatr. (2020) 48:101918. doi: 10.1016/j.ajp.2019.101918
68. Garg UC, Hanson NQ, Tsai MY, Eckfeldt JH. Simple and rapid method for extraction of DNA from fresh and cryopreserved clotted human blood. Clin Chem. (1996) 42:647–8. doi: 10.1093/clinchem/42.4.647
69. Als TD, Kurki MI, Grove J, Voloudakis G, Therrien K, Tasanko E, et al. Depression pathophysiology, risk prediction of recurrence and comorbid psychiatric disorders using genome-wide analyses. Nat Med. (2023) 29:1832–44. doi: 10.1038/s41591-023-02352-1
70. Kang Y, Wang F, Li C, Hu L, Xu J, Tan X, et al. Dimorphisms of NPY 2 R gene unassociated with alcohol dependence in Chinese Han population. Int J Clin Exp Med. (2016) 9:4527–4532. Available at: https://www.ijcem.com/files/ijcem0015894.pdf.
71. Nutt DJ, Lingford-Hughes A, Erritzoe D, Stokes PR. The dopamine theory of addiction: 40 years of highs and lows. Nat Rev Neurosci. (2015) 16:305–12. doi: 10.1038/nrn3939
72. Qi XL, Xuan JF, Xing JX, Wang BJ, Yao J. No association between dopamine D3 receptor gene Ser9gly polymorphism (Rs6280) and risk of schizophrenia: an updated meta-analysis. Neuropsychiatr Dis Treat. (2017) 13:2855–65. doi: 10.2147/ndt.S152784
73. Kaur G, Singh Chavan B, Gupta D, Sinhmar V, Prasad R, Tripathi A, et al. An association study of dopaminergic (Drd2) and serotoninergic (5-Ht2) gene polymorphism and schizophrenia in a North Indian population. Asian J Psychiatr. (2019) 39:178–84. doi: 10.1016/j.ajp.2018.10.022
74. Jönsson EG, Nöthen MM, Grünhage F, Farde L, Nakashima Y, Propping P, et al. Polymorphisms in the dopamine D2 receptor gene and their relationships to striatal dopamine receptor density of healthy volunteers. Mol Psychiatry. (1999) 4:290–6. doi: 10.1038/sj.mp.4000532
75. Mallet J, Gorwood P, Le Strat Y, Dubertret C. Major depressive disorder (Mdd) and schizophrenia- addressing unmet needs with partial agonists at the D2 receptor: A review. Int J Neuropsychopharmacol. (2019) 22:651–64. doi: 10.1093/ijnp/pyz043
76. Mizrahi R, Rusjan P, Agid O, Graff A, Mamo DC, Zipursky RB, et al. Adverse subjective experience with antipsychotics and its relationship to striatal and extrastriatal D2 receptors: A pet study in schizophrenia. Am J Psychiatry. (2007) 164:630–7. doi: 10.1176/ajp.2007.164.4.630
77. Utsunomiya K, Shinkai T, Sakata S, Yamada K, Chen HI, De Luca V, et al. Genetic association between the dopamine D3 receptor gene polymorphism (Ser9gly) and tardive dyskinesia in patients with schizophrenia: A reevaluation in East Asian populations. Neurosci Lett. (2012) 507:52–6. doi: 10.1016/j.neulet.2011.11.050
78. Park CI, Kim HW, Hwang SS, Kang JI, Kim SJ. Influence of dopamine-related genes on craving, impulsivity, and aggressiveness in Korean males with alcohol use disorder. Eur Arch Psychiatry Clin Neurosci. (2021) 271:865–72. doi: 10.1007/s00406-019-01072-3
79. Savitz J, Hodgkinson CA, Martin-Soelch C, Shen PH, Szczepanik J, Nugent A, et al. The functional Drd3 Ser9gly polymorphism (Rs6280) is pleiotropic, affecting reward as well as movement. PLoS One. (2013) 8:e54108. doi: 10.1371/journal.pone.0054108
80. Verma D, Wood J, Lach G, Mietzsch M, Weger S, Heilbronn R, et al. Npy Y2 receptors in the central amygdala reduce cued but not contextual fear. Neuropharmacology. (2015) 99:665–74. doi: 10.1016/j.neuropharm.2015.08.038
81. Maina FK, Mathews TA. A functional fast scan cyclic voltammetry assay to characterize dopamine D2 and D3 autoreceptors in the mouse striatum. ACS Chem Neurosci. (2010) 1:450–62. doi: 10.1021/cn100003u
Keywords: PTSD-MDD comorbidity, gene × gene interaction, single nucleotide polymorphism, neuropeptide Y, dopamine
Citation: Xu L, Zhang J, Yang H, Cao C, Fang R, Liu P, Luo S, Wang B, Zhang K and Wang L (2024) Epistasis in neurotransmitter receptors linked to posttraumatic stress disorder and major depressive disorder comorbidity in traumatized Chinese. Front. Psychiatry 15:1257911. doi: 10.3389/fpsyt.2024.1257911
Received: 13 July 2023; Accepted: 14 February 2024;
Published: 29 February 2024.
Edited by:
Albert Joseph Arias, Yale University, United StatesReviewed by:
Xiaobin Ding, Northwest Normal University, ChinaKhethelo Richman Xulu, University of the Witwatersrand, South Africa
Copyright © 2024 Xu, Zhang, Yang, Cao, Fang, Liu, Luo, Wang, Zhang and Wang. This is an open-access article distributed under the terms of the Creative Commons Attribution License (CC BY). The use, distribution or reproduction in other forums is permitted, provided the original author(s) and the copyright owner(s) are credited and that the original publication in this journal is cited, in accordance with accepted academic practice. No use, distribution or reproduction is permitted which does not comply with these terms.
*Correspondence: Kunlin Zhang, zhangkl@psych.ac.cn; Li Wang, wangli1@psych.ac.cn