- 1Tsinghua University School of Medicine, Beijing, China
- 2Shanghai Mental Health Center, Shanghai Jiaotong University School of Medicine, Shanghai, China
- 3Department of Psychiatry, Beijing Yuquan Hospital, Tsinghua University, Beijing, China
Background: While the molecular underpinnings of vascular dysfunction in psychosis are under active investigation, their implications remain unclear due to inconsistent and sometimes sparse observations. We conducted a comprehensive meta-analysis to critically assess the alterations of vascular-related molecules in the cerebrospinal fluid (CSF) and blood of patients with psychotic disorders compared with healthy individuals.
Methods: Databases were searched from inception to February 23, 2023. Meta-analyses were performed using a random-effects model. Meta-regression and subgroup analyses were conducted to assess the effects of clinical correlates.
Results: We identified 93 eligible studies with 30 biomarkers investigated in the CSF and/or blood. Among the biomarkers examined, psychotic disorders were associated with elevated CSF-to-serum albumin ratio (standardized mean difference [SMD], 0.69; 95% confidence interval [CI], 0.35–1.02); blood S100B (SMD, 0.88; 95% CI, 0.59–1.17), matrix metalloproteinase-9 (MMP-9; SMD, 0.66; 95% CI, 0.46–0.86), and zonulin (SMD, 1.17; 95% CI, 0.04–2.30). The blood levels of S100B, MMP-9, nerve growth factor (NGF), vascular endothelial growth factor (VEGF), intercellular adhesion molecule 1 (ICAM-1), and vascular adhesion molecule 1 (VCAM-1) were altered in patient subgroups differing in demographic and clinical characteristics. Blood S100B level was positively correlated with age and duration of illness. Substantial between-study heterogeneity was observed in most molecules.
Conclusion: The alterations in certain vascular-related fluid markers in psychotic disorders suggest disturbances in normal vascular structures and functions. However, not all molecules examined displayed clear evidence of changes. While potential impacts of clinical factors, including the administered treatment, were identified, the exploration remained limited. Further studies are needed to investigate the diverse patterns of expression, and understand how these abnormalities reflect the pathophysiology of psychosis and the impact of clinical factors.
1. Introduction
Growing evidence has revealed the presence of both peripheral and central vascular dysfunction in psychotic disorders. Meta-analyses have shown that patients with schizophrenia carry a greater risk of cardiovascular diseases even in their first episodes prior to antipsychotic exposure (1–3), with this risk being even higher in chronic schizophrenia (4). Cardiovascular risk factors are further linked to cognitive impairment (5) and contribute to mortality (6, 7). In the central nervous system (CNS), postmortem studies have revealed direct evidence of destruction of the blood–brain barrier (BBB) and neurovascular unit (NVU) (8, 9). Functionally, positron emission tomography (PET) studies have indicated increased activity of the efflux transporter P-glycoprotein in multiple brain regions, suggesting altered BBB permeability (10). Moreover, the NVU plays a key role in neurovascular coupling (NVC), in which cerebral blood flow is regulated in response to changes in energy utilization and neural activity. NVC deficits in the frontotemporal regions have been consistently observed in fMRI studies in patients with first-episode psychosis (FEP) (11). The strength of coupling is closely modulated by the density, morphology, and capacity of dilation of the vessels (12). Notably, patients with schizophrenia exhibit thinner neurites but similar capillary diameters compared to healthy controls, implying a neurite-vasculature mismatch (13, 14). Furthermore, reduced grey matter blood flow across multiple brain areas has been observed in patients with schizophrenia (15). Correlation exists between the abnormal cerebral blood flow and symptom severity (16), both of which improved with aripiprazole treatment (17).
Apart from neuropathology and neuroimaging studies, much effort has been devoted to investigating the relevant fluid biomarkers in psychotic disorders. The goal has been to obtain easily accessible information regarding the mechanisms, diagnosis, and prognosis of these disorders (9, 18). However, conflicting observations exist, and the implications of vascular-related fluid biomarkers remain a subject of debate. To date, most meta-analyses in this field focused only on specific molecules. In addition, many earlier meta-analyses included non-peer-reviewed studies or those involving participants with comorbid conditions known to affect vascular functions, such as cardiovascular diseases and cannabis use, which can introduce bias and limit the generalizability of the findings.
Here, we aim to provide a comprehensive meta-analysis to further evaluate the alterations in vascular-related fluid molecules in psychotic disorders, along with their relations with demographic and clinical characteristics.
2. Methods and materials
This study adhered to the Preferred Reporting Items for Systematic Reviews and Meta-analyses (PRISMA) reporting guidelines (Supplementary Table S1) (19). The study protocol was registered in PROSPERO (CRD42023398731). Two authors (XL and SH) independently performed the literature search, study inclusion, and bias assessment. One author (XL) extracted the data, which was then examined by another author (SH). When discrepancies occurred, consensus was reached either through discussion between the two authors or by consulting the third author (PL).
2.1. Search strategy
We searched Embase, MEDLINE, PsycINFO, and Web of Science from database inception to February 23, 2023 as well as the reference lists of the retrieved studies. The search term is presented in Supplementary Table S2. Deduplication was performed using the Bramer method (20).
2.2. Selection criteria
Studies were included if they fulfilled the following criteria: (1) peer-reviewed and published in English; (2) quantified vascular-related biomarkers in the CSF or blood from living patients and healthy controls; (3) patients were diagnosed with schizophrenia, schizoaffective disorder, schizophreniform disorder, delusional disorder, or FEP according to a recognized diagnostic system (e.g., Diagnostic and Statistical Manual of Mental Disorders [DSM], International Classification of Diseases [ICD], or Research Diagnostic Criteria [RDC]).
Studies were excluded if they (1) are reviews, letters to the editor, correspondence, or published in conferences; (2) contained participants with other psychiatric disorders, substance use, neurological disorders, cardiovascular diseases, or inflammatory diseases; (3) contained controls with personal or family history of psychiatric disorders.
For studies containing overlapping participants, we included only studies that first reported the most comprehensive information.
2.3. Data extraction
Data extracted include the number, age, gender, body mass index (BMI), and smoking status of all participants; diagnosis, diagnostic tool, age at onset, duration of illness, treatment status (i.e., drug-naïve, unmedicated, medicated, and post-ECT) and duration, the types and doses of medications and symptom severity of the patients; concentrations of the biomarkers, sample origins, and measurement methods of the biomarkers. For longitudinal studies involving different treatment statuses, both baseline and follow-up data were extracted. In cases of missing data, data reported as summary estimates other than arithmetic means and standard deviations (SDs), or uncertainty, authors of the studies were contacted to provide information.
When the requested information was not received, we extracted the data using WebPlotDigitizer, version 4.6 (Ankit Rohatgi, CA, USA) if it was reported in graphs in the articles. Medians, ranges, and interquartile ranges were converted to means and SDs using validated methods (21, 22). When the concentrations of a biomarker were reported in arithmetic and geometric scales in different studies, statistics in the scale less frequently reported in the included studies were converted to the other scale using the Higgins approach (23).
2.4. Bias assessment
Bias assessment was performed using an adapted version of the Newcastle-Ottawa Scale (NOS; Supplementary Table S3) (24). Assessment of the item “non-response rate” was not applicable, thus the maximum total score on NOS was 8. Small-study effects were assessed with contour-enhanced funnel plots (25) and the Egger test (26) for biomarkers measured in 10 or more studies.
2.5. Statistical analyses
Our primary outcome was the differences in biomarker concentration between patients and healthy controls. Secondary outcomes were the associations between biomarkers and demographic and clinical characteristics. Meta-analyses were performed using Stata/SE, version 17.0 (StataCorp LLC).
Heterogeneity between studies was assessed using the Cochran’s Q test (27). Level of significance was set at p < 0.10. Degree of heterogeneity was quantified using the I2 metric (28), with metrics of 25, 50, and 75% considered as indicating low, moderate, and high heterogeneity, respectively (29). Our analyses showed high heterogeneity among the majority of studies, thus random-effects model (30) was used to calculate the standardized mean differences (SMDs) as the effect size. SMDs were bias-corrected using Hedges’ g (28). We consider an effect size of 0.2, 0.5, and 0.8 as indicating a low, moderate, and large effect, respectively. Level of significance was set at p < 0.05. In cases of longitudinal studies where biomarkers were measured under different treatment statuses, we used the measurement under the status that most patients tested for the biomarker were under.
Outlier studies were detected using the Galbraith plot (31). Sensitivity analyses were performed by removing the outliers and recomputing the overall significance.
For biomarkers quantified in 5 or more studies, we conducted subgroup analyses to assess the effect of illness stages (i.e., FEP and non-FEP), treatment status (i.e., drug-naïve, unmedicated, medicated, and post-ECT), and sample origins (i.e., plasma and serum). For biomarkers quantified in 10 or more studies, meta-regression was conducted to investigate the effects of demographic and clinical characteristics if 10 or more data points were available. For biomarkers quantified in only one study, findings of the studies were qualitatively summarized.
3. Results
The literature search identified 11,194 studies after deduplication, of which 93 were included in the final synthesis (Figure 1; Supplementary Tables S4, S5). Thirty biomarkers in the CSF and/or blood were identified, of which 17 were quantified in two or more studies (Table 1; Figures 2–5). Findings of the remaining 13 biomarkers quantified in only one study are summarized in Supplementary Table S6.
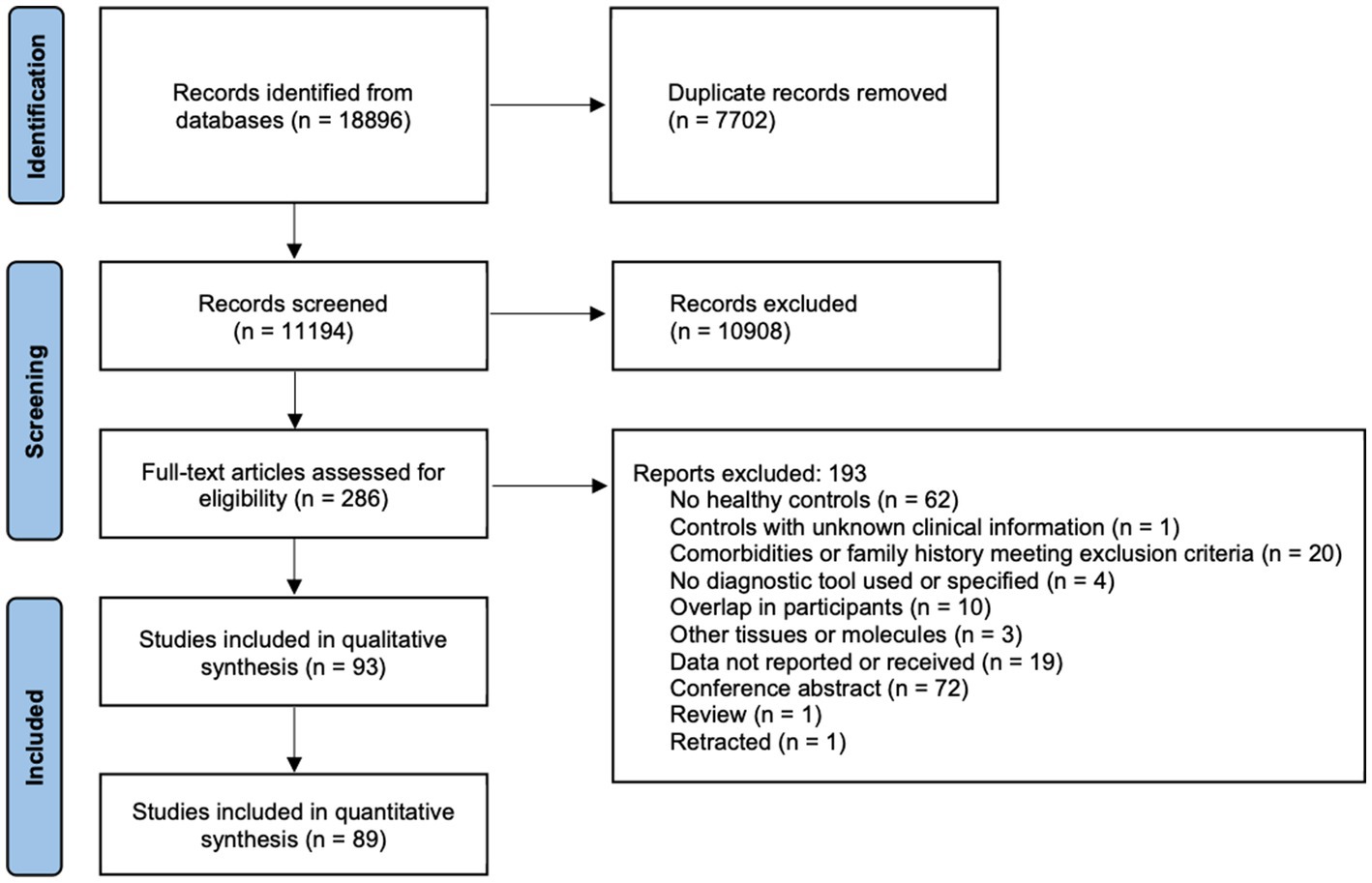
Figure 1. PRISMA 2020 flow diagram. The literature search, and the screening and inclusion of eligible studies following the PRISMA reporting guidelines are shown.
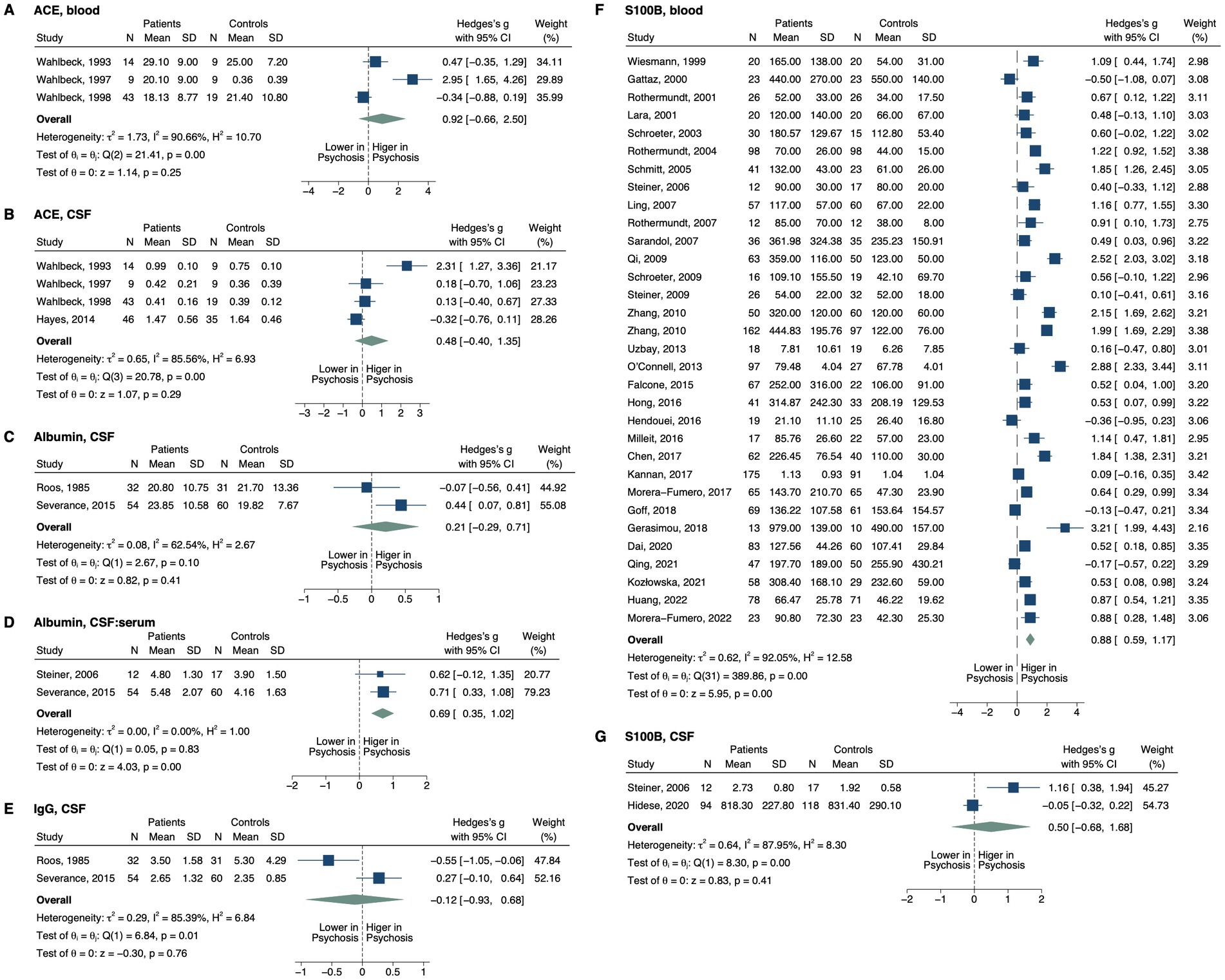
Figure 2. Forest plots of the markers of BBB permeability measured in ≥2 studies. Forest plots of (A) blood ACE, (B) CSF ACE, (C) CSF albumin, (D) CSF:serum albumin, (E) CSF IgG, (F) blood S100B, and (G) CSF S100B. The sizes of the squares are proportional to the weights calculated from random-effects analysis. Horizontal lines represent 95% CIs. The diamond denotes the overall SMD. The vertical dashed line represents the line of no difference between patients and controls.
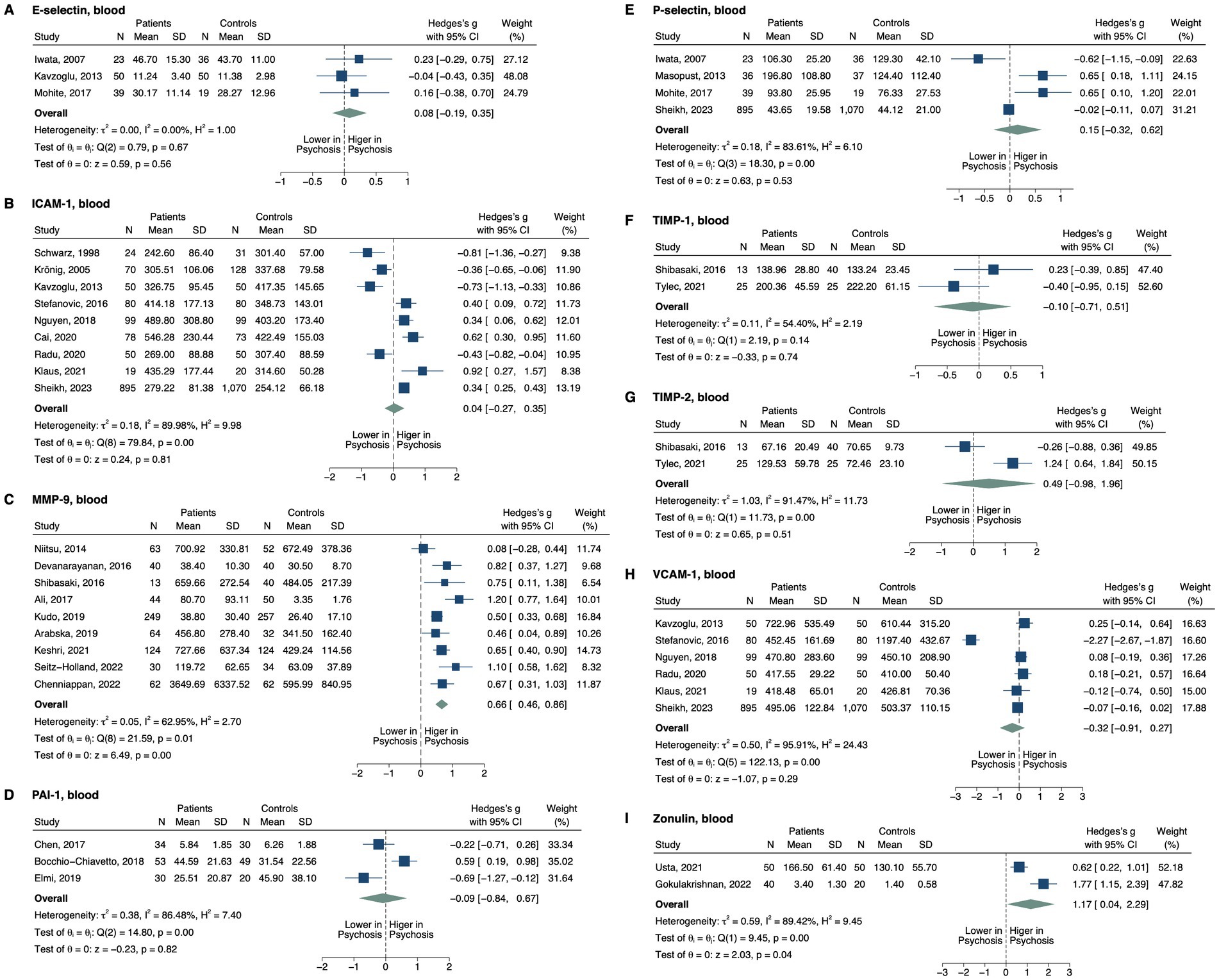
Figure 3. Forest plots of tight junction proteins and modifiers measured in ≥2 studies. Forest plots of blood (A) E-selectin, (B) ICAM-1, (C) MMP-9, (D) PAI-1, (E) P-selectin, (F) TIMP-1, (G) TIMP-2, (H) VCAM-1, and (I) zonulin. The sizes of the squares are proportional to the weights calculated from random-effects analysis. Horizontal lines represent 95% CIs. The diamond denotes the overall SMD. The vertical dashed line represents the line of no difference between patients and controls.
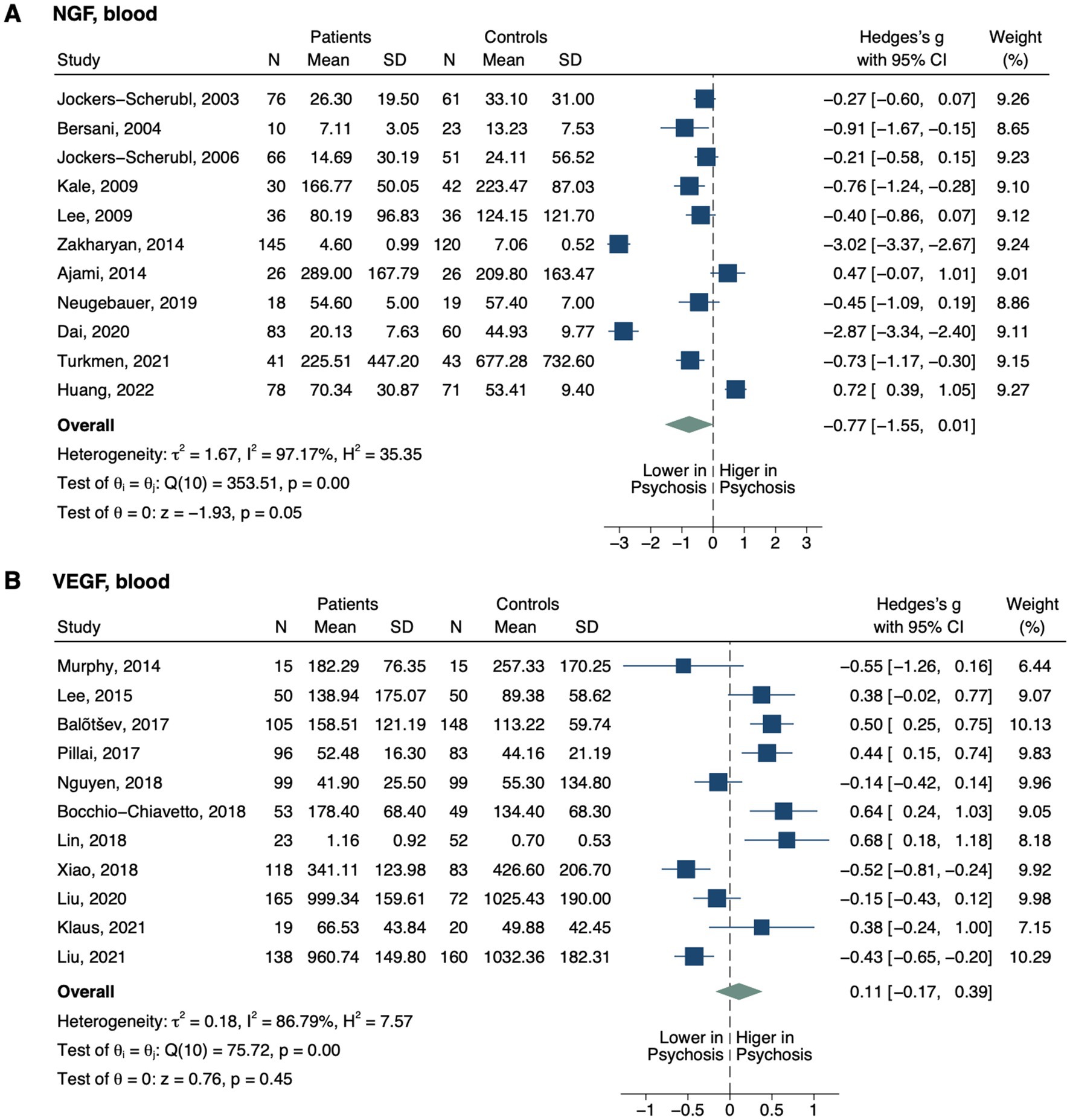
Figure 4. Forest plots of angiogenic neurotrophins measured in ≥2 studies. Forest plots of blood (A) NGF and (B) VEGF. The sizes of the squares are proportional to the weights calculated from random-effects analysis. Horizontal lines represent 95% CIs. The diamond denotes the overall SMD. The vertical dashed line represents the line of no difference between patients and controls.
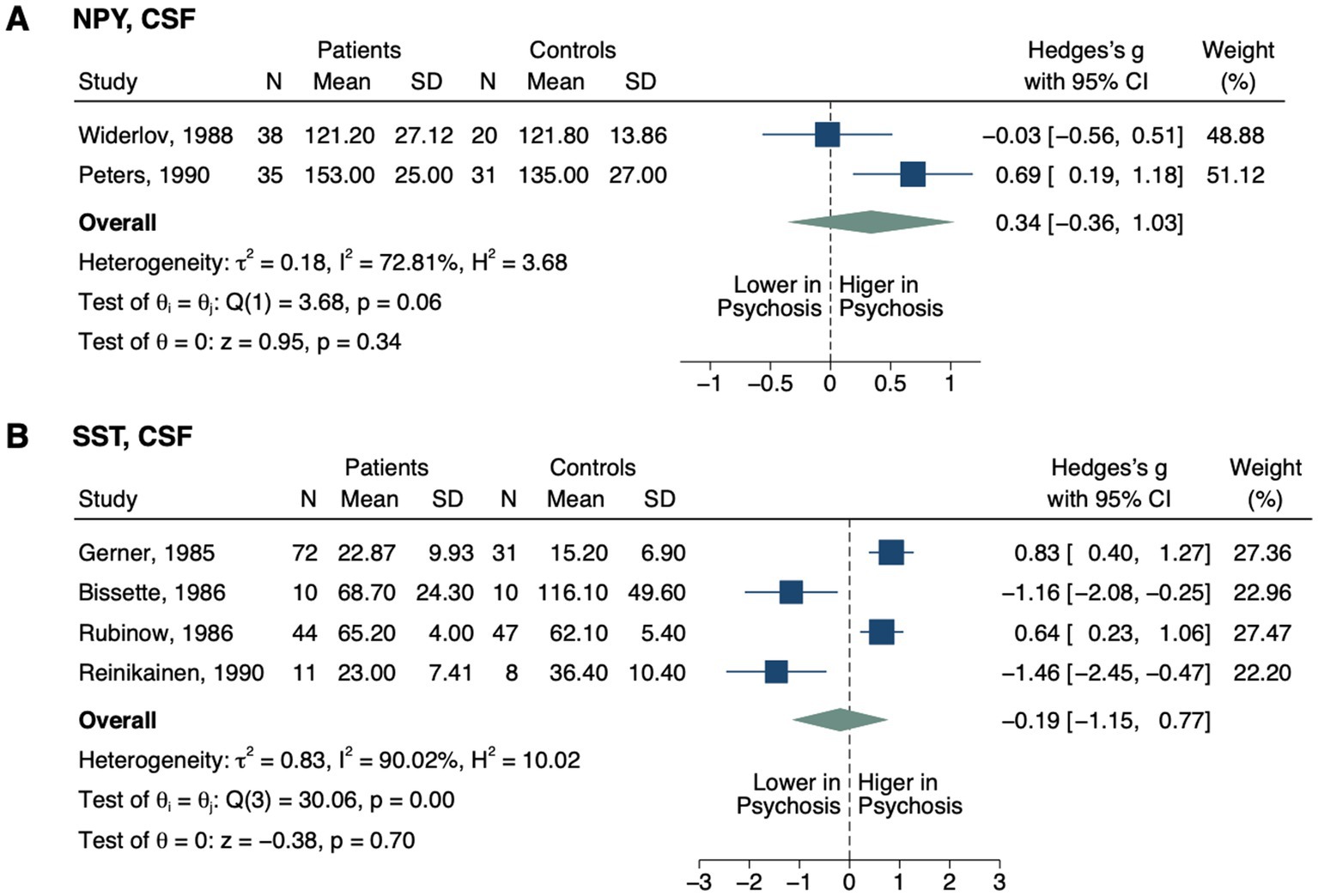
Figure 5. Forest plots of neuropeptides involved in neurovascular coupling measured in ≥2 studies. Forest plots of CSF (A) NPY and (B) SST. The sizes of the squares are proportional to the weights calculated from random-effects analysis. Horizontal lines represent 95% CIs. The diamond denotes the overall SMD. The vertical dashed line represents the line of no difference between patients and controls.
3.1. Bias assessment
The average NOS score of the studies was 4.90 ± 1.68 (mean ± SD), with 17 (18.09%) studies scoring less than 4, 76 (80.85%) scoring at least 4, and 5 (5.32%) scoring 8 (Supplementary Table S7). The majority of studies had risks of bias in case definition (54.26%) and ascertainment of exposure (79.79%). Thirty-two (34.04%) studies did not state to have matched or adjusted for any confounding factors. Others had risks of bias in case representativeness (24.47%), control selection (44.68%), and control definition (24.47%).
Contour-enhanced funnel plots of NGF, S100B, and VEGF blood levels are shown in Supplementary Figure S1. No small-study effects were detected for these 3 biomarkers (Egger test: NGF, p = 0.958; S100B, p = 0.167; VEGF, p = 0.653; Supplementary Table S8).
3.2. Blood–brain barrier
For markers evaluating BBB permeability (Figure 2), CSF-to-serum albumin ratio was significantly elevated in patients compared to controls (SMD, 0.69; 95% CI, 0.35–1.02; p < 0.001) (32, 33), whereas CSF albumin did not display a significant difference (SMD, 0.21; 95% CI, −0.29–0.71; p = 0.413) (33, 34). Blood S100B was also elevated in psychosis, with a large effect size (SMD, 0.88; 95% CI, 0.59–1.17; p < 0.001) (32, 35–65), which remained significant after excluding 3 outlier studies (Supplementary Figure S2; SMD, 0.70; 95% CI, 0.44–0.96; p < 0.001) (46, 55, 56). In contrast, CSF S100B did not differ between groups (SMD, 0.50; 95% CI, −0.68–1.68; p = 0.408) (32, 66). The increase in blood S100B was detected in patients across 3 treatment statuses—drug-naïve, unmedicated and medicated (Table 2). This pattern was consistently observed in serum (SMD, 1.06; 95% CI, 0.72–1.40; p < 0.001) and patients with non-FEP (SMD, 0.93; 95% CI, 0.61–1.24; p < 0.001), rather than in plasma (SMD, 0.35; 95% CI, −0.09–0.78; p = 0.118) or patients with FEP (SMD, 0.53; 95% CI, −0.09–1.16; p = 0.096). No significant differences were found for CSF or blood angiotensin-converting enzyme (ACE) (67–70), or CSF immunoglobulin G (IgG) levels (33, 34).
For biomarkers measured in single studies, CSF-to-serum IgG ratio was elevated in the patients (drug-naïve, p ≤ 0.002; medicated, p ≤ 0.001) (33), while CSF fibrinogen was decreased (p = 0.021) (67). CSF IgG index did not differ between groups (33).
3.3. Junction proteins and modifiers
Patients with psychosis were found to have higher blood matrix metalloproteinase-9 (MMP-9; SMD, 0.66; 95% CI, 0.46–0.86; p < 0.001; Figure 3) (71–79). In subgroup analysis, this pattern remained significant in the plasma (SMD, 0.75; 95% CI, 0.18–1.32; p = 0.010) and serum (SMD, 0.65; 95% CI, 0.40–0.90; p < 0.001), as well as in patients medicated (SMD, 0.59; 95% CI, 0.16–1.02; p = 0.007) and unmedicated (SMD, 1.21; 95% CI, 0.77–1.64; p < 0.001). Note that there was only one study with unmedicated patients and two studies with plasma levels in the subgroup analysis. In the one study involving patients post-electroconvulsive therapy (ECT) (79), blood MMP-9 did not differ between groups (SMD, 0.09; 95% CI, −0.53–0.71; p = 0.777). Blood zonulin was also elevated in the patients (SMD, 1.17; 95% CI, 0.04–2.30; p = 0.043) (80, 81). We found no significant differences between all patients and controls for blood E- (82–84) or P-selectins (82, 84–86), intercellular adhesion molecule 1 (ICAM-1) 83, 86–93), vascular cell adhesion molecule 1 (VCAM-1) (83, 86, 88, 90, 91, 93), tissue inhibitor of matrix metalloproteinase1 (TIMP-1) (79, 94), TIMP-2 (79, 94), or plasminogen activator inhibitor-1 (PAI-1) (95–97). However, blood ICAM-1 was lower in drug-naïve patients (SMD, −0.81; 95% CI, −1.10–-0.53; p < 0.001) and patients with FEP (SMD, −0.58; 95% CI, −0.87–-0.28; p < 0.001), but elevated in patients with non-FEP (SMD, 0.33; 95% CI, 0.07–0.59; p = 0.012). Blood VCAM-1 was significantly reduced in unmedicated patients in one study that was detected as an outlier (Supplementary Figure S2; SMD, −2.27; 95% CI, −2.67–-1.87; p < 0.001) (93). Removing this study from the overall analysis still displayed no significant difference in VCAM-1 level between groups (SMD, 0.00; 95% CI, −0.12–0.12; p = 0.993), but the heterogeneity was no longer significant.
For biomarkers measured in only one study, CSF MMP-2 (p = 0.031) (98) and plasma junctional adhesion molecule A (JAM-A; p < 0.001) (86) were reported to be higher in the patients. On the other hand, serum claudin 5 (p = 0.037) (81) and MMP-7 (p < 0.001) (94) displayed lower levels in patients. No between-group differences were reported for serum VE-cadherin (99), claudin (100), occludin (100), MMP-1 (94), MMP-2 (98), MMP-13 (94); plasma N-cadherin (86); or CSF MMP-3 (67).
3.4. Angiogenesis
Blood nerve growth factor (NGF) level did not differ between groups (SMD, −0.77; 95% CI, −1.55–0.01; p = 0.054; Figure 4) (44, 48, 101–109). In subgroup analysis, only drug-naïve patients displayed decreased NGF (SMD, −2.16; 95% CI, −4.02–-0.31; p = 0.022; Table 2), while no significant differences were detected based on other treatment statuses (i.e., unmedicated or medicated), illness stages (i.e., FEP and non-FEP) or sample origins (i.e., plasma and serum). Blood vascular endothelial growth factor (VEGF) level also did not differ between all patients and controls (SMD, 0.11; 95% CI, −0.17–0.39; p = 0.447) (88, 90, 95, 110–117). It was decreased in one study with drug-naïve patients (SMD, −0.77; 95% CI, −1.23–-0.31; p = 0.001) (111), but not with other treatment statuses, illness stages, or sample origins.
Decreased NGF in CSF (p = 0.038) in patients with psychosis was reported in one study (105).
3.5. Neurovascular coupling
No significant differences were detected in CSF neuropeptide Y (NPY; SMD, 0.34; 95% CI, −0.36–1.03; p = 0.341; Figure 5) (118, 119) or somatostatin (SST; SMD, −0.19; 95% CI, −1.15–0.77; p = 0.700) (120–123) between groups.
However, elevated CSF prostaglandin E (PGE; p < 0.001) was reported in one study in the patient group (124).
3.6. Heterogeneity
The majority of biomarkers displayed significant heterogeneity between studies, except for CSF albumin, CSF-to-serum albumin ratio, and blood E-selectin and TIMP-1 (Table 1). Among the biomarkers with significant heterogeneity, blood MMP-9, and CSF NPY displayed moderate heterogeneity, while the others all presented high heterogeneity. The possible sources of heterogeneity were investigated in sensitivity, subgroup and meta-regression analyses.
3.7. Meta-regression
Conduction of meta-regression analyses was only possible for blood S100B, NGF, and VEGF (Table 3). Analyses revealed positive associations between the effect sizes of blood S100B and age and duration of illness (Supplementary Figure S3). There was no significant correlation between the effect sizes of blood S100B and the percentage of males, the Positive and Negative Syndrome Scale (PANSS) scores, or the doses of medications (Supplementary Table S9). Assessment of the relevance of treatment duration, BMI and smoking status was not possible due to insufficient data, but BMI was positively correlated with the percentage of males (r = 0.700, p = 0.043) and age (r = 0.717, p = 0.037). No correlations were found between the effect sizes of blood NGF and age, or between the effect sizes of blood VEGF and the percentage of males or age.
4. Discussion
Our study provides the most comprehensive meta-analysis to date investigating the vascular-related biomarkers in psychosis. To our knowledge, this is the first meta-analysis to explore the alterations in blood ACE, TIMP-2, zonulin and PAI-1, as well as CSF ACE, S100B, NPY and SST in individuals with psychotic disorders compared to healthy controls. Overall, we revealed that psychotic disorders are associated with increased CSF-to-serum albumin ratio, and increased blood S100B, MMP-9, and zonulin. While these findings in part support the role of vascular pathology in psychosis, they also raise important questions regarding the implications of various relevant fluid molecules.
A main finding of this study is the alteration of BBB-relevant molecules in psychosis. It has been hypothesized that leakiness of the BBB leads to dysregulated flow of nutrients, metabolites and ions, along with less restricted immunity, which together disrupt the normal cerebral function in psychosis (18). CSF-to-serum albumin ratio remains the gold standard to evaluate BBB permeability. Its increase in the patients, along with the increase in predominantly CNS-originated S100B in blood, points towards the disruption of BBB, allowing molecules to abnormally cross between the circulation and CNS. The consistent elevation of blood S100B across treatment statuses in psychosis suggests the contribution of non-pharmaceutical factors, for example, pathological processes inherent to the disorders. This increase was more evident with greater age and longer disease history, with greater age also associated with higher BMI. Considering the partial release of S100B from adipose tissue (125, 126), further studies are needed to investigate whether more severe disruption of the BBB associates with a longer pathological process itself, greater treatment exposure, and/or weight gain along the course. Though a previous meta-analysis revealed associations between blood S100B level and PANSS scores (127), this was not detected in our analysis with more studies included, despite the low p values. It should be noted that evidence from the CSF levels of blood-derived large molecules (i.e., ACE, IgG and fibrinogen) does not consistently support the hypothesis of BBB leakage. Though in fact, fibrinogen, being the largest molecule of the three, displayed abnormal cerebral deposition and degradation in patients with schizophrenia (128). A possible explanation is that for larger molecules, both their leakage from blood and the clearance to CSF are more demanding; thus their fluid levels may be less sensitive to reflect an already compromised barrier. These findings are also limited by the small number of studies.
From a structural perspective, psychosis displayed alterations in tight junctions (TJs) and their modulators in our analyses. It should be noted that although these molecules regulate the sealing of endothelium, they also exist in other tissues, including the gastrointestinal tract. Given the proposed, though still controversial, contributions of dysbiosis and intestinal permeability to the pathophysiology of psychosis (129, 130), caution must be taken when linking these findings to vascular abnormalities. Nevertheless, alterations in these molecules may reflect increased barrier permeability in psychosis. MMPs are a family of endopeptidases upregulated by pro-inflammatory cytokines (131) and are responsible for degrading the extracellular matrix (ECM) and TJs (132). TIMPs, on the other hand, inhibit MMPs and protects the ECM. As a major focus of research among the family, MMP-9 is implicated in endothelial function, immune responses, and synaptic plasticity (132). MMP-9 gene polymorphism has been associated with schizophrenia (133). The elevation of blood MMP-9 in psychosis in our analyses may in part reflect ongoing inflammation that disrupts endothelial structure. This is in concordance with the alterations in several other TJ modifiers, including zonulin, and MMP-2 and -7, along with the TJ proteins claudin 5 and JAM-A, though the number of studies are small. On the other hand, no alteration was reported for the adherens junction proteins N- or VE-cadherin. Overall, since the investigation of junction proteins in body fluid in psychosis has begun only recently, the evidence is still sparse. It remains unclear to what extent the blood levels of these molecules correlate with their expression on the cell surface, and again where these molecules come from. Therefore, future studies investigating their fluid levels need to address these issues to help complement the existing knowledge of vascular dysfunction in psychosis.
Externally, the interaction between endothelial cells and leukocytes and ECM is partially mediated by cell adhesion molecules (CAMs) and PAI-1 (134). The alterations in blood VCAM-1 and ICAM-1 in patient subgroups likely reflect changes in endothelial functions and inflammation. In contrast, selectins, also family members of the CAMs, did not display alterations. Again the levels of CAMs expression on cell surface is unclear, and the discrepancies in the fluid levels of these molecules prompt further investigation on the demographic and clinical correlates of the participants.
In terms of angiogenesis, the NVU secretes two potent mitogens of the endothelial cells, VEGF and NGF, that modify the BBB (135). NGF has been found to elicit angiogenic responses both directly (136) and indirectly (137) through the upregulation of VEGF and nitric oxide synthase, though it is also involved in other processes including neuronal growth and differentiation (138). VEGF, besides regulating angiogenesis, also promotes the endocytosis of endothelial cells and subsequent BBB disruption (139). Genetic studies in patients with schizophrenia have identified alterations in both molecules (109, 140). While a previous meta-analysis showed robustly decreased blood NGF in schizophrenia patients compared to controls (141), this was only observed in drug-naïve patients in our analysis with stricter inclusion and exclusion criteria, despite including more recent studies. Though CSF NGF was reduced in the patients in one study (105). Similarly, decreased blood VEGF was only detected in one study containing drug-naïve patients (111). Overall, studies examining these molecules are highly heterogenous; addressing the covariates is required to further assess any effects.
Furthermore, the NVU regulates NVC by releasing multiple metabolic by-products, neuropeptides and neuromodulators (142–144). NPY and SST induce vasoconstriction in brain slices (142, 143), whereas PGE and vasoactive intestinal peptide causes vasodilation (144, 145). While the exact mechanisms of actions of these molecules has not been fully understood, it is proposed that they ultimately contribute to neuromodulation (146). Postmortem studies have identified reduced SST expression in the hippocampus and prefrontal cortex of patients with schizophrenia (147, 148). Similarly, NPY expression was reduced in the frontal but not temporal cortex (148, 149), suggestive of regional alterations. However, in our analysis, neither SST nor NPY differed in CSF levels between groups. Only one study revealed increased CSF PGE in the patients (124). One possible explanation is that CSF studies, by nature, lack the ability to assess these molecules acting in a time- and space-restricted manner. SST, in particular, has a very short half-life of 1–3 min (150).
4.1. Limitations
Several limitations should be acknowledged in this study. At the study level, the implications of fluid markers are inherently constrained by the lack of both spatial and temporal resolution. While alterations in these molecules prompt further investigation, the lack of changes in fluid levels does not exclude the possibility of local changes at specific time points. From a methodological standpoint, analyses of many of the biomarkers here were hindered by a limited number of studies and insufficient data, potentially resulting in underpowered results. Subgroup and meta-regression analyses thus could not be performed for all covariates. For the majority of biomarkers investigated, there was significant heterogeneity between studies, stemming at least partly from variations in participant demographics, clinical characteristics, and sample origins. Other potential sources of heterogeneity, including the differences in diagnoses across the spectrum, treatment regimens, detection methods and sampling time, could not be thoroughly investigated due to mixed or insufficient data. Notably, while subgroup analyses detected associations between the levels of certain biomarkers and treatment statuses, their associations with individual psychotropic medications, including antipsychotics, could not be further assessed due to the heterogenous treatment regimens and combination therapies administered. Analyses on treatment duration and doses were also extremely limited due to inadequate data. Thus, the observed alterations could be related to treatment effects, especially for biomarkers lacking subgroup analyses on treatment statuses. Moreover, some lifestyle factors known to influence vascular function, such as BMI and smoking, were only assessed in a subset of studies. Additionally, certain studies did not specify the history of substance use or other potentially confounding conditions among participants. The lack of this information made it even more challenging to compare the participants across studies, let alone quantitatively assess their correlations with the fluid markers. Furthermore, the majority of studies had risks of bias, with only 5 studies scoring 8 on NOS, potentially compromising the representativeness of the groups and the comparability between groups.
At the review level, it should be emphasized that although the molecules assessed here are involved in endothelial structure and function, shedding light on the vascular changes in psychosis, some of the blood markers are not vascular-specific. Other possible implications are discussed above. To reduce heterogeneity, we did not include studies containing participants with conditions known to influence vascular functions or controls with family history of psychiatric disorders. However, without a clear understanding of the extent to which these factors influence marker levels, this exclusion strategy may help control confounding variables, or may be overly cautious and reduce the power of the analyses, as in the case of analyses for blood NGF levels.
5. Conclusion and perspectives
This meta-analysis of 93 studies and 30 molecules provided evidence of the alterations in vascular-related biomarkers in psychotic disorders. Changes in certain biomarkers were further linked to age, treatment statuses, illness stages and duration, and sample origins. However, implications of the findings were limited by the heterogeneity across studies as well as the versatility and membrane-bound nature of some molecules. Addressing important covariates and confounders would be of extreme importance to clarify if the vascular changes are a primary process of psychosis, secondary to the events along the disease course, or methodologically related. Preferably, future studies should pursue rigorous participant selection and appropriate statistical adjustments to account for the differences in demographic (e.g., BMI and smoking) and clinical characteristics (e.g., illness duration, treatment regimens and symptom severity). Further, to validate the roles of these fluid markers in the vascular pathology in psychosis, future research could adopt a multimodal approach (e.g., by integrating neuroimaging and genetic testing) and incorporate multiple interrelated molecules to explore the relevant pathways and networks. Moreover, there remains a strong need for high-quality longitudinal studies to examine the temporal dynamics of vascular dysfunction in psychosis, and to elucidate the progression and stability of the observed alterations. Longitudinal data could then shed light on the relationships between vascular changes and disease course, treatment response, and clinical outcomes.
Data availability statement
The original contributions presented in the study are included in the article/Supplementary material, further inquiries can be directed to the corresponding author.
Author contributions
XL, SH, and PL: concept and design, acquisition, analysis, or interpretation of data, and critical revision of the manuscript for important intellectual content. XL: drafting of the manuscript. XL and SH: statistical analysis. PL: obtained funding and supervision. PL and XL: administrative, technical, or material support. All authors contributed to the article and approved the submitted version.
Funding
This work was supported by the Tsinghua University Initiative Scientific Research Program (grant number 20161080071). The funder had no role in the design and conduct of the study, or preparation and submission of the manuscript for publication.
Acknowledgments
We thank the authors of the included studies, in particular those who provided supplemental data for this study.
Conflict of interest
The authors declare that the research was conducted in the absence of any commercial or financial relationships that could be construed as a potential conflict of interest.
Publisher’s note
All claims expressed in this article are solely those of the authors and do not necessarily represent those of their affiliated organizations, or those of the publisher, the editors and the reviewers. Any product that may be evaluated in this article, or claim that may be made by its manufacturer, is not guaranteed or endorsed by the publisher.
Supplementary material
The Supplementary material for this article can be found online at: https://www.frontiersin.org/articles/10.3389/fpsyt.2023.1241422/full#supplementary-material
References
1. Enez Darcin, A, Yalcin Cavus, S, Dilbaz, N, Kaya, H, and Dogan, E. Metabolic syndrome in drug-naïve and drug-free patients with schizophrenia and in their siblings. Schizophr Res. (2015) 166:201–6. doi: 10.1016/j.schres.2015.05.004
2. Garrido-Torres, N, Rocha-Gonzalez, I, Alameda, L, Rodriguez-Gangoso, A, Vilches, A, Canal-Rivero, M, et al. Metabolic syndrome in antipsychotic-naïve patients with first-episode psychosis: a systematic review and meta-analysis. Psychol Med. (2021) 51:2307–20. doi: 10.1017/S0033291721002853
3. Perry, BI, McIntosh, G, Weich, S, Singh, S, and Rees, K. The association between first-episode psychosis and abnormal glycaemic control: systematic review and meta-analysis. Lancet Psychiatry. (2016) 3:1049–58. doi: 10.1016/S2215-0366(16)30262-0
4. Mitchell, AJ, Vancampfort, D, De Herdt, A, Yu, W, and De Hert, M. Is the prevalence of metabolic syndrome and metabolic abnormalities increased in early schizophrenia? A comparative meta-analysis of first episode, untreated and treated patients. Schizophr Bull. (2013) 39:295–5. doi: 10.1093/schbul/sbs082
5. Hagi, K, Nosaka, T, Dickinson, D, Lindenmayer, JP, Lee, J, Friedman, J, et al. Association between cardiovascular risk factors and cognitive impairment in people with schizophrenia: a systematic review and Meta-analysis. JAMA Psychiat. (2021) 78:510–8. doi: 10.1001/jamapsychiatry.2021.0015
6. Olfson, M, Gerhard, T, Huang, C, Crystal, S, and Stroup, TS. Premature mortality among adults with schizophrenia in the United States. JAMA Psychiat. (2015) 72:1172–81. doi: 10.1001/jamapsychiatry.2015.1737
7. Correll, CU, Solmi, M, Croatto, G, Schneider, LK, Rohani-Montez, SC, Fairley, L, et al. Mortality in people with schizophrenia: a systematic review and meta-analysis of relative risk and aggravating or attenuating factors. World Psychiatry. (2022) 21:248–1. doi: 10.1002/wps.20994
8. Uranova, NA, Zimina, IS, Vikhreva, OV, Krukov, NO, Rachmanova, VI, and Orlovskaya, DD. Ultrastructural damage of capillaries in the neocortex in schizophrenia. World J Biol Psychiatry. (2010) 11:567–8. doi: 10.3109/15622970903414188
9. Najjar, S, Pahlajani, S, De Sanctis, V, Stern, JNH, Najjar, A, and Chong, D. Neurovascular unit dysfunction and blood-brain barrier hyperpermeability contribute to schizophrenia neurobiology: a theoretical integration of clinical and experimental evidence. Front Psych. (2017) 8:83. doi: 10.3389/fpsyt.2017.00083
10. de Klerk, OL, Willemsen, AT, Bosker, FJ, Bartels, AL, Hendrikse, NH, den Boer, JA, et al. Regional increase in P-glycoprotein function in the blood-brain barrier of patients with chronic schizophrenia: a PET study with [(11)C]verapamil as a probe for P-glycoprotein function. Psychiatry Res. (2010) 183:151–6. doi: 10.1016/j.pscychresns.2010.05.002
11. Mwansisya, TE, Hu, A, Li, Y, Chen, X, Wu, G, Huang, X, et al. Task and resting-state fMRI studies in first-episode schizophrenia: a systematic review. Schizophr Res. (2017) 189:9–18. doi: 10.1016/j.schres.2017.02.026
12. Shaw, K, Bell, L, Boyd, K, Grijseels, D, Clarke, D, Bonnar, O, et al. Neurovascular coupling and oxygenation are decreased in hippocampus compared to neocortex because of microvascular differences. Nat Commun. (2021) 12:1–16. doi: 10.1038/s41467-021-23508-y
13. Saiga, R, Uesugi, M, Takeuchi, A, Uesugi, K, Suzuki, Y, Takekoshi, S, et al. Brain capillary structures of schizophrenia cases and controls show a correlation with their neuron structures. Sci Rep. (2021) 11:1–9. doi: 10.1038/s41598-021-91233-z
14. Mizutani, R, Saiga, R, Takeuchi, A, Uesugi, K, Terada, Y, Suzuki, Y, et al. Three-dimensional alteration of neurites in schizophrenia. Transl Psychiatry. (2019) 9:1–12. doi: 10.1038/s41398-019-0427-4
15. Hua, J, Brandt, AS, Lee, SW, Blair, NIS, Wu, YK, Lui, S, et al. Abnormal Grey matter arteriolar cerebral blood volume in schizophrenia measured with 3D inflow-based vascular-space-occupancy MRI at 7T. Schizophr Bull. (2017) 43:620–2. doi: 10.1093/schbul/sbw109
16. Egger, ST, Bobes, J, Rauen, K, Seifritz, E, Vetter, S, and Schuepbach, D. Psychopathological symptom load and distinguishable cerebral blood flow velocity patterns in patients with schizophrenia and healthy controls: a functional transcranial doppler study. Front Psych. (2021) 12:960. doi: 10.3389/fpsyt.2021.679021
17. Peitl, V, Badžim, VA, Markoš, IŠ, Rendulić, A, Matešić, K, and Karlović, D. Improvements of frontotemporal cerebral blood flow and cognitive functioning in patients with first episode of schizophrenia treated with long-acting aripiprazole. J Clin Psychopharmacol. (2021) 41:638–3. doi: 10.1097/JCP.0000000000001477
18. Pollak, TA, Drndarski, S, Stone, JM, David, AS, McGuire, P, and Abbott, NJ. The blood-brain barrier in psychosis. Lancet Psychiatry. (2018) 5:79–92. doi: 10.1016/S2215-0366(17)30293-6
19. Page, MJ, McKenzie, JE, Bossuyt, PM, Boutron, I, Hoffmann, TC, Mulrow, CD, et al. The PRISMA 2020 statement: an updated guideline for reporting systematic reviews. BMJ. (2021) 372:n71. doi: 10.1136/bmj.n71
20. Bramer, WM, Giustini, D, de Jonge, GB, Holland, L, and Bekhuis, T. De-duplication of database search results for systematic reviews in EndNote. J Med Libr Assoc. (2016) 104:240–3. doi: 10.3163/1536-5050.104.3.014
21. Wan, X, Wang, W, Liu, J, and Tong, T. Estimating the sample mean and standard deviation from the sample size, median, range and/or interquartile range. BMC Med Res Methodol. (2014) 14:135. doi: 10.1186/1471-2288-14-135
22. Luo, D, Wan, X, Liu, J, and Tong, T. Optimally estimating the sample mean from the sample size, median, mid-range, and/or mid-quartile range. Stat Methods Med Res. (2018) 27:1785–05. doi: 10.1177/0962280216669183
23. Higgins, JP, White, IR, and Anzures-Cabrera, J. Meta-analysis of skewed data: combining results reported on log-transformed or raw scales. Stat Med. (2008) 27:6072–92. doi: 10.1002/sim.3427
24. Wells, GA, Shea, B, O’Connell, D, Peterson, J, Welch, V, Losos, M, et al. The Newcastle-Ottawa scale (NOS) for assessing the quality of nonrandomised studies in meta-analyses. Oxford: Oxford University Press (2000).
25. Peters, JL, Sutton, AJ, Jones, DR, Abrams, KR, and Rushton, L. Contour-enhanced meta-analysis funnel plots help distinguish publication bias from other causes of asymmetry. J Clin Epidemiol. (2008) 61:991–6. doi: 10.1016/j.jclinepi.2007.11.010
26. Egger, M, Smith, GD, Schneider, M, and Minder, C. Bias in meta-analysis detected by a simple, graphical test. BMJ. (1997) 315:629–4. doi: 10.1136/bmj.315.7109.629
27. Bowden, J, Tierney, JF, Copas, AJ, and Burdett, S. Quantifying, displaying and accounting for heterogeneity in the meta-analysis of RCTs using standard and generalised Qstatistics. BMC Med Res Methodol. (2011) 11:1–12. doi: 10.1186/1471-2288-11-41
28. Higgins, JP, Thomas, J, Chandler, J, Cumpston, M, Li, T, Page, MJ, et al. Cochrane handbook for systematic reviews of interventions. New Jersey: John Wiley & Sons (2019).
29. Higgins, JP, Thompson, SG, Deeks, JJ, and Altman, DG. Measuring inconsistency in meta-analyses. BMJ. (2003) 327:557–15. doi: 10.1136/bmj.327.7414.557
30. DerSimonian, R, and Laird, N. Meta-analysis in clinical trials. Control Clin Trials. (1986) 7:177–8. doi: 10.1016/0197-2456(86)90046-2
31. Galbraith, R. Graphical display of estimates having differing standard errors. Technometrics. (1988) 30:271–1. doi: 10.1080/00401706.1988.10488400
32. Steiner, J, Bielau, H, Bernstein, HG, Bogerts, B, and Wunderlich, MT. Increased cerebrospinal fluid and serum levels of S100B in first-onset schizophrenia are not related to a degenerative release of glial fibrillar acidic protein, myelin basic protein and neurone-specific enolase from glia or neurones. J Neurol Neurosurg Psych. (2006) 77:1284–7. doi: 10.1136/jnnp.2006.093427
33. Severance, EG, Gressitt, KL, Alaedini, A, Rohleder, C, Enning, F, Bumb, JM, et al. IgG dynamics of dietary antigens point to cerebrospinal fluid barrier or flow dysfunction in first-episode schizophrenia. Brain Behav Immun. (2015) 44:148–8. doi: 10.1016/j.bbi.2014.09.009
34. Roos, RP, Davis, K, and Meltzer, HY. Immunoglobulin studies in patients with psychiatric diseases. Arch Gen Psychiatry. (1985) 42:124–8. doi: 10.1001/archpsyc.1985.01790250018002
35. Gattaz, WF, Lara, DR, Elkis, H, Portela, LV, Gonçalves, CA, Tort, AB, et al. Decreased S100-beta protein in schizophrenia: preliminary evidence. Schizophr Res. (2000) 43:91–5. doi: 10.1016/S0920-9964(99)00146-2
36. Goff, DC, Zeng, B, Ardekani, BA, Diminich, ED, Tang, Y, Fan, X, et al. Association of Hippocampal Atrophy with Duration of untreated psychosis and molecular biomarkers during initial antipsychotic treatment of first-episode psychosis. JAMA Psychiat. (2018) 75:370–8. doi: 10.1001/jamapsychiatry.2017.4595
37. Hong, W, Zhao, M, Li, HZ, Peng, FL, Wang, F, Li, NN, et al. Higher plasma S100B concentrations in schizophrenia patients, and dependently associated with inflammatory markers. Sci Rep. (2016) 6:27584. doi: 10.1038/srep27584
38. Ling, SH, Tang, YL, Jiang, F, Wiste, A, Guo, SS, Weng, YZ, et al. Plasma S-100B protein in Chinese patients with schizophrenia: comparison with healthy controls and effect of antipsychotics treatment. J Psychiatr Res. (2007) 41:36–42. doi: 10.1016/j.jpsychires.2005.11.006
39. Qing, Y, Xu, LH, Cui, GP, Sun, LY, Hu, XW, Yang, XH, et al. Salivary microbiome profiling reveals a dysbiotic schizophrenia-associated microbiota. NPJ Schizophr. (2021) 7:10. doi: 10.1038/s41537-021-00180-1
40. Rothermundt, M, Missler, U, Arolt, V, Peters, M, Leadbeater, J, Wiesmann, M, et al. Increased S100B blood levels in unmedicated and treated schizophrenic patients are correlated with negative symptomatology. Mol Psychiatry. (2001) 6:445–9. doi: 10.1038/sj.mp.4000889
41. Uzbay, T, Goktalay, G, Kayir, H, Eker, SS, Sarandol, A, Oral, S, et al. Increased plasma agmatine levels in patients with schizophrenia. J Psychiatr Res. (2013) 47:1054–60. doi: 10.1016/j.jpsychires.2013.04.004
42. Wiesmann, M, Wandinger, KP, Missler, U, Eckhoff, D, Rothermundt, M, Arolt, V, et al. Elevated plasma levels of S-100b protein in schizophrenic patients. Biol Psychiatry. (1999) 45:1508–11. doi: 10.1016/S0006-3223(98)00217-0
43. Chen, S, Tian, L, Chen, N, Xiu, MH, Wang, ZR, Yang, GG, et al. Cognitive dysfunction correlates with elevated serum S100B concentration in drug-free acutely relapsed patients with schizophrenia. Psychiatry Res. (2017) 247:6–11. doi: 10.1016/j.psychres.2016.09.029
44. Dai, N, Jie, HJ, Duan, Y, Xiong, P, Xu, XF, Chen, P, et al. Different serum protein factor levels in first-episode drug-naive patients with schizophrenia characterized by positive and negative symptoms. Psychiatry Clin Neurosci. (2020) 74:472–9. doi: 10.1111/pcn.13078
45. Falcone, T, Janigro, D, Lovell, R, Simon, B, Brown, CA, Herrera, M, et al. S100B blood levels and childhood trauma in adolescent inpatients. J Psychiatr Res. (2015) 62:14–22. doi: 10.1016/j.jpsychires.2014.12.002
46. Gerasimou, C, Tsoporis, JN, Siafakas, N, Hatziagelaki, E, Kallergi, M, Chatziioannou, SN, et al. A longitudinal study of alterations of S100B, sRAGE and Fas ligand in association to olanzapine medication in a sample of first episode patients with schizophrenia. CNS Neurol Disord Drug Targets. (2018) 17:383–8. doi: 10.2174/1871527317666180605120244
47. Hendouei, N, Hosseini, SH, Panahi, A, Khazaeipour, Z, Barari, F, Sahebnasagh, A, et al. Negative correlation between serum S100B and leptin levels in schizophrenic patients during treatment with clozapine and risperidone: preliminary evidence. Iranian J Pharm Res. (2016) 15:323–15.
48. Huang, Z, Kang, M, Li, G, Xiong, P, Chen, H, Kang, L, et al. Predictive effect of bayes discrimination in the level of serum protein factors and cognitive dysfunction in schizophrenia. J Psychiatr Res. (2022) 151:539–5. doi: 10.1016/j.jpsychires.2022.05.004
49. Kannan, G, Gressitt, KL, Yang, S, Stallings, CR, Katsafanas, E, Schweinfurth, LA, et al. Pathogen-mediated NMDA receptor autoimmunity and cellular barrier dysfunction in schizophrenia. Transl Psychiatry. (2017) 7:8. doi: 10.1038/tp.2017.162
50. Kozlowska, E, Brzezinska-Blaszczyk, E, Agier, J, Wysokinski, A, and Zelechowska, P. Alarmins (IL-33, sST2, HMGB1, and S100B) as potential biomarkers for schizophrenia. J Psychiatr Res. (2021) 138:380–7. doi: 10.1016/j.jpsychires.2021.04.019
51. Lara, DR, Gama, CS, Belmonte-de-Abreu, P, Portela, LVC, Goncalves, CA, Fonseca, M, et al. Increased serum S100B protein in schizophrenia: a study in medication-free patients. J Psychiatr Res. (2001) 35:11–4. doi: 10.1016/S0022-3956(01)00003-6
52. Milleit, B, Smesny, S, Rothermundt, M, Preul, C, Schroeter, ML, von Eiff, C, et al. Serum S100B protein is specifically related to White matter changes in schizophrenia. Front Cell Neurosci. (2016) 10:14. doi: 10.3389/fncel.2016.00033
53. Morera-Fumero, AL, Diaz-Mesa, E, Abreu-Gonzalez, P, Fernandez-Lopez, L, and Cejas-Mendez, MD. Day/night changes in serum S100B protein concentrations in acute paranoid schizophrenia. Prog Neuro-Psychopharmacol Biol Psychiatry. (2017) 75:207–2. doi: 10.1016/j.pnpbp.2017.02.007
54. Morera-Fumero, AL, Diaz-Mesa, E, Fernandez-Lopez, L, Abreu-Gonzalez, P, and Henry-Benitez, MS. Serum s100b protein levels as a neuroinflammatory biomarker of acutely relapsed paranoid schizophrenia patients. Acta Neuropsychiatr. (2023) 35:138–6. doi: 10.1017/neu.2022.37
55. O'Connell, K, Thakore, J, and Dev, KK. Levels of S100B are raised in female patients with schizophrenia. BMC Psychiatry. (2013) 13:9. doi: 10.1186/1471-244X-13-146
56. Qi, LY, Xiu, MH, Chen, DC, Wang, F, Kosten, TA, Kosten, TR, et al. Increased serum S100B levels in chronic schizophrenic patients on long-term clozapine or typical antipsychotics. Neurosci Lett. (2009) 462:113–7. doi: 10.1016/j.neulet.2009.06.019
57. Rothermundt, M, Ohrmann, P, Abel, S, Siegmund, A, Pedersen, A, Ponath, G, et al. Glial cell activation in a subgroup of patients with schizophrenia indicated by increased S100B serum concentrations and elevated myo-inositol. Prog Neuro-Psychopharmacol Biol Psychiatry. (2007) 31:361–4. doi: 10.1016/j.pnpbp.2006.09.013
58. Rothermundt, M, Ponath, G, Glaser, T, Hetzel, G, and Arolt, V. S100B serum levels and long-term improvement of negative symptoms in patients with schizophrenia. Neuropsychopharmacology. (2004) 29:1004–11. doi: 10.1038/sj.npp.1300403
59. Sarandol, A, Kirli, S, Akkaya, C, Altin, A, Demirci, M, and Sarandol, E. Oxidative-antioxidative systems and their relation with serum S100B levels in patients with schizophrenia: effects of short term antipsychotic treatment. Prog Neuro-Psychopharmacol Biol Psychiatry. (2007) 31:1164–9. doi: 10.1016/j.pnpbp.2007.03.008
60. Schmitt, A, Bertsch, T, Henning, U, Tost, H, Klimke, A, Henn, FA, et al. Increased serum S100B in elderly, chronic schizophrenic patients: negative correlation with deficit symptoms. Schizophr Res. (2005) 80:305–3. doi: 10.1016/j.schres.2005.04.013
61. Schroeter, ML, Abdul-Khaliq, H, Fruhauf, S, Hohne, R, Schick, G, Diefenbacher, A, et al. Serum S100B is increased during early treatment with antipsychotics and in deficit schizophrenia. Schizophr Res. (2003) 62:231–6. doi: 10.1016/S0920-9964(02)00383-3
62. Schroeter, ML, Abdul-Khaliq, H, Krebs, M, Dieferibacher, A, and Blasig, IE. Neuron-specific enolase is unaltered whereas S100B is elevated in serum of patients with schizophrenia - original research and meta-analysis. Psychiatry Res. (2009) 167:66–72. doi: 10.1016/j.psychres.2008.01.002
63. Steiner, J, Walter, M, Wunderlich, MT, Bernstein, HG, Panteli, B, Brauner, M, et al. A new pathophysiological aspect of S100B in schizophrenia: potential regulation of S100B by its scavenger soluble RAGE. Biol Psychiatry. (2009) 65:1107–10. doi: 10.1016/j.biopsych.2008.10.044
64. Zhang, XY, Xiu, MH, Chen, DC, Zhu, FY, Wu, GY, Haile, CN, et al. Increased S100B serum levels in schizophrenic patients with tardive dyskinesia: association with dyskinetic movements. J Psychiatr Res. (2010) 44:429–3. doi: 10.1016/j.jpsychires.2009.10.012
65. Zhang, XY, Xiu, MH, Song, C, Chen, DC, Wu, GY, Haile, CN, et al. Increased serum S100B in never-medicated and medicated schizophrenic patients. J Psychiatr Res. (2010) 44:1236–40. doi: 10.1016/j.jpsychires.2010.04.023
66. Hidese, S, Hattori, K, Sasayama, D, Tsumagari, T, Miyakawa, T, Matsumura, R, et al. Cerebrospinal fluid neuroplasticity-associated protein levels in patients with psychiatric disorders: a multiplex immunoassay study. Transl Psychiatry. (2020) 10:5. doi: 10.1038/s41398-020-0843-5
67. Hayes, LN, Severance, EG, Leek, JT, Gressitt, KL, Rohleder, C, Coughlin, JM, et al. Inflammatory molecular signature associated with infectious agents in psychosis. Schizophr Bull. (2014) 40:963–2. doi: 10.1093/schbul/sbu052
68. Wahlbeck, K, Ahokas, A, Miettinen, K, Nikkilä, H, and Rimón, R. Higher cerebrospinal fluid angiotensin-converting enzyme levels in neuroleptic-treated than in drug-free patients with schizophrenia. Schizophr Bull. (1998) 24:391–7. doi: 10.1093/oxfordjournals.schbul.a033334
69. Wahlbeck, K, Ahokas, A, Nikkilä, H, Miettinen, K, and Rimón, R. A longitudinal study of cerebrospinal fluid angiotensin-converting enzyme in neuroleptic-treated schizophrenia. Prog Neuro-Psychopharmacol Biol Psychiatry. (1997) 21:591–9. doi: 10.1016/S0278-5846(97)00034-1
70. Wahlbeck, K, Rimón, R, and Fyhrquist, F. Elevated angiotensin-converting enzyme (kininase II) in the cerebrospinal fluid of neuroleptic-treated schizophrenic patients. Schizophr Res. (1993) 9:77–82. doi: 10.1016/0920-9964(93)90013-9
71. Ali, FT, Abd El-Azeem, EM, Hamed, MA, Ali, MAM, Abd Al-Kader, NM, and Hassan, EA. Redox dysregulation, immuno-inflammatory alterations and genetic variants of BDNF and MMP-9 in schizophrenia: pathophysiological and phenotypic implications. Schizophr Res. (2017) 188:98–9. doi: 10.1016/j.schres.2017.01.016
72. Arabska, J, Margulska, A, Strzelecki, D, and Wysokinski, A. Does metabolic status affect serum levels of BDNF and MMP-9 in patients with schizophrenia? Nord J Psychiatry. (2019) 73:515–1. doi: 10.1080/08039488.2019.1658126
73. Chenniappan, R, Nandeesha, H, Kattimani, S, Goud, AC, and Thiagarajan, D. Risperidone reduces matrix Metalloproteinase-9 and increases Neurotrophin-3 in schizophrenia Spectrum of disorder. Indian J Clin Biochem. (2022) 37:342–8. doi: 10.1007/s12291-021-00985-y
74. Devanarayanan, S, Nandeesha, H, Kattimani, S, and Sarkar, S. Relationship between matrix metalloproteinase-9 and oxidative stress in drug-free male schizophrenia: a case control study. Clin Chem Lab Med. (2016) 54:447–2. doi: 10.1515/cclm-2015-0212
75. Keshri, N, Nandeesha, H, Rajappa, M, and Menon, V. Matrix metalloproteinase-9 increases the risk of cognitive impairment in schizophrenia. Nord J Psychiatry. (2021) 75:130–4. doi: 10.1080/08039488.2020.1808901
76. Kudo, N, Yamamori, H, Ishima, T, Nemoto, K, Yasuda, Y, Fujimoto, M, et al. Plasma levels of matrix metalloproteinase-9 (MMP-9) are associated with cognitive performance in patients with schizophrenia. Neuropsychopharmacol Rep. (2020) 40:150–6. doi: 10.1002/npr2.12098
77. Niitsu, T, Ishima, T, Yoshida, T, Hashimoto, T, Matsuzawa, D, Shirayama, Y, et al. A positive correlation between serum levels of mature brain-derived neurotrophic factor and negative symptoms in schizophrenia. Psychiatry Res. (2014) 215:268–3. doi: 10.1016/j.psychres.2013.12.009
78. Seitz-Holland, J, Seethaler, M, Makris, N, Rushmore, J, Cho, KIK, Rizzoni, E, et al. The association of matrix metalloproteinase 9 (MMP9) with hippocampal volume in schizophrenia: a preliminary MRI study. Neuropsychopharmacology. (2022) 47:524–15. doi: 10.1038/s41386-021-00997-5
79. Shibasaki, C, Takebayashi, M, Itagaki, K, Abe, H, Kajitani, N, Okada-Tsuchioka, M, et al. Altered serum levels of matrix Metalloproteinase-2,-9 in response to electroconvulsive therapy for mood disorders. Int J Neuropsychopharmacol. (2016) 19:pyw019. doi: 10.1093/ijnp/pyw019
80. Gokulakrishnan, K, Nikhil, J, Vs, S, Holla, B, Thirumoorthy, C, Sandhya, N, et al. Altered intestinal permeability biomarkers in schizophrenia: a possible link with subclinical inflammation. Ann Neurosci. (2022) 29:151–8. doi: 10.1177/09727531221108849
81. Usta, A, Kilic, F, Demirdas, A, Isik, U, Doguc, DK, and Bozkurt, M. Serum zonulin and claudin-5 levels in patients with schizophrenia. Eur Arch Psychiatry Clin Neurosci. (2021) 271:767–3. doi: 10.1007/s00406-020-01152-9
82. Iwata, Y, Suzuki, K, Nakamura, K, Matsuzaki, H, Sekine, Y, Tsuchiya, KJ, et al. Increased levels of serum soluble L-selectin in unmedicated patients with schizophrenia. Schizophr Res. (2007) 89:154–15. doi: 10.1016/j.schres.2006.08.026
83. Kavzoglu, SO, and Hariri, AG. Intracellular adhesion molecule (ICAM-1), vascular cell adhesion molecule (VCAM-1) and E-selectin levels in first episode schizophrenic patients. Klinik Psikofarmakoloji Bulteni-Bull Clin Psychopharmacol. (2013) 23:205–4. doi: 10.5455/bcp.20130713091100
84. Mohite, S, Yang, F, Amin, PA, Zunta-Soares, G, Colpo, GD, Stertz, L, et al. Plasma soluble L-selectin in medicated patients with schizophrenia and healthy controls. PLoS One. (2017) 12:10. doi: 10.1371/journal.pone.0174073
85. Masopust, J, Maly, R, Andrys, C, Valis, M, Bazant, J, and Hosak, L. The dynamics of haemostatic parameters in acute psychotic patients: a one-year prospective study. Psychiatr Danub. (2013) 25:142–8.
86. Sheikh, MA, O'Connell, KS, Lekva, T, Szabo, A, Akkouh, IA, Osete, JR, et al. Systemic cell adhesion molecules in severe mental illness: potential role of intercellular CAM-1 in linking peripheral and neuroinflammation. Biol Psychiatry. (2023) 93:187–6. doi: 10.1016/j.biopsych.2022.06.029
87. Cai, HQ, Catts, VS, Webster, MJ, Galletly, C, Liu, D, O'Donnell, M, et al. Increased macrophages and changed brain endothelial cell gene expression in the frontal cortex of people with schizophrenia displaying inflammation. Mol Psychiatry. (2020) 25:761–5. doi: 10.1038/s41380-018-0235-x
88. Klaus, F, Mitchell, K, Liou, SC, Eyler, LT, and Nguyen, TT. Chemokine MCP1 is associated with cognitive flexibility in schizophrenia: a preliminary analysis. J Psychiatr Res. (2021) 138:139–5. doi: 10.1016/j.jpsychires.2021.04.007
89. Kronig, H, Riedel, M, Schwarz, MJ, Strassnig, M, Moller, HJ, Ackenheil, M, et al. ICAM G241A polymorphism and soluble ICAM-1 serum levels: evidence for an active immune process in schizophrenia. Neuroimmunomodulation. (2005) 12:54–9. doi: 10.1159/000082364
90. Nguyen, TT, Dev, SI, Chen, GQ, Liou, SC, Martin, AS, Irwin, MR, et al. Abnormal levels of vascular endothelial biomarkers in schizophrenia. Eur Arch Psychiatry Clin Neurosci. (2018) 268:849–15. doi: 10.1007/s00406-017-0842-6
91. Radu, G, Luca, C, Petrescu, L, Bordejevic, DA, Tomescu, MC, Andor, M, et al. The predictive value of endothelial inflammatory markers in the onset of schizophrenia. Neuropsychiatr Dis Treat. (2020) 16:545–5. doi: 10.2147/NDT.S240349
92. Schwarz, MJ, Riedel, M, Gruber, R, Muller, N, and Ackenheil, M. Autoantibodies against 60-kDa heat shock protein in schizophrenia. Eur Arch Psychiatry Clin Neurosci. (1998) 248:282–8. doi: 10.1007/s004060050051
93. Stefanovic, MP, Petronijevic, N, Dunjic-Kostic, B, Velimirovic, M, Nikolic, T, Jurisic, V, et al. Role of sICAM-1 and sVCAM-1 as biomarkers in early and late stages of schizophrenia. J Psychiatr Res. (2016) 73:45–52. doi: 10.1016/j.jpsychires.2015.11.002
94. Tylec, A, Skalecki, M, Kocot, J, and Kurzepa, J. Activity of selected metalloproteinases in neurodegenerative diseases of the central nervous system as exemplified by dementia and schizophrenia. Psychiatr Pol. (2021) 55:1221–33. doi: 10.12740/PP/126662
95. Bocchio-Chiavetto, L, Zanardini, R, Tosato, S, Ventriglia, M, Ferrari, C, Bonetto, C, et al. Immune and metabolic alterations in first episode psychosis (FEP) patients. Brain Behav Immun. (2018) 70:315–4. doi: 10.1016/j.bbi.2018.03.013
96. Chen, SZ, Jiang, HT, Liu, Y, Hou, ZH, Yue, YY, Zhang, YQ, et al. Combined serum levels of multiple proteins in tPA-BDNF pathway may aid the diagnosis of five mental disorders. Sci Rep. (2017) 7:9. doi: 10.1038/s41598-017-06832-6
97. Elmi, S, Sahu, G, Malavade, K, and Jacob, T. Role of tissue plasminogen activator and plasminogen activator inhibitor as potential biomarkers in psychosis. Asian J Psychiatr. (2019) 43:105–15. doi: 10.1016/j.ajp.2019.05.021
98. Omori, W, Hattori, K, Kajitani, N, Okada-Tsuchioka, M, Boku, S, Kunugi, H, et al. Increased matrix metalloproteinases in cerebrospinal fluids of patients with major depressive disorder and schizophrenia. Int J Neuropsychopharmacol. (2020) 23:713–15. doi: 10.1093/ijnp/pyaa049
99. Smirnova, L, Seregin, A, Boksha, I, Dmitrieva, E, Simutkin, G, Kornetova, E, et al. The difference in serum proteomes in schizophrenia and bipolar disorder. BMC Genomics. (2019) 20:14. doi: 10.1186/s12864-019-5848-1
100. Yazla, E, Kayadibi, H, Cetin, I, Aydinoglu, U, and Karadere, ME. Evaluation of changes in peripheric biomarkers related to blood brain barrier damage in patients with schizophrenia and their correlation with symptoms. Clin Psychopharmacol Neurosci. (2022) 20:504–3. doi: 10.9758/cpn.2022.20.3.504
101. Ajami, A, Hosseini, SH, Taghipour, M, and Khalilian, A. Changes in serum levels of brain derived neurotrophic factor and nerve growth factor-beta in schizophrenic patients before and after treatment. Scand J Immunol. (2014) 80:36–42. doi: 10.1111/sji.12158
102. Bersani, G, Iannitelli, A, Massoni, E, Garavini, A, Grilli, A, Di Giannantonio, M, et al. Ultradian variation of nerve growth factor plasma levels in healthy and schizophrenic subjects. Int J Immunopathol Pharmacol. (2004) 17:367–2. doi: 10.1177/039463200401700316
103. Jockers-Scherübl, MC, Matthies, U, Danker-Hopfe, H, Lang, UE, Mahlberg, R, and Hellweg, R. Chronic cannabis abuse raises nerve growth factor serum concentrations in drug-naive schizophrenic patients. J Psychopharmacol. (2003) 17:439–5. doi: 10.1177/0269881103174007
104. Jockers-Scherübl, MC, Rentzsch, J, Danker-Hopfe, H, Radzei, N, Schürer, F, Bahri, S, et al. Adequate antipsychotic treatment normalizes serum nerve growth factor concentrations in schizophrenia with and without cannabis or additional substance abuse. Neurosci Lett. (2006) 400:262–6. doi: 10.1016/j.neulet.2006.02.056
105. Kale, A, Joshi, S, Pillai, A, Naphade, N, Raju, M, Nasrallah, H, et al. Reduced cerebrospinal fluid and plasma nerve growth factor in drug-naïve psychotic patients. Schizophr Res. (2009) 115:209–4. doi: 10.1016/j.schres.2009.07.022
106. Lee, BH, and Kim, YK. Increased plasma brain-derived neurotropic factor, not nerve growth factor-Beta, in schizophrenia patients with better response to risperidone treatment. Neuropsychobiology. (2009) 59:51–8. doi: 10.1159/000205518
107. Neugebauer, K, Hammans, C, Wensing, T, Kumar, V, Grodd, W, Mevissen, L, et al. Nerve growth factor serum levels are associated with regional Gray matter volume differences in schizophrenia patients. Front Psych. (2019) 10:275. doi: 10.3389/fpsyt.2019.00275
108. Turkmen, BA, Yazici, E, Erdogan, DG, Suda, MA, and Yazici, AB. BDNF, GDNF, NGF and klotho levels and neurocognitive functions in acute term of schizophrenia. BMC Psychiatry. (2021) 21:562. doi: 10.1186/s12888-021-03578-4
109. Zakharyan, R, Atshemyan, S, Gevorgyan, A, and Boyajyan, A. Nerve growth factor and its receptor in schizophrenia. BBA Clin. (2014) 1:24–9. doi: 10.1016/j.bbacli.2014.05.001
110. Balotsev, R, Koido, K, Vasar, V, Janno, S, Kriisa, K, Mahlapuu, R, et al. Inflammatory, cardio-metabolic and diabetic profiling of chronic schizophrenia. Eur Psychiatry. (2017) 39:1–10. doi: 10.1016/j.eurpsy.2016.05.010
111. Lee, BH, Hong, JP, Hwang, JA, Ham, BJ, Na, KS, Kim, WJ, et al. Alterations in plasma vascular endothelial growth factor levels in patients with schizophrenia before and after treatment. Psychiatry Res. (2015) 228:95–9. doi: 10.1016/j.psychres.2015.04.020
112. Lin, YZ, Peng, YM, He, S, Xu, JJ, Shi, Y, Su, YS, et al. Serum IL-1ra, a novel biomarker predicting olanzapine-induced hypercholesterolemia and hyperleptinemia in schizophrenia. Prog Neuro-Psychopharmacol Biol Psychiatry. (2018) 84:71–8. doi: 10.1016/j.pnpbp.2018.01.020
113. Liu, YH, Chen, JX, Huang, L, Yan, SX, Bian, QT, and Yang, FD. Relationships among retinal nerve Fiber layer thickness, vascular endothelial growth factor, and cognitive impairment in patients with schizophrenia. Neuropsychiatr Dis Treat. (2021) 17:3597–06. doi: 10.2147/NDT.S336077
114. Liu, YH, Huang, LZ, Chen, JX, Tan, SP, Zhao, KX, Yan, SX, et al. Retinal venule correlation with schizophrenia. Int J Clin Exp Med. (2020) 13:6927–35.
115. Murphy, BP, Pang, TY, Hannan, AJ, Proffitt, TM, McConchie, M, Kerr, M, et al. Vascular endothelial growth factor and brain-derived neurotrophic factor in quetiapine treated first-episode psychosis. Schizophr Res Treat. (2014) 2014:719395. doi: 10.1155/2014/719395
116. Pillai, A, Howell, KR, Ahmed, AO, Weinberg, D, Allen, KM, Bruggemann, J, et al. Association of serum VEGF levels with prefrontal cortex volume in schizophrenia. Mol Psychiatry. (2016) 21:686–2. doi: 10.1038/mp.2015.96
117. Xiao, WH, Zhan, QQ, Ye, F, Tang, XW, Li, J, Dong, H, et al. Baseline serum vascular endothelial growth factor levels predict treatment response to antipsychotic medication in patients with schizophrenia. Eur Neuropsychopharmacol. (2018) 28:603–9. doi: 10.1016/j.euroneuro.2018.03.007
118. Peters, J, Van Kammen, DP, Gelernter, J, Yao, J, and Shaw, D. Neuropeptide Y-like immunoreactivity in schizophrenia. Relationsh Clin Meas Schizophr Res. (1990) 3:287–94. doi: 10.1016/0920-9964(90)90012-v
119. Widerlov, E, Lindstrom, LH, Wahlestedt, C, and Ekman, R. Neuropeptide-Y and peptide-Yy as possible cerebrospinal-fluid markers for major depression and schizophrenia. Respect J Psych Res. (1988) 22:69–79. doi: 10.1016/0022-3956(88)90030-1
120. Bissette, G, Widerlöv, E, Walléus, H, Karlsson, I, Eklund, K, Forsman, A, et al. Alterations in cerebrospinal fluid concentrations of somatostatinlike immunoreactivity in neuropsychiatric disorders. Arch Gen Psychiatry. (1986) 43:1148–51. doi: 10.1001/archpsyc.1986.01800120034008
121. Gerner, RH, Vankammen, DP, and Ninan, PT. Cerebrospinal-fluid cholecystokinin, bombesin and somatostatin in schizophrenia and Normals. Prog Neuro-Psychopharmacol Biol Psychiatry. (1985) 9:73–82. doi: 10.1016/0278-5846(85)90181-2
122. Reinikainen, KJ, Koponen, H, Jolkkonen, J, and Riekkinen, PJ. Decreased somatostatin-like immunoreactivity in the cerebrospinal-fluid of chronic-schizophrenic patients with cognitive impairment. Psychiatry Res. (1990) 33:307–2. doi: 10.1016/0165-1781(90)90047-9
123. Rubinow, DR. Cerebrospinal fluid somatostatin and psychiatric illness. Biol Psychiatry. (1986) 21:341–5. doi: 10.1016/0006-3223(86)90163-0
124. Mathe, AA, Sedvall, G, Wiesel, FA, and Nyback, H. Increased content of immunoreactive prostaglandin-E in cerebrospinal-fluid of patients with schizophrenia. Lancet. (1980) 1:16–8. doi: 10.1016/s0140-6736(80)90553-x
125. Michetti, F, Corvino, V, Geloso, MC, Lattanzi, W, Bernardini, C, Serpero, L, et al. The S100B protein in biological fluids: more than a lifelong biomarker of brain distress. J Neurochem. (2012) 120:644–9. doi: 10.1111/j.1471-4159.2011.07612.x
126. Thelin, EP, Nelson, DW, and Bellander, B-M. A review of the clinical utility of serum S100B protein levels in the assessment of traumatic brain injury. Acta Neurochir. (2017) 159:209–5. doi: 10.1007/s00701-016-3046-3
127. Schumberg, K, Polyakova, M, Steiner, J, and Schroeter, ML. Serum S100B is related to illness duration and clinical symptoms in schizophrenia - a Meta-regression analysis. Front Cell Neurosci. (2016) 10:11. doi: 10.3389/fncel.2016.00046
128. Körschenhausen, DA, Hampel, HJ, Ackenheil, M, Penning, R, and Müller, N. Fibrin degradation products in post mortem brain tissue of schizophrenics: a possible marker for underlying inflammatory processes. Schizophr Res. (1996) 19:103–9. doi: 10.1016/0920-9964(95)00073-9
129. Misiak, B, Łoniewski, I, Marlicz, W, Frydecka, D, Szulc, A, Rudzki, L, et al. The HPA axis dysregulation in severe mental illness: can we shift the blame to gut microbiota? Prog Neuro-Psychopharmacol Biol Psychiatry. (2020) 102:109951. doi: 10.1016/j.pnpbp.2020.109951
130. Severance, EG, Prandovszky, E, Castiglione, J, and Yolken, RH. Gastroenterology issues in schizophrenia: why the gut matters. Curr Psychiatry Rep. (2015) 17:27. doi: 10.1007/s11920-015-0574-0
131. Lehner, C, Gehwolf, R, Tempfer, H, Krizbai, I, Hennig, B, Bauer, HC, et al. Oxidative stress and blood-brain barrier dysfunction under particular consideration of matrix metalloproteinases. Antioxid Redox Signal. (2011) 15:1305–23. doi: 10.1089/ars.2011.3923
132. Vafadari, B, Salamian, A, and Kaczmarek, L. MMP-9 in translation: from molecule to brain physiology, pathology, and therapy. J Neurochem. (2016) 139:91–4. doi: 10.1111/jnc.13415
133. Rybakowski, JK, Skibinska, M, Kapelski, P, Kaczmarek, L, and Hauser, J. Functional polymorphism of the matrix metalloproteinase-9 (MMP-9) gene in schizophrenia. Schizophr Res. (2009) 109:90–3. doi: 10.1016/j.schres.2009.02.005
134. Morrow, GB, Whyte, CS, and Mutch, NJ. A serpin with a finger in many PAIs: PAI-1's central function in Thromboinflammation and cardiovascular disease. Front Cardiovasc Med. (2021) 8:653655. doi: 10.3389/fcvm.2021.653655
135. Iadecola, C. The neurovascular unit coming of age: a journey through neurovascular coupling in health and disease. Neuron. (2017) 96:17–42. doi: 10.1016/j.neuron.2017.07.030
136. Cantarella, G, Lempereur, L, Presta, M, Ribatti, D, Lombardo, G, Lazarovici, P, et al. Nerve growth factor-endothelial cell interaction leads to angiogenesis in vitro and in vivo. FASEB J. (2002) 16:1307–9. doi: 10.1096/fj.01-1000fje
137. Calzà, L, Giardino, L, Giuliani, A, Aloe, L, and Levi-Montalcini, R. Nerve growth factor control of neuronal expression of angiogenetic and vasoactive factors. Proc Natl Acad Sci. (2001) 98:4160–5. doi: 10.1073/pnas.051626998
138. Aloe, L, Rocco, ML, Balzamino, BO, and Micera, A. Nerve growth factor: a focus on neuroscience and therapy. Curr Neuropharmacol. (2015) 13:294–3. doi: 10.2174/1570159X13666150403231920
139. Suzuki, Y, Nagai, N, Yamakawa, K, Muranaka, Y, Hokamura, K, and Umemura, K. Recombinant tissue-type plasminogen activator transiently enhances blood–brain barrier permeability during cerebral ischemia through vascular endothelial growth factor-mediated endothelial endocytosis in mice. J Cereb Blood Flow Metab. (2015) 35:2021–31. doi: 10.1038/jcbfm.2015.167
140. Huang, G, Osorio, D, Guan, J, Ji, G, and Cai, JJ. Overdispersed gene expression in schizophrenia. NPJ Schizophr. (2020) 6:9. doi: 10.1038/s41537-020-0097-5
141. Qin, XY, Wu, HT, Cao, C, Loh, YP, and Cheng, Y. A meta-analysis of peripheral blood nerve growth factor levels in patients with schizophrenia. Mol Psychiatry. (2017) 22:1306–12. doi: 10.1038/mp.2016.235
142. Edvinsson, L, Ekman, R, Jansen, I, Ottosson, A, and Uddman, R. Peptide-containing nerve fibers in human cerebral arteries: immunocytochemistry, radioimmunoassay, and in vitro pharmacology. Ann Neurol. (1987) 21:431–7. doi: 10.1002/ana.410210503
143. Cauli, B, Tong, X-K, Rancillac, A, Serluca, N, Lambolez, B, Rossier, J, et al. Cortical GABA interneurons in neurovascular coupling: relays for subcortical vasoactive pathways. J Neurosci. (2004) 24:8940–9. doi: 10.1523/JNEUROSCI.3065-04.2004
144. Yaksh, TL, Wang, J-Y, Go, V, and Harty, GJ. Cortical vasodilatation produced by vasoactive intestinal polypeptide (VIP) and by physiological stimuli in the cat. J Cereb Blood Flow Metab. (1987) 7:315–6. doi: 10.1038/jcbfm.1987.69
145. Attwell, D, Buchan, AM, Charpak, S, Lauritzen, M, Macvicar, BA, and Newman, EA. Glial and neuronal control of brain blood flow. Nature. (2010) 468:232–3. doi: 10.1038/nature09613
146. Benarroch, EE. Neuropeptide Y: its multiple effects in the CNS and potential clinical significance. Neurology. (2009) 72:1016–20. doi: 10.1212/01.wnl.0000345258.18071.54
147. Konradi, C, Yang, CK, Zimmerman, EI, Lohmann, KM, Gresch, P, Pantazopoulos, H, et al. Hippocampal interneurons are abnormal in schizophrenia. Schizophr Res. (2011) 131:165–3. doi: 10.1016/j.schres.2011.06.007
148. Fung, SJ, Webster, MJ, Sivagnanasundaram, S, Duncan, C, Elashoff, M, and Weickert, CS. Expression of interneuron markers in the dorsolateral prefrontal cortex of the developing human and in schizophrenia. Am J Psychiatr. (2010) 167:1479–88. doi: 10.1176/appi.ajp.2010.09060784
149. Kuromitsu, J, Yokoi, A, Kawai, T, Nagasu, T, Aizawa, T, Haga, S, et al. Reduced neuropeptide Y mRNA levels in the frontal cortex of people with schizophrenia and bipolar disorder. Gene Expr Patterns. (2001) 1:17–21. doi: 10.1016/S1567-133X(01)00003-5
Keywords: psychotic disorders, vascular dysfunction, blood–brain barrier, neurovascular unit, meta-analysis
Citation: Li X, Hu S and Liu P (2023) Vascular-related biomarkers in psychosis: a systematic review and meta-analysis. Front. Psychiatry. 14:1241422. doi: 10.3389/fpsyt.2023.1241422
Edited by:
Hiroyoshi Takeuchi, Keio University, JapanReviewed by:
Alfredo Bellon, Penn State Milton S. Hershey Medical Center, United StatesMelanie Foecking, Royal College of Surgeons in Ireland, Ireland
Copyright © 2023 Li, Hu and Liu. This is an open-access article distributed under the terms of the Creative Commons Attribution License (CC BY). The use, distribution or reproduction in other forums is permitted, provided the original author(s) and the copyright owner(s) are credited and that the original publication in this journal is cited, in accordance with accepted academic practice. No use, distribution or reproduction is permitted which does not comply with these terms.
*Correspondence: Pozi Liu, bGl1cG96aUBtYWlsLnRzaW5naHVhLmVkdS5jbg==