- 1Department of Otorhinolaryngology, Tianyou Hospital, Affiliated to Wuhan University of Science and Technology, Wuhan, China
- 2Department of Medical Imaging, Tianyou Hospital, Affiliated to Wuhan University of Science and Technology, Wuhan, China
- 3Department of Stomatology, Tianyou Hospital, Affiliated to Wuhan University of Science and Technology, Wuhan, China
Objective: While prior reports have characterized visible changes in neuroimaging findings in individuals suffering from sudden sensorineural hearing loss (SSNHL), the utility of regional homogeneity (ReHo) as a means of diagnosing SSNHL has yet to be established. The present study was thus conducted to assess ReHo abnormalities in SSNHL patients and to establish whether these abnormalities offer value as a diagnostic neuroimaging biomarker of SSNHL through a support vector machine (SVM) analysis approach.
Methods: Resting-state functional magnetic resonance imaging (rs-fMRI) analyses of 27 SSNHL patients and 27 normal controls were conducted, with the resultant imaging data then being analyzed based on a combination of ReHo and SVM approaches.
Results: Relative to normal control individuals, patients diagnosed with SSNHL exhibited significant reductions in ReHo values in the left cerebellum, bilateral inferior temporal gyrus (ITG), left superior temporal pole (STP), right parahippocampal gyrus (PHG), left posterior cingulum cortex (PCC), and right superior frontal gyrus (SFG). SVM analyses suggested that reduced ReHo values in the left cerebellum were associated with high levels of diagnostic accuracy (96.30%, 52/54), sensitivity (92.59%, 25/27), and specificity (100.00%, 27/27) when distinguishing between SSNHL patients and control individuals.
Conclusion: These data suggest that SSNHL patients exhibit abnormal resting-state neurological activity, with changes in the ReHo of the left cerebellum offering value as a diagnostic neuroimaging biomarker associated with this condition.
Introduction
Sudden sensorineural hearing loss (SSNHL) is a medical emergency wherein affected individuals present with sensorineural hearing loss (≥30 dB over ≥3 consecutive frequencies) of unknown origin within 3 days. SSNHL is often accompanied by symptoms including tinnitus, vertigo, and aural fullness (1). SSNHL is estimated to affect 5–27 per 100,000 persons, and its annual incidence continues to rise (2, 3). Just 5% of SSNHL cases are bilateral, with most patients exhibiting a unilateral loss of hearing without any side preference (1, 4). Although the most common suspected etiologies of SSNHL in adult patients include viral infection, vascular or hematologic disease, immune-mediated disease, tumors, trauma, and other causes, the precise etiology of SSNHL remains unclear (5). SSNHL can contribute to social difficulties and psychiatric disorders in certain cases (6), with SSNHL patients exhibiting a higher risk of depression, and depression patients similarly exhibiting increased odds of developing SSNHL (7). As such, a failure to rapidly diagnose and treat SSNHL can lead to permanent hearing loss and associated adverse effects on quality of life (8). A combination of medical history information and pure tone audiometry (PTA) is generally used to diagnose SSNHL. However, imageological approaches capable of diagnosing SSNHL are lacking.
Rapid advances in neuroimaging technologies in recent years have enabled the diagnostic evaluation of a range of neurological systems. For example, increases in the fractional amplitude of low-frequency fluctuation in the right precuneus and left superior frontal gyrus may offer value as a biomarker associated with first-episode major depressive disorder (MDD) incidence (9). Abnormal network homogeneity values of the right posterior cingulate cortex/precuneus have also been successfully used to differentiate between individuals diagnosed with obsessive-compulsive disorder and control individuals with respective sensitivity and specificity values of 67.50% and 76.32% (10). Notably, several imaging studies have reported functional and structural changes in certain brain functional networks in SSNHL patients during the acute phase (≤ 30 days) (6, 11–13). However, neuroimaging biomarkers that can be used to guide SSNHL patients diagnosis have not been reported to date.
Resting-state functional magnetic resonance imaging (rs-fMRI) is a sensitive, non-invasive imaging approach that can offer detailed insight regarding altered brain function and neuronal activity. Accordingly, rs-fMRI imaging is commonly used for the evaluation of tinnitus patients and individuals suffering from bilateral or unilateral sensorineural hearing loss (14–17). Regional homogeneity (ReHo) is a robust algorithmic approach that enables the quantification of the resting-state local synchronization of adjacent voxels (18, 19), thereby providing insight regarding the consistency of whole-brain neural activity patterns. Abnormal ReHo detected via rs-fMRI in particular brain regions may be indicative of aberrant spontaneous neural activity among and within these areas of the brain. Specifically, increased ReHo values correspond to improved neuronal synchrony, whereas reduced ReHo values indicate impaired local neuronal activity.
While the use of rs-fMRI approaches to study SSNHL is becoming increasingly common, no studies to date have utilized a combination of ReHo and support vector machine (SVM) approaches to analyze these rs-fMRI-derived data. SVM approaches rely on the use of a robust machine learning algorithm capable of analyzing data, recognizing patterns, and using the resultant insights to gauge diagnostic accuracy. By identifying the maximal margin separating a hyperplane, this SVM algorithm maintains enhanced generalizability and resists overfitting, providing optimal predictive accuracy for test data that have not yet been analyzed. By overlaying SVM-derived weight values onto the original brain space utilized for fMRI analyses, the areas of the brain that can most effectively differentiate between different groups of individuals can be identified (20). As SVM algorithms can effectively locate and differentiate patterns within a particular dataset, the interpretability of the associated model is improved (20). SVM analyses are ideally suited to high-dimensional datasets in which there are more features than there are samples, with multiple prior reports having achieved success in the use of SVM to identify brain states (20, 21), enabling the discrimination between individuals diagnosed with particular neurological disorders and healthy controls (9, 22–25). Whether ReHo abnormalities can be effectively employed to differentiate between individuals with and without SSNHL through an SVM analysis, however, remains to be assessed. As such, the present study was designed to explore ReHo in patients with SSNHL, to establish which brain regions exhibit abnormal SSNHL-related ReHo, and to employ an SVM approach to gauge the value of abnormal ReHo as a neuroimaging biomarker of SSNHL.
Methods
Subjects
Between August 2020 and December 2021, a total of 27 patients diagnosed with SSNHL and 27 normal controls from the Otolaryngology Head and Neck Surgery Department of Tianyou Hospital affiliated with Wuhan University of Science and Technology were enrolled in this study. Participants were eligible for enrollment if they exhibited: (a) ≥30 dB in ≥3 contiguous frequencies with an air-bone gap <10 dB, as measured via PTA; (b) a new-onset case of SSNHL with no prior history of this condition; (c) hearing loss of unknown origin; (d) no known neurological disease; (e) MRI and CT imaging results that excluded the presence of any space-occupying lesions within the intracranial space or internal auditory canal. SSNHL patients were excluded from this study if they (a) had any history of noise exposure, ototoxic drug use, or ear surgery; (b) were experiencing fluctuating hearing loss; (c) had a family history of neurological disease; or (d) exhibited any inflammation of the external or middle ear. All enrolled normal control subjects exhibited normal otoscopic tympanic membrane findings and their pure-tone air conduction thresholds <25 dB HL at 0.25, 0.5, 1, 2, 4, and 8 kHz. Control participants were also free of any history of neurological or otologic disease. Pure-tone thresholds at 0.25, 0.5, 1, 2, 4, and 8 kHz were recorded at the start and the end of treatment. The Institutional Review Board of Tianyou Hospital Ethics Committee approved this study, and all participants provided informed consent.
Image acquisition
An Ingenia 3.0 T MRI scanner (Philips, Amsterdam, Netherlands) was used to analyze all study subjects in the Department of Medical Imaging. Participants were positioned with their heads being placed within a foam-filled prototype quadrature birdcage head coil designed to limit motion. During fMRI scanning, participants were directed to remain still and awake with their eyes close. Scanning parameters were: TR = 2000 ms, TE = 30 ms, flip angle = 90°, FOV = 220 × 220 mm2, matrix size = 64 × 64, slice gap = 0.7 mm, slice thickness =3.5 mm, slice number = 33, and pitch = 1 mm.
Data preprocessing
MATLAB was used for the pre-processing of rs-fMRI data with the DPARSF software (26). Initially, the first five time points for each participant were excluded from analysis to reduce initial signal instability and to ensure that participants had sufficient time to adapt to the imaging. Samples were then corrected for head motion and slice time. Participants were not included in subsequent analyses if they exhibited over 2 mm of maximal displacement in any of the x, y, or z directions or over 2° of maximal rotation. Corrected imaging data were then subjected to spatial normalization based upon the standard Montreal Neurological Institute space, followed by resampling at 3 × 3 × 3 mm3. Images were then subjected to bandpass filtering (0.01–0.08 Hz) and linear detrending. Spurious covariates were then removed, including six head motion parameters derived from rigid body correction, signal from a region centered in the white matter, and signal derived from a ventricular seed-based region of interest.
ReHo analysis
ReHo analyses were conducted using the DPABI software (http://rfmri.org/dpabi). Kendall coefficients of the time series consistency between individual voxels and neighboring voxels were used to construct ReHo brain maps, with smoothing then being performed using a Gaussian kernel with a full width and half height of 4 mm to minimize the impact of noise and deformation on the standardization process, thereby improving image effects, signal-to-noise ratio values, and statistical efficiency.
Classification analysis
The MATLAB LIBSVM package was used to conduct SVM classification analyses in an effort to establish the ability of ReHo values extracted from abnormal regions of the brain to differentiate between SSNHL patients and normal control individuals. This analytical approach was performed using a leave-one-out technique.
Statistical analyses
Demographic and clinical data
Statistical analyses were performed using SPSS 22.0, with demographic data being compared between groups using chi-square tests (sex) and two-sample t-tests (age). Hearing levels were compared via the Wilcoxon test. P < 0.05 was the threshold of significance.
ReHo values
The DPABI software was used to perform two-sample t-test analyses of ReHo graphs for the patient and normal control groups, with age and sex being treated as covariates. Brain templates were selected for overlay, and analyses were subjected to Gaussian Random Field (GRF) correction with a correction threshold of P < 0.01. Those brain regions exhibiting significant differences between these two groups were extracted as a template mask, with ReHo values for individual subjects then being extracted based on this template.
Results
Patient characteristics
In total, this study incorporated 27 patients diagnosed with unilateral SSNHL and 27 normal controls. There were no significant differences between these groups with respect to the age or sex of participants (P > 0.05), while there were significant differences between pre- and post-treatment hearing levels among SSNHL patients (P < 0.05). For further details regarding participant characteristics, see Table 1.
SSNHL-related ReHo abnormalities
SSNHL patients exhibited significant reductions in ReHo in the left cerebellum, bilateral inferior temporal gyrus (ITG), left superior temporal pole (STP), right parahippocampal gyrus (PHG), left posterior cingulum cortex (PCC), and right superior frontal gyrus (SFG) relative to normal controls (Figure 1, Table 2).
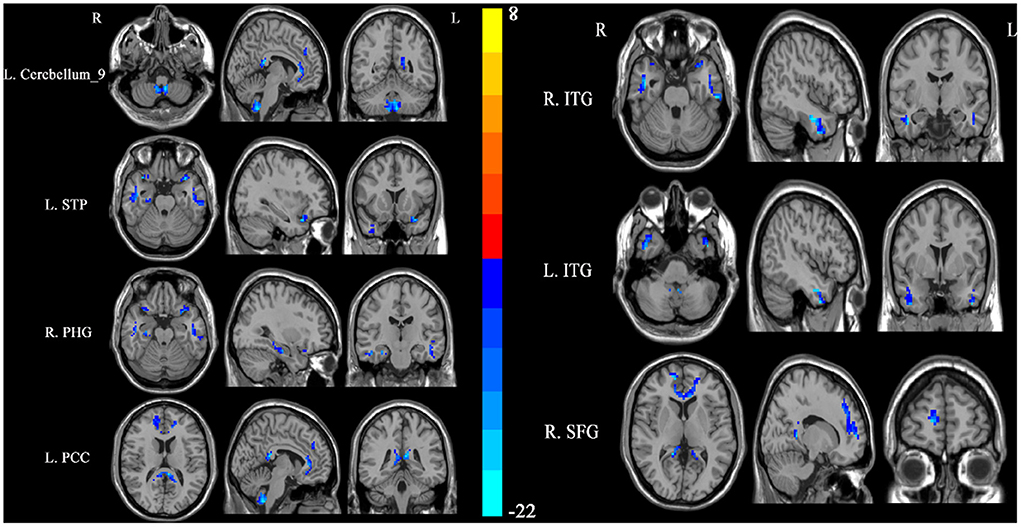
Figure 1. Differences in regional homogeneity (ReHo) values between SSNHL patients and normal controls. Decreased ReHo values (left cerebellum, left STP, right PHG, left PCC, right SFG, and bilateral ITG) were presented on the blue color, and the color bar indicates the T values of the group analysis. L, left; R, right; STP, superior temporal pole; PHG, parahippocampal gyrus; PCC, posterior cingulum cortex; SFG, superior frontal gyrus; ITG, inferior temporal gyrus.
SVM analysis results
An SVM approach was used to separately analyze abnormal ReHo values in seven regions of the brain (left cerebellum, bilateral ITG, left STP, right PHG, left PCC, and right SFG), revealing decreased ReHo in the left cerebellum to exhibit the highest diagnostic accuracy (96.30%, 52/54) when differentiating between SSNHL patients and normal controls, with a sensitivity of 92.59% (25/27) and a specificity of 100.00% (27/27) (Figure 2).
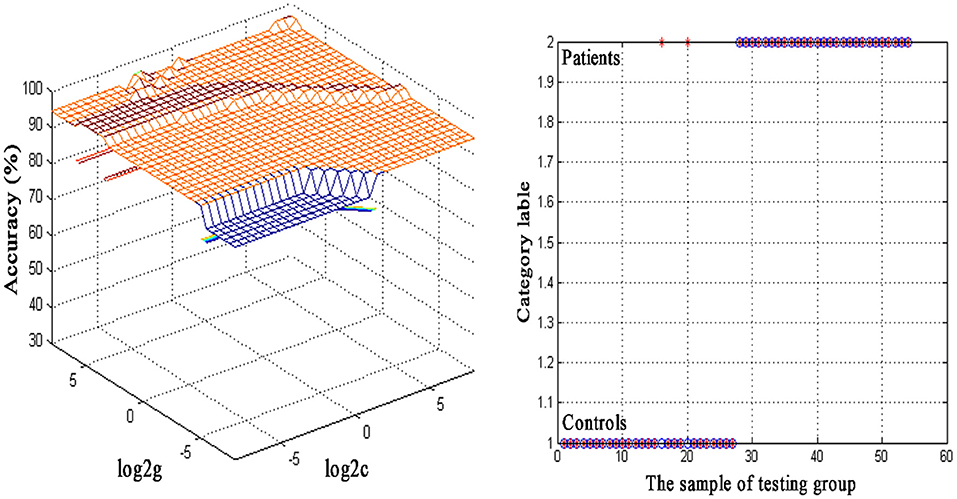
Figure 2. Visualization of classifications through support vector machine (SVM) using the decreased regional homogeneity (ReHo) values in the left cerebellum to discriminate SSNHL patients from normal controls. Left: SVM parameters result of 3D view. g means gamma, c means penalty coefficient. Right: Classified map of the ReHo values in the left cerebellum. Blue circle means true value and the red asterisk means predict value.
Discussion
Here, whole-brain ReHo was assessed at rest in both SSNHL patients and normal control individuals. Significant reductions in ReHo values were observed in the left cerebellum, bilateral ITG, left STP, right PHG, left PCC, and right SFG of SSNHL patients during the acute hearing loss period relative to control individuals. These data suggest that these seven regions of the brain exhibit abnormal spontaneous neural activity in individuals affected by acute-phase SSNHL. SVM analyses further indicated that reductions in ReHo in the left cerebellum may offer value as a neuroimaging biomarker that can distinguish between patients with SSNHL and unaffected controls.
The reduced ReHo of seven brain regions in SSNHL may present the baseline abnormality of sensory cortices in SSNHL at rest. Recent work suggests that the cerebellum plays a central role in the coordination of emotional, sensory, and cognitive processes (27, 28). Moreover, the cerebellum mediates the processing of acoustic information derived from auditory-associated brain regions (29). Xu et al. (15) determined that patients suffering from long-term moderately severe bilateral sensorineural hearing loss exhibit atypical patterns of spontaneous neural activity within the cerebellum, in line with the results of this present study. Here, decreased ReHo of the left cerebellum may reflect abnormal brain function in sensory and cognitive information processing in SSNHL patients, and an adaptation to engage other sensory systems as a compensatory mechanism for the acute hearing impairment. Thus, we speculated that the lack of sufficient acoustic input may have impaired the function of the cerebellum as reflected by reduced ReHo values in this brain region. The SVM analysis results for this region further yielded an accuracy of 96.30% (52/54), suggesting that reductions in left cerebellar ReHo values may offer utility as a putative neuroimaging biomarker that can aid in diagnosing SSNHL.
The ITG has been found to play a role in multiple functional brain networks associated with emotional regulation, language comprehension, memory, and visual processing (30–32). Much like the ITG, the temporal pole is a component of the association complex associated with emotion, language processing, and the multimodal integration of sensory inputs (33–37), and both the STP and ITG are components of the temporal lobe. Therefore, abnormal activity of the two brain regions could influence the function of the temporal lobe. The temporal lobe is the location of the auditory center, and it is also involved in functions relating to emotion, speech, balance, memory, and visual perception. Thus, SSNHL could lead to structural and functional impairment of the temporal lobe. The PHG is a center that is reportedly associated with higher-order cognitive functions such as visual-spatial processing and the encoding/retrieval of memories (38, 39). Lin et al. (38) conducted a tractography analysis that confirmed the existence of extensive cortico-cortical correlations between the PHG and other regions of the brain including the occipital, parietal, temporal, and frontal cortices. The temporal pole has also been regarded as a component of the parahippocampal region in humans as well as in non-human primates (39, 40). As such, the ITG, STP, and PHG are closely related to one another both functionally and structurally. Multiple reports have identified abnormal changes in the PHG and ITG in individuals suffering from hearing loss. Yang et al. (41) determined that individuals affected by long-term right-sided unilateral hearing loss exhibit significantly lower gray matter volumes in the right PHG, left superior/middle/inferior temporal gyrus, bilateral PCC, and precuneus. Chen et al. (42) also observed lower ReHo values in the precuneus, PHG, superior temporal gyrus, and inferior parietal lobe in presbycusis patients. In the present analysis, SSNHL patients exhibited resting-state ReHo abnormalities in the STP, ITG, and PHG, thus suggesting a role for these regions in the pathophysiology of this condition.
The PCC is a component of the posteromedial cortex located in the medial portion of the inferior parietal lobe (43). As a key default mode network (DMN) component, the function of the PCC has been linked to cognition, emotional regulation, and the retrieval of episodic memories. The DMN consists of the medial prefrontal cortex, precuneus, inferior parietal lobe, and PCC (44), and plays roles in negative ruminations, memory retrieval, cognitive functioning, and self-related thoughts (44–46). Reductions in ReHo values in the PCC in the present analysis are likely reflective of altered spontaneous neural activity in this region and between the PCC and connected regions. The SFG, located in the superior prefrontal cortex, has previously been separated based on diffusion tensor tractography into the anteromedial, dorsolateral, and posterior SFG subregions by Li et al. (47), revealing both the anteromedial and dorsolateral SFG to be primarily connected with the DMN when conducting resting-state analyses of functional connectivity. The SFG is also an integral component of emotional processing, cognitive control, and working memory (48–51), and several reports have documented altered ReHo values in the SFG. Patients with MDD, for example, reportedly exhibit elevated ReHo values in the left SFG, medial superior frontal gyrus, and left middle temporal gyrus (52). Relative to healthy individuals, diabetic optic neuropathy patients also exhibit significant reductions in ReHo values in the SFG, left anterior cingulate, and right middle frontal gyrus (53). Diabetic vitreous hemorrhage patients also reportedly present with significant increases in bilateral SFG, bilateral cerebellar posterior lobes, and right superior/middle occipital gyrus ReHo values (54). However, few studies to date have explored SSNHL-related changes in ReHo values in the SFG. Here, SSNHL patients were found to exhibit significantly reduced ReHo values in the right SFG as compared to normal controls, consistent with the weakening of the consistency of spontaneous neural activity in this region. The observed ReHo abnormalities in the PCC and SFG may thus be suggestive of abnormal structures in the DMN in patients affected by SSNHL.
Prior research has revealed SSNHL incidence to be correlated with higher rates of affective disorders including depression and anxiety (55, 56). The results of this analysis suggest that acute auditory deprivation may alter spontaneous neuronal activity following SSNHL incidence, contributing to changes in higher-order brain functions. Together, these results may offer new insight regarding the underlying neuropathological mechanisms of SSNHL and associated alterations in higher-order brain functions.
The advent of novel imaging technologies has spurred a growing interest in the use of neuroimaging biomarkers as tools for the diagnosis and monitoring of a range of diseases and disorders. Gao et al. (25) reported that the increased degree centrality of the right inferior parietal lobule and the left dorsolateral superior frontal gyrus could be used as a combined imaging biomarker of right temporal lobe epilepsy (rTLE) with respective sensitivity and specificity values of 100% and 98.55%. Abnormal ReHo values in particular brain regions have also shown promise as a tool for differentiating between schizophrenia patients and healthy controls (57). This study, however, is the first to have employed an SVM approach to examine the utility of ReHo abnormalities as SSNHL-related neuroimaging biomarkers. ReHo is an indicator of local brain regions activity. SVM is a mature and effective algorithm in machine learning and it has successfully been used in prior reports to guide the diagnosis of first-episode MDD (9), schizophrenia (58), obsessive-compulsive disorder (10), and rTLE (25). Here, an SVM approach was used to analyze the diagnostic utility of abnormal ReHo values in the left cerebellum, bilateral ITG, left STP, right PHG, left PCC, and right SFG, revealing that the left cerebellum exhibited respective accuracy, sensitivity, and specificity values of 96.30%, 92.59%, and 100.00% when differentiating between SSNHL patients and normal controls.
There are certain limitations to this analysis. For one, the sample size was relatively limited. Additionally, scanner-derived noise could not be fully eliminated even though participants were provided with earplugs. Lastly, no analyses of the correlations between ReHo and clinical findings were performed.
Conclusion
In conclusion, these results suggest that reduced ReHo in the left cerebellum, bilateral ITG, left STP, right PHG, left PCC, and right SFG correspond to abnormal spontaneous brain activity in patients diagnosed with SSNHL. Moreover, decreases in ReHo within the left cerebellum may offer value as a neuroimaging biomarker that can aid in the diagnosis of SSNHL.
Data availability statement
The original contributions presented in the study are included in the article/supplementary material, further inquiries can be directed to the corresponding author.
Ethics statement
The studies involving human participants were reviewed and approved by the Institutional Review Board of Tianyou Hospital Ethics Committee. The patients/participants provided their written informed consent to participate in this study.
Author contributions
LL, JF, and HZ designed the experiment and wrote this article. JH, RC, XX, ST, and HR collected and analyzed these data. MT and QL guided the experiment and revised the article. All authors contributed to the article and approved the submitted version.
Funding
This research was supported by the grant from the Education Department of Hubei Province scientific research project (Grant No. D20201101).
Acknowledgments
We are grateful to all subjects for participating in the study.
Conflict of interest
The authors declare that the research was conducted in the absence of any commercial or financial relationships that could be construed as a potential conflict of interest.
Publisher's note
All claims expressed in this article are solely those of the authors and do not necessarily represent those of their affiliated organizations, or those of the publisher, the editors and the reviewers. Any product that may be evaluated in this article, or claim that may be made by its manufacturer, is not guaranteed or endorsed by the publisher.
References
1. Schreiber BE, Agrup C, Haskard DO, Luxon LM. Sudden sensorineural hearing loss. Lancet. (2010) 375:1203–11. doi: 10.1016/s0140-6736(09)62071-7
2. Kim SH, Kim SJ, Im H, Kim TH, Song JJ, Chae SW, et al. Trend in sudden sensorineural hearing loss: data from a population-based study. Audiol Neurootol. (2017) 22:311–6. doi: 10.1159/000485313
3. Alexander TH, Harris JP. Incidence of sudden sensorineural hearing loss. Otol Neurotol. (2013) 34:1586–9. doi: 10.1097/mao.0000000000000222
4. Oh JH, Park K, Lee SJ, Shin YR, Choung YH. Bilateral versus unilateral sudden sensorineural hearing loss. Otolaryngol—Head Neck Surg. (2007) 136:87–91. doi: 10.1016/j.otohns.2006.05.015
5. Chau JK, Lin JR, Atashband S, Irvine RA, Westerberg BD. Systematic review of the evidence for the etiology of adult sudden sensorineural hearing loss. Laryngoscope. (2010) 120:1011–21. doi: 10.1002/lary.20873
6. Chen J, Hu B, Qin P, Gao W, Liu C, Zi D, et al. Altered brain activity and functional connectivity in unilateral sudden sensorineural hearing loss. Neural Plast. (2020) 2020:9460364. doi: 10.1155/2020/9460364
7. Kim SY, Min C, Lee CH, Park B, Choi HG. Bidirectional relation between depression and sudden sensorineural hearing loss: two longitudinal follow-up studies using a national sample cohort. Sci Rep. (2020) 10:1482. doi: 10.1038/s41598-020-58547-w
8. Dallan I, Fortunato S, Casani AP, Bernardini E, Sellari-Franceschini S, Berrettini S, et al. Long-term follow up of sudden sensorineural hearing loss patients treated with intratympanic steroids: audiological and quality of life evaluation. J Laryngol Otol. (2014) 128:669–73. doi: 10.1017/s0022215114001595
9. Gao Y, Wang X, Xiong Z, Ren H, Liu R, Wei Y, et al. Abnormal fractional amplitude of low-frequency fluctuation as a potential imaging biomarker for first-episode major depressive disorder: a resting-state fmri study and support vector machine analysis. Front Neurol. (2021) 12:751400. doi: 10.3389/fneur.2021.751400
10. Chen Y, Ou Y, Lv D, Yang R, Li S, Jia C, et al. Altered network homogeneity of the default-mode network in drug-naive obsessive-compulsive disorder. Prog Neuropsychopharmacol Biol Psychiatry. (2019) 93:77–83. doi: 10.1016/j.pnpbp.2019.03.008
11. Li YT, Chen JW, Yan LF, Hu B, Chen TQ, Chen ZH, et al. Dynamic alterations of functional connectivity and amplitude of low-frequency fluctuations in patients with unilateral sudden sensorineural hearing loss. Neurosci Lett. (2022) 772:136470. doi: 10.1016/j.neulet.2022.136470
12. Wang Q, Chen Q, Liu P, Zhang J, Zhou L, Peng L. Functional magnetic resonance imaging reveals early connectivity changes in the auditory and vestibular cortices in idiopathic sudden sensorineural hearing loss with vertigo: a pilot study. Front Hum Neurosci. (2021) 15:719254. doi: 10.3389/fnhum.2021.719254
13. Fan W, Zhang W, Li J, Zhao X, Mella G, Lei P, et al. Altered contralateral auditory cortical morphology in unilateral sudden sensorineural hearing loss. Otol Neurotol. (2015) 36:1622–7. doi: 10.1097/mao.0000000000000892
14. Zhou GP, Shi XY, Wei HL, Qu LJ Yu YS, Zhou QQ, et al. Disrupted intraregional brain activity and functional connectivity in unilateral acute tinnitus patients with hearing loss. Front Neurosci. (2019) 13:1010. doi: 10.3389/fnins.2019.01010
15. Xu XM, Jiao Y, Tang TY, Zhang J, Lu CQ, Luan Y, et al. Dissociation between cerebellar and cerebral neural activities in humans with long-term bilateral sensorineural hearing loss. Neural Plast. (2019) 2019:8354849. doi: 10.1155/2019/8354849
16. Liu B, Feng Y, Yang M, Chen JY Li J, Huang ZC, et al. Functional connectivity in patients with sensorineural hearing loss using resting-state MRI. Am J Audiol. (2015) 24:145–52. doi: 10.1044/2015_aja-13-0068
17. Zhang GY, Yang M, Liu B, Huang ZC, Chen H, Zhang PP, et al. Changes in the default mode networks of individuals with long-term unilateral sensorineural hearing loss. Neuroscience. (2015) 285:333–42. doi: 10.1016/j.neuroscience.2014.11.034
18. Liu Y, Wang K, Yu C, He Y, Zhou Y, Liang M, et al. Regional homogeneity, functional connectivity and imaging markers of Alzheimer's disease: a review of resting-state fMRI studies. Neuropsychologia. (2008) 46:1648–56. doi: 10.1016/j.neuropsychologia.2008.01.027
19. Zang Y, Jiang T, Lu Y, He Y, Tian L. Regional homogeneity approach to fMRI data analysis. Neuroimage. (2004) 22:394–400. doi: 10.1016/j.neuroimage.2003.12.030
20. Shah YS, Hernandez-Garcia L, Jahanian H, Peltier SJ. Support vector machine classification of arterial volume-weighted arterial spin tagging images. Brain Behav. (2016) 6:e00549. doi: 10.1002/brb3.549
21. LaConte S, Strother S, Cherkassky V, Anderson J, Hu X. Support vector machines for temporal classification of block design fmri data. Neuroimage. (2005) 26:317–29. doi: 10.1016/j.neuroimage.2005.01.048
22. Shan X, Cui X, Liu F, Li H, Huang R, Tang Y, et al. Shared and distinct homotopic connectivity changes in melancholic and non-melancholic depression. J Affect Disord. (2021) 287:268–75. doi: 10.1016/j.jad.2021.03.038
23. Zhang X, Wang YT, Wang Y, Jung TP, Huang M, Cheng CK, et al. Ultra-slow frequency bands reflecting potential coherence between neocortical brain regions. Neuroscience. (2015) 289:71–84. doi: 10.1016/j.neuroscience.2014.12.050
24. Librenza-Garcia D, Kotzian BJ, Yang J, Mwangi B, Cao B, Pereira Lima LN, et al. The impact of machine learning techniques in the study of bipolar disorder: a systematic review. Neurosci Biobehav Rev. (2017) 80:538–54. doi: 10.1016/j.neubiorev.2017.07.004
25. Gao Y, Xiong Z, Wang X, Ren H, Liu R, Bai B, et al. Abnormal degree centrality as a potential imaging biomarker for right temporal lobe epilepsy: a resting-state functional magnetic resonance imaging study and support vector machine analysis. Neuroscience. (2022) 487:198–206. doi: 10.1016/j.neuroscience.2022.02.004
26. Karpiel I, Klose U, Drzazga Z. Optimization of rs-fMRI parameters in the seed correlation analysis (SCA) in DPARSF toolbox: a preliminary study. J Neurosci Res. (2019) 97:433–43. doi: 10.1002/jnr.24364
27. Baumann O, Borra RJ, Bower JM, Cullen KE, Habas C, Ivry RB, et al. Consensus paper: the role of the cerebellum in perceptual processes. Cerebellum. (2015) 14:197–220. doi: 10.1007/s12311-014-0627-7
28. Adamaszek M, D'Agata F, Ferrucci R, Habas C, Keulen S, Kirkby KC, et al. Consensus paper: cerebellum and emotion. Cerebellum. (2017) 16:552–76. doi: 10.1007/s12311-016-0815-8
29. Petacchi A, Laird AR, Fox PT, Bower JM. Cerebellum and auditory function: an ALE meta-analysis of functional neuroimaging studies. Hum Brain Mapp. (2005) 25:118–28. doi: 10.1002/hbm.20137
30. Lin YH, Young IM, Conner AK, Glenn CA, Chakraborty AR, Nix CE, et al. Anatomy and white matter connections of the inferior temporal gyrus. World Neurosurg. (2020) 143:e656–e66. doi: 10.1016/j.wneu.2020.08.058
31. Buckley MJ, Gaffan D, Murray EA. Functional double dissociation between two inferior temporal cortical areas: perirhinal cortex versus middle temporal gyrus. J Neurophysiol. (1997) 77:587–98. doi: 10.1152/jn.1997.77.2.587
32. Gao YJ, Wang X, Xiong PG, Ren HW, Zhou SY, Yan YG, et al. Abnormalities of the default-mode network homogeneity and executive dysfunction in people with first-episode, treatment-naive left temporal lobe epilepsy. Eur Rev Med Pharmacol Sci. (2021) 25:2039–49. doi: 10.26355/eurrev_202102_25108
33. Olson IR, Plotzker A, Ezzyat Y. The enigmatic temporal pole: a review of findings on social and emotional processing. Brain. (2007) 130(Pt 7):1718–31. doi: 10.1093/brain/awm052
34. Skipper LM, Ross LA, Olson IR. Sensory and semantic category subdivisions within the anterior temporal lobes. Neuropsychologia. (2011) 49:3419–29. doi: 10.1016/j.neuropsychologia.2011.07.033
35. Aust S, Alkan Härtwig E, Koelsch S, Heekeren HR, Heuser I, Bajbouj M. How emotional abilities modulate the influence of early life stress on hippocampal functioning. Soc Cogn Affect Neurosci. (2014) 9:1038–45. doi: 10.1093/scan/nst078
36. Hickok G, Poeppel D. The cortical organization of speech processing. Nat Rev Neurosci. (2007) 8:393–402. doi: 10.1038/nrn2113
37. Pehrs C, Zaki J, Schlochtermeier LH, Jacobs AM, Kuchinke L, Koelsch S. The temporal pole top-down modulates the ventral visual stream during social cognition. Cereb Cortex. (2017) 27:777–92. doi: 10.1093/cercor/bhv226
38. Lin YH, Dhanaraj V, Mackenzie AE, Young IM, Tanglay O, Briggs RG, et al. Anatomy and white matter connections of the parahippocampal gyrus. World Neurosurg. (2021) 148:e218–e26. doi: 10.1016/j.wneu.2020.12.136
39. Blaizot X, Mansilla F, Insausti AM, Constans JM, Salinas-Alamán A, Pró-Sistiaga P, et al. The human parahippocampal region: I. Temporal pole cytoarchitectonic and MRI correlation. Cereb Cortex. (2010) 20:2198–212. doi: 10.1093/cercor/bhp289
40. Insausti R, Amaral DG, Cowan WM. The entorhinal cortex of the monkey: II. Cortical afferents. J Compar Neurol. (1987) 264:356–95. doi: 10.1002/cne.902640306
41. Yang M, Chen HJ, Liu B, Huang ZC, Feng Y, Li J, et al. Brain structural and functional alterations in patients with unilateral hearing loss. Hear Res. (2014) 316:37–43. doi: 10.1016/j.heares.2014.07.006
42. Chen YC, Chen H, Jiang L, Bo F, Xu JJ, Mao CN, et al. Presbycusis disrupts spontaneous activity revealed by resting-state functional MRI. Front Behav Neurosci. (2018) 12:44. doi: 10.3389/fnbeh.2018.00044
43. Parvizi J, Van Hoesen GW, Buckwalter J, Damasio A. Neural connections of the posteromedial cortex in the Macaque. Proc Natl Acad Sci U S A. (2006) 103:1563–8. doi: 10.1073/pnas.0507729103
44. Gao Y, Wang M, Yu R, Li Y, Yang Y, Cui X, et al. Abnormal default mode network homogeneity in treatment-naive patients with first-episode depression. Front Psychiatry. (2018) 9:697. doi: 10.3389/fpsyt.2018.00697
45. Greicius MD, Krasnow B, Reiss AL, Menon V. Functional connectivity in the resting brain: a network analysis of the default mode hypothesis. Proc Natl Acad Sci U S A. (2003) 100:253–8. doi: 10.1073/pnas.0135058100
46. Gao Y, Zheng J, Li Y, Guo D, Wang M, Cui X, et al. Abnormal default-mode network homogeneity in patients with temporal lobe epilepsy. Medicine. (2018) 97:e11239. doi: 10.1097/md.0000000000011239
47. Li W, Qin W, Liu H, Fan L, Wang J, Jiang T, et al. Subregions of the human superior frontal gyrus and their connections. Neuroimage. (2013) 78:46–58. doi: 10.1016/j.neuroimage.2013.04.011
48. Alagapan S, Lustenberger C, Hadar E, Shin HW, Fröhlich F. Low-frequency direct cortical stimulation of left superior frontal gyrus enhances working memory performance. Neuroimage. (2019) 184:697–706. doi: 10.1016/j.neuroimage.2018.09.064
49. du Boisgueheneuc F, Levy R, Volle E, Seassau M, Duffau H, Kinkingnehun S, et al. Functions of the left superior frontal gyrus in humans: a lesion study. Brain. (2006) 129(Pt 12):3315–28. doi: 10.1093/brain/awl244
50. Andrews-Hanna JR, Smallwood J, Spreng RN. The default network and self-generated thought: component processes, dynamic control, and clinical relevance. Ann N Y Acad Sci. (2014) 1316:29–52. doi: 10.1111/nyas.12360
51. Raichle ME. The brain's default mode network. Annu Rev Neurosci. (2015) 38:433–47. doi: 10.1146/annurev-neuro-071013-014030
52. Liu S, Ma R, Luo Y, Liu P, Zhao K, Guo H, et al. Facial expression recognition and reho analysis in major depressive disorder. Front in Psychol. (2021) 12:688376. doi: 10.3389/fpsyg.2021.688376
53. Guo GY, Zhang LJ Li B, Liang RB, Ge QM, Shu HY, et al. Altered spontaneous brain activity in patients with diabetic optic neuropathy: a resting-state functional magnetic resonance imaging study using regional homogeneity. World J Diabetes. (2021) 12:278–91. doi: 10.4239/wjd.v12.i3.278
54. Zhang YQ, Zhu FY, Tang LY Li B, Zhu PW, Shi WQ, et al. Altered regional homogeneity in patients with diabetic vitreous hemorrhage. World J Diabetes. (2020) 11:501–13. doi: 10.4239/wjd.v11.i11.501
55. Kim JY, Lee JW, Kim M, Kim MJ, Kim DK. Association of idiopathic sudden sensorineural hearing loss with affective disorders. JAMA Otolaryngol Head Neck Surg. (2018) 144:614–21. doi: 10.1001/jamaoto.2018.0658
56. Arslan F, Aydemir E, Kaya YS, Arslan H, Durmaz A. Anxiety and depression in patients with sudden one-sided hearing loss. Ear Nose Throat J. (2018) 97:E7–10. doi: 10.1177/0145561318097010-1101
57. Iwabuchi SJ, Palaniyappan L. Abnormalities in the effective connectivity of visuothalamic circuitry in schizophrenia. Psychol Med. (2017) 47:1300–10. doi: 10.1017/s0033291716003469
Keywords: sudden sensorineural hearing loss, regional homogeneity, resting-state fMRI, support vector machine, neuroimaging biomarker
Citation: Liu L, Fan J, Zhan H, Huang J, Cao R, Xiang X, Tian S, Ren H, Tong M and Li Q (2022) Abnormal regional signal in the left cerebellum as a potential neuroimaging biomarker of sudden sensorineural hearing loss. Front. Psychiatry 13:967391. doi: 10.3389/fpsyt.2022.967391
Received: 12 June 2022; Accepted: 30 June 2022;
Published: 22 July 2022.
Edited by:
Qinji Su, Guangxi Medical University, ChinaReviewed by:
Qingguo Chen, Huazhong University of Science and Technology, ChinaYi Shao, Nanchang University, China
Copyright © 2022 Liu, Fan, Zhan, Huang, Cao, Xiang, Tian, Ren, Tong and Li. This is an open-access article distributed under the terms of the Creative Commons Attribution License (CC BY). The use, distribution or reproduction in other forums is permitted, provided the original author(s) and the copyright owner(s) are credited and that the original publication in this journal is cited, in accordance with accepted academic practice. No use, distribution or reproduction is permitted which does not comply with these terms.
*Correspondence: Miao Tong, Y2FrZS1sbEAxNjMuY29t; Qian Li, MTUzNTM3OTQ4NUBxcS5jb20=