- 1Institut National de la Santé et de la Recherche Médicale (INSERM) Research Unit 1299 “Trajectoires développementales en psychiatrie”, École Normale Supérieure Paris-Saclay, Université Paris-Saclay, Centre National de la Recherche Scientifique (CNRS) 9010, Centre Borelli, Gif-sur-Yvette, France
- 2Department of Psychiatry and Addictology, Assistance Publique – Hôpitaux de Paris, Paul-Brousse Hospital, Villejuif, France
- 3Psychiatry-Comorbidities-Addictions Research Unit (PSYCOMADD), Paris-Saclay University, Gif-sur-Yvette, France
- 4Department of Psychiatry, Établissement Public de Santé (EPS) Barthélemy Durand, Etampes, France
- 5Institut National de la Santé et de la Recherche Médicale Research Unit 1018, Centre de Recherche en Epidémiologie et Santé des Populations (CESP), Paris, France
- 6Paris Sciences & Lettres (PSL) Research University-École Pratique des Hautes Études (EPHE), Paris, France
- 7Institut de Neurosciences Cognitives et Intégratives d'Aquitaine (INCIA), Centre National de la Recherche Scientifique (CNRS), Unité Mixte de Recherche (UMR) 5287, University of Bordeaux, Bordeaux, France
Background: Whether alteration in regional brain volumes can be detected in Type A alcoholics both at baseline and after a long follow-up remains to be confirmed. Therefore, we examined volume alterations at baseline, and longitudinal changes in a small follow-up subsample.
Methods: In total of 26 patients and 24 healthy controls were assessed at baseline using magnetic resonance imaging and voxel-based morphometry, among which 17 patients and 6 controls were re-evaluated 7 years later. At baseline, regional cerebral volumes of patients were compared to controls. At follow-up, three groups were compared: abstainers (n = 11, more than 2 years of abstinence), relapsers (n = 6, <2 years of abstinence), and controls (n = 6).
Results: The cross-sectional analyses detected, at both times, higher caudate nuclei volumes bilaterally in relapsers compared to abstainers. In abstainers, the longitudinal analysis indicated recovery of normal gray matter volumes in the middle and inferior frontal gyrus, and in the middle cingulate, while white matter volumes recovery was detected in the corpus callosum and in anterior and superior white matter specific regions.
Conclusions: Overall, the present investigation revealed larger caudate nuclei in the relapser AUD patient group both at baseline and at follow-up in the cross-sectional analyses. This finding suggest that a higher caudate volume could be a candidate risk factor of relapse. In patients with specific type A alcohol-dependence, we showed that long-term recovery in fronto-striato-limbic GM and WM volumes occurs during long-term abstinence. These results support the crucial role of frontal circuitry in AUD.
1. Introduction
Alcohol use disorder (AUD) causes extensive cortical and subcortical Gray Matter (GM) and White Matter (WM) brain damage (1–3), characterized by lower regional volumes. There might be a change in key-brain regions modulated by treatments available for AUD, as it is a multidimensional disorder which includes several subtypes with different neurobiological underpinnings (4, 5).
Previous studies have not exclusively included individuals without neurological complications (i.e., type A alcohol-dependent individuals). There are scarce studies examining AUD's patients with Type A alcohol-dependence, as designated by Chanraud et al. (6) with no neurological, somatic or psychiatric complications, and for whom the onset of dependence occurred late in life (6–8). Compared to 24 controls, Chanraud et al. (6) showed decreases in gray matter volumes that were detected bilaterally in 26 Type A alcohol-dependent individuals in the dorsolateral frontal cortex (up to 20% lower), and in the temporal cortex, insula, thalamus, and cerebellum. Decreases in white matter volumes were widespread, reaching 10% in the corpus callosum (6). Fein et al. analyzed 24 young to middle-aged treatment-naïve Type A alcohol-dependent individuals who showed reduced whole brain, prefrontal, and parietal cortical gray matter volumes compared to 17 controls. These structural brain changes were negatively associated with age and lifetime duration of alcohol use, which were highly intricated. Temporal cortex and white matter did not differ between the two groups (7). Finally, Pfefferbaum et al. showed that 16 Type A alcohol-dependent patients had a cortical gray matter loss over time in the prefrontal cortex and the anterior superior temporal lobe and enlarged ventricles, compared to 28 controls who drank low amounts of alcohol (8).
Several longitudinal MRI studies investigated the short-term reversibility (up to 24 months) of these structural alterations. They compared AUDs vs. controls as well as abstainers vs. relapsers after a period of abstinence ranging from 1 to 24 months (9–22) (see for review Supplementary Table 1). In patients maintaining abstinence for over 3 months, regional GM volumes partially recovered in the cingulate cortex, the orbito-frontal cortex and the insula (15). In patients maintaining abstinence over 8 months a regional GM volumes recovery was shown in the frontal and parietal regions after 1 month (14, 16).
Furthermore, there are scarce reports on structural predictors of relapse between relapsers and abstainers. These studies highlight initial hypovolumetry, in the fronto-ponto-cerebellar and mesocorticolimbic regions (12, 14), in the bilateral frontal cortex (21), the frontal cortical thickness (16), as well as in the right orbito-frontal cortex, medial prefrontal cortex, and right anterior cingulate cortex regions (17), the amygdala (23) and the striatum and the thalamus (24).
However, the follow-up duration in all these longitudinal studies varied from 1 month to 2 years.
Thus, to our knowledge, no studies have investigated the brain structure damage in AUD between abstainers and relapsers after more than 24 months.
Besides most of the longitudinal studies conducted evaluated AUD patients with somatic and psychiatric comorbidities (12–16, 20, 21, 25) (see Supplementary Table 1). Few studies (11, 15, 17, 26) have explored uncomplicated AUD men and women, i.e., Type A, as designated by Babor et al. (27).
Therefore, our study aimed to detect whether there are brain damage differences beyond 24 months between relapsers and abstainers, particularly in Type A AUD patients, and identify regional volumes as potential predictors of outcome at baseline, or as predictors of reversibility at follow-up.
Therefore, we first compared WM and GM volumetry between all healthy controls and AUD patients at baseline.
The groups were formed according to the maintenance or not of abstinence at 7 years of follow-up; afterwards, at follow-up, we compared relapsers, abstainers, and controls groups.
Secondly, we aimed to investigate the long-term changes in regional volumes at follow-up, by comparing cross-sectionally and longitudinally the followed-up subgroups of abstainers, relapsers, and controls.
In line with the literature, we hypothesized that long-term abstinence would lead to, at least partial, recovery of the prefrontal cortex, cingulate cortex, and WM volume reductions.
2. Materials and methods
2.1. Participants
At baseline (BL) twenty-nine AUD patients detoxified for at least 3 weeks (mean age 47.4 ± 7.7 years), and meeting DSM-IV criteria for alcohol-dependence were recruited from consecutive admission to addiction disorders wards of addiction departments at Paul Brousse and Emile Roux Hospitals (AP-HP).
Twenty-nine healthy controls with neither past nor current substance abuse, matched to AUD patients for age, sex, Body Mass Index (BMI) and education were recruited from the neighboring community. Body Mass Index (BMI; kg/m2) was calculated as the ratio of patient collected data on weight and height and is defined as the weight divided by the square of the body height. Because some studies have reported sex-differences regarding alcohol-dependence (28), we chose to include only men in our study, in order to limit the impact of gender and heterogeneity in our limited sample size.
At baseline (BL), twenty-nine patients and twenty-nine healthy controls were recruited.
All patients and controls were males, Caucasian and right-handed as determined by the Annett Hand Preference Questionnaire (29).
Finally, due to motion artifacts and other technical difficulties, 3 AUD and 5 controls were excluded. Thus, 26 AUD and 24 controls were finally included in analyses at baseline [see Chanraud et al. (6) and the flow chart in Supplementary Figure 1].
The inclusion criteria for the control group were a consumption of less than two standard units of alcohol per week (20 g) during the previous year and a score of ≤ 5 on the Alcohol Use Disorders Identification Test (AUDIT) (30).
Exclusion criteria for both groups included being under 25 or over 65 years of age, in order to avoid age-related increased brain vulnerability to alcohol abuse (31–33). Other exclusion criteria were left- handedness, non-fluency in French, history of substance abuse or dependence other than caffeine and tobacco, sedative treatment for at least 1 week, axis I disorder (particularly mood and/or anxiety disorders, psychosis), high scores (>5) on the Hamilton Anxiety and Hamilton Depression Rating Scale (HARS and HDRS) (34, 35), malnutrition, hepatic pathology revealed by a ratio of liver enzymes aspartate aminotransferase/alanine aminotransferase (AST/ALT) greater than 2 (36), neurological and somatic diseases including a history of head injury with loss of consciousness, stroke, or other major brain abnormalities observed on MRI scans.
The characteristics of the participants' groups are provided in Table 1. The Bicêtre Ethics Committee (CPP-IDF 7) approved the study protocol. All participants received verbal and written protocol information, signed a consent form and received monetary compensation for their participation.
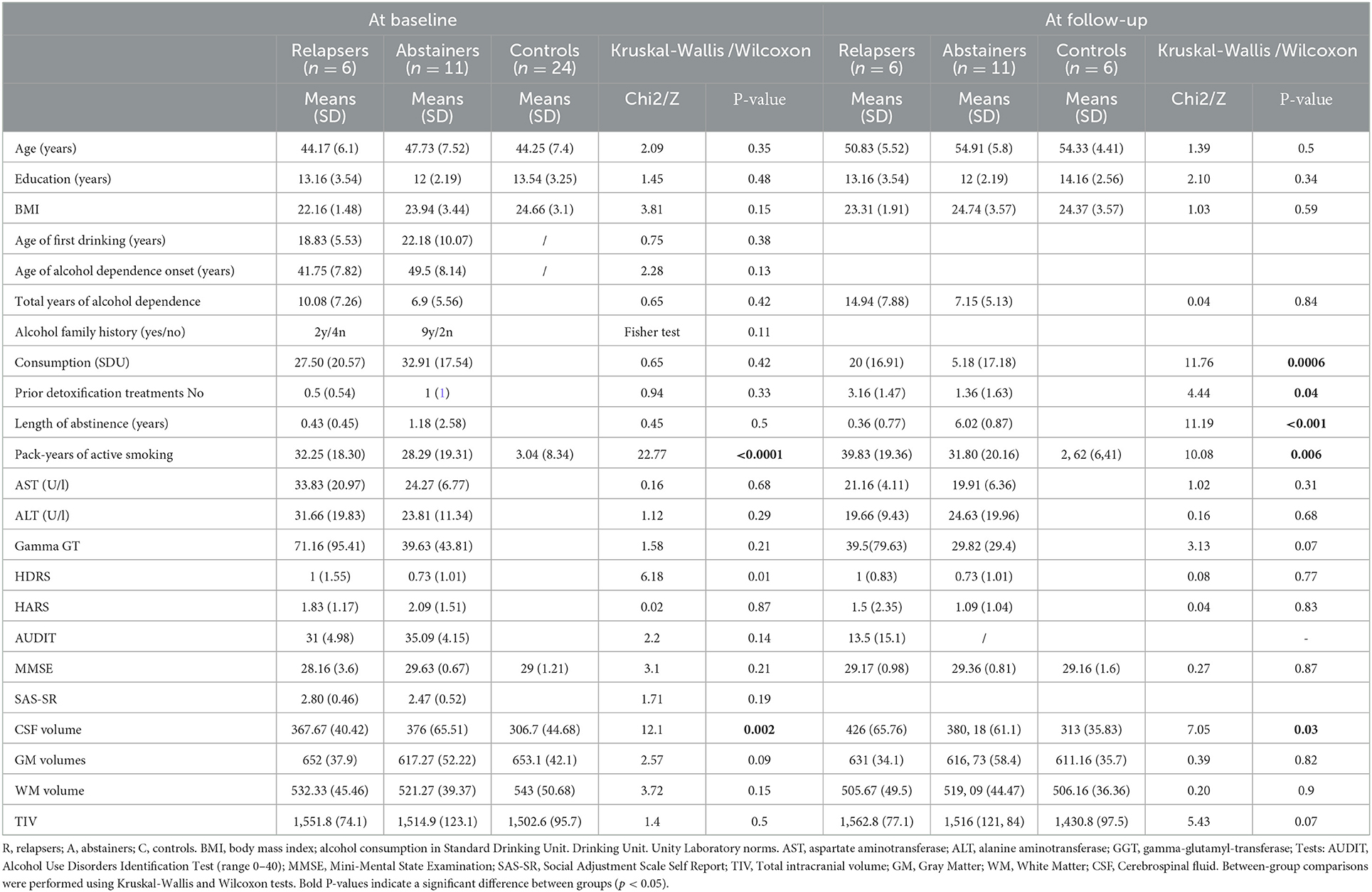
Table 1. Characteristics of the three participant groups and whole brain volumes at baseline and follow-up.
2.2. Clinical assessment
Trained psychiatrists (CM, EA, HJA, and JLM) performed a clinical evaluation of all the participants, examined their medical records and biological data at BL and follow up (FU). The presence of an axis I disorder (particularly mood and/or anxiety disorders, psychosis) was evaluated by a clinical interview. Trained psychiatrists (CM, EA, HJA, and JLM) interviewed and clinically evaluated patients, as well as examined their medical records and biological data. The diagnosis was determined after the clinical interview, by consensus of at least two interviewers and according to DSM-IV criteria.
Alcohol-dependence was assessed using the AUDIT and nicotine dependence by the Fagerström test (FTND) (38). Social functioning was evaluated using the Social Adjustment Scale Self Report (SAS-SR) (39), a self-report questionnaire, that evaluates daily functioning, and includes questions on leisure and social activities, relationships, economic status, marital status, children and extended family. Intellectual deterioration was assessed by the Mini-Mental State Examination (MMSE) (40), intellectual efficiency was assessed by the Information Subtest of the Weschsler Adult Intelligence Scale III Third Revision (41), and anxiety and depression were assessed using the Hamilton Anxiety Rating Scale and Hamilton Depression Rating Scale respectively (34, 35).
Moreover, we asked participants to rate among their first- and second-degree family members, the number of problematic alcohol drinkers.
Biological blood tests were performed for all subjects on both BL and FU. On the day of testing, fasting blood samples were drawn to investigate the somatic complications of chronic alcoholism. The panel of tests included liver function tests: aspartate aminotransferase (AST), alanine aminotransferase (ALT), gamma-glutamyl-transferase (GGT), carbohydrate-deficient transferrin (CDT), bilirubin, hemoglobin, hematocrit and mean blood volume (MCV). Abstinence was ascertained by normal blood levels of carbohydrate-deficient transferrin (CDT), of gamma-glutamyl-transferase (GGT), and of mean blood volume (MCV).
At FU, the AUDIT and the alcohol consumption self-report since BL were used to retrospectively estimate the quantity and the frequency of their alcohol consumption. Participants were also asked to report the duration and number of relapses and related-detoxifications during the follow-up period (from 0 to 3 detoxifications). Furthermore, we verified these data by reviewing their medical records.
Sub-group's characteristics: sub-groups were formed according to patients self-reported alcohol consumption during the 2 years before follow-up evaluation and confirmed by medical reports and blood alcohol tests [normal blood levels of gamma-glutamyl-transferase (GGT), carbohydrate-deficient transferrin (CDT) and mean blood volume (MCV)].
Abstainers self-reported no alcohol consumption for at least 2 years at FU that was confirmed by available medical records and available laboratory indicators of alcohol consumption [e.g., gamma glutamyltransferase (GGT)], which were within normal limits at follow-up. Relapsers self-reported alcohol consumption in the 2 years before FU, and this was confirmed by available medical records.
The two-year threshold is in line with the literature on long-term abstinent alcoholics, which commonly uses a duration of abstinence of more than 18 months (18, 37, 42) or more than 2 years (11, 43, 44).
All controls remained abstinent: three did not drink at all, one drank one drink per week, one consumed 2 drinks by month and one consumed 2 drinks by year. At 6-year follow-up, all AUD patients and controls were called by phone. The average duration between the baseline and follow-up MRI acquisitions was 77 ± 5 months and ranged from 68 to 85 months. Among the initial twenty-six AUD patients, eighteen were still followed in detoxification centers. Of those lost to follow up, two died between BL and FU. An additional research subject was excluded because of an incidental leukemia diagnosis. Five patients were unreachable. Among the twenty-four control subjects, seventeen were unreachable. At FU, a technical problem was encountered during the MRI acquisition of one patient and one control subject.
Overall, 17 AUD (11 abstainers and 6 relapsers) patients and 6 controls were included in the final analyses.
2.3. Imaging methods
2.3.1. Magnetic resonance imaging acquisition
MRI data was acquired at BL and at FU on the same Signa 1.5 Tesla Whole Body system from General Electrics (Milwaukee, Wisconsin) at SHFJ (CEA, Orsay, France), with a standard 3D T1-weighted inversion recovery fast-spoiled gradient-recalled sequence with identical parameters: axial orientation, matrix = 256 × 192 interpolated to 256 × 256, 124 slice locations, 0.9375 × 0.9375 mm2 in-plane resolution, slice thickness = 1.3 mm, TE = 2 ms, TR = 10 ms, TI = 600 ms, flip angle = 10°, and read bandwidth = 12.5 kHz.
2.3.2. Magnetic resonance image preprocessing
Spatial normalization and tissue segmentation in gray and white matter probability maps were performed for all images using the Cat12 toolbox (http://www.neuro.uni-jena.de/cat/), in SPM12 (Statistical Parametric Mapping, https://www.fil.ion.ucl.ac.uk/spm/) implemented in Matlab (https://fr.mathworks.com/help/matlab/ref/edit.html). Gray and white matter segmented images were modulated to compensate for deformations and finally smoothed with a 8-mm FWHM Gaussian filter. Total intracranial volume (TIV) was also estimated using the Cat12 toolbox. Visual quality control was performed for each raw image by one author (RM) and verified by another (CM). Cat12 quality rating was examined, and all preprocessed images were used.
Thus, participants underwent brain scanning both at BL and FU, using the same scanner, head coil, and volumetric MRI sequence parameters.
2.4. Statistical analyses
2.4.1. Sociodemographic, clinical, and biological analyses
At BL and at FU, socio demographic, clinical and biological data, brain volumes of the three groups (relapsers, abstainers and controls) were compared, with the Jmp 14 software (https://www.jmp.com/fr_fr/home.html) using non-parametric tests such as Wilcoxon and Kruskal-Wallis.
All neuroimaging analyses were performed with SPM12, in whole brain. All scans were free from abnormalities.
2.4.2. Cross-sectional voxel-based morphometry analyses
Cross-sectional voxel-based morphometry (VBM) analyses were performed on whole-brain GM and WM images (45) at both BL and FU.
A one-way ANOVA model in SPM was used, with group (relapsers, abstainers, controls) as the between-subject factor and age, years of education and TIV as confounding covariates.
At baseline, analyses were performed with all included controls (n = 24), and all AUD patients. Thereafter, we compared the baseline groups from participants included at both times (abstainers n = 11, relapsers n = 6, controls n = 6). At FU, analyses were conducted with all subjects included at both times (abstainers n = 11, relapsers n = 6, controls n = 6).
Statistical height threshold was set at p < 0.001 uncorrected, and extent threshold at p < 0.05 uncorrected (k = 300 voxels) (46). The get_totals SPM function was used to extract volumes from all significant clusters at BL (http://www0.cs.ucl.ac.uk/staff/g.ridgway/vbm/get_totals.m.).
2.4.3. Longitudinal analyses
Longitudinal analyses were conducted using a flexible factorial design (one-way ANOVA for repeated measures) with time (BL and FU) as within-subject factor and group (relapsers, abstainers, controls) as between-subject factor.
As tobacco consumption may have confounding effects (20, 47), we conducted supplementary analyses with the number of cigarette packs smoked per year, entered as covariate.
In the longitudinal analyses, the height threshold was set at p < 0.001 uncorrected and the extent threshold at p < 0.05 uncorrected (respectively k = 150 voxels and k = 210 voxels for the GM and the WM analysis).
We used the AAL atlas (48) within the xjview toolbox (https://www.alivelearn.net/xjview/) and the JHU toolbox in MRIcron software to locate regions in all VBM analyses (49).
3. Results
3.1. Participant's characteristics
The three participant groups did not significantly differ in most socio-demographic data, biological variables, rating scales scores and whole brain tissues volumes except for CSF at BL and at FU, as described in Table 1. The duration of alcohol abstinence (p < 0.0001), alcohol consumption (p = 0.0006) and the number of withdrawals (p = 0.04) differed between the two subgroups of AUDs (abstainers vs. relapsers) at FU whereas no difference was found at BL. In addition, AUD patients smoked more than controls at both time points (BL: RvC Z = 4.08; p < 0.0001; AvC Z = 3.23; p < 0.0001; RvA Z = 0.15; p = 0.88; FU: RvC Z = 2.9; p = 0.004; AvC Z=2.65; p = 0.008; RvA Z=0.45; p = 0.65). All patients had good social functioning based on the SAS-SR scale: SAS-SR scale AUD subgroups scores were not different at BL (Table 1).
Thus, AUD patients were split into two groups: relapsers, who had been abstinent for <2 years (n = 6; mean 0.36 ± 0.77 years), and abstainers, whose duration of abstinence was >2 years (n = 11; 6.02 ± 0.87 years).
It is worth noting that no significant difference in education level, age, or BMI was found between the unreachable (n = 6) and the reachable (n = 17) controls (Supplementary Table 2).
3.2. MRI results
3.2.1. Cross-sectional GM analyses at baseline
At BL, the comparison between controls and all AUD patients (controls n = 24; AUDs n = 17) revealed significant regional gray matter volume reductions in AUDs in bilateral hippocampus and para-hippocampus, left amygdala, bilateral medial frontal, right precentral, left temporal middle gyri and right thalamus (Supplementary Table 3). No larger GM volumes were found in AUD patients compared to controls.
The cross-sectional BL differences between subgroups (controls n = 24; relapsers n = 6; abstainers n = 11) with voxel-wise two-sample t-tests showed that the relapsers had a higher volume than the abstainers in the head of the caudate nucleus (CN) bilaterally (PFWE-corrected <0.05 at cluster and peak levels) (Table 2, Figure 1A). Individual plots of the bilateral heads of the caudate nuclei cluster volumes (in cm3), at baseline, in controls, relapsers and abstainers are represented in Figure 1B to illustrate this result. No significant difference was found among relapsers < abstainer's contrast.
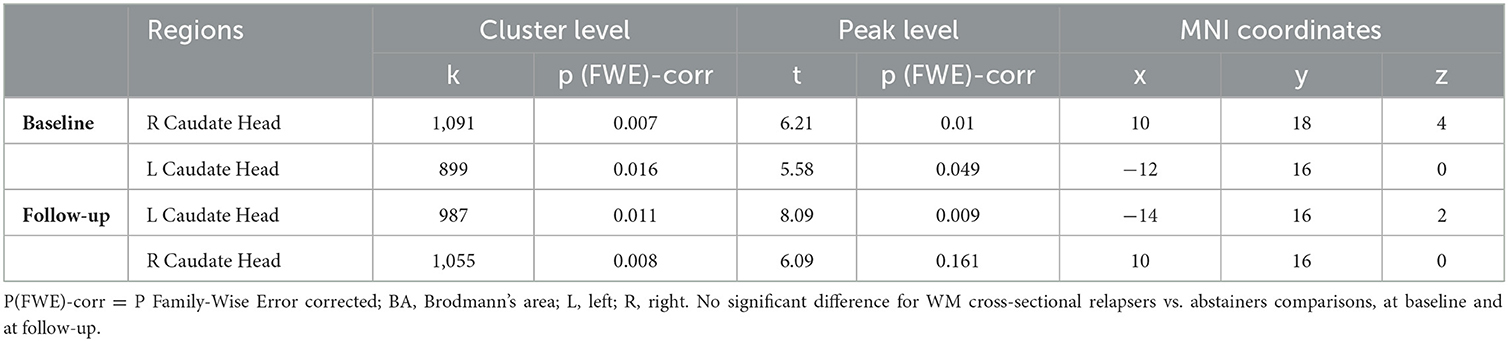
Table 2. Gray Matter (GM) cross-sectional analyses: comparisons between relapsers and abstainers: Relapsers > Abstainers.
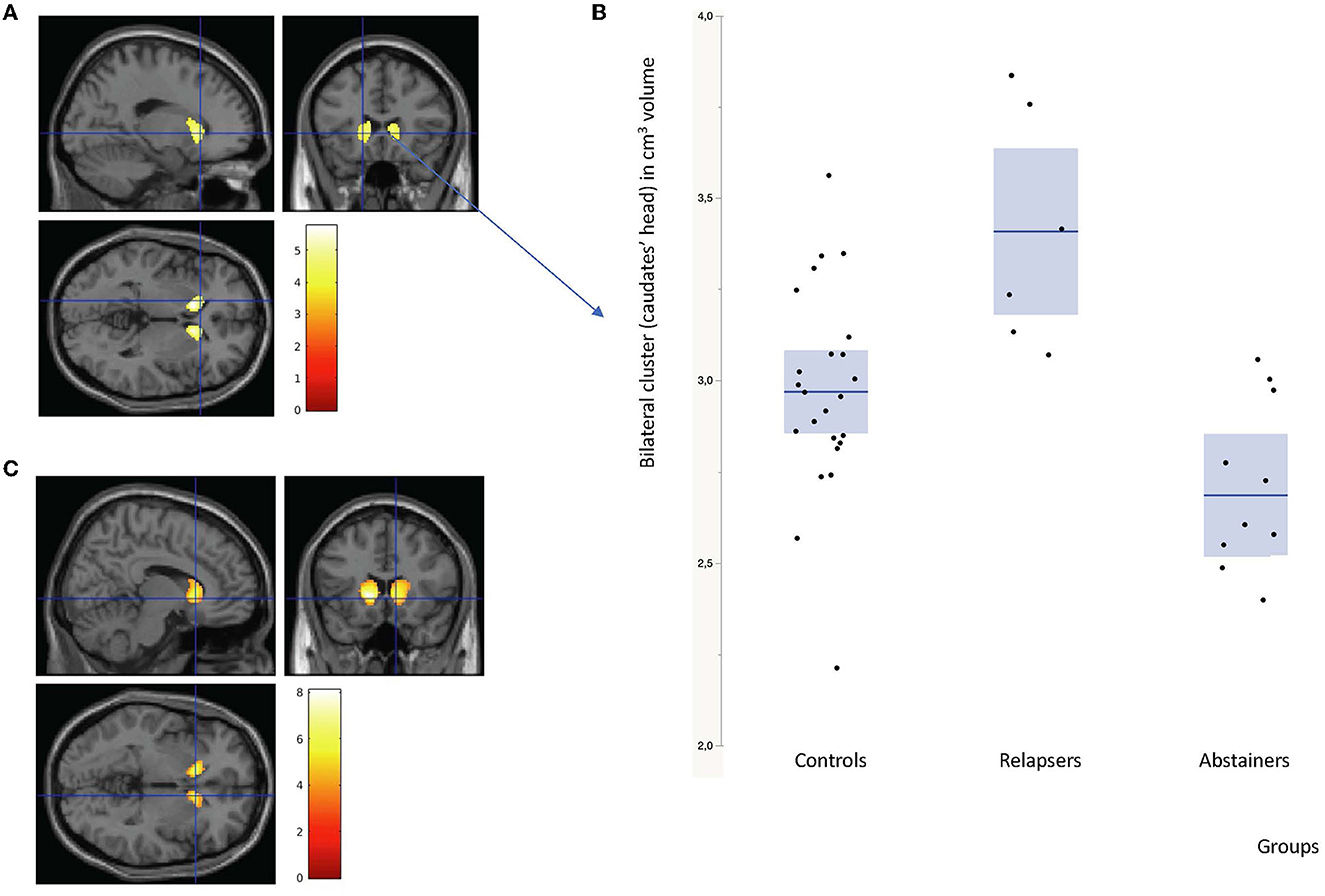
Figure 1. (A) Gray matter volumes: cross-sectional bilateral caudate nuclei increases in relapsers compared to abstainers Alcohol Use Disorders (AUD) patients, at baseline (height and extent threshold p < 0.05 FWE corrected). (B) Individual plots: bilateral heads of the caudate nuclei cluster volumes (in cm3) at baseline in controls on the left, relapsers on the middle and abstainers on the right. (C) Idem at follow-up (height threshold p < 0.001 uncorrected and extent threshold p < 0.05 FWE corrected).
With respect to controls, the relapsers only had a higher volume in the right caudate head (p < 0.001 uncorrected at peak level) (Supplementary Table 3).
Compared to controls, the abstainers had lower volumes in the right precentral gyrus, left hippocampus, left medial frontal gyrus, right para-hippocampus and bilateral thalamus (p < 0, 001 uncorrected at cluster and peak level) (Supplementary Table 3).
3.2.2. Cross-sectional GM analyses at follow-up
At FU, the comparison between the reassessed controls and all AUD patients (AUDs n = 17; controls n = 6) revealed no significant regional GM volume reduction in all AUDs vs. controls contrasts.
The cross-sectional GM differences between subgroups (relapsers n = 6, abstainers n = 11, controls n = 6) with voxel-wise two-sample t-tests still showed higher volumes in the head of the CN bilaterally in relapsers compared to abstainers (see Table 2, Figure 1C). No significant difference was found in the relapsers < abstainers' comparison.
No significant GM volume difference was found between controls and both patient sub-groups (Supplementary Table 3).
3.2.3. Cross-sectional WM analyses at baseline
At BL, the comparison between controls and all AUD patients (controls n = 24; AUDs n = 17) revealed widespread reductions of the regional WM volume in patients in the midbrain, left cerebral peduncle, right retrolenticular part of the internal capsule, superior and inferior longitudinal and inferior fronto-occipital fasciculi, right superior corona radiata and left corpus callosum. Reductions were also detected in the bilateral parietal and left middle occipital, superior temporal, cingulate, middle frontal, left medial and left superior frontal (Supplementary Table 3). No significant WM volume reduction was found in controls compared to AUD patients.
The cross-sectional BL comparisons between subgroups for volumes of WM (controls n = 24; relapsers n = 6; abstainers n = 11) showed no difference between relapsers and abstainers. However, compared to controls, relapsers had significant WM reduction adjacent to the bilateral thalamus, lingual, inferior frontal, and inferior parietal regions, as well as in the left cerebral peduncle, midbrain, and right superior longitudinal fasciculus (Supplementary Table 3). Also, compared to controls, abstainers had lower WM volume in the superior longitudinal fasciculus, left superior corona radiata, left anterior limb of internal capsule (ALIC), right external capsule, sagittal stratum and regions adjacent to the left putamen and bilateral frontal regions. No WM volume reduction was found in controls compared to abstainers (Supplementary Table 3).
3.2.4. Cross-sectional WM analyses at follow-up
At FU, the comparison between the reachable controls and all AUD patients (controls n = 6; AUDs n = 17) revealed no significant regional WM volume differences, and none were found between subgroups (Supplementary Table 3).
The cross-sectional analysis results are maintained after control by tobacco consumption (pack/year).
3.2.5. CSF at both BL and FU
AUD patients had significantly more CSF than controls at both BL and FU (BL: RvC Z = 2.70; p = 0.007; AvC Z = 2.79; p = 0.005; RvA Z = 0.45; p = 0.65; FU: RvC Z = 2.80; p = 0.005; AvC Z = 1.66; p < 0.1; RvA Z = 0.75; p = 0.45).
3.2.6. Longitudinal gray matter analysis
For relapsers vs. abstainers, significant time (BL and FU) x group interactions were found in the frontal cortex bilaterally: middle frontal gyrus (BA 9, BA 10, and BA 46), inferior frontal gyrus, pars opercularis (BA 44), and the left precuneus. Other significant clusters include the bilateral middle cingulate (BA 24 and BA 32) (see Table 3, Figure 2A).
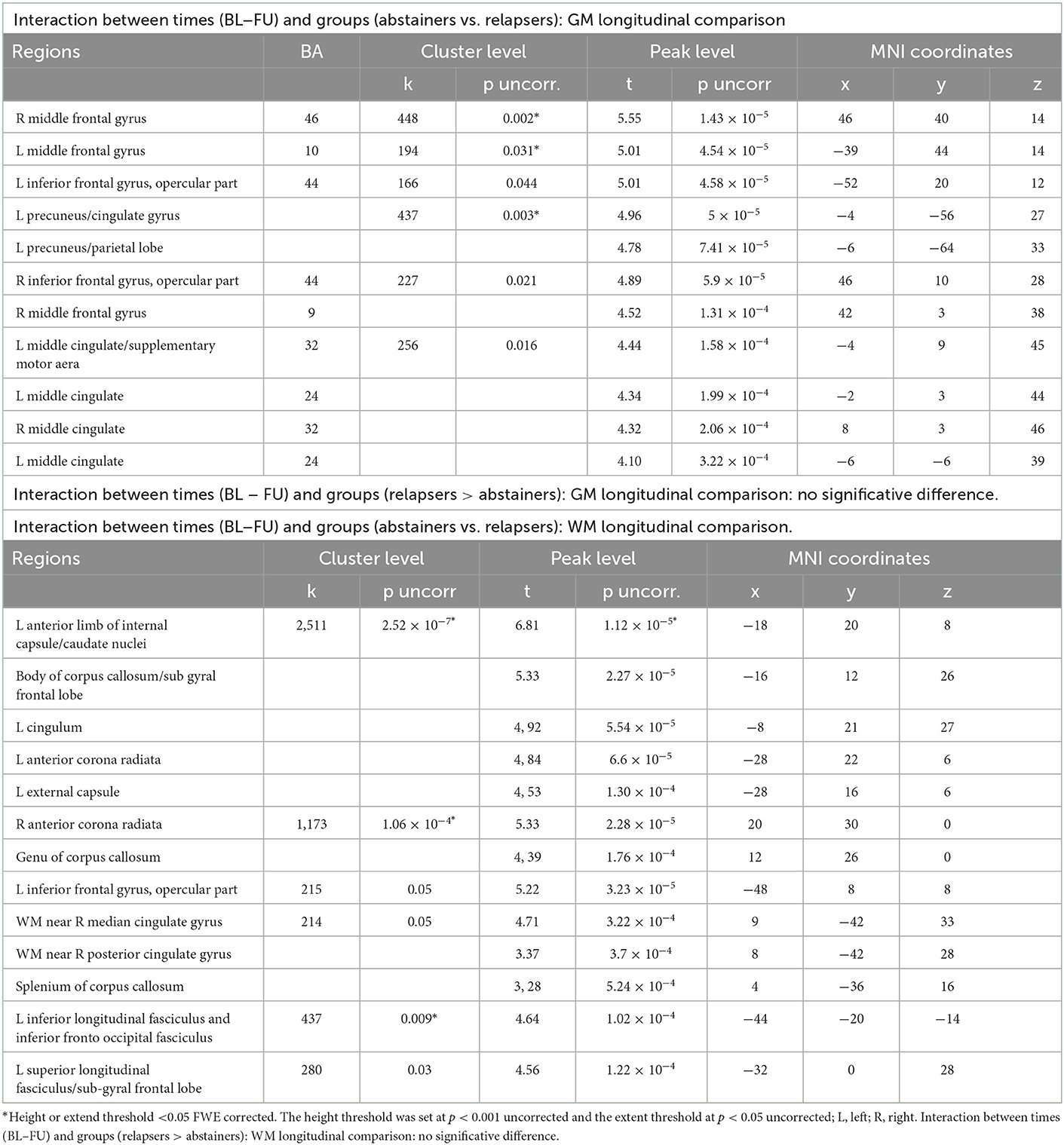
Table 3. Grey Matter (GM) and White Matter (WM) longitudinal analyses: comparisons between relapsers and abstainers.
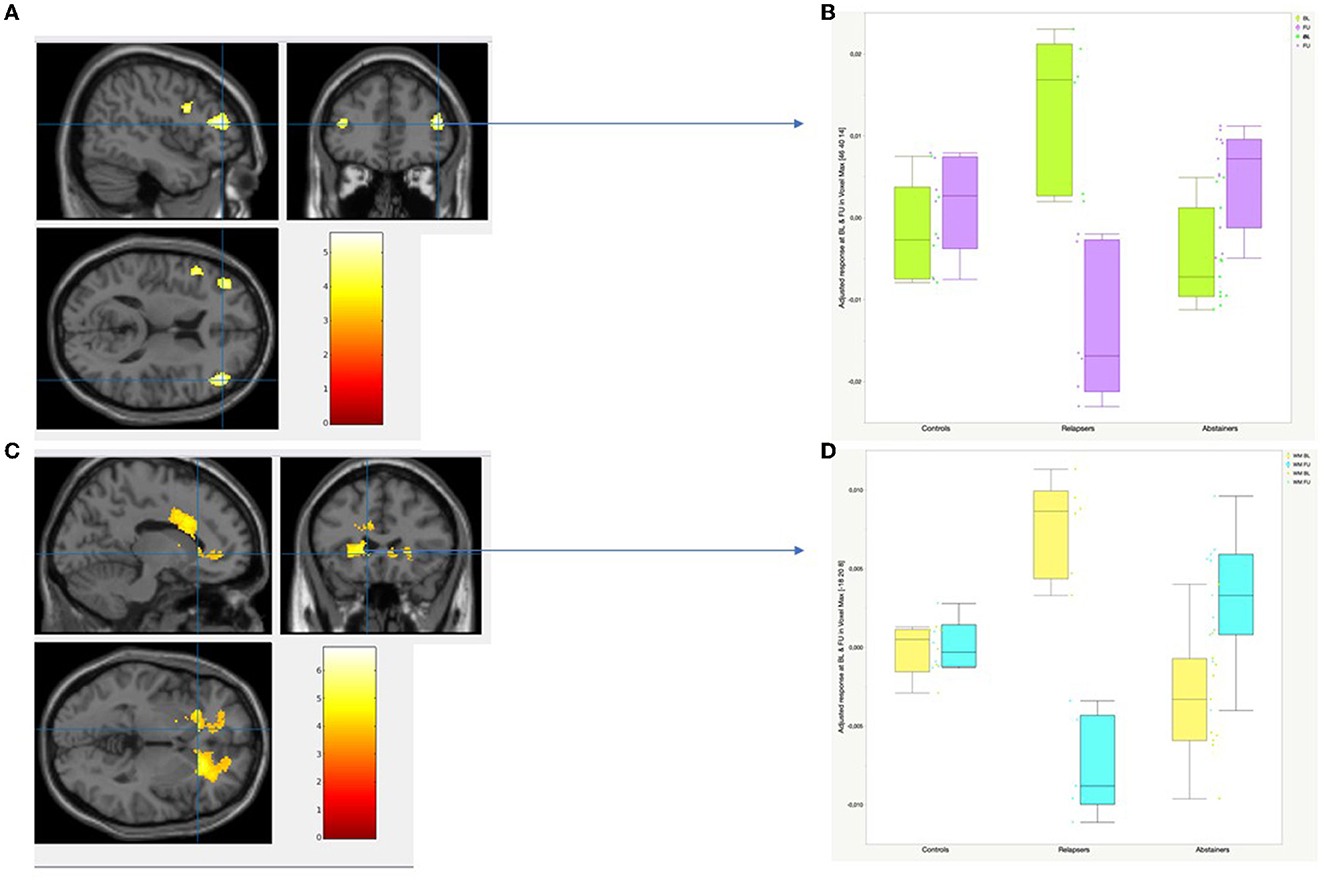
Figure 2. Longitudinal gray matter and white matter analysis: Time by Group Interaction. (A) 2D views of GM regions showing significant time x group interaction. The height threshold was set at p < 0.001 uncorrected and the extent threshold at p < 0.05 uncorrected. (B) Individual plots and adjusted voxel values at baseline (boxplots in green) and at follow-up (boxplots in purple) in control, relapser and abstainer groups at the highest peak-voxel detected by the Time by Group Interaction in GM (right middle frontal gyrus, BA 46: MNI coordinates: x = 46, y = 40, Z = 14). (C) 2D views of WM regions showing significant time x group interaction. The height threshold was set at p < 0.001 uncorrected and the extent threshold at p < 0.05 uncorrected. (D) Individual plots and adjusted voxel values at baseline (boxplots in yellow) and at follow- up (boxplots in blue) in control, relapser and abstainer groups at the highest peak-voxel detected by the Time by Group Interaction in WM (Anterior Limb of Internal Capsule: MNI coordinates: x = −18 y = 20, Z = 8).
GM volume decreased in relapsers over the course of 7 years in all listed regions, while abstainers displayed GM volume increase. An example is given for the middle frontal region in Figure 2B.
For controls vs. relapsers, significant time (BL and FU) x group interactions were found in the pars triangularis of the inferior frontal gyrus bilaterally. Post-hoc analyses indicated that relapsers had lost GM volume in this region while it had increased in controls over time.
For controls vs. abstainers, no significant time (BL, FU) x group interactions were found. Supplementary Table 4 reports all time (BL and FU) x group (relapsers, abstainers, and controls) GM volume interactions.
3.2.7. Longitudinal white matter analysis
For the relapsers vs. abstainers, significant time (BL, FU) x group interactions were found in all parts of the corpus callosum. The same interactions were found in left anterior limb of internal capsule, bilateral corona radiata, external capsule, in the regions adjacent to caudate nuclei and the cingulate gyrus (all cluster-level PFWE-corrected ≤ 0.05). Interactions were also found in the left inferior and superior longitudinal fasciculi, inferior fronto-occipital fasciculus, and regions adjacent to the inferior frontal gyrus, pars opercularis (see Table 3, Figure 2C).
For all listed regions, WM volume decreased in relapsers and increased in abstainers over the course of 7 years. An example is given for the left ALIC region in Figure 2D.
For the controls vs. relapsers, significant time (BL, FU) x group interactions were found in regions adjacent to the insula, as well as in the right external capsule, right anterior corona radiata and right ALIC (see Supplementary Table 4).
For the controls vs. abstainers, no significant time (BL, FU) x group interactions were found. The Supplementary Table 4 reports the time (baseline and follow-up) × group (relapsers, abstainers, and controls) WM volume interactions.
4. Discussion
Regional tissue volume was different during long-term (7-year) recovery in a Type A alcohol-dependent sample compared with a control group. Yet, the final numbers of participants in each group (primarily abstainers and relapsers and controls) were small and due to the small patient sample, the present findings have to be considered as exploratory. Future studies are therefore necessary to confirm these findings in larger groups. It is however noteworthy that the finding of larger caudate nuclei appears to dissociate specifically relapsers from abstainers both at BL and FU. This main finding is supported by other ones in the present study, which are in agreement with previous literature concerning shorter follow-ups (generally up to 2 years).
In line with the literature, at BL, AUD patients relative to healthy controls showed smaller gray matter volume in limbic structures (hippocampus, para-hippocampus, amygdala) as well as the medial frontal and temporal regions, the precentral gyrus, and thalamus (12, 18, 23, 47, 48). In this same comparison, a significant decrease in the volume of WM is extensive in the brainstem, in the cerebral peduncle, in the anterior regions (the right internal capsule, the superior, and anterior right corona radiata), in the cingulum middle bilaterally and the right inferior and superior longitudinal bundle, in the right fronto-occipital inferior bundle, and in the commissural fibers of the corpus callosum (genu). Volume reductions were also detected in the right superior temporal WM, the bilateral sub gyral and middle frontal WM, in the left median and superior frontal WM, the right parietal WM and in the left occipital WM (49–52). These decreases in volumes of both WM and GM correspond to the parallel increase in the volume of CSF (12, 48, 53, 54) and further confirm our results.
Both at BL and at FU, relapsers had larger heads of caudate nuclei (CN) than abstainers bilaterally.
Longitudinal analyses showed recovery of normal GM volumes in the bilateral middle and inferior frontal, left precuneus and the bilateral mid-cingulate after long-term abstinence. Findings pointed to potential recovery of WM volume in adjacent regions, as well as in commissural tracts, the corona radiata bilaterally, left ALIC, the external capsule and the left superior and left inferior longitudinal fasciculus and the inferior fronto occipital fasciculus.
4.1. Cross-sectional gray matter analysis: The caudate nuclei
The finding of larger heads of CN in relapsers compared to abstainers was bilateral and symmetrical at both BL and FU, indicative of its robustness. The longitudinal analysis did not detect any significant change in this region, confirming the stability over time of the larger CN volumes in relapsers. This suggests that the pre-existing CN volume difference at baseline might be associated with a risk of relapse and thus could be a candidate vulnerability factor. It is strikingly consistent with a recent IMAGEN consortium study reporting higher GM volume in bilateral CN at age 14, as a structural brain predictor of a larger increase in alcohol use scores over 5 years, between age 14 and 19 (55). While both studies used voxel-wise analyses methods over the whole-brain volume (Supplementary Figure 2), the present findings in AUD relapsers confirm the location of their CN findings. This is consistent with the hypothesis that larger CN may indirectly denote vulnerability to poor alcohol use outcome.
Several reports below are of note to support this suggestion.
For instance, enlarged CN volume was reported in binge drinkers (56), in cocaine dependence (57, 58) and in methamphetamine dependence (59, 60). Moreover, only a few studies in AUD patients report a longitudinal exploration of the CN volume, notably in Type A alcohol-dependent subjects, and their follow-up durations were much shorter, ranging from 3 weeks to 18 months. Among eleven longitudinal and cross-sectional studies comparing abstinent AUD patients vs. controls, seven did not explore the caudate nuclei volumes (8, 19, 61–65) three did not find any significant difference (24, 66, 67) and one reported a reduction in CN volume (68). Two previous studies comparing AUD patients abstinent for 6 years to controls, but without any longitudinal design, and did not find any difference in CN volume either (42, 69) (see Supplementary Table 1 for a review).
Among twelve longitudinal studies comparing abstainers vs. relapsers, one reported a tendency toward CN volume increase in abstainers (12) at 7-months follow- up, one noted CN volume heterogeneity (20), and five did not find any differences in CN volume between groups (13, 14, 18, 20, 21).
The remaining studies did not explore potential differences in CN volume (9, 11, 15, 17, 22, 26).
Critically, most of these studies used data from patients with comorbidity (addictions and mental health disorders) (see Supplementary Table 1). This variety of subjects contrasts with the homogeneity of our own AUD patient sample, which might account for the detection of higher CN volume in relapsers. The filter used to smooth Jacobian maps could contribute to a difficulty in accurate detection of brain matter volume differences (12). Moreover, we can note other differences in the methodology used in the only longitudinal report over 18-months in abstainers vs. relapsers, which did not find any difference in CN volume, which included the manual delineation of brain regions, with no voxel-based analysis, and a mixed-gender sample (70).
The caudate nucleus mediates higher cognitive functions, including the executive functions and cognitive control (71–73), and is highly connected with the prefrontal cortex (74).
Moreover, the CN is implicated in the reward system (75, 76). The dorsal striatum, including CN and putamen, has been strongly linked to the development and expression of habituation behaviors (72, 77, 78). A link was made between enlarged striatal volumes and higher dopamine synthesis capacity, with an increase in dopamine level in the dorsal striatum, including the caudate and putamen (79, 80). In an fMRI study, when presented with alcohol-associated stimuli, dependent AUD patients showed hyper-activation of the caudate nuclei (81).
These data and the present exploratory results may suggest that individuals who recruit more often or more strongly motivational or reward circuits have larger CNs and are more likely to feel alcohol craving and thus relapse. Replication of our findings in a larger sample could allow further confirmation of this potential risk factor for alcohol consumption relapse. Therefore, supplementary investigations are needed to test the hypothesis of an enlargement of the CN, as an appetitive region, and a risk factor of relapse through automatic behavior.
4.2. Cortical gray matter longitudinal analysis
In line with the literature and with our hypotheses, abstainers compared to relapsers showed an increase in GM volume in a number of frontal regions, including the bilateral middle (BA 9, BA 10, and BA 46) and inferior (BA 44) frontal cortex, left precuneus and bilateral mid-cingulate (BA 24, 32). Consistently, previous longitudinal comparisons among abstainers and relapsers mentioned similar results, with a frontal GM volume increase already detected after 4 weeks of abstinence (22). After 3 months (15), then 8 months of abstinence (12), GM volume recovery was reported in the cingulate cortex. After 12 months of abstinence, a GM volume increase was also detected in various frontal regions in abstainers, in the superior frontal gyrus and orbitofrontal cortex (14–16), middle frontal cortex (16), middle and anterior frontal cortices (12), anterior mesial and prefrontal cortices (9), dorsolateral frontal cortex (13), and inferior frontal cortex (26).
We can also note that the consistency of the present findings with the previous literature supports that such volumetric changes can be detected using longitudinal voxel- and pair- wise methods in small and homogeneous groups of abstainers and relapsers followed-up during a longer time.
Herein, no longitudinal difference was detected between abstainers and controls in GM volume. This may indicate a recovery of the cortical GM volume. Previous longitudinal studies comparing AUD patients and controls found smaller volumes in the medial frontal and lateral prefrontal cortices (66). After 7 months of abstinence, a volume increase was reported in the dorsolateral and orbitofrontal cortices (65).
Overall, our results are mostly in line with the literature, showing general frontal hypovolumetry in relapsers compared to abstainers, the latter having possibly recovered GM volumes at long-term (7 years).
4.3. White matter longitudinal analysis
In line with the literature and with our hypotheses, relapsers compared to abstainers showed a widespread WM volume increase along with long-term abstinence in the cingulum, inferior frontal and temporal regions and adjacent to the bilateral CN. WM volume increase was also detected in the commissural tracts (genu, body and splenium of the corpus callosum), the corona radiata bilaterally, left ALIC, the external capsule and the left superior and left inferior longitudinal fasciculi, and finally the inferior fronto occipital fasciculus.
This is in line with previous longitudinal reports stating that relapsers compared to abstainers have smaller WM volume after 24 months (11), after 13 months (9) and after as early as 8 months of abstinence, in the brainstem, corpus callosum, cerebellum, bilateral temporal, anterior, and middle frontal WM connected to the bilateral orbitofrontal cortex (12), and in the WM in close proximity to the right frontal cortex and adjacent to anterior cingulate (14). Thus, WM volume starts to increase in a linear manner in AUD patients after at least 7.5 months of abstinence (20, 21) and the present results support that this effect remains on the long-term.
No significant difference in WM volume was found, after 7 6? years, between controls and abstainers, in line with reports of recovered WM assessed by Diffusion Tensor Imaging (20, 21) in abstainers, although with shorter abstinence duration.
On a speculative note, we provide evidence of volume recovery with abstinence in cortical regions and WM, while volumes in appetitive (sub-cortical CN) regions did not vary. Imbalance between the “appetitive” network including the CN, and the “executive” network including the cingulate and prefrontal cortex (82), might therefore lead to a failure to optimize the regulation of relevant functions (follow-up of recent actions, anticipation of results and action choice) that could a fortiori increase vulnerability to relapse.
4.4. Limitations
Due to our stringent exclusion criteria and the rigorous quality control processes, eligible patient profiles (only male Caucasian subjects, characterized by good social functioning, and preserved executive functions) were rare in a hospital setting. As we included subjects with Babor's Type A alcohol addiction, our results cannot be generalized to all AUD patients. Our sample was homogeneous but small, making our findings mostly exploratory and further studies are needed to extend our results to all alcohol-dependent patients.
Indeed, from the 26 patients and 24 controls recruited at baseline, we were only able to re-include 17 patients and 6 controls at follow-up. Most control participants were difficult to reach and were lost at follow-up due to the long duration of the study. This could explain that we did not find any difference between groups in the cross-sectional analysis, at follow-up.
As we already mentioned, many participants were lost at follow-up due to the long duration of the study but also to technical difficulties (cf Flow chart in Supplementary Figure 1). Consequently, our small sample limited our possibilities to highlight correlations between neuroimaging and neuropsychology.
A technical limitation in long-term longitudinal studies is linked to evolving MRI methods. At baseline, and then at follow-up, we had access to a 1.5 Tesla MRI, but, at follow-up, the acquisition settings had been slightly updated twice. However, the same machine was used for both evaluations. We could have used another MRI machine at high field strengths at follow-up, but this would have created another bias.
Further studies should continue to investigate other typologies of alcohol-dependence, such as Type B alcoholism, which is often associated with family history of alcoholism and related genetic data (BDNF gene). Some studies showed that among AUD patients and after 7 months of abstinence, BDNF gene Val/Val homozygotes displayed an increase in hippocampal volume compared to Val/Met heterozygotes (19). Another study showed a caudate nuclei volume decrease among Val/Val after 5 weeks of abstinence, but not among Val/Met (68). Mon et al. (68), showed that caudate nuclei volume recovery in abstinent Type A alcohol-dependent individuals was dependent on BDNF genotype. Indeed, among 41 middle-aged alcohol-dependent subjects (including 5 women), who started their heavy drinking around 27 years old and without biomedical or psychiatric disorders, Val/Val genotype patients had a caudate nuclei volume decrease after 5 weeks of abstinence, whereas Val/Met did not. The BDNF Val66Met (rs6265) polymorphism was significantly related to the recovery of regional GM tissue volumes within the first 5 weeks of sobriety, suggesting genetic influences on brain tissue changes during abstinence from alcohol in this Type A alcohol-dependent cohort. The BDNF is associated with neuronal survival, neuronal growth and synaptic plasticity in the adult brain (83). The allelic association of the A1 allele of the DRD2 gene with alcohol-dependence was found in males but not in females. This discrepancy could be explained by the gender difference in dopamine D2-like receptor affinity and levels (84). Moreover, it has been shown that the presence of this A1 allele of the DRD2 gene is correlated with a lower density of the D2 receptor in the striatum, including the caudate nucleus (85).
Overall, these results suggest that caudate volume in males with type A alcoholism could be associated with BDNF genotype. In our study, we did not perform genetic analyses due to our small sample and due to the scarcity of genetic assays.
5. Conclusions
The present findings raise the hypothesis of higher caudate GM volume to be a candidate risk factor of relapse. In patients with specific type A alcohol-dependence, we showed that long-term recovery in fronto-striato-limbic GM and WM volumes occurs during long-term abstinence. These results support the crucial role of frontal circuitry in AUD.
Data availability statement
The original contributions presented in the study are included in the article/Supplementary material, further inquiries can be directed to the corresponding author.
Ethics statement
The studies involving human participants were reviewed and approved by the Bicêtre Ethics Committee (CPP-IDF 7) had approved the study protocol. The patients/participants provided their written informed consent to participate in this study.
Author contributions
J-LM obtained funding for the study. J-LM, AB, and CM designed the study and wrote the protocol. EA, J-LM, and CM conducted literature searches and analyses. SC, CM, and H-JA recruited participants. CM, EA, and J-LM conducted their clinical assessments. SC, CM, J-LM, and EA assisted the MR image acquisition in patients and controls. EA conceptualized and designed longitudinal analyses. CM and RM conducted cross-sectional and longitudinal image data processing as well as all statistical analyses. CM, EA, and J-LM interpreted the data, prepared the manuscript and wrote and edited the manuscript. RM, BR, AP, H-JA, AA, SC, and AB critically reviewed the manuscript for intellectual content and edited of final draft. All authors agree for all aspects of this work. All authors approved the final version of the manuscript for publication.
Funding
This study was supported by grants from the French Society of Alcohol Studies (Société Française d'Alcoologie, SFA), the French Interministry Mission against Drugs and Addiction (Mission Interministérielle de Lutte contre la Drogue et les Conduites Addictives, MILDECA, No. A05248LS), the Foundation for Medical Research (Fondation pour la Recherche Médicale, FRM, DPA 20140229802), the INSERM ATC Alcool 2007, and the INSERM PNR Alcool 2008.
Conflict of interest
H-JA is a member of advisory boards or DSMB for Bioprojet, and Ethypharm, and has received sponsorship to attend scientific meetings, speaker honoraria or consultancy fees from Bioprojet, D&A Pharma, Ethypharm, Kinnov Pharmaceuticals, and Lundbeck. He is also a member of the American Society of Clinical Psychopharmacology's Alcohol Clinical Trials Initiative (ACTIVE), which was supported in the last 3 years by Alkermes, Amygdala Neurosciences, Arbor Pharmaceuticals, Ethypharm, Indivior, Lundbeck, Mitsubishi, and Otsuka. AB has given talks for Lundbeck, Mylan, Merck-Serono, Bristol-Myers Squibb, and Ethypharm and is a member of the Indivior board.
The remaining authors declare that the research was conducted in the absence of any commercial or financial relationships that could be construed as a potential conflict of interest.
Publisher's note
All claims expressed in this article are solely those of the authors and do not necessarily represent those of their affiliated organizations, or those of the publisher, the editors and the reviewers. Any product that may be evaluated in this article, or claim that may be made by its manufacturer, is not guaranteed or endorsed by the publisher.
Supplementary material
The Supplementary Material for this article can be found online at: https://www.frontiersin.org/articles/10.3389/fpsyt.2023.1067326/full#supplementary-material
References
1. Harper C, Kril J. An introduction to alcohol-induced brain damage and its causes. Alcohol Alcohol Oxf Oxfs. (1994) 2:237–43.
2. Schulte T, Oberlin BG, Kareken DA, Marinkovic K, Müller-Oehring EM, Meyerhoff DJ, et al. How acute and chronic alcohol consumption affects brain networks: Insights from multimodal neuroimaging. Alcohol Clin Exp Res. (2012) 36:2017–27. doi: 10.1111/j.1530-0277.2012.01831.x
3. Zahr NM, Pfefferbaum A, Sullivan EV. Perspectives on fronto-fugal circuitry from human imaging of alcohol use disorders. Neuropharmacology. (2017) 122:189–200. doi: 10.1016/j.neuropharm.2017.01.018
4. Florence L, Lassi DLS, Kortas GT, Lima DR, de Azevedo-Marques Périco C, Andrade AG, et al. Brain Correlates of the Alcohol Use Disorder Pharmacotherapy Response: A Systematic Review of Neuroimaging Studies. Brain Sci. (2022) 12:386. doi: 10.3390/brainsci12030386
5. Castaldelli-Maia JM, Malbergier A, de Oliveira ABP, Amaral RA, Negrão AB, Gonçalves PD, et al. Exploring the Role of Alcohol Metabolizing Genotypes in a 12-Week Clinical Trial of Naltrexone for Alcohol Use Disorder. Biomolecules. (2021) 11:1495. doi: 10.3390/biom11101495
6. Chanraud S, Martelli C, Delain F, Kostogianni N, Douaud G, Aubin H-J, et al. Brain morphometry and cognitive performance in detoxified alcohol-dependents with preserved psychosocial functioning. Neuropsychopharmacol. (2007) 32:429–38. doi: 10.1038/sj.npp.1301219
7. Fein G, Di Sclafani V, Cardenas VA, Goldmann H, Tolou-Shams M, Meyerhoff DJ. Cortical gray matter loss in treatment-naïve alcohol dependent individuals. Alcohol Clin Exp Res. (2002) 26:558–64. doi: 10.1111/j.1530-0277.2002.tb02574.x
8. Pfefferbaum A, Sullivan EV, Rosenbloom MJ, Mathalon DH, Lim KO, A. Controlled Study of Cortical Gray Matter and Ventricular Changes in Alcoholic Men Over a 5-Year Interval. Arch Gen Psychiatry. (1998) 55:905. doi: 10.1001/archpsyc.55.10.905
9. Pfefferbaum A, Sullivan EV, Mathalon DH, Shear PK, Rosenbloom MJ, Lim KO. Longitudinal changes in magnetic resonance imaging brain volumes in abstinent and relapsed alcoholics. Alcohol Clin Exp Res. (1995) 19:1177–91. doi: 10.1111/j.1530-0277.1995.tb01598.x
10. Gazdzinski S, Durazzo TC, Meyerhoff DJ. Temporal dynamics and determinants of whole brain tissue volume changes during recovery from alcohol dependence. Drug Alcohol Depend. (2005) 78:263–73. doi: 10.1016/j.drugalcdep.2004.11.004
11. Rohlfing T, Sullivan EV, Pfefferbaum A. Deformation-based brain morphometry to track the course of alcoholism: Differences between intra-subject and inter-subject analysis. Psychiatry Res Neuroim. (2006) 146:157–70. doi: 10.1016/j.pscychresns.2005.12.002
12. Cardenas VA, Studholme C, Gazdzinski S, Durazzo TC, Meyerhoff DJ. Deformation-based morphometry of brain changes in alcohol dependence and abstinence. Neuroimage. (2007) 34:879–87. doi: 10.1016/j.neuroimage.2006.10.015
13. Durazzo TC, Gazdzinski S, Yeh P-H, Meyerhoff DJ. Combined neuroimaging, neurocognitive and psychiatric factors to predict alcohol consumption following treatment for alcohol dependence. Alcohol Alcohol. (2008) 43:683–91. doi: 10.1093/alcalc/agn078
14. Cardenas VA, Durazzo TC, Gazdzinski S, Mon A, Studholme C, Meyerhoff DJ. Brain morphology at entry into treatment for alcohol dependence is related to relapse propensity. Biol Psychiatry. (2011) 70:561–7. doi: 10.1016/j.biopsych.2011.04.003
15. Demirakca T, Ende G, Kämmerer N, Welzel-Marquez H, Hermann D, Heinz A, et al. Effects of alcoholism and continued abstinence on brain volumes in both genders: Effects of alcoholism on brain volumes. Alcohol Clin Exp Res. (2011) 35:1678–85. doi: 10.1111/j.1530-0277.2011.01514.x
16. Durazzo TC, Tosun D, Buckley S, Gazdzinski S, Mon A, Fryer SL, et al. Cortical thickness, surface area, and volume of the brain reward system in alcohol dependence: relationships to relapse and extended abstinence. Alcohol Clin Exp Res. (2011) 35:1187–200. doi: 10.1111/j.1530-0277.2011.01452.x
17. Beck A, Wüstenberg T, Genauck A, Wrase J, Schlagenhauf F, Smolka MN, et al. Effect of brain structure, brain function, and brain connectivity on relapse in alcohol-dependent patients. Arch Gen Psychiatry. (2012) 69:842–52. doi: 10.1001/archgenpsychiatry.2011.2026
18. Fein G, Fein D. Subcortical volumes are reduced in short-term and long-term abstinent alcoholics but not those with a comorbid stimulant disorder. NeuroImage Clin. (2013) 3:47–53. doi: 10.1016/j.nicl.2013.06.018
19. Hoefer ME, Pennington DL, Durazzo TC, Mon A, Abé C, Truran D, et al. Genetic and behavioral determinants of hippocampal volume recovery during abstinence from alcohol. Alcohol. (2014) 48:631–8. doi: 10.1016/j.alcohol.2014.08.007
20. Durazzo TC, Mon A, Pennington D, Abé C, Gazdzinski S, Meyerhoff DJ. Interactive effects of chronic cigarette smoking and age on brain volumes in controls and alcohol-dependent individuals in early abstinence: Interactive effects. Addict Biol. (2014) 19:132–43. doi: 10.1111/j.1369-1600.2012.00492.x
21. Durazzo TC, Mon A, Gazdzinski S, Yeh P-H, Meyerhoff DJ. Serial longitudinal magnetic resonance imaging data indicate non-linear regional gray matter volume recovery in abstinent alcohol-dependent individuals: Non-linear volume recovery. Addict Biol. (2015) 20:956–67. doi: 10.1111/adb.12180
22. Durazzo TC, Mon A, Gazdzinski S, Meyerhoff DJ. Regional brain volume changes in alcohol-dependent individuals during early abstinence: associations with relapse following treatment: Brain volumes. Addict Biol. (2017) 22:1416–25. doi: 10.1111/adb.12420
23. Wrase J, Makris N, Braus DF, Mann K, Smolka MN, Kennedy DN, et al. Amygdala volume associated with alcohol abuse relapse and craving. Am J Psychiatry. (2008) 165:1179–84. doi: 10.1176/appi.ajp.2008.07121877
24. Segobin SH, Chételat G, Le Berre A-P, Lannuzel C, Boudehent C, Vabret F, et al. Relationship between brain volumetric changes and interim drinking at six months in alcohol-dependent patients. Alcohol Clin Exp Res. (2014) 38:739–48. doi: 10.1111/acer.12300
25. Pfefferbaum A, Rosenbloom MJ, Chu W, Sassoon SA, Rohlfing T, Pohl KM, et al. White matter microstructural recovery with abstinence and decline with relapse in alcoholism: Interaction with normal aging revealed with longitudinal DTI. Lancet Psychiatry. (2014) 1:202–12. doi: 10.1016/S2215-0366(14)70301-3
26. Noel X. Contribution of frontal cerebral blood flow measured by 99mtc-bicisate spect and executive function deficits to predicting treatment outcome in alcohol-dependent patients. Alcohol Alcohol. (2002) 37:347–54. doi: 10.1093/alcalc/37.4.347
27. Babor TF. Types of alcoholics, I: Evidence for an empirically derived typology based on indicators of vulnerability and severity. Arch Gen Psychiatry. (1992) 49:599. doi: 10.1001/archpsyc.1992.01820080007002
28. Pfefferbaum A, Rosenbloom M, Deshmukh A, Sullivan EV. Sex differences in the effects of alcohol on brain structure. Am J Psychiatry. (2001) 158:188–97. doi: 10.1176/appi.ajp.158.2.188
29. Annett M. The binomial distribution of right, mixed and left handedness. Q J Exp Psychol. (1967) 19:327–33. doi: 10.1080/14640746708400109
30. Reinert DF, Allen JP. The alcohol use disorders identification test (AUDIT): A review of recent research. Alcohol Clin Exp Res. (2002) 26:272–9. doi: 10.1111/j.1530-0277.2002.tb02534.x
31. Pfefferbaum A, Zipursky RB, Mathalon DH, Rosenbloom MJ, Lane B, Ha CN, et al. Brain gray and white matter volume loss accelerates with aging in chronic alcoholics: a quantitative MRI study. Alcohol Clin Exp Res. (1992) 16:1078–89. doi: 10.1111/j.1530-0277.1992.tb00702.x
32. Squeglia LM, Tapert SF, Sullivan EV, Jacobus J, Meloy MJ, Rohlfing T, et al. Brain development in heavy-drinking adolescents. Am J Psychiatry. (2015) 172:531–42. doi: 10.1176/appi.ajp.2015.14101249
33. DeWit DJ. Age at First Alcohol Use: A Risk Factor for the Development of Alcohol Disorders. Am J Psychiatry. (2000) 157:745–50. doi: 10.1176/appi.ajp.157.5.745
34. Hamilton M. A rating scale for depression. J Neurol Neurosurg Psychiatry. (1960) 23:56–62. doi: 10.1136/jnnp.23.1.56
35. Hamilton M. The assessment of anxiety states by rating. Br J Med Psychol. (1959) 32:50–5. doi: 10.1111/j.2044-8341.1959.tb00467.x
36. Cohen JA, Kaplan MM. The SGOT/SGPT ratio? An indicator of alcoholic liver disease. Dig Dis Sci. (1979) 24:835–8. doi: 10.1007/BF01324898
37. Fein G, Shimotsu R, Chu R, Barakos J. Parietal gray matter volume loss is related to spatial processing deficits in long-term abstinent alcoholic men. Alcohol Clin Exp Res. (2009) 33:1806–14. doi: 10.1111/j.1530-0277.2009.01019.x
38. Heatherton TF, Kozlowski LT, Frecker RC, Fagerström KO. The fagerström test for nicotine dependence: A revision of the fagerström tolerance questionnaire. Br J Addict. (1991) 86:1119–27. doi: 10.1111/j.1360-0443.1991.tb01879.x
39. Weissman MM, Bothwell S. Assessment of social adjustment by patient self-report. Arch Gen Psychiatry. (1976) 33:1111–5. doi: 10.1001/archpsyc.1976.01770090101010
40. Folstein MF, Folstein SE, McHugh PR. “Mini-mental state”. J Psychiatr Res. (1975) 12:189–98. doi: 10.1016/0022-3956(75)90026-6
41. Barona A, Reynolds CR, Chastain R, A. demographically based index of premorbid intelligence for the WAIS—R. J Consult Clin Psychol. (1984) 52:885–7. doi: 10.1037/0022-006X.52.5.885
42. Sameti M, Smith S, Patenaude B, Fein G. Subcortical Volumes in Long-Term Abstinent Alcoholics: Associations With Psychiatric Comorbidity: subcortical volumes in abstinent alcoholics. Alcohol Clin Exp Res. (2011) 35:1067–80. doi: 10.1111/j.1530-0277.2011.01440.x
43. Cardenas VA, Price M, Fein G, EEG. coherence related to fMRI resting state synchrony in long-term abstinent alcoholics. NeuroImage Clin. (2017) 17:481–90. doi: 10.1016/j.nicl.2017.11.008
44. Camchong J, Stenger A, Fein G. Resting-state synchrony during early alcohol abstinence can predict subsequent relapse. Cereb Cortex N Y N. (2013) 23:2086–99. doi: 10.1093/cercor/bhs190
45. Ashburner J, Friston KJ. Voxel-Based Morphometry—The Methods. Neuroimage. (2000) 11:805–21. doi: 10.1006/nimg.2000.0582
46. Yeung AWK. An updated survey on statistical thresholding and sample size of fMRI studies. Front Hum Neurosci. (2018) 12:16. doi: 10.3389/fnhum.2018.00016
47. Agartz I, Momenan R, Rawlings RR, Kerich MJ, Hommer DW. Hippocampal volume in patients with alcohol dependence. Arch Gen Psychiatry. (1999) 56:356–63. doi: 10.1001/archpsyc.56.4.356
48. Mechtcheriakov S, Brenneis C, Egger K, Koppelstaetter F, Schocke M, Marksteiner J, et al. widespread distinct pattern of cerebral atrophy in patients with alcohol addiction revealed by voxel-based morphometry. J Neurol Neurosurg Psychiatry. (2007) 78:610–4. doi: 10.1136/jnnp.2006.095869
49. Monnig MA, Tonigan JS, Yeo RA, Thoma RJ, McCrady BS. White matter volume in alcohol use disorders: a meta-analysis: White matter volume in AUDs. Addict Biol. (2013) 18:581–92. doi: 10.1111/j.1369-1600.2012.00441.x
50. Pfefferbaum A, Adalsteinsson E, Sullivan EV. Dysmorphology and microstructural degradation of the corpus callosum: Interaction of age and alcoholism. Neurobiol Aging. (2006) 27:994–1009. doi: 10.1016/j.neurobiolaging.2005.05.007
51. Pfefferbaum A, Rosenbloom M, Rohlfing T, Sullivan EV. Degradation of association and projection white matter systems in alcoholism detected with quantitative fiber tracking. Biol Psychiatry. (2009) 65:680–90. doi: 10.1016/j.biopsych.2008.10.039
52. Yeh P-H, Simpson K, Durazzo TC, Gazdzinski S, Meyerhoff DJ. Tract-based spatial statistics (TBSS) of diffusion tensor imaging data in alcohol dependence: Abnormalities of the motivational neurocircuitry. Psychiatry Res Neuroimaging. (2009) 173:22–30. doi: 10.1016/j.pscychresns.2008.07.012
53. Jang D-P, Namkoong K, Kim J-J, Park S, Kim I-Y, Kim SI, et al. The relationship between brain morphometry and neuropsychological performance in alcohol dependence. Neurosci Lett. (2007) 428:21–6. doi: 10.1016/j.neulet.2007.09.047
54. Zahr NM. Structural and microstructral imaging of the brain in alcohol use disorders. In: Handbook of Clinical Neurology. Elsevier (2014). p. 275–290 doi: 10.1016/B978-0-444-62619-6.00017-3
55. Kühn S, Mascharek A, IMAGEN Consortium. Predicting development of adolescent drinking behaviour from whole brain structure at 14 years of age. Elife. (2019) 2:1–15. doi: 10.7554/eLife.44056.011
56. Howell NA, Worbe Y, Lange I, Tait R, Irvine M, Banca P, et al. Increased ventral striatal volume in college-aged binge drinkers. PLoS ONE. (2013) 8:e74164. doi: 10.1371/journal.pone.0074164
57. Ersche KD, Barnes A, Jones PS, Morein-Zamir S, Robbins TW, Bullmore ET. Abnormal structure of frontostriatal brain systems is associated with aspects of impulsivity and compulsivity in cocaine dependence. Brain J Neurol. (2011) 134:2013–24. doi: 10.1093/brain/awr138
58. Jacobsen LK, Giedd JN, Gottschalk C, Kosten TR, Krystal JH. Quantitative morphology of the caudate and putamen in patients with cocaine dependence. Am J Psychiatry. (2001) 158:486–9. doi: 10.1176/appi.ajp.158.3.486
59. Chang LAD, Ernst TVN. Structural and metabolic brain changes in the striatum associated with methamphetamine abuse. Addiction. (2007) 102:16–32. doi: 10.1111/j.1360-0443.2006.01782.x
60. Jernigan TL, Gamst AC, Archibald SL, Fennema-Notestine C, Mindt MR, Marcotte TL, et al. Effects of methamphetamine dependence and HIV infection on cerebral morphology. Am J Psychiatry. (2005) 162:1461–72. doi: 10.1176/appi.ajp.162.8.1461
61. Agartz I, Brag S, Franck J, Hammarberg A, Okugawa G, Svinhufvud K, et al. volumetry during acute alcohol withdrawal and abstinence: a descriptive study. Alcohol Alcohol Oxf Oxfs. (2003) 38:71–8. doi: 10.1093/alcalc/agg020
62. Gazdzinski S, Durazzo TC, Mon A, Yeh P-H, Meyerhoff DJ. Cerebral white matter recovery in abstinent alcoholics—a multimodality magnetic resonance study. Brain. (2010) 133:1043–53. doi: 10.1093/brain/awp343
63. van Eijk J, Demirakca T, Frischknecht U, Hermann D, Mann K, Ende G. Rapid partial regeneration of brain volume during the first 14 days of abstinence from alcohol. Alcohol Clin Exp Res. (2013) 37:67–74. doi: 10.1111/j.1530-0277.2012.01853.x
64. Yeh P-H, Gazdzinski S, Durazzo TC, Sjöstrand K, Meyerhoff DJ. Hierarchical linear modeling (HLM) of longitudinal brain structural and cognitive changes in alcohol-dependent individuals during sobriety. Drug Alcohol Depend. (2007) 91:195–204. doi: 10.1016/j.drugalcdep.2007.05.027
65. Zou X, Durazzo TC, Meyerhoff DJ. Regional brain volume changes in alcohol-dependent individuals during short-term and long-term abstinence. Alcohol Clin Exp Res. (2018) 42:1062–72. doi: 10.1111/acer.13757
66. Rando K, Hong K-I, Bhagwagar Z, Li C-SR, Bergquist K, Guarnaccia J, et al. Association of frontal and posterior cortical gray matter volume with time to alcohol relapse: a prospective study. Am J Psychiatry. (2011) 168:183–92. doi: 10.1176/appi.ajp.2010.10020233
67. Wang G-Y, Demirakca T, van Eijk J, Frischknecht U, Ruf M, Ucar S, et al. Longitudinal mapping of gyral and sulcal patterns of cortical thickness and brain volume regain during early alcohol abstinence. Eur Addict Res. (2016) 22:80–9. doi: 10.1159/000438456
68. Mon A, Durazzo TC, Gazdzinski S, Hutchison KE, Pennington D, Meyerhoff DJ. Brain-derived neurotrophic factor genotype is associated with brain gray and white matter tissue volumes recovery in abstinent alcohol-dependent individuals: The influence of BDNF on brain tissue volumes recovery. Genes Brain Behav. (2013) 12:98–107. doi: 10.1111/j.1601-183X.2012.00854.x
69. Makris N, Oscar-Berman M, Jaffin SK, Hodge SM, Kennedy DN, Caviness VS, et al. Decreased volume of the brain reward system in alcoholism. Biol Psychiatry. (2008) 64:192–202. doi: 10.1016/j.biopsych.2008.01.018
70. Fein G, Greenstein D, Cardenas VA, Cuzen NL, Foucheb J-P, Ferrett H, et al. Cortical and subcortical volumes in adolescents with alcohol dependence but without substance or psychiatric comorbidities. Psychiatry Res. (2013) 214:1–8. doi: 10.1016/j.pscychresns.2013.06.001
71. Balleine BW, O'Doherty JP. Human and rodent homologies in action control: Corticostriatal determinants of goal-directed and habitual action. Neuropsychopharmacology. (2010) 35:48–69. doi: 10.1038/npp.2009.131
72. Grahn JA, Parkinson JA, Owen AM. The cognitive functions of the caudate nucleus. Prog Neurobiol. (2008) 86:141–55. doi: 10.1016/j.pneurobio.2008.09.004
73. Postuma RB, Dagher A. Basal ganglia functional connectivity based on a meta-analysis of 126 positron emission tomography and functional magnetic resonance imaging publications. Cereb Cortex N Y N. (2006) 16:1508–21. doi: 10.1093/cercor/bhj088
74. Lehéricy S, Ducros M, Van De Moortele P-F, Francois C, Thivard L, Poupon C, et al. Diffusion tensor fiber tracking shows distinct corticostriatal circuits in humans: DTI Corticostriatal Fibers. Ann Neurol. (2004) 55:522–9. doi: 10.1002/ana.20030
75. Fein G, Cardenas VA. Neuroplasticity in human alcoholism: studies of extended abstinence with potential treatment implications. Alcohol Res Curr Rev. (2015) 37:125–41.
76. Goldstein RZ, Volkow ND. Dysfunction of the prefrontal cortex in addiction: neuroimaging findings and clinical implications. Nat Rev Neurosci. (2011) 12:652–69. doi: 10.1038/nrn3119
77. Everitt BJ, Robbins TW. Neural systems of reinforcement for drug addiction: from actions to habits to compulsion. Nat Neurosci. (2005) 8:1481–9. doi: 10.1038/nn1579
78. Yager LM, Garcia AF, Wunsch AM, Ferguson SM. The ins and outs of the striatum: Role in drug addiction. Neuroscience. (2015) 301:529–41. doi: 10.1016/j.neuroscience.2015.06.033
79. Boileau I, Payer D, Chugani B, Lobo DSS, Houle S, Wilson AA, et al. In vivo evidence for greater amphetamine-induced dopamine release in pathological gambling: a positron emission tomography study with [(11)C]-(+)-PHNO. Mol Psychiatry. (2014) 19:1305–13. doi: 10.1038/mp.2013.163
80. van Holst RJ, Sescousse G, Janssen LK, Janssen M, Berry AS, Jagust WJ, et al. Increased striatal dopamine synthesis capacity in gambling addiction. Biol Psychiatry. (2018) 83:1036–43. doi: 10.1016/j.biopsych.2017.06.010
81. Heinz A, Beck A, Grüsser SM, Grace AA, Wrase J. Identifying the neural circuitry of alcohol craving and relapse vulnerability. Addict Biol. (2009) 14:108–18. doi: 10.1111/j.1369-1600.2008.00136.x
82. Camchong J, Stenger VA, Fein G. Resting-state synchrony in short-term versus long-term abstinent alcoholics. Alcohol Clin Exp Res. (2013) 37:794–803. doi: 10.1111/acer.12037
83. Lu B, Nagappan G, Lu Y. BDNF and synaptic plasticity, cognitive function, and dysfunction. In:Lewin GR, Carter BD, , editors. Neurotrophic Factors. Handbook of Experimental Pharmacology. Berlin, Heidelberg: Springer Berlin Heidelberg (2014). p. 223–250. doi: 10.1007/978-3-642-45106-5_9
84. Limosin F, Gorwood P, Loze J-Y, Dubertret C, Gouya L, Deybach J-C, et al. Male limited association of the dopamine receptor D2 gene TaqI a polymorphism and alcohol dependence. Am J Med Genet. (2002) 112:343–6. doi: 10.1002/ajmg.10712
Keywords: caudate nuclei, alcohol use disorder, relapsers, abstainers, structural magnetic resonance imaging, longitudinal analysis
Citation: Martelli C, Artiges E, Miranda R, Romeo B, Petillion A, Aubin H-J, Amirouche A, Chanraud S, Benyamina A and Martinot J-L (2023) Caudate gray matter volumes and risk of relapse in Type A alcohol-dependent patients: A 7-year MRI follow-up study. Front. Psychiatry 14:1067326. doi: 10.3389/fpsyt.2023.1067326
Received: 11 October 2022; Accepted: 20 January 2023;
Published: 15 February 2023.
Edited by:
Johannes Petzold, University Hospital Carl Gustav Carus, GermanyReviewed by:
Dieter J. Meyerhoff, University of California, San Francisco, United StatesDomenico De Berardis, Department of Mental Health (ASL 4), Italy
Copyright © 2023 Martelli, Artiges, Miranda, Romeo, Petillion, Aubin, Amirouche, Chanraud, Benyamina and Martinot. This is an open-access article distributed under the terms of the Creative Commons Attribution License (CC BY). The use, distribution or reproduction in other forums is permitted, provided the original author(s) and the copyright owner(s) are credited and that the original publication in this journal is cited, in accordance with accepted academic practice. No use, distribution or reproduction is permitted which does not comply with these terms.
*Correspondence: Catherine Martelli, Y2F0aGVyaW5lLm1hcnRlbGxpQGFwaHAuZnI=