Corrigendum: The alternations of nucleus accumbent in schizophrenia patients with auditory verbal hallucinations during low-frequency rTMS treatment
- 1School of Education, Xinyang College, Xinyang, China
- 2Department of Radiology, Xijing Hospital, Air Force Medical University, Xi'an, China
- 3Department of Neurodevelopment Psychology, School of Psychology, Army Medical University, Chongqing, China
- 4Department of Mental Health, Xi'an Medical University, Xi'an, China
- 5Department of Psychiatry, Xijing Hospital, Air Force Medical University, Xi'an, China
- 6Department of Clinical Psychology, Air Force Medical University, Xi'an, China
- 7Department of Military Medical Psychology, Air Force Medical University, Xi'an, China
Low-frequency repetitive transcranial magnetic stimulation (rTMS) has been shown to reduce the severity of auditory verbal hallucinations (AVH) and induce beneficial functional and structural alternations of the brain in schizophrenia patients with AVH. The nucleus accumbens (NAcc) as an important component of the ventral striatum is implicated with the pathology in AVH. However, the induced characteristic patterns of NAcc by low-frequency rTMS in schizophrenia with AVH are seldom explored. We investigated the functional and structural characteristic patterns of NAcc by using seed-based functional connectivity (FC) analysis and gray matter volume (GMV) measurement in schizophrenia patients with AVH during 1 Hz rTMS treatment. Although low-frequency rTMS treatment did not affect the volumetric changes of NAcc, the abnormal FC patterns of NAcc, including increased FC of NAcc with the temporal lobes and decreased FC of NAcc with the frontal cortices in the pretreatment patients compared to healthy controls, were normalized or reversed after treatment. These FC changes were associated with improvements in clinical symptoms and neurocognitive functions. Our findings may extend our understanding of the NAcc in the pathology of schizophrenia with AVH and might be a biomarker of clinical effect for low-frequency rTMS treatment in schizophrenia.
Introduction
Schizophrenia is a chronic and disabling disease that affects ~0.7% of the population (1). Symptoms associated with schizophrenia can be divided into three domains: positive symptoms (e.g., hallucinations and delusions), negative symptoms (e.g., avolition and withdrawal), and cognitive symptoms (e.g., memory and executive function) (2). The etiology of schizophrenia is still poorly understood. However, the neurobiology of the psychotic symptoms has been associated with dopaminergic abnormality in the striatum (3). Abnormal dopaminergic regulation of striatal function could explain the mechanisms underlying the symptoms of schizophrenia (4, 5). Of particular interest is the nucleus accumbens (NAcc), a central component of the ventral striatum, which plays an important role in the pathology of schizophrenia (6). The modulation of the striatal circuit activity can reduce psychotic symptoms (7). Thus, NAcc has been proposed as the critical target for antipsychotic medications (8).
NAcc receives intensive excitatory afferents from the frontal cortex, hippocampus, and amygdala, closely associated with dopaminergic changes in schizophrenia pathology (9). Several studies have reported increased dopaminergic activity in the NAcc in schizophrenia (10, 11). Subsequent animal studies have confirmed similar findings (12–14). Structural abnormalities in the NAcc have been consistently illustrated in schizophrenia. There were significant reductions in gray matter volume (GMV)of the NAcc in schizophrenic brains from the structural magnetic resonance imaging data (15–17). In addition, resting-state functional magnetic resonance imaging (fMRI) studies have observed abnormal intrinsic functional connectivity (FC) of NAcc in schizophrenia (18–20), regions mainly located in the frontal, parietal, temporal, and limbic systems (e.g., the cingulate cortex, insula, parahippocampal gyrus, and ventral tegmental area). Therefore, NAcc is the primary region interacting with multiple areas of cortical and limbic systems and could provide a supplementary understanding of pathology in schizophrenia.
Current treatments of antipsychotics are thought to target the NAcc and can reduce a hyperdopaminergic state of the striatum (21, 22). However, antipsychotics are only responded to symptoms and are confined in their effectiveness, and frequently accompanied by side effects (23). Meta-analysis and system review studies have indicated that the application of low-frequency repetitive transcranial magnetic stimulation (rTMS) during schizophrenia can effectively reduce the severity of auditory verbal hallucinations (AVH) (24–28), although negative findings were reported (29, 30), probably because the heterogeneity of treatment protocols and placebo response (31). AVH are defined as perceptions in the absence of external verbal stimuli and are prominent among the core symptoms of schizophrenia (32). The activation of NAcc is associated with the vividness of hallucinations (33) and auditory verbal imagery in schizophrenia patients (34). Moreover, the abnormal FC (20, 35) and gray matter changes (36) of NAcc appeared to be associated with the presence of AVH and neurocognitive impairments. The results may indicate the unique role of NAcc in investigating the neural mechanisms of schizophrenia with AVH. Nevertheless, its underlying changes in schizophrenia with AVH during rTMS are seldom explored.
The purpose of the present study aimed to investigate the potential alternations of NAcc in schizophrenia patients with AVH during low-frequency rTMS treatment by using the seed-based FC analysis and GMV measurement. Correlation analyses were further done between the possible alternations of NAcc and clinical responses of patients after treatment. We hypothesized that low-frequency rTMS treatment could normalize or inverse the abnormal functional or structural patterns of NAcc and associated with the reduction of clinical symptom severity.
Materials and methods
Participants
Thirty-two patients with AVH were recruited from the Department of Psychiatry, Xijing Hospital of Fourth Military Medical University. The diagnosis of schizophrenia was made by experienced psychiatrists according to the Chinese version of the Structured Clinical Interview for Diagnosis and Statistical Manual of Mental Disorder (DSM-V). The inclusion criteria of the patient group were as follows: (1) AVH daily occurred with at least two antipsychotic medications, and (2) no less than five episodes of AVH per day over the past month. All patients who received a steady dose of antipsychotic medications remained unchanged during the study period. In addition, thirty-five healthy controls matched by age, sex, and education were recruited from the local community through advertising and had no history of psychiatric diseases. For all the participants, the exclusion criteria were as follows: (1) any past or current neurological diseases, (2) history of head injury, (3) alcohol or substance abuse, and (4) contraindications to MRI scans.
This study was approved by the Medical Ethics Committee of the Xijing Hospital and was conducted following the Declaration of Helsinki. Informed written consent was obtained from all the participants. The study was registered in the Chinese Clinical Trial Register (http://www.chictr.org/cn/, registration number: ChiCTR2100041876).
Clinical measurements
The severity of psychotic symptoms was assessed using the Positive and Negative Syndrome Scale (PANSS) (37). The AVH was assessed by the auditory Hallucination Rating Scale (AHRS) (38). The Chinese version of the MATRICS Consensus Cognitive Battery (MCCB) was used to measure neurocognitive impairment in patients consisting of 10 tasks across seven cognitive domains (39): speed of processing test (SOPT), attention and vigilance test (AVT), working memory (WMT) test, verbal learning test (VERBLT), visual learning test (VISLT), reasoning and problem-solving test (RPST), and social cognition test (SCT). All clinical measures were performed by experienced psychiatrists at baseline and after treatment.
rTMS protocol
A type of 8-figure coil magnetic stimulator (YIRUIDE Inc., Wuhan, China) was used to perform 1 Hz rTMS treatment, and the left temporoparietal junction (TPJ) was selected as the stimulation target, which is referred to as the International 10–20 electrode location system (TP3). This stimulation target has been widely applied to treat AVH in schizophrenia by using low-frequency rTMS (40, 41). Patients were treated for 15 consecutive days at 15 min per day (once per second, 5 s interval) with a 110% resting motor threshold, generating 60 trains of 600 pulses.
MRI data acquisition
MRI data were obtained using a 3.0-Tesla scanner (GE Medical Systems, Milwaukee, WI) equipped with an 8-channel phased-array head coil. The patient group was scanned twice (before and after treatment), while the control group was scanned only once. During the entire scan, the participants were instructed to stay awake with their eyes closed and remain awake and keep their heads motionless. Resting-state functional images were obtained using a gradient-echo-planar imaging sequence with the following parameters: 45 axial slices, repetition time (TR) = 2,000 ms, echo time (TE) = 40 ms, matrix = 64 × 64, field of view (FOV) = 260 × 260 mm2, flip angle = 90°; slice thickness = 3.5 mm (no gap), and 210 volumes were acquired. The T1-weighted structural images were obtained during the same scanning session by an MP-RAGE sequence as the following parameters: TR = 8.1 ms, TE = 3.2 ms, matrix size = 256 × 256, flip angle = 12°, FOV = 240 × 240 mm2, 176 slices, and thickness=1.0 mm.
Neuroimaging data preprocessing
Resting-state functional imaging data were preprocessed using the SPM (https://www.fil.ion.ucl.ac.uk/spm/) and DPABI (http://rfmri.org/dpabi) toolbox. For each participant, the first ten functional volumes were removed to assure equilibration of the magnetic field. The remaining volumes were corrected for slice acquisition and head motion. Subsequently, the corrected images were normalized into the standard Montreal Neurological Institute (MNI) space by the Exponentiated Lie Algebra (DARTEL) algorithm (42) and then resampled to a 3 × 3 × 3 mm3 resolution. Then, the normalized images were linearly detrended and regressed the nuisance covariates, including Friston 24 motion parameters (43), white matter signal, cerebrospinal fluid signal, and whole-brain global signal. Band-pass temporal filtering (0.01–0.1 Hz) was performed to reduce high-frequency physiological noise. Finally, spatial smoothing was conducted with a 6-mm Gaussian kernel for statistical analyses.
Structural imaging data were processed using SPM (https://www.fil.ion.ucl.ac.uk/spm/) and VBM (https://dbm.neuro.uni-jena.de/wordpress/vbm/) toolbox. The structural images were subjected to bias correction and tissue-classified into gray matter, white matter, and cerebrospinal fluid with the volume probability maps. The gray matter images were then normalized to standard Montreal Neurological Institute (MNI) space. Subsequently, intensity modulation and an 8 mm Gaussian kernel smoothing of the resulting images were completed.
FC analysis
The bilateral NAcc were defined as seeds based on the Anatomical Automatic Labeling (AAL3) atlas (44), see Figure 1 for details. Subsequent procedures were executed in the left and right seed individually. Pearson correlation analyses were performed between the seed reference time course and time series of the whole brain. The resulting correlation coefficients were converted into z-scores using to enhance normality.
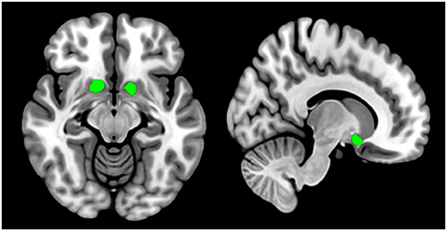
Figure 1. Nucleus accumbent seed regions of interest are defined by the Anatomical Automatic Labeling (AAL3) atlas (https://www.oxcns.org/aal3.html). Bilateral nucleus accumbent seeds are used in resting-state functional connectivity analysis and gray matter volume measure.
GMV analysis
The values of GMV from the NAcc were then extracted from the preprocessed gray matter images with the seed mask. The GMV differences of left and right NAcc were then compared between the patient and control groups or patients before and after treatment.
Statistical analysis
Statistical analysis of the demographic and clinical data was carried out using the SPSS (version 23.0; Chicago, IL, United States). Independent-sample t-test and chi-square test were conducted according to the characteristics of the data. In addition, the independent-sample t-tests were done to investigate group differences in FC and GMV between patients at baseline and controls with age, gender, education, and mean head motion (Framewise displacement, FD) parameter as covariates. These different brain regions were defined as a mask for subsequent analysis. A paired-sample t-test was used to examine the treatment effect of the two measures between patients after treatment and before treatment with the mask created above. Group statistical maps were thresholded at p < 0.05 and a voxel level of p < 0.05 with 30 voxel size using the Gaussian random field (GRF) method.
Finally, partial correlation coefficients were calculated between the altered measures and clinical responses in patients using the dosage of antipsychotics as a covariate. To explore the effect of antipsychotics on clinical symptoms and measure changes, correlations of the medication dosage with clinical response and measure changes were examined. For all correlation coefficients, a two-tailed p level of 0.05 was used as the criterion of statistical significance and corrected for multiple comparisons with the false discovery rate correction (FDR) method.
Results
Demographic and clinical data comparisons
The demographic and clinical characteristics of the participants are displayed in Table 1. The difference in age (t = 0.954, p = 0.345), sex (χ2 = 0.101, p = 0.751), and educational years (t = 1.708, p = 0.094) distribution did not reach significance in the patients at baseline and controls.
But after rTMS treatment, the clinical responses, including positive symptoms (14.45 ± 2.80 vs. 19.65 ± 4.60, t = 4.324, p = 0.000), AVH (13.75 ± 7.07 vs. 27.45 ± 6.14, t = 6.542, p = 0.000), and certain neurocognitive functions such as verbal memory (29.60 ± 12.60 vs. 39.80 ± 12.24, t = 2.597, p = 0.047) and visual memory (34.55 ± 15.95 vs. 47.00 ± 10.54, t = 2.912, p = 0.042), were improved in patients compared to before treatment. Details are displayed in Table 2.
FC comparison of NAcc seeds
The analyses of FC in the NAcc seeds between patients at baseline and controls are shown in Figure 2 and Table 3. For the left NAcc seed, the patients exhibited significantly increased FC in the left inferior temporal gyrus and right fusiform gyrus, and decreased FC in the right superior frontal gyrus and left anterior cingulate gyrus when compared with the controls (GRF correction; voxel-level p < 0.05, cluster level p < 0.05, clusters size > 30 voxels). Similar, significantly increased FC of the right NAcc seed was seen in the left middle temporal gyrus and right fusiform gyrus, and decreased FC was seen in the right inferior frontal gyrus and left anterior cingulate gyrus in patients at baseline relative to the controls (GRF correction; voxel-level p < 0.05, cluster level p < 0.05, clusters size > 30 voxels). These abnormal FC regions were defined as mask for subsequent comparisons between patients before and after treatment.
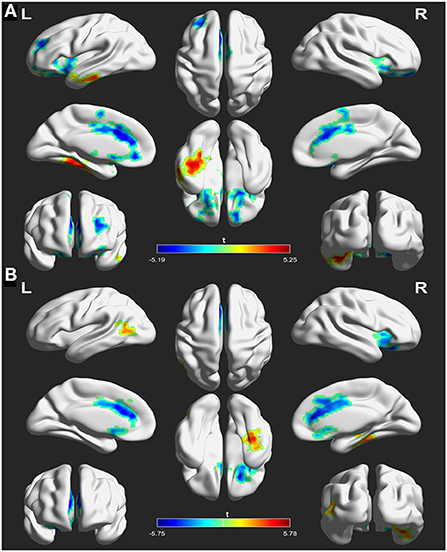
Figure 2. Differences in functional connectivity (FC) between patients at baseline and healthy controls using left nucleus accumbent (A) and right nucleus accumbent (B) seed regions. The warm color indicates an increased FC of seed with the whole brain and the cool color indicates a decreased FC of seed with the whole brain. The color scale is represented by the t-value of statistically significant clusters with the voxel-level statistical threshold of p < 0.05 and a cluster-level threshold of p < 0.05 corrected for the Gaussian random field (size >30).
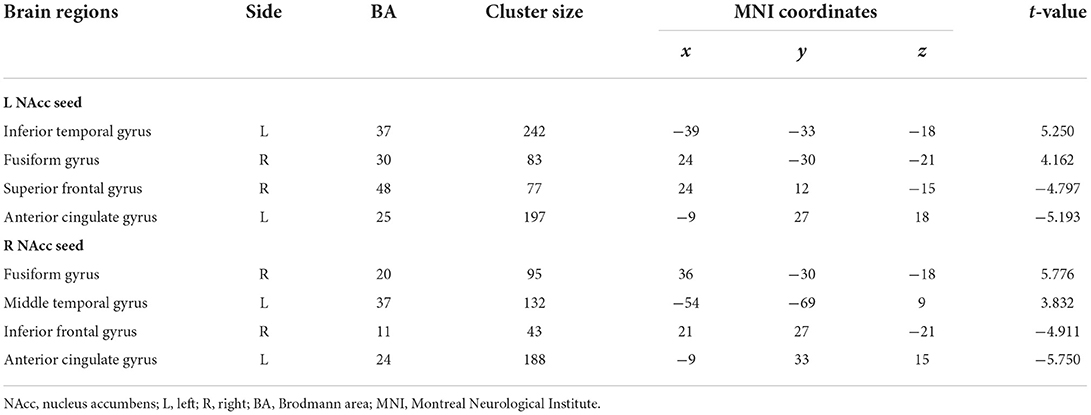
Table 3. Functional connectivity differences of the nucleus accumbens seeds between patients at baseline and controls (patients > controls).
However, these abnormal FC patterns did not persistent after rTMS treatment. Instead, initial FC of NACC with the left inferior temporal gyrus (posttreatment vs. pretreatment: −0.025 ± 0.089 vs. 0.023 ± 0.097, t = 2.723, p = 0.011) and right inferior frontal gyrus (posttreatment vs. pretreatment: 0.235 ± 0.108 vs. 0.180 ± 0.122, t = 2.652, p = 0.013) in patients before treatment was inversed after treatment. Details are displayed in Figure 3 and Table 4.
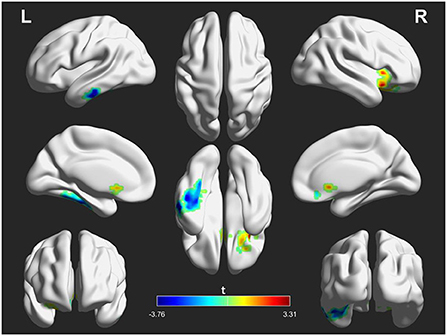
Figure 3. Differences in functional connectivity (FC) of the nucleus accumbens seeds between patients after treatment and before treatment. The warm color indicates an increased FC of seed with the whole brain and the cool color indicates a decreased FC of seed with the whole brain. The color scale is represented by the t-value of statistically significant clusters with the voxel-level statistical threshold of p < 0.05 and a cluster-level threshold of p < 0.05 corrected for the Gaussian random field (size >30).
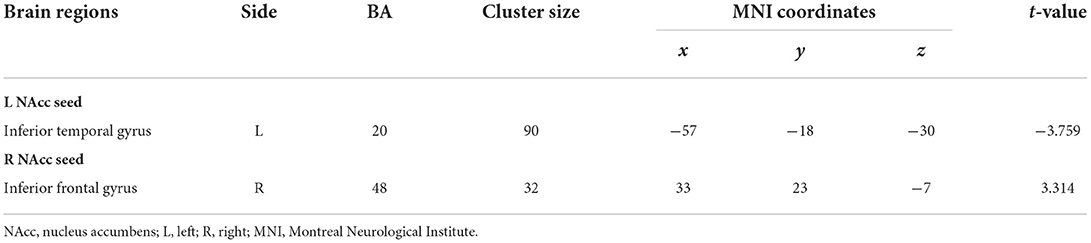
Table 4. Functional connectivity differences of the nucleus accumbens seeds between patients after treatment and before treatment (after treatment > before treatment).
GMV comparison of NAcc seeds
The volumetric analysis showed that the patients at baseline had decreased GMV in left NAcc compared to the controls (t = 2.18, p = 0.038) (Figure 4), while the rTMS treatment did not affect the volumetric changes in the left or right NAcc in patients (p > 0.05) (Figure 4).
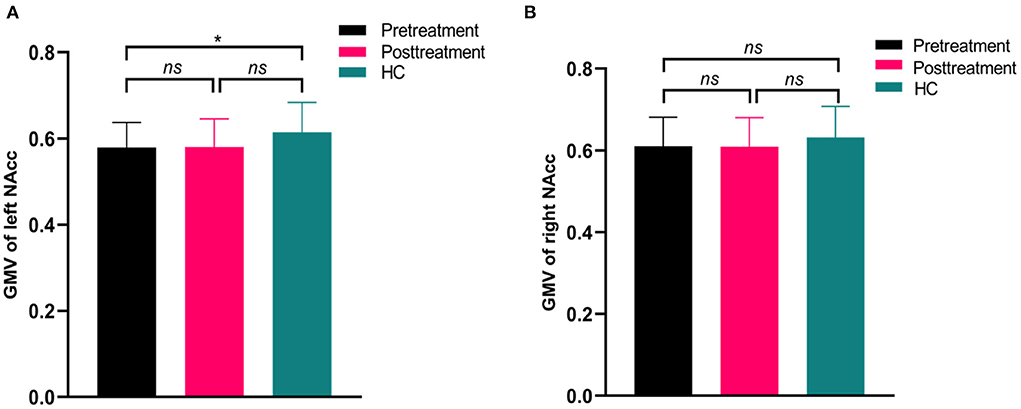
Figure 4. Differences in gray matter volume (GMV) of nucleus accumbens between patients (pretreatment and posttreatment) and healthy controls (HC). The pretreatment patients showed significantly decreased GMV in the left nucleus accumbent compared to HC (A). While there were no significant differences in the right nucleus accumbens between patients (pretreatment and posttreatment) and HC (B). *p > 0.05; ns, no significance.
Correlation analysis
In the patient group, the changed FC value in the left NAcc seed with the left inferior temporal gyrus was positively correlated to the changed positive symptom score of PNASS (r = −0.545, p = 0.024, FDR correction). In addition, the changed FC value of the right NAcc seed with the right inferior frontal gyrus was negatively correlated to changed verbal memory score (r = 0.526, p = 0.016, FDR correction) in the patients. But the medication dosage was not significantly correlated with the clinical symptom score FC value changes (all p > 0.05, Supplementary Table 1). Details are displayed in Figure 5.
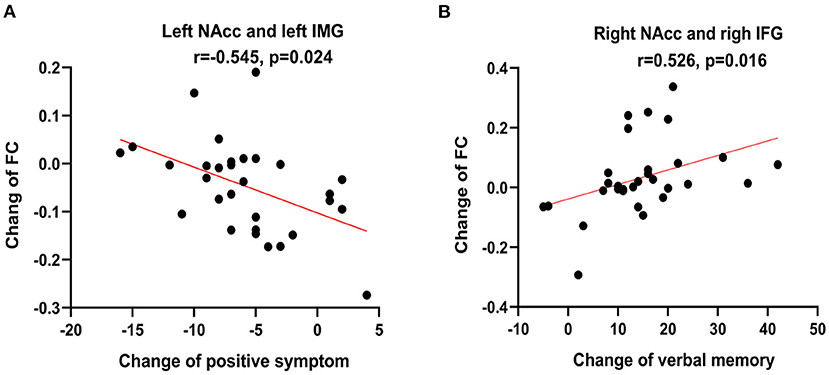
Figure 5. Correlations analysis showed that the changed functional connectivity (FC) value of left nucleus accumbens (NAcc) with the left inferior temporal gyrus (IMG) is negatively correlated with the change of positive symptom score of PNASS (r = −0.545, p = 0.024, False discovery rate correction) (A) and FC value of the right NAcc with the right inferior frontal gyrus (IFG) is positively correlated with the change of verbal memory score (r = 0.526, p = 0.016, False discovery rate correction) (B).
Discussion
In the present study, we investigated FC and GMV alternations of NAcc when schizophrenia patients with AVH received low-frequency rTMS treatment. Our findings demonstrated the patients at baseline had abnormal FC of NAcc with the temporal, frontal, and anterior cingulate cortices and decreased GMV in left NACC compared to controls. Although low-frequency rTMS did not affect the volumetric changes of NAcc, the abnormal FC patterns of NAcc with the temporal and frontal cortices were reversed in patients after treatment. The alternations of FC patterns were associated with clinical improvements in patients. These findings suggested that the NAcc may play an important role in the underlying pathology of schizophrenia and contribute to the effect of low-frequency rTMS on schizophrenia patients with AVH.
Our results indicated that patients at baseline had higher FC of NAcc with the temporal lobes (left middle temporal gyrus, left inferior temporal, and right fusiform gyrus) compared to control. These regions represent the speech processing areas (46, 47) and are known to be associated with AVH (48). Neuroimaging studies have indicated that auditory hallucinations are associated with hyperactivity in the auditory language cortex (49–51). Increased metabolism of temporal lobes has been reported in schizophrenia patients (52) and was related to positive symptoms (53). The hyperactive FC between NAcc and temporal lobes might be involved in an impaired function in speech perceptions and could be associated with the poor functional outcomes of patients with AVH. Higher FC between the NAcc and the temporal lobes appearing in schizophrenia patients with AVH was agreed with the previous report (19, 20), which might suggest a functional deficit of langue processing in the striatum-related circuits.
Decreased FC of NAcc with the frontal cortices (e.g., right superior frontal gyrus and inferior frontal gyrus) and anterior cingulate gyrus was also observed in patients at baseline relative to controls. The results are consistent with previous studies that reported hypoconnectivity of the frontostriatal loop in schizophrenia (18, 54). Specifically, Broca's region and its right hemisphere counterpart in the inferior frontal gyrus are involved in language processing (55, 56). There is common activation between the inferior frontal gyrus and NAcc during cognitive task processing (57) and decreased tract connections between them in schizophrenia (58, 59). In addition, the anterior cingulate gyrus is a critical area to integrate cognitive control processes (e.g., error monitoring) (60–62). Dysfunction of this region is found in schizophrenia (63) and may involve the misattribution of external sources of speech (64). The decreased FC of NAcc may partly explain the cognitive control deficits in patients that are characteristic of the clinical manifestations of schizophrenia, since the NAcc is implicated in cognitive functions, including memory, motivation, and decision-making (65) and is a virtual interface for information transmission between cortical and subcortical structures (66). Therefore, this hypoconnectivity of the NAcc circuit may lead to impairments of langue processing in schizophrenia.
However, these abnormal FC patterns of NAcc were normalized or inversed in patients after rTMS treatment. Several studies have indicated that low-frequency rTMS can increase the contribution of connected regions associated with auditory hallucinations (40, 41) due to long-lasting neuroplastic changes derived from the rTMS. Thus, the clinical effect of low-frequency rTMS on AVH may be associated with the reduction of hyperactivity in the auditory language cortex and relevant areas that propagate through remote pathways. Consistent with the hypothesis, we observed that initial increased FC between the NAcc and left inferior temporal gyrus in patients were inversed after treatment, which supports the long-term depression phenomenon induced by low-frequency rTMS (67). The inhibitory effect may shift from the target site to adjacent regions (e.g., NAcc) since there are well-established projections between them (68). This beneficial alternation may lead to the induced spread of the physiological effect in the auditory language circuit and may play an indirect modulatory effect on the NAcc connection loops, which could be associated with the reduction of clinical symptoms (e.g., positive symptom).
In addition, we found that the decreased FC of NAcc with the right inferior frontal gyrus was reversed in patients after rTMS treatment. Induced metabolic alternation in the frontal cortex by the low-frequency rTMS has been reported in schizophrenia with AVH (52). This alternation could be due to the induction of integration of frontotemporal disconnection that is documented in schizophrenia (69, 70). Studies have indicated that the NAcc connected with the inferior frontal gyrus (20) and TMS over the frontal cortex can induce dopamine and glutamate changes in the NAcc (71). These findings suggest that low-frequency rTMS could have a modulatory effect on neurotransmitters released in the NAcc through the remote effects of stimulation at the interconnected regions and thus could be associated the neurocognitive improvements such as verbal learning and memory.
Structural abnormalities in NAcc have been consistently demonstrated in schizophrenia. Two meta-analyses studies showed significant reductions in NAcc volume in patients with schizophrenia (16, 72). There is evidence from studies in adolescents (73) and adults (74) that NACC volumes are larger in the left but not in the right hemisphere. However, we found a significantly smaller volume in the left NACC in patients compared to controls. This finding was consistent with the previous studies that deficit schizophrenia patients displayed smaller left NAcc volumes compared to controls (75) and may reflect the changes in structural asymmetries in the schizophrenia brain. Although we did not find any volumetric changes in NAcc in patients after rTMS treatment, the asymmetry of NAcc presented in schizophrenia may represent the alternations in specific deep gray matter nuclei associated with an endophenotype of schizophrenia with AVH.
Some limitations of the present stud should be considered. Firstly, the sample size was small and limited the statistical power. Future studies should consider collecting larger datasets to improve the statistical power. Secondly, patients enrolled in this study were under stable antipsychotic medication treatment, and the impact of antipsychotic medication on FC of the NAcc should be taken into account, although no correlations were found between medication and FC alterations of NAcc in patients. Finally, the absence of placebo sham stimuli may lead to caution about the efficacy of the stimulus paradigm.
Conclusions
In summary, our findings revealed abnormal FC and GMV changes of NAcc in patients and suggested an involvement of the striatal pathway in schizophrenia with AVH. Moreover, the abnormal FC patterns of the NAcc were inversed by low-frequency rTMS treatment and could be biomarkers of the clinical effectiveness of low-frequency rTMS treatment in schizophrenia with AVH.
Data availability statement
The original contributions presented in the study are included in the article/Supplementary material, further inquiries can be directed to the corresponding author/s.
Ethics statement
The studies involving human participants were reviewed and approved by the Medical Ethics Committee of the Xijing Hospital. The patients/participants provided their written informed consent to participate in this study.
Author contributions
YX, MG, and ZW design and organized the research. YX, YC, ZW, and PF collected the imaging and cognitive data. YX and PF analyzed the data. YX, YC, and MG wrote and revised the manuscript. PF and HW provided fund support. All authors contributed to the article and approved the submitted version.
Funding
This study was funded by the Natural Science Foundation of China (Nos. 81971255 and 81571651), Postdoctoral Science Foundation (2019M653963), and the Military Medical Science and Technology Youth Training Program of China (20QNPY049).
Conflict of interest
The authors declare that the research was conducted in the absence of any commercial or financial relationships that could be construed as a potential conflict of interest.
Publisher's note
All claims expressed in this article are solely those of the authors and do not necessarily represent those of their affiliated organizations, or those of the publisher, the editors and the reviewers. Any product that may be evaluated in this article, or claim that may be made by its manufacturer, is not guaranteed or endorsed by the publisher.
Supplementary material
The Supplementary Material for this article can be found online at: https://www.frontiersin.org/articles/10.3389/fpsyt.2022.971105/full#supplementary-material
References
1. Tandon R, Keshavan MS, Nasrallah HA. Schizophrenia, “just the facts” what we know in 2008. Epidemiol Etiol Schizophr Res. (2008) 102:1–18. doi: 10.1016/j.schres.2008.04.011
2. Mikell CB, McKhann GM, Segal S, McGovern RA, Wallenstein MB, Moore H. The hippocampus and nucleus accumbens as potential therapeutic targets for neurosurgical intervention in schizophrenia. Stereotact Funct Neurosurg. (2009) 87:256–65. doi: 10.1159/000225979
3. Miyake N, Thompson J, Skinbjerg M, Abi-Dargham A. Presynaptic dopamine in schizophrenia. CNS Neurosci Ther. (2011) 17:104–9. doi: 10.1111/j.1755-5949.2010.00230.x
4. Davis KL, Kahn RS, Ko G, Davidson M. Dopamine in schizophrenia: a review and reconceptualization. Am J Psychiatry. (1991) 148:1474–86. doi: 10.1176/ajp.148.11.1474
5. Kapur S. Psychosis as a state of aberrant salience: a framework linking biology, phenomenology, and pharmacology in schizophrenia. Am J Psychiatry. (2003) 160:13–23. doi: 10.1176/appi.ajp.160.1.13
6. Lum JS, Millard SJ, Huang X-F, Ooi L, Newell KA. A postmortem analysis of NMDA ionotropic and group 1 metabotropic glutamate receptors in the nucleus accumbens in schizophrenia. J Psychiatry Neurosci. (2018) 43:102–10. doi: 10.1503/jpn.170077
7. Abi-Dargham A, Rodenhiser J, Printz D, Zea-Ponce Y, Gil R, Kegeles Lawrence S, et al. Increased baseline occupancy of D2 receptors by dopamine in schizophrenia. Proc Nat Acad Sci USA. (2000) 97:8104–9. doi: 10.1073/pnas.97.14.8104
8. Xu S, Gullapalli RP, Frost DO. Olanzapine antipsychotic treatment of adolescent rats causes long term changes in glutamate and GABA levels in the nucleus accumbens. Schizophr Res. (2015) 161:452–7. doi: 10.1016/j.schres.2014.10.034
9. Britt JP, Benaliouad F, McDevitt RA, Stuber GD, Wise RA, Bonci A. Synaptic and behavioral profile of multiple glutamatergic inputs to the nucleus accumbens. Neuron. (2012) 76:790–803. doi: 10.1016/j.neuron.2012.09.040
10. Mackay AVP, Iversen LL, Rossor M, Spokes E, Bird E, Arregui A, et al. Increased brain dopamine and dopamine receptors in schizophrenia. Arch Gen Psychiatry. (1982) 39:991–7. doi: 10.1001/archpsyc.1982.04290090001001
11. Memo M, Kleinman Joel E, Hanbauer I. Coupling of dopamine D1 recognition sites with aenylate cyclase in nuclei accumbens and caudatus of schizophrenics. Science. (1983) 221:1304–7. doi: 10.1126/science.6310753
12. Hadar R, Soto-Montenegro ML, Götz T, Wieske F, Sohr R, Desco M, et al. Using a maternal immune stimulation model of schizophrenia to study behavioral and neurobiological alterations over the developmental course. Schizophr Res. (2015) 166:238–47. doi: 10.1016/j.schres.2015.05.010
13. Luchicchi A, Lecca S, Melis M, De Felice M, Cadeddu F, Frau R, et al. Maternal immune activation disrupts dopamine system in the offspring. Int J Neuropsychopharmacol. (2016) 19:1–10. doi: 10.1093/ijnp/pyw007
14. Meehan C, Harms L, Frost JD, Barreto R, Todd J, Schall U, et al. Effects of immune activation during early or late gestation on schizophrenia-related behaviour in adult rat offspring. Brain Behav Immun. (2017) 63:8–20. doi: 10.1016/j.bbi.2016.07.144
15. Bois C, Levita L, Ripp I, Owens DCG, Johnstone EC, Whalley HC, et al. Hippocampal, amygdala and nucleus accumbens volume in first-episode schizophrenia patients and individuals at high familial risk: a cross-sectional comparison. Schizophr Res. (2015) 165:45–51. doi: 10.1016/j.schres.2015.03.024
16. Okada N, Fukunaga M, Yamashita F, Koshiyama D, Yamamori H, Ohi K, et al. Abnormal asymmetries in subcortical brain volume in schizophrenia. Mol Psychiatry. (2016) 21:1460–6. doi: 10.1038/mp.2015.209
17. van Erp TGM, Hibar DP, Rasmussen JM, Glahn DC, Pearlson GD, Andreassen OA, et al. Subcortical brain volume abnormalities in 2028 individuals with schizophrenia and 2540 healthy controls via the ENIGMA consortium. Mol Psychiatry. (2016) 21:547–53. doi: 10.1038/mp.2015.63
18. Lin P, Wang X, Zhang B, Kirkpatrick B, Öngür D, Levitt JJ, et al. Functional dysconnectivity of the limbic loop of frontostriatal circuits in first-episode, treatment-naive schizophrenia. Hum Brain Mapp. (2018) 39:747–57. doi: 10.1002/hbm.23879
19. Potvin S, Dugre JR, Fahim C, Dumais A. Increased connectivity between the nucleus accumbens and the default mode network in patients with schizophrenia during cigarette cravings. J Dual Diagn. (2019) 15:8–15. doi: 10.1080/15504263.2018.1526432
20. Rolland B, Amad A, Poulet E, Bordet R, Vignaud A, Bation R, et al. Resting-state functional connectivity of the nucleus accumbens in auditory and visual hallucinations in schizophrenia. Schizophr Bull. (2015) 41:291–9. doi: 10.1093/schbul/sbu097
21. Deutch AY, Cameron DS. Pharmacological characterization of dopamine systems in the nucleus accumbens core and shell. Neuroscience. (1992) 46:49–56. doi: 10.1016/0306-4522(92)90007-O
22. Deutch AY, Lee MC, Iadarola MJ. Regionally specific effects of atypical antipsychotic drugs on striatal Fos expression: the nucleus accumbens shell as a locus of antipsychotic action. Mol Cell Neurosci. (1992) 3:332–41. doi: 10.1016/1044-7431(92)90030-6
23. Rummel-Kluge C, Komossa K, Schwarz S, Hunger H, Schmid F, Lobos CA, et al. Head-to-head comparisons of metabolic side effects of second generation antipsychotics in the treatment of schizophrenia: a systematic review and meta-analysis. Schizophr Res. (2010) 123:225–33. doi: 10.1016/j.schres.2010.07.012
24. He H, Lu J, Yang L, Zheng J, Gao F, Zhai Y, et al. Repetitive transcranial magnetic stimulation for treating the symptoms of schizophrenia: a PRISMA compliant meta-analysis. Clin Neurophysiol. (2017) 128:716–24. doi: 10.1016/j.clinph.2017.02.007
25. Kennedy NI, Lee WH, Frangou S. Efficacy of non-invasive brain stimulation on the symptom dimensions of schizophrenia: a meta-analysis of randomized controlled trials. Eur Psychiatry. (2018) 49:69–77. doi: 10.1016/j.eurpsy.2017.12.025
26. Li J, Cao X, Liu S, Li X, Xu Y. Efficacy of repetitive transcranial magnetic stimulation on auditory hallucinations in schizophrenia: a meta-analysis. Psychiatry Res. (2020) 290:113141. doi: 10.1016/j.psychres.2020.113141
27. Nathou C, Etard O, Dollfus S. Auditory verbal hallucinations in schizophrenia: current perspectives in brain stimulation treatments. Neuropsychiatr Dis Treat. (2019) 15:2105–17. doi: 10.2147/NDT.S168801
28. Slotema CW, Blom JD, van Lutterveld R, Hoek HW, Sommer IEC. Review of the efficacy of transcranial magnetic stimulation for auditory verbal hallucinations. Biol Psychiatry. (2014) 76:101–10. doi: 10.1016/j.biopsych.2013.09.038
29. Bais L, Vercammen A, Stewart R, van Es F, Visser B, Aleman A, et al. Short and long term effects of left and bilateral repetitive transcranial magnetic stimulation in schizophrenia patients with auditory verbal hallucinations: a randomized controlled trial. PLoS ONE. (2014) 9:e108828. doi: 10.1371/journal.pone.0108828
30. Slotema CW, Blom JD, de Weijer AD, Hoek HW, Sommer IE. Priming does not enhance the efficacy of 1 Hertz repetitive transcranial magnetic stimulation for the treatment of auditory verbal hallucinations: results of a randomized controlled study. Brain Stimul. (2012) 5:554–9. doi: 10.1016/j.brs.2011.10.005
31. Dollfus S, Lecardeur L, Morello R, Etard O. Placebo response in repetitive transcranial magnetic stimulation trials of treatment of auditory hallucinations in schizophrenia: a meta-analysis. Schizophr Bull. (2016) 42:301–8. doi: 10.1093/schbul/sbv076
32. Silbersweig DA, Stern E, Frith C, Cahill C, Holmes A, Grootoonk S, et al. A functional neuroanatomy of hallucinations in schizophrenia. Nature. (1995) 378:176–9. doi: 10.1038/378176a0
33. Raij TT, Valkonen-Korhonen M, Holi M, Therman S, Lehtonen J, Hari R. Reality of auditory verbal hallucinations. Brain. (2009) 132:2994–3001. doi: 10.1093/brain/awp186
34. Shergill SS, Bullmore E, Simmons A, Murray R, McGuire P. Functional anatomy of auditory verbal imagery in schizophrenic patients with auditory hallucinations. Am J Psychiatry. (2000) 157:1691–3. doi: 10.1176/appi.ajp.157.10.1691
35. Zhou C, Xue C, Chen J, Amdanee N, Tang X, Zhang H, et al. Altered functional connectivity of the nucleus accumbens network between deficit and non-deficit schizophrenia. Front Psychiatry. (2021) 12:704631. doi: 10.3389/fpsyt.2021.704631
36. Sampedro F, Roldán A, Alonso-Solís A, Grasa E, Portella MJ, Aguilar EJ, et al. Grey matter microstructural alterations in schizophrenia patients with treatment-resistant auditory verbal hallucinations. J Psychiatr Res. (2021) 138:130–8. doi: 10.1016/j.jpsychires.2021.03.037
37. Kay SR, Fiszbein A, Opler LA. The positive and negative syndrome scale (PANSS) for schizophrenia. Schizophr Bull. (1987) 13:261–76. doi: 10.1093/schbul/13.2.261
38. Hoffman RE, Hawkins KA, Gueorguieva R, Boutros NN, Rachid F, Carroll K, et al. Transcranial magnetic stimulation of left temporoparietal cortex and medication-resistant auditory hallucinations. Arch Gen Psychiatry. (2003) 60:49–56. doi: 10.1001/archpsyc.60.1.49
39. Shi C, Kang L, Yao S, Ma Y, Li T, Liang Y, et al. The MATRICS Consensus Cognitive Battery (MCCB): co-norming and standardization in China. Schizophr Res. (2015) 169:109–15. doi: 10.1016/j.schres.2015.09.003
40. Bais L, Liemburg E, Vercammen A, Bruggeman R, Knegtering H, Aleman A. Effects of low frequency rTMS treatment on brain networks for inner speech in patients with schizophrenia and auditory verbal hallucinations. Progr Neuropsychopharmacol Biol Psychiatry. (2017) 78:105–13. doi: 10.1016/j.pnpbp.2017.04.017
41. Vercammen A, Knegtering H, den Boer JA, Liemburg EJ, Aleman A. Auditory hallucinations in schizophrenia are associated with reduced functional connectivity of the temporo-parietal area. Biol Psychiatry. (2010) 67:912–8. doi: 10.1016/j.biopsych.2009.11.017
42. Ashburner J. A fast diffeomorphic image registration algorithm. Neuroimage. (2007) 38:95–113. doi: 10.1016/j.neuroimage.2007.07.007
43. Friston KJ, Williams S, Howard R, Frackowiak RSJ, Turner R. Movement-related effects in fMRI time-series. Magn Reson Med. (1996) 35:346–55. doi: 10.1002/mrm.1910350312
44. Rolls ET, Huang C-C, Lin C-P, Feng J, Joliot M. Automated anatomical labelling atlas 3. Neuroimage. (2020) 206:116189. doi: 10.1016/j.neuroimage.2019.116189
45. Woods SW. Chlorpromazine equivalent doses for the newer atypical antipsychotics. J Clin Psychiatry. (2003) 64:663–7. doi: 10.4088/JCP.v64n0607
46. Hickok G. The functional neuroanatomy of language. Phys Life Rev. (2009) 6:121–43. doi: 10.1016/j.plrev.2009.06.001
47. Turkeltaub PE, Eden GF, Jones KM, Zeffiro TA. Meta-analysis of the functional neuroanatomy of single-word reading: method and validation. Neuroimage. (2002) 16:765–80. doi: 10.1006/nimg.2002.1131
48. Diederen KMJ, Daalman K, de Weijer AD, Neggers SFW, van Gastel W, Blom JD, et al. Auditory hallucinations elicit similar brain activation in psychotic and nonpsychotic individuals. Schizophr Bull. (2012) 38:1074–82. doi: 10.1093/schbul/sbr033
49. Bohlken MM, Hugdahl K, Sommer IEC. Auditory verbal hallucinations: neuroimaging and treatment. Psychol Med. (2017) 47:199–208. doi: 10.1017/S003329171600115X
50. Cleghorn JM, Garnett ES, Nahmias C, Brown GM, Kaplan RD, Szechtman H, et al. Regional brain metabolism during auditory hallucinations in chronic schizophrenia. Br J Psychiatry. (1990) 157:562–70. doi: 10.1192/bjp.157.4.562
51. Copolov DL, Seal ML, Maruff P, Ulusoy R, Wong MTH, Tochon-Danguy HJ, et al. Cortical activation associated with the experience of auditory hallucinations and perception of human speech in schizophrenia: a PET correlation study. Psychiatry Res Neuroimaging. (2003) 122:139–52. doi: 10.1016/S0925-4927(02)00121-X
52. Horacek J, Brunovsky M, Novak T, Skrdlantova L, Klirova M, Bubenikova-Valesova V, et al. Effect of low-frequency rTMS on electromagnetic tomography (LORETA) and regional brain Metabolism (PET) in schizophrenia patients with auditory hallucinations. Neuropsychobiology. (2007) 55:132–42. doi: 10.1159/000106055
53. Paulman RG, Devous MD, Gregory RR, Herman JH, Jennings L, Bonte FJ, et al. Hypofrontality and cognitive impairment in schizophrenia: dynamic single-photon tomography and neuropsychological assessment of schizophrenic brain function. Biol Psychiatry. (1990) 27:377–99. doi: 10.1016/0006-3223(90)90549-H
54. Zhang B, Lin P, Wang X, Öngür D, Ji X, Situ W, et al. Altered functional connectivity of striatum based on the integrated connectivity model in first-episode schizophrenia. Front Psychiatry. (2019) 10:756. doi: 10.3389/fpsyt.2019.00756
55. Hagoort P, Levelt Willem JM. The speaking brain. Science. (2009) 326:372–3. doi: 10.1126/science.1181675
56. Saur D, Kreher Björn W, Schnell S, Kümmerer D, Kellmeyer P, Vry M-S, et al. Ventral and dorsal pathways for language. Proc Nat Acad Sci USA. (2008) 105:18035–40. doi: 10.1073/pnas.0805234105
57. Matthews SC Simmons AN Lane SD and Paulus MP. Selective activation of the nucleus accumbens during risk-taking decision making. Neuroreport. (2004) 15:2123–7. doi: 10.1097/00001756-200409150-00025
58. de Leeuw M, Bohlken MM, Mandl RCW, Kahn RS, Vink M. Reduced fronto–striatal white matter integrity in schizophrenia patients and unaffected siblings: a DTI study. npj Schizophrenia. (2015) 1:15001. doi: 10.1038/npjschz.2015.1
59. James A, Joyce E, Lunn D, Hough M, Kenny L, Ghataorhe P, et al. Abnormal frontostriatal connectivity in adolescent-onset schizophrenia and its relationship to cognitive functioning. Eur Psychiatry. (2016) 35:32–8. doi: 10.1016/j.eurpsy.2016.01.2426
60. Kerns JG, Cohen JD, MacDonald AW, Johnson MK, Stenger VA, Aizenstein H, et al. Decreased conflict- and error-related activity in the anterior cingulate cortex in subjects with schizophrenia. Am J Psychiatry. (2005) 162:1833–9. doi: 10.1176/appi.ajp.162.10.1833
61. Paus T. Primate anterior cingulate cortex: where motor control, drive and cognition interface. Nat Rev Neurosci. (2001) 2:417–24. doi: 10.1038/35077500
62. Walton ME, Croxson PL, Behrens TEJ, Kennerley SW, Rushworth MFS. Adaptive decision making and value in the anterior cingulate cortex. Neuroimage. (2007) 36:T142–54. doi: 10.1016/j.neuroimage.2007.03.029
63. Fornito A, Yücel M, Dean B, Wood SJ, Pantelis C. Anatomical abnormalities of the anterior cingulate cortex in schizophrenia: bridging the gap between neuroimaging and neuropathology. Schizophr Bull. (2009) 35:973–93. doi: 10.1093/schbul/sbn025
64. Mechelli A, Allen P, Amaro JrE, Fu CH, Williams SC, Brammer MJ. et al. Misattribution of speech and impaired connectivity in patients with auditory verbal hallucinations. Hum. Brain Mapp. (2007) 28:1213–22. doi: 10.1002/hbm.20341
65. West EA, Moschak TM, Carelli RM. Distinct functional microcircuits in the nucleus accumbens underlying goal-directed decision-making. In: Morris R, Bornstein A, Shenhav A, editors. Goal-Directed Decision Making. Cambridge, MA: Academic Press (2018). p. 199–219.
66. Dürschmid S, Zaehle T, Kopitzki K, Voges J, Schmitt F, Heinze H-J, et al. Phase-amplitude cross-frequency coupling in the human nucleus accumbens tracks action monitoring during cognitive control. Front Hum Neurosci. (2013) 7:635. doi: 10.3389/fnhum.2013.00635
67. Pascual-Leone A, Tarazona F, Keenan J, Tormos JM, Hamilton R, Catala MD. Transcranial magnetic stimulation and neuroplasticity. Neuropsychologia. (1998) 37:207–17. doi: 10.1016/S0028-3932(98)00095-5
68. Cauda F, Cavanna AE, D'Agata F, Sacco K, Duca S, Geminiani GC. Functional connectivity and coactivation of the nucleus accumbens: a combined functional connectivity and structure-based meta-analysis. J Cogn Neurosci. (2011) 23:2864–77. doi: 10.1162/jocn.2011.21624
69. Stevens AA, Goldman-Rakic PS, Gore JC, Fulbright RK, Wexler BE. Cortical dysfunction in schizophrenia during auditory word and tone working memory demonstrated by functional magnetic resonance imaging. Arch Gen Psychiatry. (1998) 55:1097–103. doi: 10.1001/archpsyc.55.12.1097
70. Yurgelun-Todd DA, Waternaux CM, Cohen BM, Gruber SA, English CD, Renshaw PF. Functional magnetic resonance imaging of schizophrenic patients and comparison subjects during word production. Am J Psychiatry. (1996) 153:200–5. doi: 10.1176/ajp.153.2.200
71. Zangen A, Hyodo K. Transcranial magnetic stimulation induces increases in extracellular levels of dopamine and glutamate in the nucleus accumbens. Neuroreport. (2002) 13:2401–5. doi: 10.1097/00001756-200212200-00005
72. Tang Y, Wang M, Zheng T, Yuan F, Yang H, Han F, et al. Grey matter volume alterations in trigeminal neuralgia: a systematic review and meta-analysis of voxel-based morphometry studies. Progr Neuropsychopharmacol Biol Psychiatry. (2020) 98:109821. doi: 10.1016/j.pnpbp.2019.109821
73. Dennison M, Whittle S, Yücel M, Vijayakumar N, Kline A, Simmons J, et al. Mapping subcortical brain maturation during adolescence: evidence of hemisphere- and sex-specific longitudinal changes. Dev Sci. (2013) 16:772–91. doi: 10.1111/desc.12057
74. Ahsan RL, Allom R, Gousias IS, Habib H, Turkheimer FE, Free S, et al. Volumes, spatial extents and a probabilistic atlas of the human basal ganglia and thalamus. Neuroimage. (2007) 38:261–70. doi: 10.1016/j.neuroimage.2007.06.004
Keywords: schizophrenia, auditory verbal hallucination, nucleus accumbent, functional connectivity, gray matter volume, repetitive transcranial magnetic stimulation
Citation: Xie Y, Cai Y, Guan M, Wang Z, Ma Z, Fang P and Wang H (2022) The alternations of nucleus accumbent in schizophrenia patients with auditory verbal hallucinations during low-frequency rTMS treatment. Front. Psychiatry 13:971105. doi: 10.3389/fpsyt.2022.971105
Received: 16 June 2022; Accepted: 16 August 2022;
Published: 06 September 2022.
Edited by:
Wenbin Guo, Second Xiangya Hospital, Central South University, ChinaReviewed by:
Xiao Chang, King's College London, United KingdomYongbin Wei, Beijing University of Posts and Telecommunications (BUPT), China
Copyright © 2022 Xie, Cai, Guan, Wang, Ma, Fang and Wang. This is an open-access article distributed under the terms of the Creative Commons Attribution License (CC BY). The use, distribution or reproduction in other forums is permitted, provided the original author(s) and the copyright owner(s) are credited and that the original publication in this journal is cited, in accordance with accepted academic practice. No use, distribution or reproduction is permitted which does not comply with these terms.
*Correspondence: Yuanjun Xie, xieyuanj@gmail.com; Peng Fang, fangpeng@fmmu.edu.cn