- 1Department of Psychiatry and Psychology, Mayo Clinic, Phoenix, AZ, United States
- 2Department of Neuroscience, Graduate School of Biomedical Sciences, Mayo Clinic College of Medicine, Phoenix, AZ, United States
- 3Neuroimaging Research Branch, National Institute on Drug Abuse, National Institutes of Health, Baltimore, MD, United States
- 4Department of Quantitative Health Sciences, Mayo Clinic Rochester, Rochester, MN, United States
- 5Institute of Sports and Sports Science, Karlsruhe Institute of Technology, Karlsruhe, Germany
- 6Department of Neurology, and the Franke Barrow Global Neuroscience Education Center, Barrow Neurological Institute, Phoenix, AZ, United States
Background: We investigated a potential sex difference in the relationship between alcohol consumption, brain age gap and cognitive function in older adults without cognitive impairment from the population-based Mayo Clinic Study of Aging.
Methods: Self-reported alcohol consumption was collected using the food-frequency questionnaire. A battery of cognitive testing assessed performance in four different domains: attention, memory, language, and visuospatial. Brain magnetic resonance imaging (MRI) was conducted using 3-T scanners (Signa; GE Healthcare). Brain age was estimated using the Brain-Age Regression Analysis and Computational Utility Software (BARACUS). We calculated the brain age gap as the difference between predicted brain age and chronological age.
Results: The sample consisted of 269 participants [55% men (n=148) and 45% women (n=121) with a mean age of 79.2 ± 4.6 and 79.5 ± 4.7 years respectively]. Women had significantly better performance compared to men in memory, (1.12 ± 0.87 vs 0.57 ± 0.89, P<0.0001) language (0.66 ± 0.8 vs 0.33 ± 0.72, P=0.0006) and attention (0.79 ± 0.87 vs 0.39 ± 0.83, P=0.0002) z-scores. Men scored higher in visuospatial skills (0.71 ± 0.91 vs 0.44 ± 0.90, P=0.016). Compared to participants who reported zero alcohol drinking (n=121), those who reported alcohol consumption over the year prior to study enrollment (n=148) scored significantly higher in all four cognitive domains [memory: F3,268 = 5.257, P=0.002, Language: F3,258 = 12.047, P<0.001, Attention: F3,260 = 22.036, P<0.001, and Visuospatial: F3,261 = 9.326, P<0.001] after correcting for age and years of education. In addition, we found a significant positive correlation between alcohol consumption and the brain age gap (P=0.03). Post hoc regression analysis for each sex with language z-score revealed a significant negative correlation between brain age gap and language z-scores in women only (P=0.008).
Conclusion: Among older adults who report alcohol drinking, there is a positive association between higher average daily alcohol consumption and accelerated brain aging despite the fact that drinkers had better cognitive performance compared to zero drinkers. In women only, accelerated brain aging is associated with worse performance in language cognitive domain. Older adult women seem to be vulnerable to the negative effects of alcohol on brain structure and on certain cognitive functions.
1 Introduction
Recent epidemiological studies document substantial growth in the older adult population in the United States and worldwide (1, 2). Older women seem to enjoy more healthy aging compared to men (3), who tend to have a higher prevalence of mild cognitive impairment (MCI) (4). However, women tend to live longer and the prevalence of all-type dementia in women aged 60 to 69 years is almost double that in men (5). Chronic heavy alcohol drinking is a known risk factor for MCI (6) and for the transition from MCI to dementia (7).
Recent data show robust increase in the prevalence of excessive alcohol drinking among women (8–12). Women exhibit more sensitivity to alcohol; with sooner progression to alcohol-related diseases at less drinking levels compared to men (13–15). The deleterious effects of excessive alcohol drinking on cognitive functions are well documented (16–19) specifically in women (20, 21). However, the association between social drinking and the risk of cognitive decline remains controversial (22–24). Social drinking refers to drinking patterns that are accepted by the society in which they occur and has often been confused with the concept of moderate drinking which may be defined as drinking that does not generally cause problems, either for the drinker or for society (25, 26).
While several large-scale studies in elderly population suggest that moderate drinking could be associated with better cognitive performance compared to non-drinkers (6, 27–40), other studies found no cognitive benefit for alcohol drinking (41, 42) and no significant correlation between alcohol consumption and cognitive performance (43, 44). We have recently reported that low-level alcohol consumption is associated with premature brain aging (45). Others have reported similar findings in patients with alcohol use disorder (46).
Premature, advanced, or accelerated brain aging are terms used to describe the gap between chronological age and estimated brain age derived from different machine learning algorithms. Accelerated brain aging is a non-specific observation that has been reported in many conditions such as major depression (47–50), psychosis (51, 52), obsessive compulsive disorder (53), traumatic brain injury (54, 55), tobacco smoking (56), diabetes mellitus (57, 58), glioma patients following radiotherapy (59), and in healthy individuals with inadequate sleep (60). Few studies have reported negative association between accelerated brain aging and cognitive performance in patients with major psychoses (51), in older patients with depressive symptoms (61) and in patients with traumatic brain injury (54).
The relationship between casual drinking, brain aging, and cognitive function in older men and women received little attention despite rich literature documenting sex differences in brain morphology (62–66), and the effect of alcohol on brain structure (67, 68) and cognitive performance (21). Therefore, this study aimed to investigate the relationship between alcohol consumption, brain age gap and cognitive function in older adults free of cognitive impairment from the Mayo Clinic Study of Aging (MCA). We hypothesized that the degree of alcohol drinking will directly correlate with the brain age gap and the relationship between brain age gap and certain cognitive domains will be sex dependent.
2 Methods
2.1 Study setting
The study was conducted in the setting of the population-based Mayo Clinic Study of Aging (MCSA) in Olmsted County, Minnesota. The Mayo Clinic Study of Aging (MCSA) is a population-based study that utilized the medical records-linkage system to construct a sampling frame of Olmsted County residents aged 70–89 on October 1, 2004, who had been in contact with the system at least once within the 3 years prior to the index date. Duplicate records for the same persons were identified and excluded and a single record was created for each resident. Addresses of residences located on the border of Olmsted County were manually checked by a nurse abstractor to exclude subjects who were nonresidents and unique individuals were enumerated in the study sampling frame. The medical records of subjects selected for the study that were archived by the records-linkage system were screened to identify dementia. If a diagnosis of dementia was identified or suspected, the complete medical record was reviewed by a behavioral neurologist to confirm the diagnosis of dementia. Persons with a confirmed diagnosis of dementia were not contacted for participation. Subjects presumed to be free of dementia were sent a letter of invitation that briefly described the rationale and purpose of the study. This letter of invitation was followed by a recruitment telephone call, and finally an in-person evaluation or a telephone assessment. Both the study participant and informant are interviewed (69). Current or previous symptoms of anxiety, depression, or alcoholism were assessed using screening instruments such as the Patient Health Questionnaire version of the PRIME MD (70), the Beck Depression Inventory (71) and the Beck Anxiety Inventory (72). Subjects who screened positive for psychiatric symptoms were assessed further by a physician using the Clinician Evaluation Guideline component of the PRIME MD, a semistructured interview that generates Diagnostic and Statistical Manual of Mental Disorders – IV (DSM-IV) (73) diagnoses for depressive disorders, anxiety disorders or alcohol use disorders (70). The details of study design, sampling and measures have been described in the Methodology paper of the Mayo Clinic Study of aging (69). For this analysis, we considered participants aged 70 years and older and free of cognitive impairment. Enrolled participants underwent a comprehensive in-person evaluation including the Clinical Dementia Rating Scale, alcohol consumption pattern over the past year, a neurological evaluation and neuropsychological testing to assess four cognitive domains: memory, executive function, language, and visuospatial skills (4, 69). Data included in this analysis was collected between March 2006 and March 2022.
2.2 Alcohol consumption history
Participants completed a 128-item food-frequency questionnaire (74) which is a modification of the Block 1995 Revision of the Health Habits and History Questionnaire (75). The questionnaire included 25 beverages and focused on usual drinking habits during the year prior to enrollment. Alcoholic beverages were categorized into four groups: red wine, white wine, beer, and liquor. Participants were asked to provide the average number of servings per month in each category over the year prior to study enrollment. Ethanol content in each serving was calculated as follows: For red wine [volume per serving = 147.9 mL* ethanol concentration per mL = 0.106 gm], for white wine [volume per serving = 147.9 mL* ethanol concentration per mL = 0.103 gm], for beer [volume per serving = 354.9 mL* ethanol concentration per mL = 0.039 gm], and for liquor [volume per serving = 44.4 mL* ethanol concentration per mL = 0.347 gm]. Total monthly ethanol consumption was calculated by summing all consumed alcohol in all four categories. Finally, average daily ethanol intake (in gm) was obtained by dividing the monthly consumption over 30 days.
2.3 Cognitive testing
All the participants included in the study are cognitively normal older adults, and their Clinical Dementia Rating (CDR) score is 0. The Methodology paper of Mayo Clinic Study of Aging (69) describes, in detail, the protocol we follow in classifying a person to be cognitively normal or having Mild Cognitive Impairment or dementia based on published criteria. A weekly multidisciplinary consensus panel classifies a person to be cognitively unimpaired, or MCI or dementia based on published criteria.
Neuropsychological testing was performed using nine validated tests to assess impairment in four domains (A) attention using (1) Trail Making Test B (76) and (2) Digit Symbol Substitution Test (77); (B) language using (3) Boston Naming Test (78) and (4) Category Fluency (79); (C) memory using (5) Logical Memory-II (delayed recall), (6) Visual Reproduction-II (delayed recall) (80) and (7) Auditory Verbal Learning Test (delayed recall) (81), and (D) visuospatial using (8) Picture Completion and (9) Block Design (77). The raw scores on each test were transformed into an age-adjusted score using normative data from Mayo’s Older American Normative Studies and were scaled to have a mean of 10 and a SD of 3 (81). Domain scores were computed by summing the adjusted and scaled scores within a domain and scaling the combined scores to allow comparisons across domains (4, 69, 82).
2.4 Severity weighted sum of disease
Medical comorbidity was measured using the Charlson index, which is a widely used weighted index that takes into account the number and severity of diseases (83).
2.5 MRI data acquisition
Magnetic resonance imaging (MRI) was performed using 3-T scanners (Signa; GE Healthcare) equipped with an 8-channel phased array coil (GE Healthcare). A 3-dimensional magnetization–prepared rapid gradient echo sequence was performed (84, 85) with the following sequence: TR = 200 ms, TE = 20 ms, section thickness = 3.3 mm with 49 sections, flip angle = 20° and in-plane matrix of 256 × 224 (86). All images have the following resolution (x,y,z)= 1.016 × 1.016 × 1.200 mm3. Images were corrected for distortion due to gradient nonlinearity and for bias field (87).
2.6 Brain age prediction
The Brain-Age Regression Analysis and Computational Utility Software (BARACUS version 1.1.4; https://github.com/BIDS-Apps/baracus (88)) was used to generate each participant’s brain age prediction. The software requires a T1-weighted structural MRI to compute the brain age prediction. In the first step, the software processes the structural MRI using FreeSurfer version 5.3. The FreeSurfer uses three atlas: Desikan-Killiany Atlas, DKT Atlas and Destrieux Atlas (CorticalParcellation - Free Surfer Wiki (harvard.edu)) to generate its output. The FreeSurfer output is then used to generate the brain age prediction, with several model options. The prediction model was trained on the BARACUS database (default model: Liem2016:OCI_norm). For more information on the prediction model, see Liem et al. (89). Scans were processed using the computational resources of the NIH High Performance Computing (HPC) Biowulf cluster (http://hpc.nih.gov).
2.7 Statistical analyses
2.7.1 Characterization measures
Clinical characterization, alcohol consumption and performance in cognitive domains (z-scores) were first tested for normality using Shapiro-Wilk’s test. Data are shown as mean ( ± SD) if normally distributed and as median and interquartile range if not normally distributed. Unpaired t-tests or Mann Whitney U tests were used to compare clinical variables’ means or medians respectively between men and women and between drinkers and zero-drinkers. We used univariate analysis with age and years of education as covariates to compare performance in cognitive tests between drinkers and zero-drinkers.
2.7.2 Age gap
The brain age gap was calculated by subtracting the chronological age from the predicted age derived from BARACUS. Further analyses were conducted in R (version 4.2.0; (90)). To ensure generalizability of BARACUS to our sample, we conducted a Pearson correlation analysis comparing the predicted brain age to the chronological age. The relationship between the brain age gap, average total daily ethanol (gm) consumed over the preceding year, and cognitive function domain z-scores was assessed using linear regression via the lm function in the stats package for R (90). We included chronological age, chronological age squared (because the relationship between predicted and chronological age is not perfectly linear (89), sex, years of education, smoke status, BMI, and comorbid medical condition (severity weighted sum of disease) as covariates for the regression models. Participants who reported zero alcohol consumption over the past 12 months were excluded from this analysis. Post hoc regression analysis for each sex was then used to untangle a potential sex difference on the effect of alcohol consumption on brain age gap and cognitive function.
Results from the statistical analyses were considered statistically significant at P<0.05.
3 Results
3.1 Demographics
A total of 269 participants were sorted into two groups based on their sex with 55% (n=148) men and 45% women (n=121). Both groups did not display statistically significant differences in terms of their median age at time of MRI [median (IQ range): 78.6 (7.6) in men vs 78.2 (7.0) in women], age at time of cognitive testing, body mass index (BMI), or severity weighted sum of diseases. Significantly more men reported history of smoking (47.3% in men vs 35.5% in women). Fifty five percent of participants reported alcohol drinking and 45% reported zero alcohol consumption over the past over the past 12 months. Compared to zero drinkers, drinkers were younger (P=0.002), had significantly more years of education (P=0.009). Significantly more drinkers reported smoking (47.9% in drinkers vs 42% in zero drinkers), but scored lower in the severity weighted sum of diseases (P=0.02). See Table 1 for more details.
3.2 Performance in cognitive tests
All four cognitive domains had highly significant intercorrelations (P<0.001 each) as shown in Supplementary Table S1. Compared to men, women participants scored significantly higher in all cognitive domains [memory z-score (mean ± SD: 1.12 ± 0.87 in women vs 0.57 ± 0.89 in men), language z-score (mean ± SD: 0.66 ± 0.8 in women vs 0.33 ± 0.72 in men), and attention z-score (mean ± SD: 0.79 ± 0.87 in women vs 0.39 ± 0.83 in men)] except in visuospatial tasks where men performed better than women (mean ± SD: 0.71 ± 0.91 in men vs 0.44 ± 0.90 in women). Comparing zero drinkers with drinkers shows that drinkers scored significantly higher in language (mean ± SD: 0.57 ± 0.74 in drinkers vs 0.37 ± 0.79 in zero drinkers), and attention (mean ± SD: 0.7 ± 0.75 in drinkers vs 0.42 ± 0.98in zero drinkers). No significant differences were observed between zero drinkers and drinkers in memory or visuospatial domains. Since the alcohol drinking group were significantly younger with more years of education compared to the non-alcohol drinkers, we repeated the analysis using both age and years of education as covariates. The results show drinkers performed better in all four cognitive domains [memory: F3,268 = 5.257, P=0.002, Language: F3,258 = 12.047, P<0.001, Attention: F3,260 = 22.036, P<0.001, and Visuospatial: F3,261 = 9.326, P<0.001] (Table 2).
3.3 Sex differences in demographics, alcohol consumption and performance in cognitive tests among individuals who reported alcohol drinking over the year prior to enrollment
Among participants who reported alcohol drinking over the past year, 58% (n=86) were men and 42% (n=62) were women. No differences between the two groups were observed in median age at time of MRI, years of education, BMI, smoking history, or severity weighted sum of diseases. men alcohol drinkers reported consuming more average daily beer [median (IQ range): 0.83 (3.7) g/d in men vs 0.0 (0.83) g/d in women) and more total average daily alcohol [median (IQ range): 7.58 (14.75) g/d in men vs 4.15 (7.58) g/d in women]. Women reported drinking more white wine compared to men [median (IQ range): 0.46 (1.33) g/d in women vs 0.0 (0.91) g/d in men]. Compared to men, women participants who reported alcohol drinking over the past year had better memory (mean ± SD: 1.12 ± 0.86 in women vs 0.61 ± 0.9 in men), language (mean ± SD: 0.74 ± 0.76 in women vs 0.45 ± 0.7 in men), and attention (mean ± SD: 0.92 ± 0.78 in women vs 0.54 ± 0.69 in men). Men performed better in visuospatial domain (mean ± SD: 0.87 ± 0.89 in men vs 0.44 ± 1.01 in women) (Table 3).
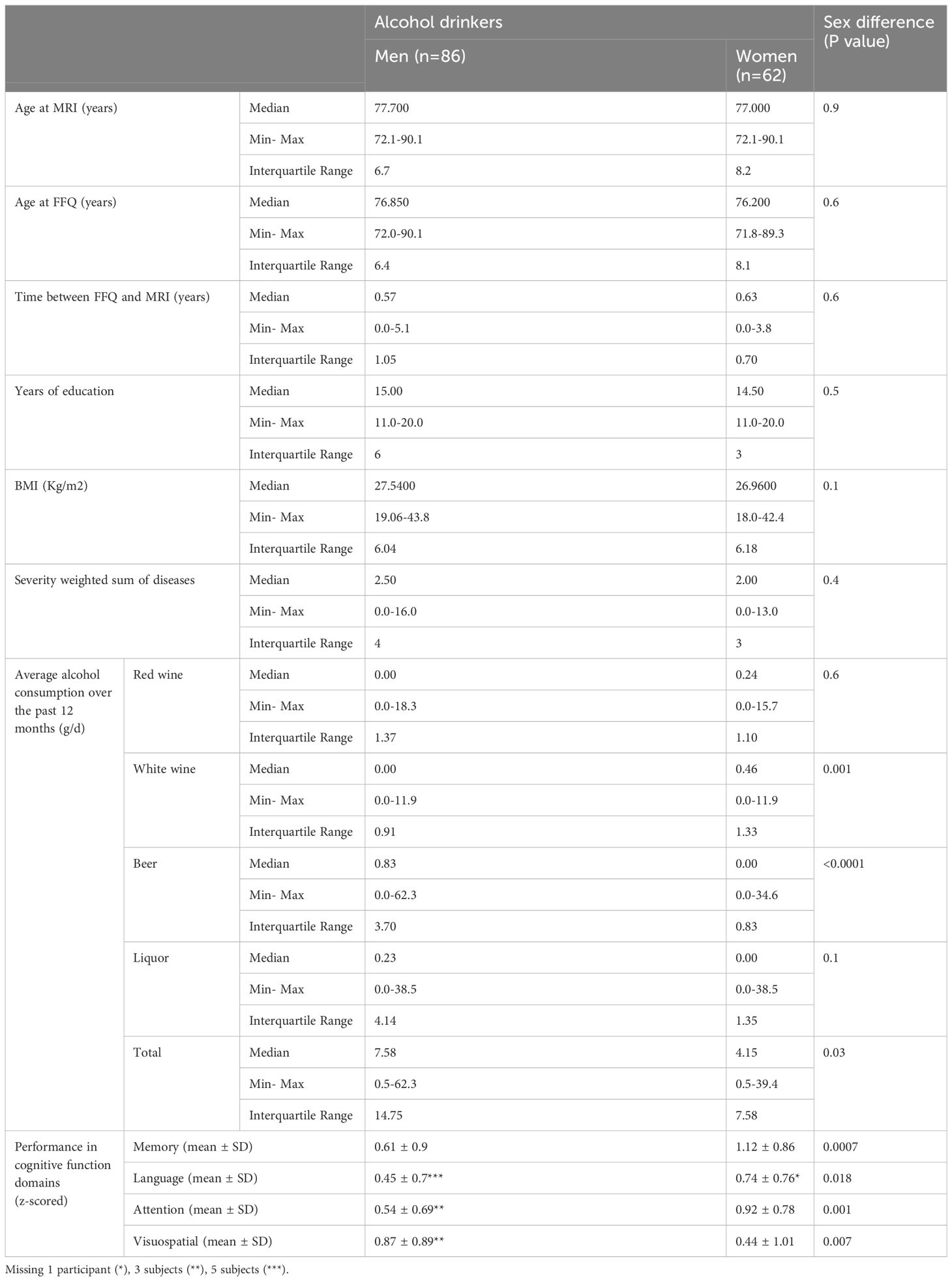
Table 3 Demographics, average daily drinking and cognitive performance in participants who reported alcohol drinking over the past 12 months.
3.4 Testing the utility of BARACUS in predicting brain age in this cohort of elderly individuals
Since the mean age of our study participants is 79.4 ± 4.7 years, which is significantly older compared to the training cohort used for BARACUS development, we examined the correlation between chronological age and brain age in all four outputs. Stacked anatomy had an intrinsic ceiling effect at age 75.6 years (Supplementary Figure S1) as previously reported (89). Fortunately, the other three prediction outputs (cortical area, cortical thickness, or subcortical volume) predicted brain age with robust correlations (Supplementary Figures S2A–C), so those were included in further analysis. We calculated brain age gap as the difference between predicted brain age and chronological age.
3.5 The association between brain age gaps and average alcohol consumption over the past 12 month
For this analysis, we excluded from the original cohort (n=269) participants that reported zero alcohol consumption over the past year (n=121), leading to a final sample of 148. Besides the covariates mentioned in the methods section, interaction of sex and alcohol consumption was also included in each model. Significant positive association was observed only between the age gap, calculated from the predicted age “cortical thickness”, and alcohol consumption (β = 0.2, P = 0.03). However, the association of age gap and alcohol consumption was not significant when age gap was calculated either from “cortical area” (β = 0.02, P = 0.79) or from “subcortical volume” (β = 0.13, P = 0.30). For this reason, the age gap calculated from “cortical thickness” was the only one considered for further analysis.
3.6 The association between average alcohol consumption over the past year, brain age gap and cognitive function z-scores
Using cortical thickness prediction output, we examined the correlation between brain age gap and alcohol consumption with cognitive measures for the cohort of 148 participants who reported alcohol consumption on the past 12 month. For this purpose, the z-scores of four different cognitive domains: memory, language, attention and visuospatial were used in four different models. In addition to the covariates mentioned in the methods, interaction of sex and alcohol consumption and of sex and the respective cognitive measures were also included in each model. We found significant positive correlation between alcohol consumption and brain age gap in all four models: memory (β = 0.20, P=0.033), attention (β = 0.20, P=0.038), Visual spatial (β = 0.20, P=0.039) and language (β = 0.19, P=0.037). However, only language domain z-score showed significant correlation with age gap (β = -1.92, P=0.0454). In addition, we also found significant association between age gap and language-sex interaction (β = 2.91, P=0.0261).
Post hoc regression analysis for each sex (82 men and 61 women), of age gap with alcohol consumption and language z-score, including the covariates mentioned in the methods, revealed significant negative correlation between brain age gap and language z-scores in women only (β = -2.54, P=0.00876, Pcorr = 0.017 (Pcorr = P-value corrected for multiple comparison)) (Figure 1).
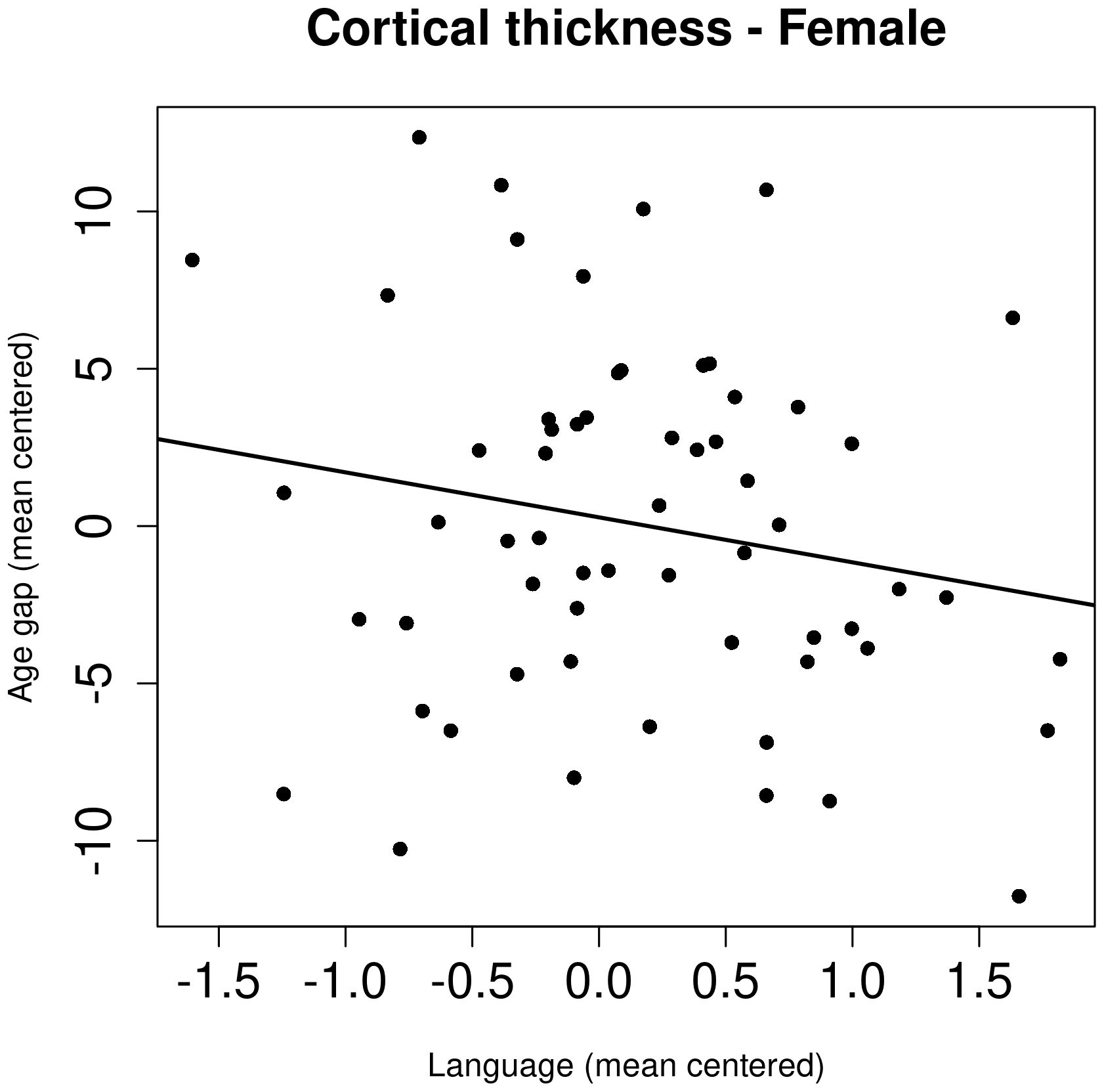
Figure 1 The association between brain age gap, calculated from cortical thickness, and language z-score in female participants. The age gap and language z-score were mean-centered.
4 Discussion
The results of this study lend more evidence to prior reports that women perform better in memory, language, and attention cognitive tasks (91–94), while men tend to have higher scores in visual-spatial domain (94, 95). In addition, older individuals who reported social drinking over the 12 months prior to study enrollment performed better in language and attention compared to those who reported zero drinking during the same time interval (6, 27–40). Furthermore, these data also replicate our recent results showing a direct correlation between higher daily alcohol consumption and brain age gap, i.e., accelerated brain aging (45). In addition, we show here that the relationship between brain age gap and certain cognitive functions is sex-dependent where we observed significant negative correlation between brain age gap and language z-score only in women. These results of sex-specific effect of brain age gap on cognitive function support our hypothesis and lend more evidence to the concept of women’s vulnerability to alcohol drinking in in a range of cognitive process (96). More importantly, our study and others (62–66, 97) bring more attention to the need for sex-difference studies in brain morphology differences with aging.
4.1 Social drinkers, compared to zero-drinkers, score higher in cognitive tasks
Our results are consistent with a large body of literature documenting that moderate alcohol consumption is associated with better cognition. Large-scale studies such as the Nurses’ Health Study (n=12,480, age range 70-81 years, with follow up at 2 years), reported that moderate (<15 g alcohol/day) drinkers had better mean cognitive scores than nondrinkers and the relative risk of a substantial decline in performance over a two-year period was significantly less [0.85 (95% CI: 0.74-0.98)] among moderate drinkers, as compared with nondrinkers (27). Similar results were found in a large-scale community-based study in Ireland, the Netherlands, and Scotland (n=5804, with 3000 women, aged 70-82 years, followed up for 3.2 years). In that study, cognitive performance was better for women drinkers than nondrinkers for all cognitive domains over the 3.2-year follow-up; no significant effects were seen for men. The rate of cognitive decline was similar for drinkers and nondrinkers for all cognitive domains, except for MMSE, which declined significantly less in women drinkers than nondrinkers (28). In contrast, the Health and Retirement Study (n= 19,887 with 11,943 women who underwent serial cognitive assessments over 12 years between 1996 and 2008) showed that low to moderate drinkers (<8 drinks/week in women and < 15 drinks/week in men), compared with never drinkers, were less likely to have a consistently low cognitive trajectory and also had decreased annual rates of total cognitive function decline (40).In addition, four heart studies [Honolulu Heart Program (n=3556 aged 71 to 93 years) (29), Caerphilly Prospective Study of Heart Disease and stroke (n=1870 men aged 55-69 years) (30), Framingham Heart Study (n=1788, aged 55-88 years) (31) Cardiovascular Health Study (n=373 cases with incident dementia and 373 controls aged 65 years and older) (32)] and the Vascular Aging Study (n=1389 aged 59-71 years) (33), all showed better cognitive performance or decreased probability of cognitive impairment or decreased odds for the incidence of dementia in moderate drinkers compared to both non-drinkers and heavy drinkers. Similar results were reported in several population-based studies (6, 34–39).
There are several, non-biological factors that could explain this intriguing observation. For example, non-drinkers could be former drinkers who stopped because of alcohol-related complications. Similarly, socioeconomic status could mediate some of the supposedly beneficial effects of alcohol on cognition since individuals with higher socioeconomic status are more likely to afford alcohol drinking compared to those with lower socioeconomic status. Years of education could be another factor.
Individuals who reported alcohol drinking (compared to zero drinkers) had more years of education and performed significantly better in all four cognitive domains. This observation could be related to the cognitive reserve hypothesis, which postulates that the brains of individuals with more years of education, leisure or mentally stimulating activities, and more complex occupations, among other factors, are more tolerant to age-related changes with less likelihood of developing mild cognitive impairment and dementia (98–100). Kaur et al. reported a significant difference in the cognitive reserve between those with normal and abnormal cognitive function in a cohort of community dwelling older adults (≥60 years, n=370) (101). In a meta-analysis by Meng and D’Arcy, individuals with lower education had a higher risk for dementia, the pooled OR was 2.61 (95%CI 2.21– 3.07) for prevalence studies and 1.88 (95%CI 1.51–2.34) for incidence studies (102). Similarly, high education was associated with a decreased rate of post-stroke dementia in a recent systematic review and meta-analysis (103).
It is important here to note that several studies did not find any cognitive benefit for alcohol drinking. In the Women’s Health Initiative Memory Study (n=4461 elderly women aged 65-79 years, followed for an average of 4.2 years), compared with no alcohol intake, intake of one or more drinks per day was associated with higher baseline Modified Mini-Mental State Examination scores (p < 0.001) and a covariate-adjusted odds ratio of 0.40 (95% confidence interval: 0.28, 0.99) was indicative of significant declines in cognitive function (41). A census-based study from Spain (n=3888, mane age = 55 years with longitudinal follow up at 2.5 and 4.5 years) reported no benefit for low-to-moderate alcohol consumption on cognitive decline. Compared to zero drinkers, the adjusted odds ratio for men with less than 12 g alcohol/day and men with 12-24 g alcohol/day and former drinkers were 0.61 (95% confidence interval (CI): 0.31, 1.20), 1.19 (95% CI: 0.61, 2.32), and 1.03 (95% CI: 0.59, 1.82), respectively. Similar results were observed in women, i.e., odds ratios for mild, moderate and former drinkers were 0.88 (95% CI: 0.45, 1.72), 2.38 (95% CI: 0.98, 5.77), and 1.03 (95% CI: 0.48, 2.23) respectively (42). One study from California examined the association of cigarette smoking and alcohol consumption at baseline with risk of poor cognitive function 13-18 years later (n=511). Among women, increasing consumption of alcohol predicted a significant decline in the long-term recall and savings scores of the visual reproduction test. Moderate drinking, approximately two drinks per day, predicted categorically defined poor performance on the Buschke long-term recall task in women. In contrast, alcohol consumption was not associated with cognitive function in men (104). Two small studies from Australia [ (43) (n=209 World War II veteran men) and (44) (n=327 aged ≥75 years)] reported no significant correlation between alcohol consumption and cognitive performance.
Given the inconsistent results and the fact that any potentially beneficial cognitive effects for moderate drinking are outweighed by increased risk for other health conditions, particularly cancer (105), clinicians should err on safety and avoid encouraging social drinking.
4.2 Direct correlation between average daily alcohol consumption and brain age gap
Our results of significant association between the average daily alcohol consumption and brain age gap is in agreement with our recent report of dose-dependent association between social drinking and brain age gap (45) as well as with Guggenmos et al. (46), in patients with alcohol use disorder, and with the Bostrand et al. study utilizing data from the UK Biobank where alcohol use showed a positive association with higher predicted brain age (106). Whether the alcohol-associated aging process extends beyond the brain, remains to be seen. However, recent studies report that alcohol-associated aging could be related to triggering neuroimmune responses, including pro-inflammatory cytokine release (107) which could affect telomere length. This was shown recently by Jung et al. who reported that DNA methylation derived telomere length was 0.06 kilobases shorter per year in patients with alcohol use disorder compared to healthy controls after correcting for BMI and smoking status (p = 0.002) (108). The current study brings new insight by linking brain aging to cognitive performance which is critical for functionality and overall quality of life.
4.3 Inverse correlation between brain age gap and language z-score in women
Our current understanding for the neuroanatomical substrate of sex difference in cognition is limited despite the rich literature documenting sex difference in brain structure (109–111). Furthermore, BARACUS and other brain age estimating algorithms do not provide data on the specific structural correlates of brain age prediction (112). Despite these limitations, one can envision a three-step approach starting by mapping the neuroanatomical locations of cognitive domains, second examining whether structural differences between men and women are reported in these locations and finally studying sex-specific vulnerability of these brain regions to alcohol. We found that brain age gap showed a significant inverse association with language performance in women. In this study, language was tested using Boston Naming (78) and Category Fluency Tests (79). Using functional MRI, Baldo et al. reported that performance on the Boston Naming Test was associated with a network consisting mainly of the left mid to posterior middle temporal gyrus and underlying white matter (113). Language lateralization shows sex difference (114) with more temporal lobe asymmetry in men. Men have larger grey matter volumes in right middle temporal gyrus (115) and alcohol dependence is associated with significant reductions in temporal lobe tissue content (116). In addition, studies have reported sex differences in the effect of aging on brain morphology. For example, men, compared to women, show greater variability in volumetric measures (62, 63), but not in cortical thickness (63). Moreover, while both men and women show decline in hippocampal volume with age (64), men tend to have steeper and significant negative correlation between age and hippocampal volume compared to women in some (65, 66), but not all studies (97). Understanding such sex differences in brain structure, may provide useful information to probe the mechanisms that underlie sex-specific vulnerabilities in mental disorders including the effect of social drinking on cognition in older adults [reviewed in (117)].
5 Limitations and conclusion
The results of this study should be interpreted in the light of its strengths and limitations. Our study has large, well characterized population-based sample with rigorous neuropsychological and neurological testing. However, 98% of the participants are of Caucasian descent and they have relatively highly educated. Our cross-sectional study, despite an agreement with numerous cross-sectional brains aging studies (47–60) cannot make a definitive conclusion about accelerated aging and longitudinal studies are rather needed. In addition, more men, compared to women [58.1% (86/148) vs 51.2% 52/121)] reported alcohol drinking. While this difference is not statistically significant, it could have impacted the power for identifying sex differences in the association between drinking, cognitive function, and brain age gap. All participants were ambulatory, however, the effect of physical activity on cognitive function was not the focus of this study as we, and others, have shown that physical activity may help to improve cognitive function and, consequently, delay the progression of cognitive impairment in the elderly (118–120). Carvalho et al. reviewed 27 studies and reported a significant positive correlation between physical activity and cognitive function in late life (121). In addition, MCSA did not discriminate between social drinking and moderate drinking and did not collect data on alcohol use disorder history or other addictions besides tobacco use. The time interval between brain imaging and neuropsychological testing was long (≥5 years) in few patients. Despite these limitations, our finding of a significant association between higher average daily alcohol consumption and accelerated brain aging, as well as an inverse correlation between larger brain age gap and performance in older women highlights the specific negative effect of social drinking on women.
Data availability statement
The original contributions presented in the study are included in the article/Supplementary Material. Further inquiries can be directed to the corresponding author.
Ethics statement
The studies involving humans were approved by Mayo Clinic Rochester, IRB # 14-004401. The studies were conducted in accordance with the local legislation and institutional requirements. The participants provided their written informed consent to participate in this study.
Author contributions
OA has full access to all data in the study and take responsibility for the integrity of the data and the accuracy of the data analysis. Concept and design: OA. Acquisition, and analysis of data: OA and EC. Interpretation of data: All authors. Drafting of the manuscript: OA. Critical revision of the manuscript for important intellectual content: All authors. All authors contributed to the article and approved the submitted version.
Funding
The author(s) declare financial support was received for the research, authorship, and/or publication of this article. OA is funded internally by Mayo Clinic Arizona. Support for this research was provided by NIH grants: National Institute on Aging (R01AG069453; R01AG057708; U01AG006786; P50 AG016574; R01AG034676; R01AG011378; R01AG041851), National Institute of Mental Health (K01 MH068351), and National Institute of Neurological Disorders and Stroke (R01 NS097495). This project was also supported by the Robert H. and Clarice Smith and Abigail Van Buren Alzheimer’s Disease Research Program, the GHR Foundation, BARROW Neurological Foundation and the Mayo Foundation for Medical Education and Research, and the department of Psychiatry and Psychology at the Mayo Clinic Arizona.
Acknowledgments
We would like to acknowledge all MCSA team specifically Dr. Luke Christenson, PhD for his assistance in data extraction and transfer from MCSA to NIDA. This work was funded in part by the Intramural Research Program of the National Institute on Drug Abuse.
Conflict of interest
The authors declare that the research was conducted in the absence of any commercial or financial relationships that could be construed as a potential conflict of interest.
Publisher’s note
All claims expressed in this article are solely those of the authors and do not necessarily represent those of their affiliated organizations, or those of the publisher, the editors and the reviewers. Any product that may be evaluated in this article, or claim that may be made by its manufacturer, is not guaranteed or endorsed by the publisher.
Supplementary material
The Supplementary Material for this article can be found online at: https://www.frontiersin.org/articles/10.3389/fpsyt.2024.1235171/full#supplementary-material
Supplementary Figure 1 | Correlation between chronological age and predicted brain age using stacked anatomy.
Supplementary Figure 2 | Correlation between chronological age and predicted brain age using (A) cortical area, (B) cortical thickness, and (C) subcortical volume.
Supplementary Table 1 | Intercorrelations (Pearson correlation r values) between different cognitive domains.
References
1. Roberts AW, Ogunwole SU, Blakeslee L, Rabe MA. The population 65 years and older in the United States: 2016. US: US Department of Commerce, Economics and Statistics Administration (2018).
2. Roman Y. The United States 2020 Census data: implications for precision medicine and the research landscape. Personalized Med. (2022) 19:5–8. doi: 10.2217/pme-2021-0129
3. Chu L, Lor A, Moisan M-G, Phi KM. Gender disparities in healthy aging: A cross-national comparative study in the United States and South Korea from 2006 to 2016. Int J Aging Hum Dev. (2023) 96:33–50. doi: 10.1177/00914150221106643
4. Petersen RC, Roberts RO, Knopman DS, Geda YE, Cha RH, Pankratz VS, et al. Prevalence of mild cognitive impairment is higher in men. The Mayo Clinic Study of Aging. Neurology. (2010) 75:889–97. doi: 10.1212/WNL.0b013e3181f11d85
5. Cao Q, Tan CC, Xu W, Hu H, Cao XP, Dong Q, et al. The prevalence of dementia: A systematic review and meta-analysis. J Alzheimers Dis. (2020) 73:1157–66. doi: 10.3233/JAD-191092
6. Anttila T, Helkala EL, Viitanen M, Kareholt I, Fratiglioni L, Winblad B, et al. Alcohol drinking in middle age and subsequent risk of mild cognitive impairment and dementia in old age: a prospective population based study. BMJ. (2004) 329:539. doi: 10.1136/bmj.38181.418958.BE
7. Xu G, Liu X, Yin Q, Zhu W, Zhang R, Fan X. Alcohol consumption and transition of mild cognitive impairment to dementia. Psychiatry Clin Neurosci. (2009) 63:43–9. doi: 10.1111/j.1440-1819.2008.01904.x
8. Keyes KM, Grant BF, Hasin DS. Evidence for a closing gender gap in alcohol use, abuse, and dependence in the United States population. Drug Alcohol Depend. (2008) 93:21–9. doi: 10.1016/j.drugalcdep.2007.08.017
9. Seedat S, Scott KM, Angermeyer MC, Berglund P, Bromet EJ, Brugha TS, et al. Cross-national associations between gender and mental disorders in the World Health Organization World Mental Health Surveys. Arch Gen Psychiatry. (2009) 66:785–95. doi: 10.1001/archgenpsychiatry.2009.36
10. White A, Castle IJP, Chen CM, Shirley M, Roach D, Hingson R. Converging patterns of alcohol use and related outcomes among females and males in the United States, 2002 to 2012. Alcoholism: Clin Exp Res. (2015) 39:1712–26. doi: 10.1111/acer.12815
11. Agabio R, Pisanu C, Luigi Gessa G, Franconi F. Sex differences in alcohol use disorder. Curr Med Chem. (2017) 24:2661–70. doi: 10.2174/0929867323666161202092908
12. Kang M, Min A, Min H. Gender convergence in alcohol consumption patterns: Findings from the Korea National Health and Nutrition Examination Survey 2007–2016. Int J Environ Res Public Health. (2020) 17:9317. doi: 10.3390/ijerph17249317
13. Randall CL, Roberts JS, Del Boca FK, Carroll KM, Connors GJ, Mattson ME, et al. Telescoping of landmark events associated with drinking: a gender comparison. J Stud Alcohol. (1999) 60:252–60. doi: 10.15288/jsa.1999.60.252
14. Hernandez-Avila CA, Rounsaville BJ, Kranzler HR. Opioid-, cannabis-and alcohol-dependent women show more rapid progression to substance abuse treatment. Drug Alcohol Depend. (2004) 74:265–72. doi: 10.1016/j.drugalcdep.2004.02.001
15. Jimenez Chavez CL, Van Doren E, Matalon J, Ogele N, Kharwa A, Madory L, et al. Alcohol-drinking under limited-access procedures during mature adulthood accelerates the onset of cognitive impairment in mice. Front Behav Neurosci. (2022) 16:732375. doi: 10.3389/fnbeh.2022.732375
16. Stavro K, Pelletier J, Potvin S. Widespread and sustained cognitive deficits in alcoholism: a meta-analysis. Addict Biol. (2013) 18:203–13. doi: 10.1111/j.1369-1600.2011.00418.x
17. Day AM, Kahler CW, Ahern DC, Clark US. Executive functioning in alcohol use studies: A brief review of findings and challenges in assessment. Curr Drug Abuse Rev. (2015) 8:26–40. doi: 10.2174/1874473708666150416110515
18. Le Berre AP, Fama R, Sullivan EV. Executive functions, memory, and social cognitive deficits and recovery in chronic alcoholism: A critical review to inform future research. Alcohol Clin Exp Res. (2017) 41:1432–43. doi: 10.1111/acer.13431
19. Montgomery C, Fisk JE, Murphy PN, Ryland I, Hilton J. The effects of heavy social drinking on executive function: a systematic review and meta-analytic study of existing literature and new empirical findings. Hum Psychopharmacol. (2012) 27:187–99. doi: 10.1002/hup.1268
20. Flannery B, Fishbein D, Krupitsky E, Langevin D, Verbitskaya E, Bland C, et al. Gender differences in neurocognitive functioning among alcohol-dependent Russian patients. Alcohol Clin Exp Res. (2007) 31:745–54. doi: 10.1111/j.1530-0277.2007.00372.x
21. Fama R, Le Berre AP, Sullivan EV. Alcohol's unique effects on cognition in women: A 2020 (Re)view to envision future research and treatment. Alcohol Res. (2020) 40:03. doi: 10.35946/arcr.v40.2.03
22. Panza F, Capurso C, D'Introno A, Colacicco AM, Frisardi V, Lorusso M, et al. Alcohol drinking, cognitive functions in older age, predementia, and dementia syndromes. J Alzheimers Dis. (2009) 17:7–31. doi: 10.3233/JAD-2009-1009
23. Panza F, Frisardi V, Seripa D, Logroscino G, Santamato A, Imbimbo BP, et al. Alcohol consumption in mild cognitive impairment and dementia: harmful or neuroprotective? Int J Geriatr Psychiatry. (2012) 27:1218–38. doi: 10.1002/gps.3772
24. Neafsey EJ, Collins MA. Moderate alcohol consumption and cognitive risk. Neuropsychiatr Dis Treat. (2011) 7:465–84. doi: 10.2147/NDT.S23159
25. Romeo J, Wärnberg J, Marcos A. Drinking pattern and socio-cultural aspects on immune response: an overview. Proc Nutr Soc. (2010) 69:341–6. doi: 10.1017/S0029665110001904
27. Stampfer MJ, Kang JH, Chen J, Cherry R, Grodstein F. Effects of moderate alcohol consumption on cognitive function in women. N Engl J Med. (2005) 352:245–53. doi: 10.1056/NEJMoa041152
28. Stott DJ, Falconer A, Kerr GD, Murray HM, Trompet S, Westendorp RG, et al. Does low to moderate alcohol intake protect against cognitive decline in older people? J Am Geriatr Soc. (2008) 56:2217–24. doi: 10.1111/j.1532-5415.2008.02007.x
29. Galanis DJ, Joseph C, Masaki KH, Petrovitch H, Ross GW, White L. A longitudinal study of drinking and cognitive performance in elderly Japanese American men: the Honolulu-Asia Aging Study. Am J Public Health. (2000) 90:1254–9. doi: 10.2105/AJPH.90.8.1254
30. Elwood PC, Gallacher JE, Hopkinson CA, Pickering J, Rabbitt P, Stollery B, et al. Smoking, drinking, and other life style factors and cognitive function in men in the Caerphilly cohort. J Epidemiol Community Health. (1999) 53:9–14. doi: 10.1136/jech.53.1.9
31. Elias PK, Elias MF, D'Agostino RB, Silbershatz H, Wolf PA. Alcohol consumption and cognitive performance in the Framingham Heart Study. Am J Epidemiol. (1999) 150:580–9. doi: 10.1093/oxfordjournals.aje.a010056
32. Mukamal KJ, Kuller LH, Fitzpatrick AL, Longstreth WT Jr, Mittleman MA, Siscovick DS, et al. Prospective study of alcohol consumption and risk of dementia in older adults. JAMA. (2003) 289:1405–13. doi: 10.1001/jama.289.11.1405
33. Dufouil C, Ducimetiere P, Alperovitch A. Sex differences in the association between alcohol consumption and cognitive performance. EVA Study Group. Epidemiology of Vascular Aging. Am J Epidemiol. (1997) 146:405–12. doi: 10.1093/oxfordjournals.aje.a009293
34. Zuccala G, Onder G, Pedone C, Cesari M, Landi F, Bernabei R, et al. Dose-related impact of alcohol consumption on cognitive function in advanced age: results of a multicenter survey. Alcohol Clin Exp Res. (2001) 25:1743–8. doi: 10.1111/j.1530-0277.2001.tb02185.x
35. Hendrie HC, Gao S, Hall KS, Hui SL, Unverzagt FW. The relationship between alcohol consumption, cognitive performance, and daily functioning in an urban sample of older black Americans. J Am Geriatr Soc. (1996) 44:1158–65. doi: 10.1111/j.1532-5415.1996.tb01364.x
36. Ruitenberg A, van Swieten JC, Witteman JC, Mehta KM, van Duijn CM, Hofman A, et al. Alcohol consumption and risk of dementia: the Rotterdam Study. Lancet. (2002) 359:281–6. doi: 10.1016/S0140-6736(02)07493-7
37. Leroi I, Sheppard JM, Lyketsos CG. Cognitive function after 11.5 years of alcohol use: relation to alcohol use. Am J Epidemiol. (2002) 156:747–52. doi: 10.1093/aje/kwf107
38. Herbert LE, Scherr PA, Beckett LA, Albert MS, Rosner B, Taylor JO, et al. Relation of smoking and low-to-moderate alcohol consumption to change in cognitive function: a longitudinal study in a defined community of older persons. Am J Epidemiol. (1993) 137:881–91. doi: 10.1093/oxfordjournals.aje.a116749
39. Launer LJ, Feskens EJ, Kalmijn S, Kromhout D. Smoking, drinking, and thinking. The Zutphen Elderly Study. Am J Epidemiol. (1996) 143:219–27. doi: 10.1093/oxfordjournals.aje.a008732
40. Zhang R, Shen L, Miles T, Shen Y, Cordero J, Qi Y, et al. Association of low to moderate alcohol drinking with cognitive functions from middle to older age among US adults. JAMA Network Open. (2020) 3:e207922. doi: 10.1001/jamanetworkopen.2020.7922
41. Espeland MA, Gu L, Masaki KH, Langer RD, Coker LH, Stefanick ML, et al. Association between reported alcohol intake and cognition: results from the Women's Health Initiative Memory Study. Am J Epidemiol. (2005) 161:228–38. doi: 10.1093/aje/kwi043
42. Lobo E, Dufouil C, Marcos G, Quetglas B, Saz P, Guallar E, et al. Is there an association between low-to-moderate alcohol consumption and risk of cognitive decline? Am J Epidemiol. (2010) 172:708–16. doi: 10.1093/aje/kwq187
43. Dent OF, Sulway MR, Broe GA, Creasey H, Kos SC, Jorm AF, et al. Alcohol consumption and cognitive performance in a random sample of Australian soldiers who served in the Second World War. BMJ. (1997) 314:1655–7. doi: 10.1136/bmj.314.7095.1655
44. Broe GA, Creasey H, Jorm AF, Bennett HP, Casey B, Waite LM, et al. Health habits and risk of cognitive impairment and dementia in old age: a prospective study on the effects of exercise, smoking and alcohol consumption. Aust N Z J Public Health. (1998) 22:621–3. doi: 10.1111/j.1467-842X.1998.tb01449.x
45. Angebrandt A, Abulseoud OA, Kisner M, Diazgranados N, Momenan R, Yang Y, et al. Dose-dependent relationship between social drinking and brain aging. Neurobiol Aging. (2022) 111:71–81. doi: 10.1016/j.neurobiolaging.2021.11.008
46. Guggenmos M, Schmack K, Sekutowicz M, Garbusow M, Sebold M, Sommer C, et al. Quantitative neurobiological evidence for accelerated brain aging in alcohol dependence. Transl Psychiatry. (2017) 7:1279. doi: 10.1038/s41398-017-0037-y
47. Luo Y, Chen W, Qiu J, Jia T. Accelerated functional brain aging in major depressive disorder: evidence from a large scale fMRI analysis of Chinese participants. Transl Psychiatry. (2022) 12:397. doi: 10.1038/s41398-022-02162-y
48. Jha MK, Fatt CC, Minhajuddin A, Mayes T, Trivedi MH. Accelerated brain aging in adults with major depressive disorder predicts poorer outcome with sertraline: findings from the EMBARC study. Biol Psychiatry Cognit Neurosci Neuroimaging. (2022) 8(4):462–70 doi: 10.1016/j.bpsc.2022.09.006
49. Dunlop K, Victoria LW, Downar J, Gunning FM, Liston C. Accelerated brain aging predicts impulsivity and symptom severity in depression. Neuropsychopharmacology. (2021) 46:911–9. doi: 10.1038/s41386-021-00967-x
50. Ballester PL, Suh JS, Nogovitsyn N, Hassel S, Strother SC, Arnott SR, et al. Accelerated brain aging in major depressive disorder and antidepressant treatment response: A CAN-BIND report. NeuroImage Clin. (2021) 32:102864. doi: 10.1016/j.nicl.2021.102864
51. Demro C, Shen C, Hendrickson TJ, Arend JL, Disner SG, Sponheim SR, et al. Advanced brain-age in psychotic psychopathology: evidence for transdiagnostic neurodevelopmental origins. Front Aging Neurosci. (2022) 14:872867. doi: 10.3389/fnagi.2022.872867
52. McWhinney S, Kolenic M, Franke K, Fialova M, Knytl P, Matejka M, et al. Obesity as a risk factor for accelerated brain ageing in first-episode psychosis-A longitudinal study. Schizophr Bull. (2021) 47:1772–81. doi: 10.1093/schbul/sbab064
53. Liu L, Liu J, Yang L, Wen B, Zhang X, Cheng J, et al. Accelerated brain aging in patients with obsessive-compulsive disorder. Front Psychiatry. (2022) 13:852479. doi: 10.3389/fpsyt.2022.852479
54. Gan S, Shi W, Wang S, Sun Y, Yin B, Bai G, et al. Accelerated brain aging in mild traumatic brain injury: longitudinal pattern recognition with white matter integrity. J Neurotrauma. (2021) 38:2549–59. doi: 10.1089/neu.2020.7551
55. Dennis EL, Taylor BA, Newsome MR, Troyanskaya M, Abildskov TJ, Betts AM, et al. Advanced brain age in deployment-related traumatic brain injury: A LIMBIC-CENC neuroimaging study. Brain Inj. (2022) 36:662–72. doi: 10.1080/02699052.2022.2033844
56. Linli Z, Feng J, Zhao W, Guo S. Associations between smoking and accelerated brain ageing. Prog Neuropsychopharmacol Biol Psychiatry. (2022) 113:110471. doi: 10.1016/j.pnpbp.2021.110471
57. Jha MK, Chin Fatt CR, Minhajuddin A, Mayes TL, Berry JD, Trivedi MH. Accelerated brain aging in individuals with diabetes: Association with poor glycemic control and increased all-cause mortality. Psychoneuroendocrinology. (2022) 145:105921. doi: 10.1016/j.psyneuen.2022.105921
58. Antal B, McMahon LP, Sultan SF, Lithen A, Wexler DJ, Dickerson B, et al. Type 2 diabetes mellitus accelerates brain aging and cognitive decline: Complementary findings from UK Biobank and meta-analyses. Elife. (2022) 11. doi: 10.7554/eLife.73138
59. Huisman SI, van der Boog ATJ, Cialdella F, Verhoeff JJC, David S. Quantifying the post-radiation accelerated brain aging rate in glioma patients with deep learning. Radiother Oncol. (2022) 175:18–25. doi: 10.1016/j.radonc.2022.08.002
60. Ramduny J, Bastiani M, Huedepohl R, Sotiropoulos SN, Chechlacz M. The association between inadequate sleep and accelerated brain ageing. Neurobiol Aging. (2022) 114:1–14. doi: 10.1016/j.neurobiolaging.2022.02.005
61. Christman S, Bermudez C, Hao L, Landman BA, Boyd B, Albert K, et al. Accelerated brain aging predicts impaired cognitive performance and greater disability in geriatric but not midlife adult depression. Transl Psychiatry. (2020) 10:317. doi: 10.1038/s41398-020-01004-z
62. Wierenga LM, Doucet GE, Dima D, Agartz I, Aghajani M, Akudjedu TN, et al. Greater male than female variability in regional brain structure across the lifespan. Hum Brain Mapp. (2022) 43:470–99. doi: 10.1002/hbm.25204
63. Forde NJ, Jeyachandra J, Joseph M, Jacobs GR, Dickie E, Satterthwaite TD, et al. Sex differences in variability of brain structure across the lifespan. Cereb Cortex. (2020) 30:5420–30. doi: 10.1093/cercor/bhaa123
64. Wang Y, Xu Q, Luo J, Hu M, Zuo C. Effects of age and sex on subcortical volumes. Front Aging Neurosci. (2019) 11:259. doi: 10.3389/fnagi.2019.00259
65. Raz N, Gunning-Dixon F, Head D, Rodrigue KM, Williamson A, Acker JD. Aging, sexual dimorphism, and hemispheric asymmetry of the cerebral cortex: replicability of regional differences in volume. Neurobiol Aging. (2004) 25:377–96. doi: 10.1016/S0197-4580(03)00118-0
66. Pruessner JC, Collins DL, Pruessner M, Evans AC. Age and gender predict volume decline in the anterior and posterior hippocampus in early adulthood. J Neurosci. (2001) 21:194–200. doi: 10.1523/JNEUROSCI.21-01-00194.2001
67. Pfefferbaum A, Rosenbloom M, Deshmukh A, Sullivan EV. Sex differences in the effects of alcohol on brain structure. Am J Psychiatry. (2001) 158:188–97. doi: 10.1176/appi.ajp.158.2.188
68. Flores-Bonilla A, Richardson HN. Sex differences in the neurobiology of alcohol use disorder. Alcohol research: Curr Rev. (2020). doi: 10.35946/arcr.v40.2.04
69. Roberts RO, Geda YE, Knopman DS, Cha RH, Pankratz VS, Boeve BF, et al. The Mayo Clinic Study of Aging: design and sampling, participation, baseline measures and sample characteristics. Neuroepidemiology. (2008) 30:58–69. doi: 10.1159/000115751
70. Spitzer RL, Williams JB, Kroenke K, Linzer M, deGruy FV, Hahn SR, et al. Utility of a new procedure for diagnosing mental disorders in primary care: the PRIME-MD 1000 study. Jama. (1994) 272:1749–56. doi: 10.1001/jama.1994.03520220043029
71. Beck AT, Steer RA, Brown G. Beck depression inventory–II. psychol Assess. (1996). doi: 10.1037/t00742-000
72. Beck AT, Epstein N, Brown G, Steer RA. An inventory for measuring clinical anxiety: psychometric properties. J consulting Clin Psychol. (1988) 56:893. doi: 10.1037/0022-006X.56.6.893
73. American Psychiatric Association, A. and A.P. Association. Diagnostic and statistical manual of mental disorders: DSM-IV. Vol. 4. DC: American psychiatric association Washington (1994).
74. Roberts RO, Cerhan JR, Geda YE, Knopman DS, Cha RH, Christianson TJ, et al. Polyunsaturated fatty acids and reduced odds of MCI: the Mayo Clinic Study of Aging. J Alzheimers Dis. (2010) 21:853–65. doi: 10.3233/JAD-2010-091597
75. Block G, Coyle LM, Hartman AM, Scoppa SM. Revision of dietary analysis software for the Health Habits and History Questionnaire. Am J Epidemiol. (1994) 139:1190–6. doi: 10.1093/oxfordjournals.aje.a116965
76. Reitan RM. Validity of the Trail Making Test as an indicator of organic brain damage. Perceptual motor skills. (1958) 8:271–6. doi: 10.2466/pms.1958.8.3.271
77. Wechsler D. Wechsler adult intelligence scale-revised (WAIS-R). Psychological Corporation (1981).
79. Lucas JA, Ivnik RJ, Smith GE, Bohac DL, Tangalos EG, Graff-Radford NR, et al. Mayo's older Americans normative studies: category fluency norms. J Clin Exp Neuropsychol. (1998) 20:194–200. doi: 10.1076/jcen.20.2.194.1173
81. Ivnik R, Malec J, Smith G. WAIS-R, WMS-R and AVLT norms for ages 56 through 97. Clin Neuropsychol. (1992) 6:1–104. doi: 10.1080/13854049208401877
82. Roberts RO, Geda YE, Knopman DS, Cha RH, Pankratz VS, Boeve BF, et al. The incidence of MCI differs by subtype and is higher in men: the Mayo Clinic Study of Aging. Neurology. (2012) 78:342–51. doi: 10.1212/WNL.0b013e3182452862
83. Charlson ME, Pompei P, Ales KL, MacKenzie CR. A new method of classifying prognostic comorbidity in longitudinal studies: development and validation. J chronic Dis. (1987) 40:373–83. doi: 10.1016/0021-9681(87)90171-8
84. Jack CR Jr, Bernstein MA, Borowski BJ, Gunter JL, Fox NC, Thompson PM, et al. Update on the magnetic resonance imaging core of the Alzheimer's disease neuroimaging initiative. Alzheimer's Dementia. (2010) 6:212–20. doi: 10.1016/j.jalz.2010.03.004
85. Jack CR Jr, Lowe VJ, Senjem ML, Weigand SD, Kemp BJ, Shiung MM, et al. 11C PiB and structural MRI provide complementary information in imaging of Alzheimer's disease and amnestic mild cognitive impairment. Brain. (2008) 131:665–80. doi: 10.1093/brain/awm336
86. Flemming KD, Graff-Radford J, Aakre J, Kantarci K, Lanzino G, Brown RD, et al. Population-based prevalence of cerebral cavernous malformations in older adults: Mayo Clinic Study of Aging. JAMA Neurol. (2017) 74:801–5. doi: 10.1001/jamaneurol.2017.0439
87. Gunter JL, Bernstein MA, Borowski BJ, Ward CP, Britson PJ, Felmlee JP, et al. Measurement of MRI scanner performance with the ADNI phantom. Med Phys. (2009) 36:2193–205. doi: 10.1118/1.3116776
89. Liem F, Varoquaux G, Kynast J, Beyer F, Kharabian Masouleh S, Huntenburg JM, et al. Predicting brain-age from multimodal imaging data captures cognitive impairment. Neuroimage. (2017) 148:179–88. doi: 10.1016/j.neuroimage.2016.11.005
90. R Core Team. R: A language and environment for statistical computing. Vienna, Austria: R Foundation for Statistical Computing (2019). Available at: https://www.R-project.org/.
91. Ferreira L, Ferreira Santos-Galduroz R, Ferri CP, Fernandes Galduroz JC. Rate of cognitive decline in relation to sex after 60 years-of-age: a systematic review. Geriatr Gerontol Int. (2014) 14:23–31. doi: 10.1111/ggi.12093
92. Gerstorf D, Herlitz A, Smith J. Stability of sex differences in cognition in advanced old age: the role of education and attrition. J Gerontol B Psychol Sci Soc Sci. (2006) 61:245–9. doi: 10.1093/geronb/61.4.P245
93. Finkel D, Reynolds CA, Berg S, Pedersen NL. Surprising lack of sex differences in normal cognitive aging in twins. Int J Aging Hum Dev. (2006) 62:335–57. doi: 10.2190/C39X-9QHY-49DM-X9GJ
94. de Frias CM, Nilsson LG, Herlitz A. Sex differences in cognition are stable over a 10-year period in adulthood and old age. Neuropsychol Dev Cognit B Aging Neuropsychol Cognit. (2006) 13:574–87. doi: 10.1080/13825580600678418
95. Resnick SM. Sex differences in mental rotations: an effect of time limits? Brain Cognit. (1993) 21:71–9. doi: 10.1006/brcg.1993.1005
96. Agabio R, Sinclair JMA. 'Mother's ruin'-why sex and gender differences in the field of alcohol research need consideration. Alcohol Alcohol. (2019) 54:342–4. doi: 10.1093/alcalc/agz053
97. Murphy DG, DeCarli C, McIntosh AR, Daly E, Mentis MJ, Pietrini P, et al. Sex differences in human brain morphometry and metabolism: an in vivo quantitative magnetic resonance imaging and positron emission tomography study on the effect of aging. Arch Gen Psychiatry. (1996) 53:585–94. doi: 10.1001/archpsyc.1996.01830070031007
98. Allen JS, Bruss J, Damasio H. The aging brain: the cognitive reserve hypothesis and hominid evolution. Am J Hum Biol. (2005) 17:673–89. doi: 10.1002/ajhb.20439
99. Harrison SL, Sajjad A, Bramer WM, Ikram MA, Tiemeier H, Stephan BC. Exploring strategies to operationalize cognitive reserve: A systematic review of reviews. J Clin Exp Neuropsychol. (2015) 37:253–64. doi: 10.1080/13803395.2014.1002759
100. Pettigrew C, Soldan A. Defining cognitive reserve and implications for cognitive aging. Curr Neurol Neurosci Rep. (2019) 19:1. doi: 10.1007/s11910-019-0917-z
101. Kaur A, Sonal A, Ghosh T, Ahamed F. Cognitive reserve and other determinants of cognitive function in older adults: Insights from a community-based cross-sectional study. J Family Med Prim Care. (2023) 12:1957–64. doi: 10.4103/jfmpc.jfmpc_2458_22
102. Meng X, D'Arcy C. Education and dementia in the context of the cognitive reserve hypothesis: a systematic review with meta-analyses and qualitative analyses. PloS One. (2012) 7:e38268. doi: 10.1371/journal.pone.0038268
103. Contador I, Alzola P, Stern Y, de la Torre-Luque A, Bermejo-Pareja F, Fernandez-Calvo B. Is cognitive reserve associated with the prevention of cognitive decline after stroke? A Systematic review and meta-analysis. Ageing Res Rev. (2023) 84:101814. doi: 10.1016/j.arr.2022.101814
104. Edelstein SL, Kritz-Silverstein D, Barrett-Connor E. Prospective association of smoking and alcohol use with cognitive function in an elderly cohort. J Womens Health. (1998) 7:1271–81. doi: 10.1089/jwh.1998.7.1271
105. Collaborators GBDA. Alcohol use and burden for 195 countries and territories, 1990-2016: a systematic analysis for the Global Burden of Disease Study 2016. Lancet. (2018) 392:1015–35. doi: 10.1016/S0140-6736(18)31310-2
106. Bostrand SMK, Vaher K, de Nooij L, Harris MA, Cole JH, Cox SR, et al. Associations between alcohol use and accelerated biological ageing. Addict Biol. (2022) 27:e13100. doi: 10.1111/adb.13100
107. Leon BE, Kang S, Franca-Solomon G, Shang P, Choi DS. Alcohol-induced neuroinflammatory response and mitochondrial dysfunction on aging and alzheimer's disease. Front Behav Neurosci. (2021) 15:778456. doi: 10.3389/fnbeh.2021.778456
108. Jung J, McCartney DL, Wagner J, Rosoff DB, Schwandt M, Sun H, et al. Alcohol use disorder is associated with DNA methylation-based shortening of telomere length and regulated by TESPA1: implications for aging. Mol Psychiatry. (2022). doi: 10.1038/s41380-022-01624-5
109. Bennett IJ, Madden DJ. Disconnected aging: cerebral white matter integrity and age-related differences in cognition. Neuroscience. (2014) 276:187–205. doi: 10.1016/j.neuroscience.2013.11.026
110. Cosgrove KP, Mazure CM, Staley JK. Evolving knowledge of sex differences in brain structure, function, and chemistry. Biol Psychiatry. (2007) 62:847–55. doi: 10.1016/j.biopsych.2007.03.001
111. Tranel D. Theories of clinical neuropsychology and brain-behavior relationships: Luria and beyond. Textbook Clin Neuropsychol. (2007) 1027:25–37.
112. Beheshti I, Ganaie M, Paliwal V, Rastogi A, Razzak I, Tanveer M. Predicting brain age using machine learning algorithms: A comprehensive evaluation. IEEE J Biomed Health Inf. (2021) 26:1432–40. doi: 10.1109/JBHI.2021.3083187
113. Baldo JV, Arévalo A, Patterson JP, Dronkers NF. Grey and white matter correlates of picture naming: evidence from a voxel-based lesion analysis of the Boston Naming Test. Cortex. (2013) 49:658–67. doi: 10.1016/j.cortex.2012.03.001
114. Kansaku K, Yamaura A, Kitazawa S. Sex differences in lateralization revealed in the posterior language areas. Cereb Cortex. (2000) 10:866–72. doi: 10.1093/cercor/10.9.866
115. Chen X, Sachdev PS, Wen W, Anstey KJ. Sex differences in regional gray matter in healthy individuals aged 44-48 years: a voxel-based morphometric study. Neuroimage. (2007) 36:691–9. doi: 10.1016/j.neuroimage.2007.03.063
116. Cardenas VA, Studholme C, Gazdzinski S, Durazzo TC, Meyerhoff DJ. Deformation-based morphometry of brain changes in alcohol dependence and abstinence. Neuroimage. (2007) 34:879–87. doi: 10.1016/j.neuroimage.2006.10.015
117. Paus T. Sex differences in the human brain: a developmental perspective. Prog Brain Res. (2010) 186:13–28. doi: 10.1016/B978-0-444-53630-3.00002-6
118. Krell-Roesch J, Syrjanen JA, Bezold J, Trautwein S, Barisch-Fritz B, Kremers WK, et al. Mid-and late-life physical activity and neuropsychiatric symptoms in dementia-free older adults: mayo clinic study of aging. J neuropsychiatry Clin Neurosci. (2023) 35:133–40. doi: 10.1176/appi.neuropsych.20220068
119. Wang S, Luo X, Barnes D, Sano M, Yaffe K. Physical activity and risk of cognitive impairment among oldest-old women. Am J Geriatric Psychiatry. (2014) 22:1149–57. doi: 10.1016/j.jagp.2013.03.002
120. Krell-Roesch J, Syrjanen JA, Bezold J, Trautwein S, Barisch-Fritz B, Boes K. Physical activity and trajectory of cognitive change in older persons: Mayo Clinic Study of Aging. J Alzheimer's Dis. (2021) 79:377–88. doi: 10.3233/JAD-200959
Keywords: social drinking, brain age estimating, cognitive function, sex difference, alcohol
Citation: Abulseoud OA, Caparelli EC, Krell‐Roesch J, Geda YE, Ross TJ and Yang Y (2024) Sex-difference in the association between social drinking, structural brain aging and cognitive function in older individuals free of cognitive impairment. Front. Psychiatry 15:1235171. doi: 10.3389/fpsyt.2024.1235171
Received: 05 June 2023; Accepted: 19 March 2024;
Published: 08 April 2024.
Edited by:
Susana Muñoz Maniega, University of Edinburgh, United KingdomReviewed by:
Emmanuelle Baillet, Université de Bordeaux, FranceAlejandra Mondragón-Maya, National Autonomous University of Mexico, Mexico
Copyright © 2024 Abulseoud, Caparelli, Krell‐Roesch, Geda, Ross and Yang. This is an open-access article distributed under the terms of the Creative Commons Attribution License (CC BY). The use, distribution or reproduction in other forums is permitted, provided the original author(s) and the copyright owner(s) are credited and that the original publication in this journal is cited, in accordance with accepted academic practice. No use, distribution or reproduction is permitted which does not comply with these terms.
*Correspondence: Osama A. Abulseoud, Abulseoud.osama@mayo.edu