- 1Telethon Kids Institute, Centre for Child Health Research, The University of Western Australia, Perth, WA, Australia
- 2School of Clinical Medicine, University of New South Wales, Sydney, NSW, Australia
- 3Department of Paediatrics, Monash University, Clayton, VIC, Australia
Aim: This systematic review aimed to identify the most important social, environmental, biological, and/or genetic risk factors for intellectual disability (ID).
Methods: Eligible were published prospective or retrospective comparative studies investigating risk factors for ID in children 4–18 years. Exclusions were single group studies with no comparator without ID and a sample size <100. Electronic databases (Medline, Cochrane Library, EMBASE, PsycInfo, Campbell Collaboration, and CINAHL) were searched for eligible publications from 1980 to 2020. Joanna Briggs Institute critical appraisal instruments, appropriate for study type, were used to assess study quality and risk of bias. Descriptive characteristics and individual study results were presented followed by the synthesis for individual risk factors, also assessed using GRADE.
Results: Fifty-eight individual eligible studies were grouped into six exposure topics: sociodemographic; antenatal and perinatal; maternal physical health; maternal mental health; environmental; genetic or biological studies. There were few eligible genetic studies. For half the topics, the certainty of evidence (GRADE) was moderate or high.
Conclusion: Multiple studies have examined individual potential determinants of ID, but few have investigated holistically to identify those populations most at risk. Our review would indicate that there are vulnerable groups where risk factors we identified, such as low socioeconomic status, minority ethnicity, teenage motherhood, maternal mental illness, and alcohol abuse, may cluster, highlighting a target for preventive strategies. At-risk populations need to be identified and monitored so that interventions can be implemented when appropriate, at preconception, during pregnancy, or after birth. This could reduce the likelihood of ID and provide optimal opportunities for vulnerable infants.
Systematic review registration: [https://www.crd.york.ac.uk/prospero/display_record.php?RecordID=120032], identifier [CRD42019120032].
Introduction
Intellectual disability (ID) is a neurodevelopmental disorder (NDD) characterized by impairments in cognitive function and adaptive functioning, manifest prior to the age of 18 years (1). The diagnosis of ID is generally made on the basis of intelligence quotient (IQ) scores, derived from standardized measures of intelligence (2). ID is defined as approximately two standard deviations or more below the population mean, which is equivalent to an IQ score of approximately 70 or less (1). Diagnosis also requires associated impairment in adaptive functioning (approximately two standard deviations or more below the population mean on standardized measures) and onset in childhood (2). The classification of severity of ID is often defined as mild, moderate, severe, or profound on the basis of IQ scores and the intensity of associated support needs (3). More recently, greater emphasis has been placed on the impact of ID on adaptive function (4). Accordingly, the Diagnostic and Statistical Manual for Mental Disorders—5th Edition (DSM-5), defines ID severity on the basis of adaptive functioning, rather than IQ scores alone (5).
The prevalence of ID varies between studies, geographical location, and time periods, impacted by different criteria and cutoff scores used to define ID (4). A recent meta-analysis across low-, middle-, and high-income countries reported an overall prevalence of 18.3/1,000 (6). One of the few population-based studies worldwide found that the prevalence of ID has risen over the last decade, from 14.3/1,000 between 1983 and 1992 (7) to 17.0/1,000 livebirths between 1983 and 2005 (2). This increase may be explained, at least in part, by the increase in the prevalence of autism spectrum disorder (ASD) over the same period, as up to 70% of individuals with a diagnosis of ASD had comorbid ID (2). The individual, family, and societal burden associated with ID is immense, with high associated health service needs (8–11).
The etiology of ID is poorly understood, and both genetic and environmental factors are implicated. In some cases, it is attributed to identifiable disorders, such as a genetic anomaly, for example, Down syndrome, or in utero exposure to infection or teratogens, such as alcohol. However, in 50–60% of cases (or more for mild ID), no associated genetic or medical disorder is identified (2, 7, 12–14). Individuals with ID also commonly present with additional neurodevelopmental problems and may also be diagnosed with ASD or cerebral palsy (CP) (15). Additional health conditions, such as epilepsy, often co-occur resulting in considerable heterogeneity in presentation.
Myriad social, biological, and genetic risk factors have been associated with ID. Demographic factors associated with an increased likelihood of ID include male sex (16), lower socioeconomic status (6, 17, 18), and ethnic minorities (2). Numerous pre- and perinatal factors have also been implicated, including advanced maternal age, high parity, maternal alcohol use, maternal tobacco use, gestational diabetes, maternal hypertension, preterm birth, and low birthweight (19). Other biological risks relate to factors, such as exposure to teratogens, viruses, or trauma (20). Genetic risk factors include a broad range of chromosomal abnormalities, autosomal trisomies, aneuploidies of the X-chromosome, and pathogenic copy number variants (14, 20).
At least some of the identifiable risk factors may be on the causal pathway for ID, either from biological perspectives, or as social determinants. Identification of known risk factors enables holistic consideration as to which factors may be modifiable. It has been shown that a small proportion of a childhood population can contribute to a large proportion of adult health burden (21). Similarly, it is possible that certain combinations of risk factors could disproportionally contribute to the burden of ID. This knowledge could be used to develop risk indices which can be applied on a population level to identify the most vulnerable sectors of the community, where there will be the most gain from early intervention but where there is the highest risk of non-participation in universal developmental surveillance (22, 23). The concept of proportionate universalism (24) provides a framework where universal services, both antenatally and into infancy, can be supplemented with additional services directed at those at highest risk. Thereby these populations can be supported in efforts to reduce the likelihood of ID or at least to reduce its impact. The purpose of this systematic review was thus to identify the most important social, environmental, biological, and/or genetic factors which will help to characterize these high-risk groups.
Methods
This systematic review was conducted and reported in accordance with the Preferred Reporting Items for Systematic Reviews and Meta-Analyses (PRISMA) Statement (25, 26). The study protocol was developed a priori and registered with PROSPERO (Registration number: CRD 42019120032).
Search strategy
The search range was 1980–2020 (initial search conducted in January 2019; with an extended search conducted in October 2020 of any eligible articles published between January 2019 and October 2020). The databases searched were Medline 1996—present (Ovid platform), Cochrane Library (Wiley platform), EMBASE, PsycInfo, Campbell Collaboration, and the Cumulative Index to Nursing and Allied Health Literature (CINAHL). This review sought to identify the breadth of potential risk factors for ID, including (but not limited to) individual child characteristics (biological/genetic risk factors), family characteristics (maternal age at birth, education, income, socioeconomic status, single-parent status, family size, parental mental health, and social capital/support), socio-cultural factors (membership to culturally and linguistically diverse populations, migrant populations, or indigenous populations), and other societal and geographical factors. Expander terms, such as MeSH headings and explode, were used to broaden the search in each database. The search terms and strategy are documented in Supplementary Table 1. Duplicates were removed, and abstracts were screened (ES, HL). The full texts of remaining articles were then assessed for eligibility against the inclusion and exclusion criteria (ES, HL, and EG). At each stage, for studies where eligibility was unclear, a collaborative decision was reached through discussion. Reasons for exclusion at the full-text stage were recorded. Included studies underwent a process of critical assessment (see quality assessment). The results of the search, including the numbers of articles at each stage and reasons for exclusion at the full-text stage, are presented in a PRISMA flow diagram (Figure 1).
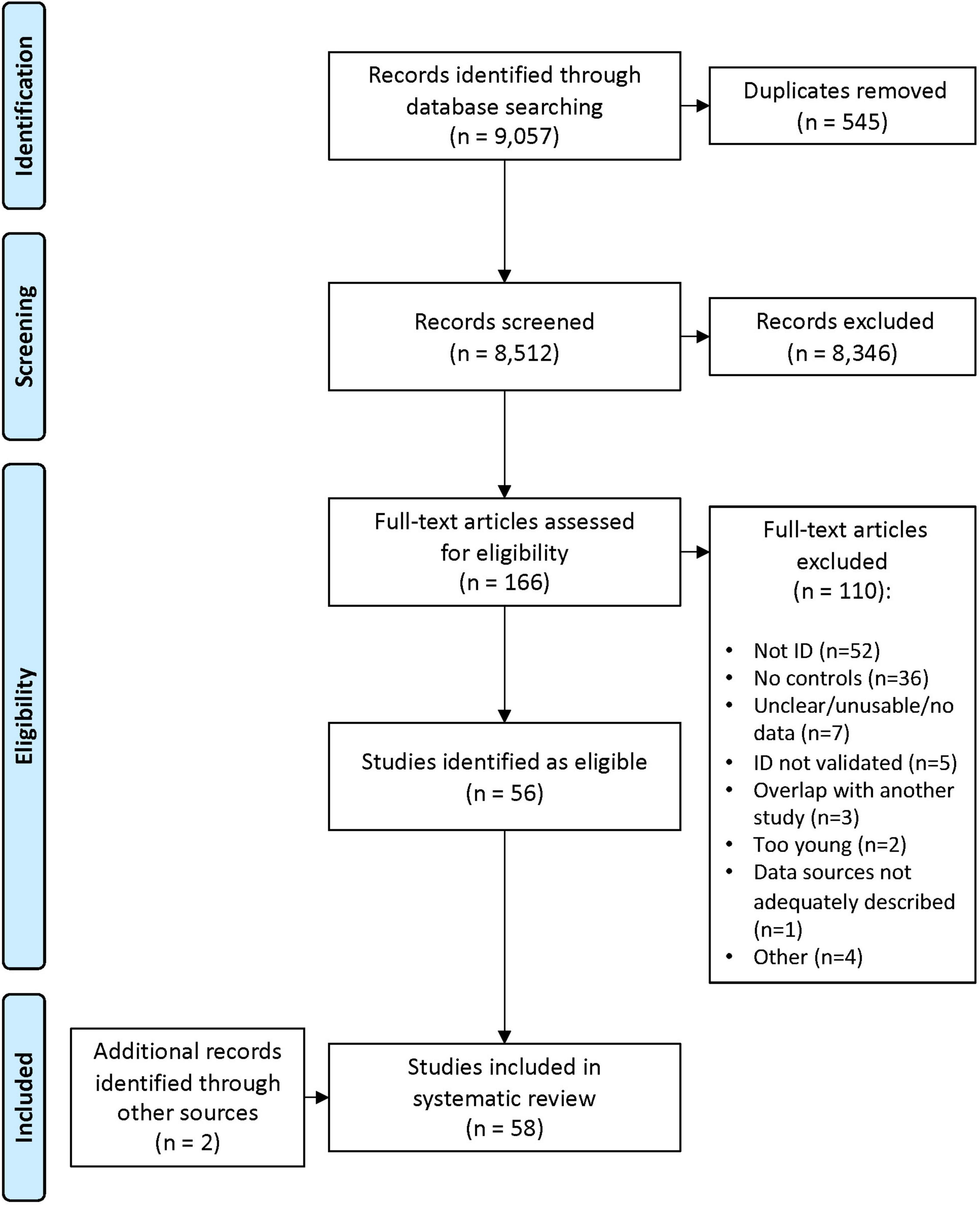
Figure 1. Preferred reporting items for systematic reviews and meta-analyses (PRISMA) flow diagram of the literature review and paper inclusion pathways.
Eligibility criteria
Published, peer-reviewed reports of prospective or retrospective comparative studies, including birth cohort studies, which examined risk factors for ID in children aged 4–18 years of age were included to ensure a valid IQ score could be obtained. For the purpose of this systematic review, ID was defined as an IQ of 70 or less (approximately two standard deviations below the mean), associated difficulties with adaptive functioning, and the condition manifesting prior to the age of 18 years. Articles that included individuals with ID and any other comorbid condition, including but not limited to autism, fetal alcohol spectrum disorder, cerebral palsy, epilepsy, and known genetic syndromes, were eligible. Articles including children with a diagnosis of developmental delay without ID diagnosis were not eligible. Where comorbid disorders were the focus of an article and individuals both with and without ID were included in the sample, articles were only included if subgroup data were presented, pertaining specifically to the subgroup with ID. The comparison group, or controls, were subjects without ID as defined above. Studies were excluded if they were single group studies with no comparator without ID, the sample size was less than 100, or the article was published before 1980.
The primary outcomes were factors that were identified to be significantly associated with the risk of ID in children and adolescents, as well as the strength of these associations. These determinants relate to individual, familial, biological, genetic, social, or environmental factors.
Data extraction
Data were extracted regarding the country, study design, data source and time period, sample size (including number with ID), method of assigning ID status and exclusions, comparison group, risk factors examined (and their categorization), covariates, analysis type, and risk factors identified. Data were extracted independently by three reviewers (ES, HL, and EG) using a standardized data extraction form. Any disagreements were discussed until a consensus was reached.
Quality assessment
All individual studies were critically appraised by two of seven independent assessors (VE, EG, HL, BM, AMa, AMo, and SW) at the study level for methodological quality using standardized critical appraisal instruments from the Joanna Briggs Institute (27). Three tools were used according to study type (cohort, cross-sectional, and case–control), for which each criterion was rated “Yes,” “No,” or “Unsure.” The “Yes” ratings were tallied for each study, and any disagreements were resolved through the input of a third assessor. Quality assessment ratings were standardized across study type by allocating a low, medium, or high level of quality for each study according to the percent of “Yes” ratings received (low < 75%, medium 75–89%, and high > 89%). Furthermore, using methodology similar to previous research (Supplementary Table 2) (28), we used the GRADE approach to assess the quality of evidence for specific outcomes as opposed to specific studies (as was done using the Joanna Briggs tools). We restricted GRADE assessments to those where the risk factors and levels of ID were comparable across studies. Given that for observational studies the maximum baseline level of evidence is considered to be moderate, a high level of certainty is difficult to achieve.
Data synthesis
We present descriptive characteristics of the individual studies in Table 1, which included type of study, data source, time period, sample size including number with ID, comparator group, criteria for assessing ID, and risk factors considered. Individual studies were categorized into six broad groups according to the main risk factors presented. In Table 2, we provide a summary of the findings of each individual study, as well as the type of analysis (e.g., logistic regression), outcome measure, covariates, unadjusted and adjusted results, and quality rating. Statistical outputs in the main included unadjusted and adjusted ratios and risk, including the odds ratio (OR, aOR), relative risk (RR, aRR), hazard ratio (HR, aHR), and population-attributable risk percent (PAR). Estimates and their 95% confidence intervals were presented where possible. One study only presented results using a relative risk ratio (RRR, aRRR) which is the ratio of two relative risks (29). Supplementary Table 3 provides further details for each study, including exposures, covariates, and category of ID. Table 3 presents data according to individual risk factor rather than by study, providing the opportunity to compare qualitatively across studies and provide a summary of the findings. This presentation style was adopted as a quantitative synthesis (or meta-analysis) was not feasible given the heterogeneity of the findings.
Results
Our initial search yielded a total of 8512 articles after exclusion of duplicates (see PRISMA flow diagram, Figure 1). After screening titles and abstracts, 166 full-text articles were retained and assessed for eligibility. An additional five articles were added from the extended time frame, and a further two articles were added from the reference lists of already included articles. Subsequently, 58 articles were included in the data extraction phase.
Study selection
Description of studies
Among the 58 studies (13, 29–85) included in this systematic review, there were 20 population-based cohort studies (13, 29, 36, 42, 45, 46, 56, 60–64, 66–68, 72, 77, 80, 82, 84), 20 other cohort studies (31, 34, 35, 37, 40, 44, 48–55, 59, 69, 71, 73, 81, 83), 14 case–control studies (32, 33, 39, 41, 47, 57, 58, 65, 70, 75, 76, 78, 79, 85), and four studies with cross-sectional study designs (30, 38, 43, 74) (Table 1). These studies were published from 1984 to 2020 and were predominantly based in North America (n = 33), Europe (n = 13), and Australia (n = 10).
The studies were initially grouped according to the main exposures into six themes (Tables 1, 2): (1) sociodemographic, (2) antenatal and perinatal, (3) maternal physical health, (4) maternal mental health, (5) environmental, (6) genetic or biological studies. For some studies, the exposures under investigation traversed more than one category (see Table 3).
The first group (n = 17) encompassed sociodemographic variables which included maternal employment, education, maternal ethnicity/race, marital status, socioeconomic status (including family income), geographical remoteness, country of birth, and age group. Sociodemographic variables pertaining to infants were sex and birth order. The second group (n = 14) which was classified as “antenatal and perinatal” included preterm birth, low birthweight, and intrauterine growth restriction but excluded maternal physical or mental health as these were included in separate categories. The third group, “maternal physical health” (n = 13), comprised among others smoking, obesity and diabetes (including gestational), pre-eclampsia and hypertension, epilepsy, urinary tract infection, and use of anti-epileptic medication. The fourth group, “maternal mental health” (n = 5), was classified as any mental health condition or specific groups, such as affective disorders including bipolar, schizophrenia, and maternal alcohol use disorder. The fifth group, “environmental” (n = 5), included air pollution, soil pollution, and seasonality. The sixth group only contained four “genetic or biological” studies.
In the first four categories, the majority were cohort studies (n = 34) usually incorporating data linkage (n = 30) whereas the comparison group was the population of individuals not affected with ID (Table 1). However, some studies, such as when cases were identified from the Metropolitan Atlanta Developmental Disabilities Study (MADDS) (75, 78, 79), used a case–control design and there was also a small number of cross-sectional studies (n = 4) (30, 38, 43, 74). The birth cohorts started as early as 1980 (29) and as late as 2011 (84) and had varying durations of follow-up, while children in the United States National Collaborative Perinatal Project (NCPP) were born from 1959 to 1974 and followed for 8 years (86). Children with ID were identified in a range of ways. In Western Australia (WA), it was through linkage to the WA IDEA (Intellectual Disability Exploring Answers) Database (87), in Atlanta through MADDS (78), in South Carolina through multiple sources including ICD codes in Medicaid billing records, the Department of Education and ID Support services (53). ID was generally defined as IQ < 70 on most recent psychometric test on scales, such as the Wechsler Intelligence Scale for Children (WISC) or Stanford Binet. In a substantial proportion of instances, children with a biomedical cause of ID were excluded, given that the risk factors under study would not be appropriate in these cases.
There was variability in the way ID severity was classified (4), for example, as “any ID,” “mild ID and severe ID,” or “mild-moderate ID and severe ID.” Few studies categorized ID as “mild” (IQ 50–55 to 69); “moderate” (IQ 35–40 to 40–54); or “severe (including profound)” (IQ < 35 or 40 according to DSM IV recommendations (American Psychiatric Association, 1994) (60). ID severity was more likely to be classified according to the International Classification of Diseases, Ninth Revision (ICD-9), as mild (IQ = 50–70), severe (IQ < 50), or unspecified (13). Some studies did not specify the level of ID. The unadjusted and adjusted results along with the relevant covariates are presented according to study in Table 2, and in Table 3 the results are presented according to risk factor given the overlap among studies. Some form of multinomial/multivariate logistic regression was used for analysis in most studies with most results presented as odds ratios although a few used Poisson (13, 37, 65) or Cox regression accounting for age at diagnosis (53, 59).
Risk factors
The results from the various categories of risk factors are presented in Table 3.
Sociodemographic factors
Using a range of different methods to assess socioeconomic status (SES) in Finland (3) (42, 58, 69), the United States (2) (75, 83), Australia (60), and United Kingdom and Norway (1 each) (38, 74), a clear relationship was identified between low SES and presence of ID in the offspring-mainly mild but also moderate or severe (OR range, 1.60–6.30). The consistency of the findings despite different methods provided moderate evidence of certainty. In Australia, a similar pattern was also seen with the offspring of single compared with partnered mothers [OR 2.18, (95% CI, 1.97–2.42)] (60). In studies from the United States (3) (13, 43, 75), Australia (2) (60, 64), Finland (1) (58), and China (1) (30), the increased risk of mild and severe ID for males was demonstrated (OR range, 1.23–1.90) with high evidence of certainty.
Lower maternal education conferred an increased risk of ID (both mild and severe) in the offspring from studies mostly undertaken over 20 years ago in the United States (75, 79, 82, 83). Despite differences in how maternal education was defined and how ID was categorized, the results were consistent across studies (OR range, 2.34–6.10). The findings were similar in a more recent study from China (30).
Both younger (<20 years) (82) and older (>35 years) (13, 75, 82) maternal age (with moderate certainty of evidence) were examined in United States studies and younger maternal age in Australia (60, 62). Children of younger mothers had an increased risk (RRR/OR range, 1.88–2.70) of ID (62, 82) as did offspring of older mothers (OR range, 1.40–3.88) (13, 33, 75, 82). There have been fewer studies investigating paternal age, but one Australian (62) study identified an increased risk [aOR 1.59 (95% CI, 1.36–1.86)] in offspring of fathers >39 years.
Maternal ethnicity/race was examined in five United States studies (13, 32, 33, 43, 75) and three Australian studies (29, 60, 62). We found higher proportions of children with ID in priority populations, including Black, Hispanic, and Indigenous groups, even after adjustment for SES or maternal education in most studies.
Antenatal and perinatal factors
Preterm birth was examined in a number of ways. One study compared the results for all ID with ID exclusive of a genetic cause although the ORs were similar for both groups (85). Another compared mild and severe according to the presence or absence of low birthweight (47) and another according to gestational age (68). Yet another compared IQ levels between those born preterm and those born at term (57). Despite the differing methodologies, the risks for ID overall were increased (OR range, 1.90–3.58) (40, 47, 64, 68, 85) and greater for severe ID (47) and at lower gestations (40, 68) contributing to the certainty of evidence.
Low birthweight was assessed in several of the same studies, and the findings were quite similar to those with preterm birth (OR range, 2.40–4.30) (40, 47, 85). The risk increased the lower the birthweight (40), was higher for severe than mild ID (47, 85) and generally consistent across studies, again providing high certainty of evidence. A number of studies also assessed the impact on ID of fetal growth restriction again by measuring in different ways (40, 58, 61, 64, 80, 85), including the use of the algorithm for percentage of optimal birthweight (POBW) (88). Growth restriction conferred increased risk of ID (OR range, 1.71–4.40) (40, 58, 61, 64, 80, 85).
Parental physical health
We only identified one study, published in 1996 and with low certainty of evidence, investigating the relationship between maternal smoking and ID in the offspring and finding a slightly increased risk with confidence limits overlapping unity (76). Six studies considered the impact either of pre-pregnancy obesity or diabetes of any type (including pre-gestational and gestational) and their combinations on the risk of ID in the offspring (53, 54, 59, 63, 64, 69). Pre-pregnancy or pregnancy obesity (54, 69), and maternal diabetes at any stage (53, 63, 64) conferred higher risk of ID in offspring (OR range 1.09–2.9), the latter with low evidence of certainty. However, the risks were not uniform across studies with risks being higher when there was pre-pregnancy morbid obesity (OR range, 1.52–3.63) (54, 59), or when autism was associated with ID (59, 63). Gestational maternal weight gain or loss was not associated with a greater risk (54).
As early as 1984, the first investigation of the relationship with pregnancy hypertension identified an increased risk of ID in the offspring [aRR 6.10, (95%CI, 1.30–28.90)] (41). An increased risk but with a lesser effect size [aOR 1.39, (95% CI, 1.25–1.54)] was found in a much more recent study providing very low evidence of certainty for pregnancy hypertension (64). This was very similar in magnitude to that obtained in another study focusing on pre-eclamptic toxemia (PET) (71).
In a 2006 Australian study, an increased risk of mild-moderate ID in children of mothers with epilepsy was first demonstrated [aOR 3.01, (95% CI, 2.10–4.33)] (63) and replicated again in a recent 2020 study [aHR 2.4, (95%CI, 1.8–3.1)] (36), providing a moderate certainty of evidence. There was no increased risk for the offspring of fathers with epilepsy (36). However, this recent study also focused on the risk posed by specific anti-seizure medications showing that the risk was highest with valproate and carbamazepine. An increased risk with valproate was also reported in another 2020 study (84).
Urinary tract infection during pregnancy was assessed in various ways in different studies, including the use of Medicaid (51) and prescription claims (49) and through midwife-collected data (63). All studies showed consistent but small (OR/RR < 1.65) increased risks of ID in the offspring providing moderate evidence of certainty.
Two studies investigated maternal anemia (63, 83), the United States study providing specific results for the Black population where an increased risk was detected (83). In the Australian study, the increased risk was specifically for severe ID [aOR 4.93, (95% CI, 2.00–12.09)] (63). A third study found an association between early childhood anemia and subsequent mild-to-moderate ID (67). Thus, the heterogeneity reduced the certainty of the evidence. Maternal asthma has only been studied using Australian data with a slight (28%) increased adjusted risk for ID in the offspring (63, 64) and maternal trichomonas during pregnancy in one United States study with a similarly small adjusted risk (55). However, no reason was found for downgrading the certainty of evidence. Two recent studies assessing mid-gestational cytokine and chemokine levels as markers of inflammation were not comparable and thus ineligible for GRADE analysis but provided fairly weak evidence of an increased risk of offspring ID despite some impact on developmental progress (65, 81).
Mental health
Five studies investigated the relationship between maternal mental health and risk of ID in the offspring, including four from Australia (45, 46, 72, 77) and one from the United States (34). All were published in the last decade, with most using birth cohort designs and data linkage. The striking findings were the increased risk of ID with any severe maternal mental illness [aOR 1.70, (95% CI, 1.50–1.90)] (77) with moderate evidence of certainty but specifically with schizophrenia [aOR 2.53, (95% CI, 1.40–4.50)] (72) and affective disorders, such as bipolar [aOR 2.60, (95% CI, 1.50–4.40)] (46) and unipolar depression [aOR 2.70, (95% CI, 1.60–4.50)] (46). The United States study identified maternal mental health as a high yield target for the prevention of ID (34). One of the Australian studies identified those women who had had a hospitalization for an alcohol-related disorder had an almost 3-fold increase in the adjusted odds [aOR 3.12, (95% CI, 2.13–4.56)] for their children compared with a matched cohort without such an admission, but the certainty of evidence was considered low (45).
Environmental factors
A comparatively smaller number of (30, 44, 48, 52, 56) studies investigated the impact of environmental factors on child ID. Three studies from North Carolina (44, 48, 52) estimated the soil concentrations of various metals in the areas close to where mothers were residing during their pregnancies. Increased concentrations of mercury, arsenic, and lead were most likely to be associated with ID in the children. A Scottish study investigated whether there was any relationship between seasonality of birth and subsequent ID by linking maternity with educational databases relating to 801,592 singleton children and found that rates were highest among children conceived in the first quarter of the year and lowest among those conceived in the third quarter (56). Geographical remoteness has been occasionally studied as a risk factor but may depend on location. In the Chinese study, there was a higher risk for children born in a rural setting (30) but such an association was not found in Australia (60, 62).
Quality appraisal and certainty of evidence
A high-quality assessment rating was given to 25 of the 58 studies (43.1%), a medium rating for 22 studies (37.9%), and a low rating for 11 studies (19.0%) using the Joanna Briggs Institute method. One common shortcoming was inadequate approaches toward confounding factors. For the case–control studies, lower scoring studies did not measure the exposures in a standard, valid, and reliable way or had a short exposure time. Cross-sectional studies with low-quality scores typically did not treat confounding factors adequately or did not have valid and reliable outcome measures.
The certainty of evidence was high for the studies investigating male gender, preterm birth, and low birthweight as risk factors for ID, partly because the magnitudes of association were large but also because there was often a dose response. It was moderate for the studies investigating socioeconomic status, race, maternal age and birth order, and maternal conditions, such as PET, epilepsy, asthma, trichomonas infection, urinary tract infection, and mental illness. Evidence for marital status, parental ID, maternal smoking, diabetes, hypertension, and depression as risk factors for ID was of low certainty.
Discussion
This comprehensive systematic review identified and confirmed a range of risk factors for ID. The greatest numbers of studies fell either into the sociodemographic or the pre- and perinatal domains. Fewer studies related to maternal physical health and fewer still to maternal mental health or environmental factors. We found very few eligible genetic studies given that the genetic risk factors for ID would be rarely studied in a comparison population.
Several of the studies investigating sociodemographic factors had been undertaken over 20 years ago using infrastructures, such as the MADDS (78) and the United States NCPP1 (83). One of the earliest data linkage studies included in this review was that undertaken in Florida in 2002 where birth records were linked to public school records (82). Despite the limitations of that time period, these studies were generally of a high quality and their findings have stood the test of time. Children of teenage (82), socially disadvantaged (75, 83), and multiparous (75) mothers were first identified as being at particular risk almost 20 years ago, findings which have been confirmed more recently in Australian (62) and United Kingdom (74) datasets. Despite other supporting evidence in relation to low maternal education level (82), with a PAR as high as 10%, it is not clear whether any public health approaches have been undertaken to reduce the risk of ID by recognizing and providing the additional support needed for this group of women (both prenatally and post-natally) and their children (72). Minority ethnicities, such as Indigenous, Blacks, and Hispanics, who are already at increased risk of psychosocial stressors, such as discrimination and poverty, are also over-represented among those with less access to education.
Preterm birth and low birthweight were identified as risk factors in a very early case–control study using the MADDS (47) and have continued to be investigated into more recent years (40, 68). A recent Swedish study sourcing data on singletons from the Stockholm Youth Cohort (birth years 1984–2011) demonstrated that the risk of ID increased substantially as birthweight decreased, as it also did when gestational age reduced (68). In contrast to an earlier Australian study which showed that the effect of growth restriction on subsequent ID was greater in term than preterm infants (61), the Stockholm study identified an interaction with growth restriction such that, as might be expected, those who were preterm and growth-restricted, had the highest risk of ID (68). In another recent Swedish study limited to infants born at >36-week gestation, a marginally greater effect size for risk of ID was found in those born at 37/38 weeks compared with 40 weeks (80). Complementing and building on our findings is another Swedish study published in 2022 which confirmed, as we had reported, that the risk of ID increases as gestational age falls, but also showed that the risk also increases again post-term (89). Again similar to our findings, the risk was greater with severe than with mild/moderate ID. Importantly, in all of these studies (40, 47, 61, 68, 80) including this most recent one (89) adjustment had been made for socioeconomic status and/or maternal education (40, 47, 61, 68, 80).
Evidence for the role of maternal physical and mental health as determinants of ID in the offspring has gradually been accumulating over the last two decades. There is a body of evidence building from Australia (63, 64), Finland (69), the United States (53, 54, 59), and now Sweden (90, 91) in relation to maternal obesity, pre-gestational, and gestational diabetes, all showing some level of increased risks. A recently published study focusing on the association of maternal diabetes with a range of neurodevelopmental outcomes has confirmed these relationships with the greatest odds associated with outcomes that included ID (90). Subsequent to this, another study has been published investigating the relationship between gestational weight gain and the risk of ID. This study found that inadequate gestational weight gain increased the risk as did excessive weight gain but only in women who were already obese and had a pre-pregnancy BMI > 25 kg/m2 (91). Yet much still remains unknown about the mechanisms underlying these findings and the opportunities for reducing these risks. Some associations with maternal urinary infection have also been demonstrated (49, 51, 63, 64), but there is likely a need for replication and further research given this could be another potentially modifiable factor. Although it is several years since first reported (63), maternal epilepsy and the association with ID and now particularly ASD in the offspring and the potential role of anti-seizure medications (92) is becoming of greater interest (93). The results of the Swedish study included in our review (36) have since been supported by a Danish study (93) with similar results. The risk has been shown to be greatest for women taking valproate during pregnancy but also increased to a lesser extent for those taking carbamazepine, oxcarbazepine, and clonazepam but not lamotrigine (93). This latest study does have implications for appropriate management of epilepsy in pregnancy to reduce the risk of ID through this avenue.
One study by Wang and colleagues in 2015 set out to identify risk factors among children with unknown cause of ID and estimate the PAR associated with these factors (34). This was a retrospective birth cohort study of 123,922 children born between 2004 and 2010 in South Carolina linked to Medicaid billing records and other data. All potential associations with ID were tabulated and the percentages with and without ID were compared. Odds ratios and PARs were calculated. In addition to other known factors, the study found elevated risks for maternal depression and bipolar disease, factors already identified in Australian data (46). Subsequent studies (72, 77) have further elaborated on these findings. What is not understood is the mechanism underlying these findings. There is much recent research investigating relationships between antidepressant medications and ASD (94, 95) but despite the positive associations, a causal link has not been firmly substantiated. Much less is known about such a relationship with ID (96). Although this could be a potential mechanism for our findings, other possibilities could include epigenetic and intergenerational effects, as postulated for ASD (97, 98).
Strengths and limitations of the reviewed studies
Given the comparatively low prevalence of ID, with just under 2% of children affected (2), the challenges associated with their identification (4), and the lengthy time (40 years) period of the review, studies were generally of medium-to-high quality with consistencies in the findings. In the absence of the existence of infrastructures, such as the WA IDEA Database (87) established especially for that purpose (99), many studies had used creative methods to identify these children in their respective birth cohorts (49, 56, 82) either using ICD codes from Medicaid claims or linking to education or disability services. Exemplifying the rigor of their research, many authors openly acknowledged the shortcomings of their studies, generally only a consequence of the limitations of the available data. For example, the populations for the South Carolina studies were limited to those covered by the Medicaid program which is only available to low-income families. Therefore, the authors explained that their results might not be generalizable to high-income families (53). Despite this, the results were generally consistent with those from WA where population-based data were used (64). Since the majority of studies were located in North America, Scandinavia, and Australia, factors of importance in low- and middle-income countries may not have been adequately represented. Furthermore, we did not identify any studies measuring biomarkers as numbers of cases and controls would likely be too low to meet our eligibility criteria given the prevalence of ID. For example, a study of neuropeptide and neurotrophin levels included only 60 children with ID and 54 control children (100).
In some studies, the type of educational placement was by necessity used as a surrogate for level of ID (67, 74, 82). A significant proportion of studies appropriately excluded ID of biomedical etiology, for example, Down syndrome from the outcome especially in recent studies, one of which specifically stipulated this in the title (68). Most errors associated with exposure misclassification would have resulted in non-differential misclassification and not systematic bias (68). The studies that did not include covariates tended either to be earlier (38, 42, 60, 82) and/or those where data were not available. Most studies reported adjusted odds ratios and some both crude and adjusted (see Table 2).
Strengths and weaknesses in relation to other studies
The methodology for this review involved a comparison of the presence of potential risk factors in an ID population with their presence in a control population with a sample size of >100 individuals. Thus, in this study, we were not investigating the generally rare but established causes of ID, many of which have a clear genetic etiology. Other than Down syndrome, the commonest known cause with a birth prevalence of ∼1/1,000 live births (101), other much rarer syndromes include Angelman, ARX, Coffin-Lowry syndrome, Cornelia de Lange, Fragile X, PCDH19, Prader–Willi, and Rett and Williams syndromes (102). In this study rather than identifying these individually rare disorders, we were investigating those determinants or risk factors that increase a child’s propensity to having an ID. These factors, which may act individually or in combination, may be modifiable and thus have an important potential to reduce the risk for a child or a population. Improved knowledge about these factors could help in future to identify children at particular risk from an early age, to minimize these risks, and to prioritize such populations for early intervention programs.
In contrast to ASD where over two-thirds of children have comorbid ID (2), reviews on the determinants of ID are comparatively sparse. Although the challenges and opportunities relating to the epidemiology of ID were reviewed in 2002 (4), there have been few subsequent reviews of the topic. In the 2015 study by Wang and colleagues (34), PARs were calculated for many of the factors identified in our current review in descending order of magnitude as follows: parental ID; maternal education; race; genitourinary infection in pregnancy; maternal asthma; maternal hypertension; maternal bipolar disease; maternal depression and low birthweight. The following year, Huang et al. undertook the first systematic review of determinants of ID, but in contrast to ours, theirs was limited to prenatal, perinatal, and neonatal factors and hence only included 17 studies (19). Their findings were consistent with ours for advanced maternal age, being from a priority population [Black race (United States)], low maternal education, parity, maternal smoking, diabetes, hypertension, epilepsy and asthma, male sex, preterm birth, and low birthweight. However, we also found some effects with young maternal age, advanced paternal age, socioeconomic status, fetal growth restriction, maternal urinary tract infection, and various psychiatric conditions. In 2018, Muller and colleagues used a large French longitudinal population-based preterm (<35 weeks) cohort to study the relative contributions of prenatal complications, perinatal characteristics, neonatal morbidities, and socioeconomic conditions to the occurrence of a NDD. In this already preterm population, it was found that, as well as perinatal characteristics, socioeconomic conditions still played a significant role (103). Furthermore, for a similar purpose, the Mannheim cohort of 362 children born between February 1986 and February 1998 was recruited through a two-factorial design which would allocate them into one of nine groups according to their degree of biological (e.g., preterm, low birthweight, or Apgar) or psychosocial (e.g., parental low education, psychiatric disorder, and early parenthood) risk of later developmental disorders (104). When assessed at the age of 4 years, those children at highest risk for both categories were performing most poorly, demonstrating that the risks were additive. Although we could not include this study in our analysis because the presence or absence of ID was not a specific endpoint, the findings concur very much with the interpretations we have made of our own results.
Despite the clearly established relationship between the impact of lead on the developing brain (105–107), environmental factors have generally been somewhat neglected in recent literature reviews on the determinants of ID, possibly because of the new regulations banning the use of leaded petrol (107). One exception is a recent commentary from the United States which considers the combined impact of environmental exposures intertwined with social stressors on risk of NDDs. The authors used a complex system approach based on the structure originally devised by Bronfenbrenner and Evans (108) and thus provided a framework for reshaping public policy (109). Their article elegantly demonstrates the multilevel contributing factors to neurodevelopmental vulnerability and how they may interact. It begins by considering residential locations and the inequities in education, race, income, and immigration status which exist across localities. Then, it examines the factors which either provide beneficial resources or conversely increase stress at the community level, specifically the many environmental hazards and pollutants, evidence for some of which we have seen in this review. Pregnant women living in communities under stress are at greater risk of unhealthy behaviors and likely mental health problems which compound the risk to their unborn child. Moreover, although we only identified one study on this topic which met the criteria for our review, this UK study found that children with ID were more likely than their peers to be living in residential areas with high rates of air pollution (73). However, there has been further recent commentary on the adverse effects of air pollution on neurological development in children and the biological pathways through which these may be mediated (110).
Meaning of the review
There is a need for improved strategies both to prevent ID and to intervene as early as possible in those infants and young children at most risk. We would concur with the work of Laucht et al. (104) and more recently, Payne-Sturges et al. (109) that there are vulnerable populations where risk factors, we have identified, such as low SES, poor access to education, minority ethnicity, teenage motherhood, mental illness, alcohol abuse, and obesity, are likely to cluster. It will be important to develop strategies to target the modifiable risk factors in these populations preconception. Furthermore, intervention services, leveraging the neuroplasticity of the rapidly developing brain in early childhood, offer the best hope of optimal outcomes and enduring optimization of quality of life for children at risk of ID (111). Yet, their identification is currently contingent on the presentation of behavioral symptoms of developmental delay that typically emerge after 2–3 years of age, and many children are not identified until they reach school age. As a result, timely institution of intervention services is currently suboptimal, and there is a major need for identification of children at risk of ID on the basis of risk factors, prior to the onset of symptoms, or as early as possible in the neurodevelopmental trajectory. A further next step could be to use this information to develop algorithms that can be applied to population-level datasets and in clinical practice to capture as early as possible families and children at greatest risk of ID. At-risk populations could be monitored and interventions implemented where appropriate—preconception, during pregnancy, or after birth—both to reduce the likelihood of ID and to provide optimal opportunities for these vulnerable infants. The ultimate aim was to ensure that these groups of children are identified early to have equitable access to early intervention that is culturally appropriate in line with the concept of proportionate universalism.
Data availability statement
The original contributions presented in the study are included in the article/Supplementary material, further inquiries can be directed to the corresponding author/s.
Author contributions
HL, EG, AMa, KW, AF-J, ES, JD, and AMo generated the outline of this review. HL, EG, and AMo wrote the first draft. ES developed the search strategy in conjunction with other authors and undertook the database searches. ES and HL screened the records. EG and HL further assessed the articles for eligibility. EG, HL, VE, AMo, AMa, SW, and BW undertook the JBI quality appraisals. HL and EG performed the GRADE assessments with assistance from JD. BW, HL, and EG undertook the data synthesis. BW prepared the tables. All authors including AW, MS, ML, KV, GA, and KE revised the manuscript and approved the final version.
Funding
We would like to acknowledge funding from the Telethon Kids Institute. HL was also funded by an Australian National Health and Medical Research Council (NHMRC) Senior Research Fellowship (#1117105).
Conflict of interest
The authors declare that the research was conducted in the absence of any commercial or financial relationships that could be construed as a potential conflict of interest.
Publisher’s note
All claims expressed in this article are solely those of the authors and do not necessarily represent those of their affiliated organizations, or those of the publisher, the editors and the reviewers. Any product that may be evaluated in this article, or claim that may be made by its manufacturer, is not guaranteed or endorsed by the publisher.
Supplementary material
The Supplementary Material for this article can be found online at: https://www.frontiersin.org/articles/10.3389/fpsyt.2022.926681/full#supplementary-material
References
1. Schalock R, Borthwick-Duffy S, Bradley V, Buntinx W, Coulter D, Craig E, et al. Intellectual Disability: Definition, Classification, and Systems of Supports. 11th ed. Washington, DC: American Association on Intellectual and Developmental Disabilities (2010).
2. Bourke J, de Klerk N, Smith T, Leonard H. Population-based prevalence of intellectual disability and autism spectrum disorders in western Australia: a comparison with previous estimates. Medicine. (2016) 95:e3737. doi: 10.1097/MD.0000000000003737
3. Luckasson R, Coulter D, Polloway E, Reiss S, Schalock R, Snell M, et al. Mental Retardation: Definition, Classification, and Systems of Supports. Washington DC: American Association on Mental Retardation (1992).
4. Leonard H, Wen X. The epidemiology of mental retardation: challenges and opportunities in the new millennium. Ment Retard Dev Disabil Res Rev. (2002) 8:117–34. doi: 10.1002/mrdd.10031
5. American Psychiatric Association. Diagnostic and Statistical Manual of Mental Disorders. 5th ed. Washington, DC: Author (2012). doi: 10.1176/appi.books.9780890425596
6. Maulik PK, Mascarenhas MN, Mathers CD, Dua T, Saxena S. Prevalence of intellectual disability: a meta-analysis of population-based studies. Res Dev Disabil. (2011) 32:419–36. doi: 10.1016/j.ridd.2010.12.018
7. Leonard H, Petterson B, Bower C, Sanders R. Prevalence of intellectual disability in Western Australia. Paediatr Perinat Epidemiol. (2003) 17:58–67. doi: 10.1046/j.1365-3016.2003.00469.x
8. Hiscock H, Roberts G, Efron D, Sewell JR, Bryson HE, Price AM, et al. Children attending paediatricians study: a national prospective audit of outpatient practice from the Australian paediatric research network. Med J Aust. (2011) 194:392–7. doi: 10.5694/j.1326-5377.2011.tb03028.x
9. Bethell CD, Newacheck PW, Fine A, Strickland BB, Antonelli RC, Wilhelm CL, et al. Optimizing health and health care systems for children with special health care needs using the life course perspective. Matern Child Health J. (2014) 18:467–77. doi: 10.1007/s10995-013-1371-1
10. Bebbington A, Glasson E, Bourke J, De Klerk N, Leonard H. Hospitalisation rates for children with intellectual disability or autism born in Western Australia 1983-1999: a population-based cohort study. BMJ Open. (2013) 3:e002356. doi: 10.1136/bmjopen-2012-002356
11. Bourke J, Nembhard WN, Wong K, Leonard H. Twenty-five year survival of children with intellectual disability in Western Australia. J Pediatr. (2017) 188:232.e–9.e. doi: 10.1016/j.jpeds.2017.06.008
12. Stromme P. Aetiology in severe and mild mental retardation: a population-based study of Norwegian children. Dev Med Child Neurol. (2000) 42:76–86. doi: 10.1017/S0012162200000165
13. Croen LA, Grether JK, Selvin S. The epidemiology of mental retardation of unknown cause. Pediatrics. (2001) 107:E86. doi: 10.1542/peds.107.6.e86
14. Rauch A, Hoyer J, Guth S, Zweier C, Kraus C, Becker C, et al. Diagnostic yield of various genetic approaches in patients with unexplained developmental delay or mental retardation. Am J Med Genet. (2006) 140:2063–74. doi: 10.1002/ajmg.a.31416
15. Goldfeld S, O’Connor M, Sayers M, Moore T, Oberklaid F. Prevalence and correlates of special health care needs in a population cohort of Australian children at school entry. J Dev Behav Pediatr. (2012) 33:319–27. doi: 10.1097/DBP.0b013e31824a7b8e
16. McLaren J, Bryson SE. Review of recent epidemiological studies of mental retardation: prevalence, associated disorders, and etiology. Am J Ment Retard. (1987) 92:243–54.
17. Gilman SE, Hornig M, Ghassabian A, Hahn J, Cherkerzian S, Albert PS, et al. Socioeconomic disadvantage, gestational immune activity, and neurodevelopment in early childhood. Proc Natl Acad Sci U.S.A. (2017) 114:6728–33. doi: 10.1073/pnas.1617698114
18. Durkin MS, Yeargin-Allsopp M. Socioeconomic status and pediatric neurologic disorders: current evidence. Semin Pediatr Neurol. (2018) 27:16–25. doi: 10.1016/j.spen.2018.03.003
19. Huang J, Zhu T, Qu Y, Mu D. Prenatal, perinatal and neonatal risk factors for intellectual disability: a systemic review and meta-analysis. PLoS One. (2016) 11:e0153655. doi: 10.1371/journal.pone.0153655
20. Kaufman L, Ayub M, Vincent JB. The genetic basis of non-syndromic intellectual disability: a review. j Neurodev Disord. (2010) 2:182–209. doi: 10.1007/s11689-010-9055-2
21. Caspi A, Houts RM, Belsky DW, Harrington H, Hogan S, Ramrakha S, et al. Childhood forecasting of a small segment of the population with large economic burden. Nat Hum Behav. (2016) 1:0005. doi: 10.1038/s41562-016-0005
22. Ayer C, Eapen V, Overs B, Descallar J, Jalaludin B, Eastwood JG, et al. Risk factors for non-participation in a universal developmental surveillance program in a population in Australia. Aust Health Rev. (2020) 44:512–20. doi: 10.1071/AH18236
23. Taylor CL, Christensen D, Jose K, Zubrick SR. Universal child health and early education service use from birth through Kindergarten and developmental vulnerability in the preparatory year (age 5 years) in Tasmania, Australia. Aust J Soc Issues. (2021) 57:289–313. doi: 10.1002/ajs4.186
24. Marmot M, Bell R. Fair society, healthy lives. Public Health. (2012) 126(Suppl. 1):S4–10. doi: 10.1016/j.puhe.2012.05.014
25. Moher D, Liberati A, Tetzlaff J, Altman DG, Group P. Preferred reporting items for systematic reviews and meta-analyses: the PRISMA statement. Open Med. (2009) 3:e123–30. doi: 10.1371/journal.pmed.1000097
26. Page MJ, McKenzie JE, Bossuyt PM, Boutron I, Hoffmann TC, Mulrow CD, et al. The PRISMA 2020 statement: an updated guideline for reporting systematic reviews. BMJ. (2021) 372:n71. doi: 10.1136/bmj.n71
27. Aromataris E, Fernandez R, Godfrey C, Holly C, Kahlil H, Tungpunkom P. Summarizing systematic reviews: methodological development, conduct and reporting of an Umbrella review approach. Int J Evid Based Healthc. (2015) 13:132–40. doi: 10.1097/XEB.0000000000000055
28. Fiolet T, Mahamat-Saleh Y, Frenoy P, Kvaskoff M, Romana Mancini F. Background exposure to polychlorinated biphenyls and all-cause, cancer-specific, and cardiovascular-specific mortality: a systematic review and meta-analysis. Environ Int. (2021) 154:106663. doi: 10.1016/j.envint.2021.106663
29. Abdullahi I, Wong K, Mutch R, Glasson EJ, de Klerk N, Cherian S, et al. Risk of developmental disorders in children of immigrant mothers: a population-based data linkage evaluation. J Pediatr. (2019) 204:275–84e3. doi: 10.1016/j.jpeds.2018.08.047
30. Zheng X, Chen R, Li N, Du W, Pei L, Zhang J, et al. Socioeconomic status and children with intellectual disability in China. J Intellect Disabil Res. (2012) 56:212–20. doi: 10.1111/j.1365-2788.2011.01470.x
31. Zhen H, Lawson AB, McDermott S, Lamichhane AP, Aelion M. A spatial analysis of mental retardation of unknown cause and maternal residence during pregnancy. Geospat Health. (2008) 2:173–82. doi: 10.4081/gh.2008.241
32. Yeargin-Allsopp M, Drews CD, Decoufle P, Murphy CC. Mild mental retardation in black and white children in metropolitan Atlanta: a case-control study. Am J Public Health (1995) 85:324–8. doi: 10.2105/AJPH.85.3.324
33. Williams LO, Decoufle P. Is maternal age a risk factor for mental retardation among children? Am J Epidemiol. (1999) 149:814–23. doi: 10.1093/oxfordjournals.aje.a009897
34. Wang Y, McDermott S, Mann JR, Hardin JW. Preventing intellectual disability during pregnancy: what are the potentially high yield targets? J Perinat Med. (2016) 44:421–32. doi: 10.1515/jpm-2015-0059
35. Van Naarden Braun K, Schieve L, Daniels J, Durkin M, Giarelli E, Kirby RS, et al. Relationships between multiple births and autism spectrum disorders, cerebral palsy, and intellectual disabilities: autism and developmental disabilities monitoring (ADDM) network-2002 surveillance year. Autism Res. (2008) 1:266–74. doi: 10.1002/aur.41
36. Tomson T, Muraca G, Razaz N. Paternal exposure to antiepileptic drugs and offspring outcomes: a nationwide population-based cohort study in Sweden. J Neurol Neurosurg Psychiatry. (2020) 91:907–13. doi: 10.1136/jnnp-2020-323028
37. Takei N, Murray G, O’Callaghan E, Sham PC, Glover G, Murray RM. Prenatal exposure to influenza epidemics and risk of mental retardation. Eur Arch Psychiatry Clin Neurosci. (1995) 245:255–9. doi: 10.1007/BF02191805
38. Stromme P, Magnus P. Correlations between socioeconomic status, IQ and aetiology in mental retardation: a population-based study of Norwegian children. Soc Psychiatry Psychiatr Epidemiol. (2000) 35:12–8. doi: 10.1007/s001270050003
39. Shaw GM, Jelliffe-Pawlowski L, Nelson V, Zhu H, Harris JA, Finnell RH. Infant C677T MTHFR polymorphism and severe mental retardation. Birth Defects Res Part A Clin Mol Teratol. (2007) 79:24–6. doi: 10.1002/bdra.20321
40. Schieve L, Clayton H, Durkin M, Wingate M, Drews-Botsch C. Comparison of perinatal risk factors associated with autism spectrum disorder (ASD), intellectual disability (ID), and Co-occurring ASD and ID. J Autism Dev Disord. (2015) 45:2361–72. doi: 10.1007/s10803-015-2402-0
41. Salonen JT, Heinonen OP. Mental retardation and mother’s hypertension during pregnancy. J Ment Defic Res. (1984) 28:53–6. doi: 10.1111/j.1365-2788.1984.tb01601.x
42. Rantakallio P. Social class differences in mental retardation and subnormality. Scand J Soc Med. (1987) 15:63–6. doi: 10.1177/140349488701500202
43. Oswald DP, Coutinho MJ, Best AM, Nguyen N. Impact of sociodemographic characteristics on the identification rates of minority students as having mental retardation. Ment Retard. (2001) 39:351–67. doi: 10.1352/0047-6765(2001)039<0351:IOSCOT>2.0.CO;2
44. Onicescu G, Lawson AB, McDermott S, Aelion CM, Cai B. Bayesian importance parameter modeling of misaligned predictors: soil metal measures related to residential history and intellectual disability in children. Environ Sci Pollut Res. (2014) 21:10775–86. doi: 10.1007/s11356-014-3072-8
45. O’Leary C, Leonard H, Bourke J, D’Antoine H, Bartu A, Bower C. Intellectual disability: population-based estimates of the proportion attributable to maternal alcohol use disorder during pregnancy. Dev Med Child Neurol. (2013) 55:271–7. doi: 10.1111/dmcn.12029
46. Morgan VA, Croft ML, Valuri GM, Zubrick SR, Bower C, McNeil TF, et al. Intellectual disability and other neuropsychiatric outcomes in high-risk children of mothers with schizophrenia, bipolar disorder and unipolar major depression. Br J Psychiatry. (2012) 200:282–9. doi: 10.1192/bjp.bp.111.093070
47. Mervis CA, Decoufle P, Murphy CC, Yeargin-Allsopp M. Low birthweight and the risk for mental retardation later in childhood. Paediatr Perinat Epidemiol. (1995) 9:455–68. doi: 10.1111/j.1365-3016.1995.tb00168.x
48. McDermott S, Wu J, Cai B, Lawson A, Marjorie Aelion C. Probability of intellectual disability is associated with soil concentrations of arsenic and lead. Chemosphere. (2011) 84:31–8. doi: 10.1016/j.chemosphere.2011.02.088
49. McDermott S, Szwejbka L, Mann H, Durkin M, Callaghan W. Urinary tract infections during pregnancy in South Carolina. J S C Med Assoc. (2001) 97:195–200.
50. McDermott S, Coker AL, McKeown RE. Low birthweight and risk of mild mental retardation by ages 5 and 9 to 11. Paediatr Perinat Epidemiol. (1993) 7:195–204. doi: 10.1111/j.1365-3016.1993.tb00393.x
51. McDermott S, Callaghan W, Szwejbka L, Mann H, Daguise V. Urinary tract infections during pregnancy and mental retardation and developmental delay. Obstet Gynecol. (2000) 96:113–9. doi: 10.1097/00006250-200007000-00023
52. McDermott S, Bao W, Tong X, Cai B, Lawson A, Aelion CM. Are different soil metals near the homes of pregnant women associated with mild and severe intellectual disability in children? Dev Med Child Neurol. (2014) 55:888–97. doi: 10.1111/dmcn.12442
53. Mann JR, Pan C, Rao GA, McDermott S, Hardin JW. Children born to diabetic mothers may be more likely to have intellectual disability. Matern Child Health J. (2013) 17:928–32. doi: 10.1007/s10995-012-1072-1
54. Mann JR, McDermott SW, Hardin J, Pan C, Zhang Z. Pre-pregnancy body mass index, weight change during pregnancy, and risk of intellectual disability in children. BJOG. (2013) 120:309–19. doi: 10.1111/1471-0528.12052
55. Mann JR, McDermott S, Barnes TL, Hardin J, Bao H, Zhou L. Trichomoniasis in pregnancy and mental retardation in children. Ann Epidemiol. (2009) 19:891–9. doi: 10.1016/j.annepidem.2009.08.004
56. Mackay DF, Smith GC, Cooper SA, Wood R, King A, Clark DN, et al. Month of conception and learning disabilities: a record-linkage study of 801,592 children. Am J Epidemiol. (2016) 184:485–93. doi: 10.1093/aje/kww096
57. Luu TM, Ment LR, Schneider KC, Katz KH, Allan WC, Vohr BR. Lasting effects of preterm birth and neonatal brain hemorrhage at 12 years of age. Pediatrics. (2009) 123:1037−44. doi: 10.1542/peds.2008-1162
58. Louhiala P. Risk indicators of mental retardation: changes between 1967-1981. Dev Med Child Neurol. (1995) 37:631–6. doi: 10.1111/j.1469-8749.1995.tb12051.x
59. Li M, Fallin M, Riley A, Landa R, Walker SO, Silverstein M, et al. The association of maternal obesity and diabetes with autism and other developmental disabilities. Pediatrics. (2016) 137:1–10. doi: 10.1542/peds.2015-2206
60. Leonard H, Petterson B, De Klerk N, Zubrick SR, Glasson E, Sanders R, et al. Association of sociodemographic characteristics of children with intellectual disability in Western Australia. Soc Sci Med. (2005) 60:1499–513. doi: 10.1016/j.socscimed.2004.08.014
61. Leonard H, Nassar N, Bourke J, Blair E, Mulroy S, de Klerk N, et al. Relation between intrauterine growth and subsequent intellectual disability in a ten-year population cohort of children in Western Australia. Am J Epidemiol. (2008) 167:103–11. doi: 10.1093/aje/kwm245
62. Leonard H, Glasson E, Nassar N, Whitehouse A, Bebbington A, Bourke J, et al. Autism and intellectual disability are differentially related to sociodemographic background at birth. PLoS One. (2011) 6:e17875. doi: 10.1371/journal.pone.0017875
63. Leonard H, de Klerk N, Bourke J, Bower C. Maternal health in pregnancy and intellectual disability in the offspring: a population-based study. Ann Epidemiol. (2006) 16:448–54. doi: 10.1016/j.annepidem.2005.05.002
64. Langridge AT, Glasson EJ, Nassar N, Jacoby P, Pennell C, Hagan R, et al. Maternal conditions and perinatal characteristics associated with autism spectrum disorder and intellectual disability. PLoS One. (2013) 8:e50963. doi: 10.1371/journal.pone.0050963
65. Jones KL, Croen LA, Yoshida CK, Heuer L, Hansen R, Zerbo O, et al. Autism with intellectual disability is associated with increased levels of maternal cytokines and chemokines during gestation. Mol Psychiatry. (2017) 22:273–9. doi: 10.1038/mp.2016.77
66. Jelliffe-Pawlowski LL, Shaw GM, Nelson V, Harris JA. Risk of mental retardation among children born with birth defects. Arch Pediatr Adolesc Med. (2003) 157:545–50. doi: 10.1001/archpedi.157.6.545
67. Hurtado EK, Claussen AH, Scott KG. Early childhood anemia and mild or moderate mental retardation. Am J Clin Nutr. (1999) 69:115–9. doi: 10.1093/ajcn/69.1.115
68. Heuvelman H, Abel K, Wicks S, Gardner R, Johnstone E, Lee B, et al. Gestational age at birth and risk of intellectual disability without a common genetic cause. Eur J Epidemiol. (2018) 33:667–78. doi: 10.1007/s10654-017-0340-1
69. Heikura U, Taanila A, Hartikainen A, Olsen P, Linna S, von Wendt L, et al. Variations in prenatal sociodemographic factors associated with intellectual disability: a study of the 20-year interval between two birth cohorts in northern Finland. Am J Epidemiol. (2008) 167:169–77. doi: 10.1093/aje/kwm291
70. Guo TW, Zhang FC, Yang MS, Gao XC, Bian L, Duan SW, et al. Positive association of the DIO2 (deiodinase type 2) gene with mental retardation in the iodine-deficient areas of China. J Med Genet. (2004) 41:585–90. doi: 10.1136/jmg.2004.019190
71. Griffith MI, Mann JR, McDermott S. The risk of intellectual disability in children born to mothers with preeclampsia or eclampsia with partial mediation by low birth weight. Hypertens. (2011) 30:108–15. doi: 10.3109/10641955.2010.507837
72. Fairthorne J, Hammond G, Bourke J, de Klerk N, Leonard H. Maternal psychiatric disorder and the risk of autism spectrum disorder or intellectual disability in subsequent offspring. J Autism Dev Disord. (2015) 46:523–33. doi: 10.1007/s10803-015-2594-3
73. Emerson E, Robertson J, Hatton C, Baines S. Risk of exposure to air pollution among British children with and without intellectual disabilities. J Intellect Disabil Res. (2019) 63:161–7. doi: 10.1111/jir.12561
74. Emerson E. Deprivation, ethnicity and the prevalence of intellectual and developmental disabilities. J Epidemiol Community Health. (2012) 66:218–24. doi: 10.1136/jech.2010.111773
75. Drews CD, Yeargin-Allsopp M, Decoufle P, Murphy CC. Variation in the influence of selected sociodemographic risk factors for mental retardation. Am J Public Health. (1995) 85:329–34. doi: 10.2105/AJPH.85.3.329
76. Drews CD, Murphy CC, Yeargin-Allsopp M, Decoufle P. The relationship between idiopathic mental retardation and maternal smoking during pregnancy. Pediatrics. (1996) 97:547–53. doi: 10.1542/peds.97.4.547
77. Di Prinzio P, Morgan VA, Bjork J, Croft M, Lin A, Jablensky A, et al. Intellectual disability and psychotic disorders in children: association with maternal severe mental illness and exposure to obstetric complications in a whole-population cohort. Am J Psychiatry. (2018) 175:1232–42. doi: 10.1176/appi.ajp.2018.17101153
78. Decoufle P, Murphy CC, Drews CD, Yeargin-Allsopp M. Mental retardation in ten-year-old children in relation to their mothers’ employment during pregnancy. Am J Ind Med (1993) 24:567–86. doi: 10.1002/ajim.4700240507
79. Decoufle P, Boyle CA. The relationship between maternal education and mental retardation in 10-year-old children. Ann Epidemiol (1995) 5:347–53. doi: 10.1016/1047-2797(95)00031-2
80. Chen R, Tedroff K, Villamor E, Lu D, Cnattingius S. Risk of intellectual disability in children born appropriate-for-gestational-age at term or post-term: impact of birth weight for gestational age and gestational age. Eur J Epidemiol. (2020) 35:273–82. doi: 10.1007/s10654-019-00590-7
81. Chen C, Lu D, Xue L, Ren P, Zhang H, Zhang J. Association between placental inflammatory pathology and offspring neurodevelopment at 8 months and 4 and 7 years of age. J Pediatr. (2020) 225:132–7e2. doi: 10.1016/j.jpeds.2020.05.049
82. Chapman DA, Scott KG, Mason CA. Early risk factors for mental retardation: role of maternal age and maternal education. Am J Ment Retard. (2002) 107:46–59. doi: 10.1352/0895-8017(2002)107<0046:ERFFMR>2.0.CO;2
83. Camp BW, Broman SH, Nichols PL, Leff M. Maternal and neonatal risk factors for mental retardation: defining the ‘at-risk’ child. Early Hum Dev. (1998) 50:159–73. doi: 10.1016/S0378-3732(97)00034-9
84. Blotiere PO, Miranda S, Weill A, Mikaeloff Y, Peyre H, Ramus F, et al. Risk of early neurodevelopmental outcomes associated with prenatal exposure to the antiepileptic drugs most commonly used during pregnancy: a French nationwide population-based cohort study. BMJ Open. (2020) 10:e034829. doi: 10.1136/bmjopen-2019-034829
85. Bilder DA, Pinborough-Zimmerman J, Bakian AV, Miller JS, Dorius JT, Nangle B, et al. Prenatal and perinatal factors associated with intellectual disability. Am J Intellect Dev Disabil. (2013) 118:156–76. doi: 10.1352/1944-7558-118.2.156
86. McDermott S, Daguise V, Mann H, Szwejbka L, Callaghan W. Perinatal risk for mortality and mental retardation associated with maternal urinary-tract infections. J Fam Pract. (2001) 50:433–7.
87. Leonard H, Glasson E, Bebbington A, Hammond G, Croft D, Pikora T, et al. Application of population-based linked data to the study of intellectual disability and autism. In: RC Urbano editor. International Review of Research in Developmental Disabilities. Cambridge, MA: Academic Press (2013). p. 281–327. doi: 10.1016/B978-0-12-407760-7.00008-6
88. Pereira G, Blair E, Lawrence D. Validation of a model for optimal birth weight: a prospective study using serial ultrasounds. BMC Pediatr. (2012) 12:73. doi: 10.1186/1471-2431-12-73
89. Yin W, Doring N, Persson M, Persson M, Tedroff K, Aden U, et al. Gestational age and risk of intellectual disability: a population-based cohort study. Arch Dis Child. (2022). doi: 10.1136/archdischild-2021-323308
90. Chen S, Zhao S, Dalman C, Karlsson H, Gardner R. Association of maternal diabetes with neurodevelopmental disorders: autism spectrum disorders, attention-deficit/hyperactivity disorder and intellectual disability. Int J Epidemiol. (2021) 50:459–74. doi: 10.1093/ije/dyaa212
91. Lee PMY, Tse LA, Laszlo KD, Wei D, Yu Y, Li J. Association of maternal gestational weight gain with intellectual developmental disorder in the offspring: a nationwide follow-up study in Sweden. BJOG. (2022) 129:540–9. doi: 10.1111/1471-0528.16887
92. Christensen J, Gronborg TK, Sorensen MJ, Schendel D, Parner ET, Pedersen LH, et al. Prenatal valproate exposure and risk of autism spectrum disorders and childhood autism. JAMA. (2013) 309:1696–703. doi: 10.1001/jama.2013.2270
93. Daugaard CA, Pedersen L, Sun Y, Dreier JW, Christensen J. Association of prenatal exposure to valproate and other antiepileptic drugs with intellectual disability and delayed childhood milestones. JAMA Netw Open. (2020) 3:e2025570. doi: 10.1001/jamanetworkopen.2020.25570
94. Rai D, Lee BK, Dalman C, Newschaffer C, Lewis G, Magnusson C. Antidepressants during pregnancy and autism in offspring: population based cohort study. BMJ. (2017) 358:j2811. doi: 10.1136/bmj.j2811
95. Uguz F. Neonatal and childhood outcomes in offspring of pregnant women using antidepressant medications: a critical review of current meta-analyses. J Clin Pharmacol. (2021) 61:146–58. doi: 10.1002/jcph.1724
96. Viktorin A, Uher R, Kolevzon A, Reichenberg A, Levine SZ, Sandin S. Association of antidepressant medication use during pregnancy with intellectual disability in offspring. JAMA Psychiatry. (2017) 74:1031–8. doi: 10.1001/jamapsychiatry.2017.1727
97. Panisi C, Guerini FR, Abruzzo PM, Balzola F, Biava PM, Bolotta A, et al. Autism spectrum disorder from the womb to adulthood: suggestions for a paradigm shift. J Pers Med. (2021) 11:70. doi: 10.3390/jpm11020070
98. Saxena R, Babadi M, Namvarhaghighi H, Roullet FI. Chapter two – role of environmental factors and epigenetics in autism spectrum disorders. In: M Ilieva, WK-W Lau editors. Progress in Molecular Biology and Translational Science. Cambridge, MA: Academic Press (2020). p. 35–60. doi: 10.1016/bs.pmbts.2020.05.002
99. Balogh R, Leonard H, Bourke J, Brameld K, Downs J, Hansen M, et al. Data linkage: canadian and Australian perspectives on a valuable methodology for intellectual and developmental disability research. Intellect Dev Disabil. (2019) 57:439–62. doi: 10.1352/1934-9556-57.5.439
100. Nelson KB, Grether JK, Croen LA, Dambrosia JM, Dickens BF, Jelliffe LL, et al. Neuropeptides and neurotrophins in neonatal blood of children with autism or mental retardation. Ann Neurol. (2001) 49:597–606. doi: 10.1002/ana.1024
101. Glasson EJ, Jacques A, Wong K, Bourke J, Leonard H. Improved survival in Down syndrome over the last 60 years and the impact of perinatal factors in recent decades. J Pediatr. (2016) 169:214.e–20.e. doi: 10.1016/j.jpeds.2015.10.083
102. Patel DR, Cabral MD, Ho A, Merrick J. A clinical primer on intellectual disability. Trans Pediatr. (2020) 9:S23–35. doi: 10.21037/tp.2020.02.02
103. Muller JB, Hanf M, Flamant C, Olivier M, Rouger V, Gascoin G, et al. Relative contributions of prenatal complications, perinatal characteristics, neonatal morbidities and socio-economic conditions of preterm infants on the occurrence of developmental disorders up to 7 years of age. Int J Epidemiol. (2019) 48:71–82. doi: 10.1093/ije/dyy240
104. Laucht M, Esser G, Schmidt MH. Developmental outcome of infants born with biological and psychosocial risks. J Child Psychol Psychiatry. (1997) 38:843–53. doi: 10.1111/j.1469-7610.1997.tb01602.x
105. David O, Hoffman S, McGann B, Sverd J, Clark J. Low lead levels and mental retardation. Lancet. (1976) 2:1376–9. doi: 10.1016/S0140-6736(76)91918-8
106. Moore MR, Meredith PA, Goldberg A. A retrospective analysis of blood-lead in mentally retarded children. Lancet (1977) 1:717–9. doi: 10.1016/S0140-6736(77)92165-1
107. Yan Y, Yang S, Zhou Y, Song Y, Huang J, Liu Z, et al. Estimating the national burden of mild intellectual disability in children exposed to dietary lead in China. Environ Int. (2020) 137:105553. doi: 10.1016/j.envint.2020.105553
108. Bronfenbrenner U, Evans GW. Developmental science in the 21st century: emerging questions, theoretical models, research designs and empirical findings. Soc Dev. (2000) 9:115–25. doi: 10.1111/1467-9507.00114
109. Payne-Sturges DC, Cory-Slechta DA, Puett RC, Thomas SB, Hammond R, Hovmand PS. Defining and intervening on cumulative environmental neurodevelopmental risks: introducing a complex systems approach. Environ Health Perspect. (2021) 129:35001. doi: 10.1289/EHP7333
110. Ha S. Air pollution and neurological development in children. Dev Med Child Neurol. (2021) 63:374–81. doi: 10.1111/dmcn.14758
Keywords: intellectual disability, systematic review, sociodemographic, antenatal, risk factor, maternal health, data linkage, population data
Citation: Leonard H, Montgomery A, Wolff B, Strumpher E, Masi A, Woolfenden S, Williams K, Eapen V, Finlay-Jones A, Whitehouse A, Symons M, Licari M, Varcin K, Alvares G, Evans K, Downs J and Glasson E (2022) A systematic review of the biological, social, and environmental determinants of intellectual disability in children and adolescents. Front. Psychiatry 13:926681. doi: 10.3389/fpsyt.2022.926681
Received: 22 April 2022; Accepted: 27 June 2022;
Published: 25 August 2022.
Edited by:
Marinos Kyriakopoulos, King’s College London, United KingdomReviewed by:
Roberto Keller, ASL Città di Torino, ItalyIlias Partsenidis, South London and Maudsley NHS Foundation Trust, United Kingdom
Copyright © 2022 Leonard, Montgomery, Wolff, Strumpher, Masi, Woolfenden, Williams, Eapen, Finlay-Jones, Whitehouse, Symons, Licari, Varcin, Alvares, Evans, Downs and Glasson. This is an open-access article distributed under the terms of the Creative Commons Attribution License (CC BY). The use, distribution or reproduction in other forums is permitted, provided the original author(s) and the copyright owner(s) are credited and that the original publication in this journal is cited, in accordance with accepted academic practice. No use, distribution or reproduction is permitted which does not comply with these terms.
*Correspondence: Helen Leonard, aGVsZW4ubGVvbmFyZEB0ZWxldGhvbmtpZHMub3JnLmF1