- 1Department of Radiology, Guang'anmen Hospital, China Academy of Chinese Medical Sciences, Beijing, China
- 2Department of Psychiatric, Beijing First Hospital of Integrated Chinese and Western Medicine, Beijing, China
- 3Department of Neurology, Xuanwu Hospital, Capital Medical University, Beijing, China
Objective: In this study, we used amplitude of low-frequency fluctuation (ALFF) and regional homogeneity (ReHo) to observe differences in local brain functional activity and its characteristics in patients with treatment-resistant depression (TRD) and non-treatment-resistant depression (nTRD), and to explore the correlation between areas of abnormal brain functional activity and clinical symptoms.
Method: Thirty-seven patients with TRD, 36 patients with nTRD, and 35 healthy controls (HCs) were included in resting-state fMRI scans. ALFF and ReHo were used for image analysis and further correlation between abnormal brain regions and clinical symptoms were analyzed.
Results: ANOVA revealed that the significantly different brain regions of ALFF and ReHo among the three groups were mainly concentrated in the frontal and temporal lobes. Compared with the nTRD group, the TRD group had decreased ALFF in the left/right inferior frontal triangular gyrus, left middle temporal gyrus, left cuneus and bilateral posterior lobes of the cerebellum, and increased ALFF in the left middle frontal gyrus and right superior temporal gyrus, and the TRD group had decreased ReHo in the left/right inferior frontal triangular gyrus, left middle temporal gyrus, and increased ReHo in the right superior frontal gyrus. Compared with the HC group, the TRD group had decreased ALFF/ReHo in both the right inferior frontal triangular gyrus and the left middle temporal gyrus. Pearson correlation analysis showed that both ALFF and ReHo values in these abnormal brain regions were positively correlated with HAMD-17 scores (P < 0.05).
Conclusion: Although the clinical symptoms were similar in the TRD and nTRD groups, abnormal neurological functional activity were present in some of the same brain regions. Compared with the nTRD group, ALFF and ReHo showed a wider range of brain area alterations and more complex neuropathological mechanisms in the TRD group, especially in the inferior frontal triangular gyrus of the frontal lobe and the middle temporal gyrus of the temporal lobe.
Introduction
Major depressive disorder (MDD) is a common clinical disorder of the psychiatric system, characterized by significant and persistent depressed mood, reduced interest, reduced cognitive function and somatic disturbances (1). MDD has a high global prevalence, affecting over 300 million people worldwide, and is expected to be the leading global disease burden by the end of 2030 (2). Despite the availability of psychological, physical and pharmacological therapies, 30–40% of people with MDD still fail to respond to these treatments (3). According to the most common previous definition of treatment-resistant depression(TRD), the patient does not improve after at least two or more treatments of adequate dose and duration (4). Therefore, the study of TRD has become a challenging research domain, where substantial effort has been invested worldwide (5).
TRD is a complex disorder whose pathogenesis is not fully understood, and it reflects the coexistence of multiple depressive subtypes, psychiatric comorbidity, and coexisting medical illnesse (6). Compared with the non-treatment-resistant depression (nTRD), patients with TRD are more difficult to treat and consume more treatment costs (4, 7). Overall medical costs for TRD patients are almost twice or more than twice as high as those for nTRD patients (8, 9). In terms of clinical symptoms, compared with nTRD patients, TRD patients had more severe depressive symptoms, cognitive impairment, anxiety and distress, longer onset period, and lower work productivity (7, 10–12). Therefore, it is necessary to explore the biomarkers of TRD, which in turn will facilitate the understanding of the differences in the pathogenesis of TRD and nTRD.
Currently, although brain tissue or cerebrospinal fluid is an ideal resource for studying MDD biomarkers, it is more difficult to obtain in the clinic (13). Although peripheral blood proteins are easy to obtain but there are many types of studies and no uniform standards have been established (14). In recent years, resting-state functional magnetic resonance imaging (rs-fMRI) has been gradually applied in the field of psychiatric disorders, such as MDD (15, 16), schizophrenia (17) and autism (18), because of its simplicity and ease of acquisition. It has also been further applied to the study of subtypes of depression (19–21). The application of rs-fMRI technology facilitates a deeper understanding of the neuropathology of depression and the development of effective antidepressant drugs (22).
Regional homogeneity (ReHo) and amplitude of low-frequency fluctuations (ALFF) are commonly used in rs-fMRI to study functional brain activity. ReHo is used to assess the level of coordination of neural activity in local brain regions by calculating ReHo values to indirectly reflect the spontaneous activity of local neurons in time synchronization (23). ALFF reflects the intensity of spontaneous activity in a region of the brain over a short period of time by calculating the average amplitude of low-frequency oscillations in that region (24). However, the diseases currently studied using a combined ALFF and ReHo approach mainly include Parkinson's disease (26), generalized anxiety disorder (25), bipolar disorder (27), and migraine without aura (28), while differential studies of TRD and nTRD are lacking. So far, there have been some studies on the difference between TRD and nTRD, but most of them use ALFF or ReHo as a single indicator. Previous studies have shown differences in the default mode network (DMN), visual recognition circuits, right auditory, right sensory/somatomotor hand and cerebellar ALFF in the TRD group compared to the nTRD group (29, 30). In addition, other studies have shown differences in ReHo in the left inferior frontal gyrus, right middle temporal gyrus, left precuneus, middle cingulate gyrus and left fusiform gyrus in the TRD group compared to the nTRD group (31, 32). The above rs-fMRI study found that ALFF and ReHo may be highly sensitive to changes in TRD. Previous studies have consistently shown that changes in different indices may represent different sensitivities and that changes in multiple indices in the same brain region during the same period can help improve the sensitivity of the diagnosis (33). In addition, from the results of previous studies, it also can be seen that the main difference between TRD and nTRD is in the frontal and temporal lobes and other brain regions. It has also been shown that patients with TRD have gray matter volume changes in frontal and temporal lobe regions (34). The frontal and temporal lobes are not only important brain regions in the pathogenesis of MDD, but also closely related to the DMN and cognitive control network (CCN) (35–38).
Therefore, we used ALFF and ReHo to differentiate the differences in functional brain activity among the TRD group, nTRD group and healthy control (HC) group based on previous studies, and further analyzed the correlation between the differential brain areas and clinical characteristics. We hypothesized that there are differences in neural circuits in the brain of TRD and nTRD patients, especially closely related to the frontal lobe, temporal lobe. This study will provide a neuroimaging basis for the differences in neuropathological mechanisms between TRD and nTRD, which will also contribute more to the understanding of the pathogenesis of TRD.
Materials and Methods
Participants
Seventy-three patients with MDD were from Guang'anmen Hospital, China Academy of Chinese Medical Sciences, Beijing First Hospital of Integrated Chinese and Western Medicine, and Xuanwu Hospital, Capital Medical University. All patients met the MDD criteria of the Diagnostic and Statistical Manual of Mental Disorders, Fifth Edition (DSM-5). We used the 17-item Hamilton Rating Scale for Depression scale (HAMD-17) to assess the severity of depression in all MDD patients and divided these patients into those with TRD (TRD, n = 37) and those with nTRD (nTRD, n = 36). The inclusion criteria for all patients were: (1) age 18–55 years; (2) HAMD-17 score >17; (3) right-handedness; (4) The TRD group was eligible to receive two or more adequate doses and courses of antidepressant therapy that were not effective and had been stable for more than 6 weeks on antidepressant medication prior to enrollment, with non-responsiveness defined as a decrease in HAMD-17 score of <50%. The nTRD group was in symptomatic remission on one antidepressant, with responsiveness defined as a decrease of more than 50% in HAMD-17 scores, relapse prior to enrollment. Thirty-five gender- and age-matched HCs (21 females and 14 males): (1) age 18–55 years; (2) HAMD-17 score <7; (3) right-handedness; (4) no history of any mental illness in first-degree relatives.
The exclusion criteria for patients and HCs were as follows: (1) history of drug abuse; (2) any contraindications to MRI, such as presence of a heart pacemaker, metal fixed false teeth, or severe claustrophobia; (3) pregnant or lactating status; (4) bipolar disorder, suicidal thoughts, and any other mental illness; (5) tumors, history of head trauma with loss of consciousness, and any cardiovascular, kidney or other major medical condition; (6) previous participation in electrical stimulation therapy.
All patients were required to sign an informed consent form before enrollment. This study was approved by the ethics committee of Guang'anmen Hospital, China Academy of Chinese Medical Sciences.
Scan Acquisition
All patients in this study underwent MRI using a Magnetom Skyra 3.0-T scanner (Siemens, Erlangen, Germany). Before the scanning procedure, the patients were instructed to remain awake and avoid active thinking. During the scanning process, the patients were required to wear earplugs and noise-canceling headphones, to use a hood to immobilize the head, and to lie flat on the examination bed. The scanning procedure involved a localizer scan, high-resolution three-dimensional T1-weighted imaging, and BOLD-fMRI.
The scanning parameters were as follows: for three-dimensional T1-weighted imaging, time repetition/time echo = 2500/2.98 ms, flip angle = 7°, matrix = 64 × 64, field of view = 256 mm × 256 mm, slice thickness = 1 mm, slice number = 48, slices = 192, scanning time = 6 min 3 s; for BOLD-fMRI, time repetition/time echo = 2000/30 ms, flip angle = 90°, matrix = 64 × 64, field of view = 240 mm × 240 mm, slice number = 43, slice thickness/spacing = 3.0/1.0 mm, number of obtained volumes = 200, and scanning time = 6 min 40 s.
Image Processing
fMRI Data Preprocessing
The acquired rs-fMRI data were pre-processed using MATLAB-based DPARSF 5.1 software (DPARSF 5.1, http://www.rfmri.org/DPARSF). (1) conversion of DICOM raw data to NIFTI format; (2) removal of the first 10 time points in order to put the data in a stable state; (3) slice-timing; (4) realignment of head-motion (removal of patients with head movements > 2 mm in any direction and motor rotation >2°); (5) regression of covariates, including brain white matter signal, cerebrospinal fluid signal and head movement parameters; (6) spatial normalization: functional images of all subjects were converted to Montreal Neurological Institute (MNI) standard space using DARTEL; (7) linear detrending and filtering (0.01 to 0.08 Hz).
ALFF Analysis
The data were spatially normalized and smoothed, and then a fast fourier transform (FFT) was performed to switch the time series to the frequency domain to obtain the power spectrum. The square root of the power spectrum at each frequency is calculated to obtain the average square root of the ALFF measurement for each voxel in the range of 0.01 to 0.08 Hz. Finally, time bandpass filtering (0.01 to 0.08 Hz) is then performed. To reduce inter-individual variability, ALFF was transformed to zALFF by Fisher's z transformation prior to statistical analysis.
ReHo Analysis
The similarity of the time series of each voxel to its neighboring voxels (26 neighboring voxels) was assessed using the Kendall's coefficient concordance(KCC), i.e., ReHo values. The whole-brain ReHo images of the subjects were obtained by calculating the KCC values of the whole-brain voxels. To improve the signal-to-noise ratio, the ReHo images were spatially smoothed using a 6 mm × 6 mm × 6 mm full-width half-height Gaussian kernel.
Statistical Analyses
Clinical Data Analysis
Clinical data were analyzed with SPSS 23.0 statistical software (IBM Corporation, Somers, New York). One-way analysis of variance (ANOVA) was used to compare ages and education levels among the three groups, and the chi-square test was used to compare gender. A two-sample t-test was used to compare the duration of disease and HAMD-17 scores between the two groups, with P < 0.05 (two-tailed) as the threshold for statistical significance.
fMRI Data Analysis
Image data were analyzed using the DPARSF toolbox, and a voxel-based one-way ANOVA was performed to compare whole-brain ALFF/ReHo map of the three groups. Gender, age, education level and framewise displacement (FD) metric (derived from Jenkinson's formula) of the three groups of subjects were used as covariates, and brain areas with ALFF/ReHo differences between the three groups were corrected for Gaussian random fields (GRF), and corrected cluster levels were set as P < 0.05 (two-tailed) and threshold voxel levels P < 0.005 were defined as statistically different. We performed post-hoc t-test analysis using DPARSF 5.1 software for two-by-two comparisons between groups, and Bonferroni correction was applied to the results, setting a threshold of P < 0.016 (0.05/3) for statistical significance.
In order to verify the relationship between ALFF/ReHo values and clinical symptoms, we extracted the mean ALFF/ReHo values of the three different brain regions and did Pearson correlation analysis with the clinical scale scores of each group, and the statistical threshold of P < 0.05 (two-tailed) was statistically significant.
Results
Characteristics of Research Samples
Two patients with TRD and one patient with nTRD were excluded because of excessive head movement. Therebefore, a total of 35 patients with TRD, 35 patients with nTRD, and 35 HCs were eligible for the study criteria. There were no statistical differences among the three groups in terms of gender, age and years of education. There was no statistical difference between the TRD and nTRD groups in terms of HAMD-17 scores and a statistical difference in terms of illness duration (Table 1).
Differences in ALFF/ ReHo Among the TRD Group, nTRD Group, and HC Group
A one-way ANOVA revealed significant differences in ALFF and ReHo among the three groups in the left/right inferior frontal triangular gyrus, left middle temporal gyrus and right superior temporal gyrus. Meanwhile, there were significant differences among the three groups in ALFF in the left precentral gyrus, left cuneus, left superior occipital gyrus, and bilateral posterior lobes of the cerebellum, and significant differences in ReHo in the right superior frontal gyrus (Tables 2, 3 and Figures 1, 2).
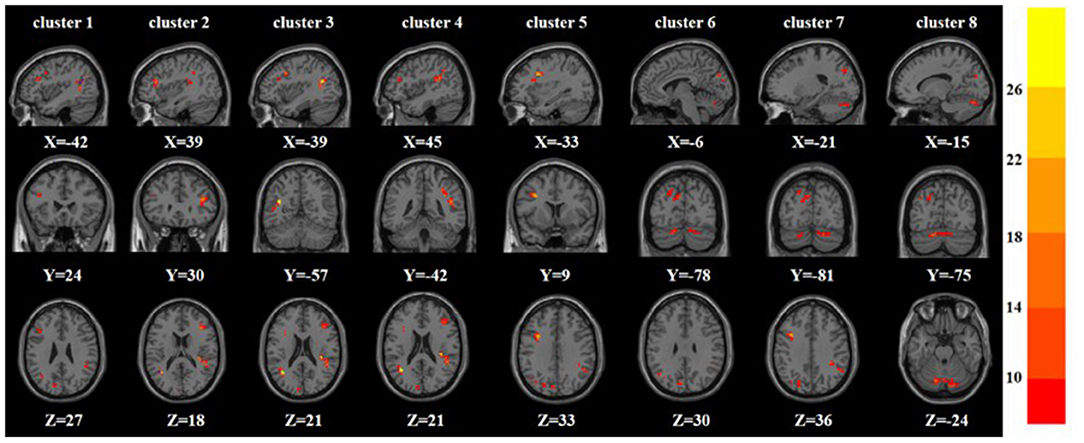
Figure 1. Based on one-way ANOVA for three groups of ALFF abnormal brain regions. The color bars indicate the F-value.
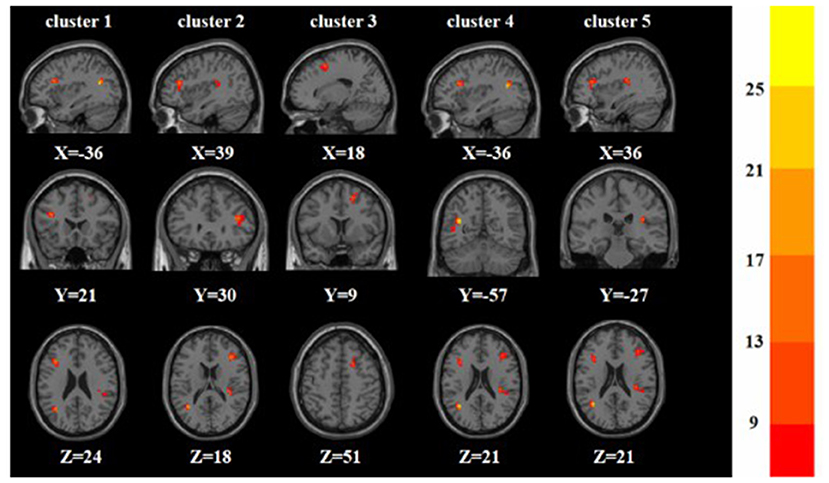
Figure 2. Based on one-way ANOVA for three groups of ReHo abnormal brain regions. The color bars indicate the F-value.
Compared with the nTRD group, the TRD group had increased ALFF in the left middle frontal gyrus, right superior temporal gyrus, and decreased ALFF in the left/right inferior frontal triangular gyrus, left middle temporal gyrus, left cuneus, and bilateral posterior lobes of the cerebellum. On the other hand, the TRD group had increased ReHo in the right superior frontal gyrus and decreased ReHo in the left/right inferior frontal triangular gyrus, and left middle temporal gyrus (Tables 2, 3 and Figure 3).
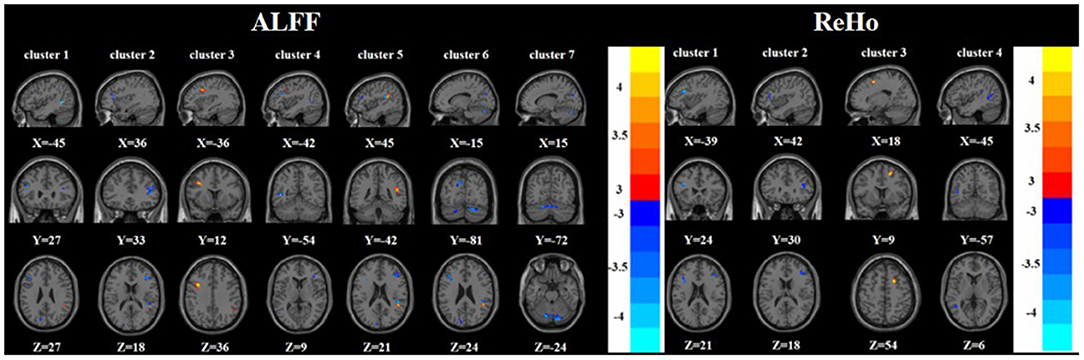
Figure 3. Brain regions with abnormal ALFF (left) and ReHo (right) between TRD group and nTRD group based on post hoc T-tests. The color bars indicate the T-value.
Compared with the HC group, the TRD group had increased ALFF in the left middle frontal gyrus and decreased ALFF in the right inferior frontal triangular gyrus and left middle temporal gyrus. On the other hand, the TRD group had decreased ReHo in the right inferior frontal triangular gyrus and left middle temporal gyrus (Tables 2, 3 and Figure 4).
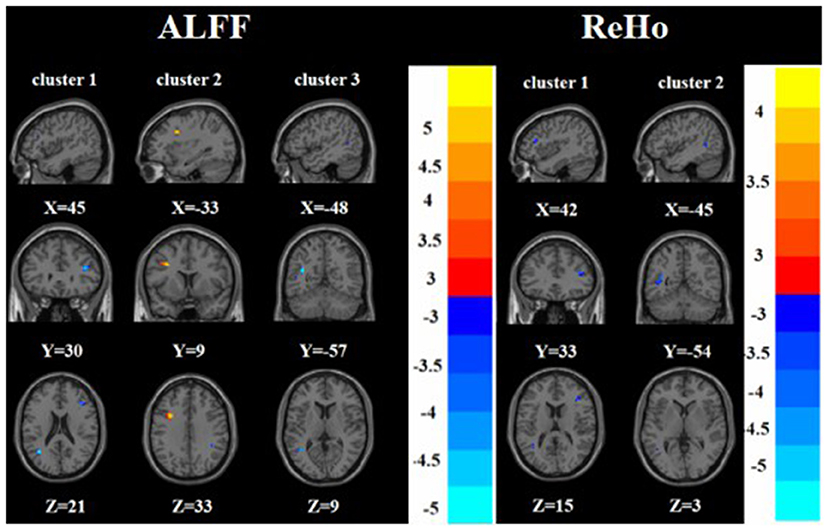
Figure 4. Brain regions with abnormal ALFF (left) and ReHo (right) between TRD group and HC group based on post hoc T-tests. The color bars indicate the T-value.
Compared with the HC group, the nTRD group had increased ALFF in the right superior temporal gyrus and decreased ALFF in the left angular gyrus. On the other hand, the nTRD group had increased ReHo in the right insula and decreased ReHo in the left angular gyrus (Tables 2, 3 and Figure 5).
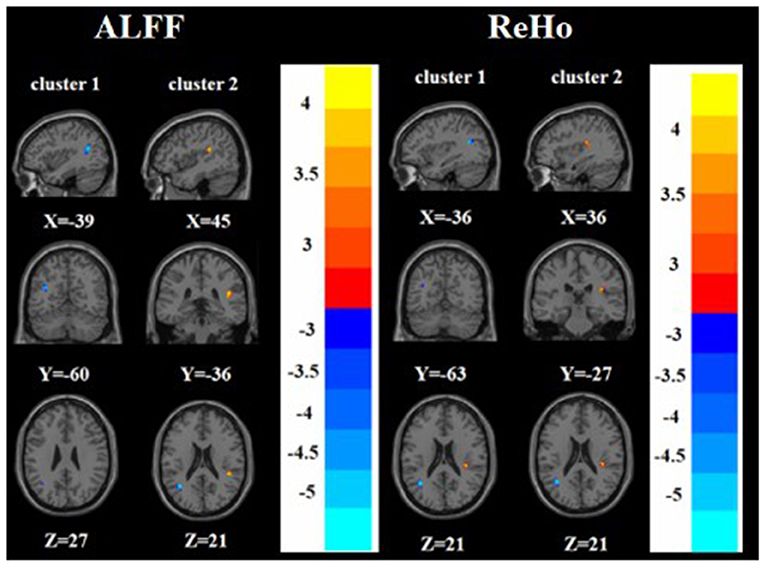
Figure 5. Brain regions with abnormal ALFF (left) and ReHo (right) between nTRD group and HC group based on post hoc T-tests. The color bars indicate the T-value.
Significant Correlation Between Functional Image and Clinical Feature
To test the correlation between areas of abnormal brain activity and the severity of clinical depressive symptoms, we further performed Pearson correlation analysis. We found that the ALFF values of the left inferior frontal triangular gyrus, left middle temporal gyrus, and bilateral posterior lobes of the cerebellum in the TRD group were positively correlated with the HAMD-17 score (r = 0.432, P = 0.009; r = 0.483, P = 0.003; r = 0.360, P = 0.033). Meanwhile, the ReHo values of the left middle temporal gyrus in the TRD group were positively correlated with the HAMD-17 scores (r = 0.335, P = 0.048). On the other hand, the ALFF values of the left/right inferior frontal triangular gyrus in the nTRD group were positively correlated with the HAMD-17 score (r = 0.342, P = 0.043; r = 0.395, P = 0.018). In addition, the ReHo values of the right inferior frontal triangular gyrus in the nTRD group were positively correlated with the HAMD-17 score (r = 0.407, P = 0.015) (Table 4 and Figure 6).
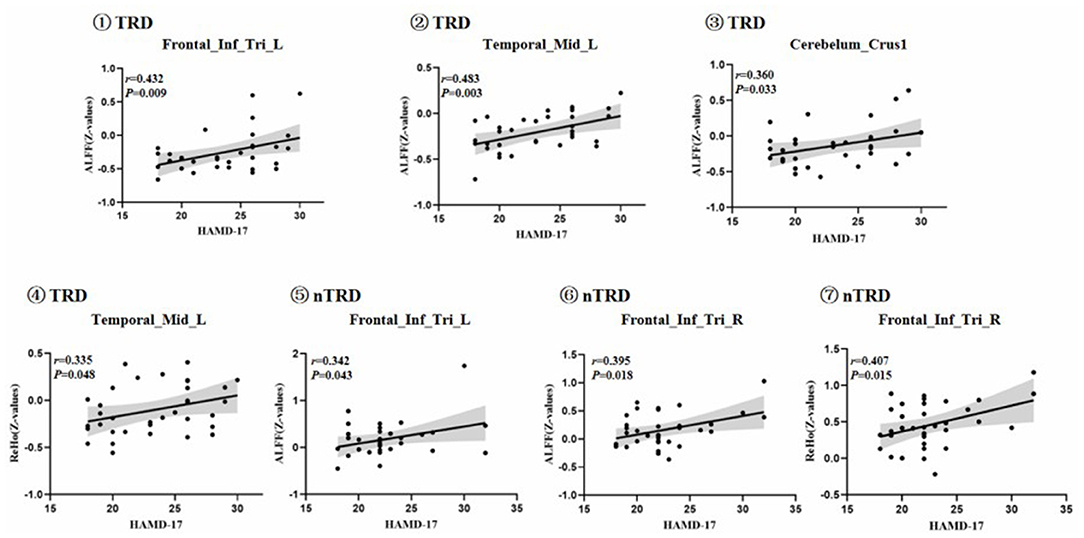
Figure 6. Positive correlation between the ALFF and ReHo of the abnormal brain regions: ①Frontal_Inf_Tri_L, Left inferior frontal triangular gyrus; ALFF value of TRD group; ②Temporal_Mid_L, left middle temporal gyrus; ALFF value of TRD group; ③Cerebelum_Crus1, bilateral posterior lobes of the cerebellum(Crus I); ALFF value of TRD group; ④Temporal_Mid_L, left middle temporal gyrus; ReHo value of TRD group; ⑤Frontal_Inf_Tri_L, Left inferior frontal triangular gyrus; ALFF value of nTRD group; ⑥Frontal_Inf_Tri_R, Right inferior frontal triangular gyrus; ALFF value of nTRD group; ⑦Frontal_Inf_Tri_R, Right inferior frontal triangular gyrus; ReHo value of nTRD group.
Discussion
To our knowledge, this is the first study of the relationship between changes in local functional brain activity in TRD and nTRD using ALFF and ReHo. The results of this study showed no significant differences in clinical depressive symptoms between the TRD and nTRD groups, but abnormal neurofunctional activity in some of the same brain regions. Compared with the nTRD group, the brain regions of ALFF and ReHo in the TRD group were more extensively altered, and the neuropathological mechanisms was more complex. This study provides a reference value for the differences in functional brain activity between TRD and nTRD.
In this study, we found TRD group had decreased ALFF/ReHo in the left/right inferior frontal triangular gyrus compared to the nTRD group. The inferior frontal triangular gyrus is located in the pre-frontal lobe, near the orbito frontal cortex (OFC) and the middle frontal gyrus, and is a key brain region for emotional and cognitive control circuits, and is associated with certain types of behavioral inhibition (39–41). The inferior frontal triangular gyrus is not only an important part of the CCN, but is also closely related to MDD (38, 39). In addition, the inferior frontal triangle gyrus is known for its involvement in speech function and language processing (42). Patients with MDD are characterized by a wide range of abnormalities in language comprehension (43). A study suggests that glutamatergic neurotransmission may play a regulatory role in language acquisition, comprehension, and production (44). Abnormal glutamate signaling plays an important role in the etiology of MDD (45, 46). More research is needed to understand how MDD-related genes affect the inferior frontal triangular gyrus. Previous studies have also found ALFF differences in the TRD group, treatment response depression (TSD) group and HC group in the inferior frontal gyrus (32). Another study found that TRD had decreased ReHo in the left inferior frontal gyrus than in the nTRD group, which is consistent with the results of this study (31). A study also found that significantly dereduced glucose metabolism in the right inferior frontal gyrus of patients with MDD was associated with the severity of pleasure deficits in depression (47). Therefore, the results of this study showed that ALFF and ReHo were decreased in the TRD group compared to the nTRD group, suggesting a more severely impaired inferior frontal triangular gyrus in the TRD group. Correlation analysis showed that ALFF/ReHo values in the left/right inferior frontal triangular gyrus of the nTRD group were positively correlated with HAMD-17 scores, suggesting that the occurrence of depressive symptoms in the nTRD group was more closely related to dysfunction in the inferior frontal triangular gyrus, and that the inferior frontal triangular gyrus was an important differential brain area between the TRD and nTRD groups.
We found that the TRD group had increased ALFF in the left middle frontal gyrus and ReHo in the right superior frontal gyrus compared to the nTRD group. The left middle frontal gyrus and the right superior frontal gyrus are key brain regions of the dorsolateral prefrontal cortex (DLPFC) and an important component of the CCN (48, 49). Several studies have shown that treatment with repetitive transcranial magnetic stimulation and transcranial direct current stimulation in the CCN, especially DLPFC, can directly improve core symptoms such as mood and cognition in MDD patients (50). Previous studies also found that the TRD group had significantly increased ALFF in the left DLPFC compared to the nTRD group, which is consistent with the results of the present study (30). Therefore, the results of this study suggest that both ALFF and ReHo are sensitive to the left middle frontal gyrus in TRD patients, suggesting that impaired function of the DLPFC is one of the causes of the complex pathological mechanisms in TRD patients.
We also found that ALFF/ReHo in the left middle temporal gyrus was decreased in the TRD group compared to the nTRD group. The middle temporal gyrus is involved in tasks related to lexical cognition and semantic understanding in humans and is important for understanding visual and auditory information (51, 52). The possibility of functional disruption of the middle temporal gyrus in MDD and schizophrenia exists (53, 54). The middle temporal gyrus is also closely related to the DMN and is the basis of the DMN in the functional role of language (51). Previous studies have also found differences in ReHo in the right middle temporal gyrus between the TRD and nTRD groups (31). A clinical study also showed that electroconvulsive therapy improved TRD and was associated with the ability to enhance high connectivity between the anterior subgenual anterior cingulate and the middle temporal gyrus (55). Therefore, both ALFF and ReHo in the left middle temporal gyrus were decreased in the TRD group in this study, suggesting that compensatory hypofunction of the left middle temporal gyrus may be an important mechanism in the pathogenesis of TRD patients. In addition, correlation analysis showed that ALFF and ReHo values of the left middle temporal gyrus in the TRD group were positively correlated with HAMD-17 scores, while this was not found in the nTRD group, suggesting that the left middle temporal gyrus may be a neuroimaging marker of TRD.
The right superior temporal gyrus also plays an important role in social-emotional processing as part of the DMN (56, 57). Patients with TRD are vulnerable to suicide risk during adolescence, and reduced volume of the right superior temporal gyrus can be a marker for suicide attempts during adolescence (57). Previous studies have found ReHo was decreased in the right superior temporal gyrus in TRD group than in the HC group (31). The results of this study showed that ALFF in the right superior temporal gyrus was increased in the TRD group compared to the nTRD group, indicating an important differential brain area between TRD and nTRD in the right superior temporal gyrus.
In this study, ALFF in the left cuneus was decreased in the TRD group compared to the nTRD group. The cuneus is part of the occipital lobe and is involved in visual perception functions (e.g., facial emotion) and plays an important role in social interaction (58, 59). Functional changes in the cuneus lobe are also closely related to MDD (60, 61). A study of an fMRI reward processing task found that adolescents with unremitting depression showed greater activation in the frontal middle gyrus and less activation in the cuneus compared to adolescents with remitting depression (62). Previous studies found that the ReHo in the left precuneus was decreased in the TRD group compared to the nTRD group (31). Another study also found decreased FC between the medial pre-frontal cortex and the cuneus in the TRD group compared to the nTRD group (63). Therefore, the results of this study suggest that abnormal function of the left cuneus is one of the reasons for the complex neuropathological mechanism of TRD.
Compared with the nTRD group, the ALFF of bilateral posterior lobes of the cerebellum in the TRD group was decreased compared to the nTRD group. In addition to the motor domain, the cerebellum is also involved in the cognitive and emotional aspects of the human body (64). Atrophy of the cerebellum often leads to cognitive and emotional symptoms, sometimes referred to as “cerebellar cognitive-emotional syndrome” (65). Cerebellar damage predisposes to language processing, aspects of executive function, and emotional dysregulation (66). Animal model studies have shown that electrical stimulation experiments also link cerebellar neural activity to depression and impulsive behavior (67). Previous studies have shown that ReHo was decreased in the bilateral cerebellum in the TRD group compared to the TSD group. Therefore, the results of the present study suggest that the posterior lobes of the cerebellum is further involved in emotion regulation in patients with TRD. In addition, correlation analysis showed a positive correlation between ALFF values and HAMD-17 scores in the bilateral posterior lobes of the cerebellum. Therefore, bilateral posterior lobes of the cerebellum may be a neuroimaging marker in patients with TRD.
Meanwhile, we also found that the TRD group had decreased ALFF/ReHo in the right inferior frontal triangular gyrus and left middle temporal gyrus compared to the HC group. Previous studies found that the TRD group had decreased ALFF in the right inferior frontal gyrus and left middle temporal gyrus compared to the HC group, which is consistent with the present study (68). However, it has also been found that the ReHo in the TRD group was decreased in the left lateral inferior frontal gyrus than in the HC group, and increased in the right middle temporal gyru scompared to the HC group, which is different from the present study (31). This variation in results may be related to differences in patient medication use, sample size and scanning and analysis methods. However, all of the above studies suggest that the inferior frontal gyrus and middle temporal gyrus are important differential brain regions between the TRD and HC groups. Interestingly, the inferior frontal triangular gyrus and middle temporal gyrus were also important differences between the TRD and nTRD groups, while no differences were found between the nTRD and HC groups in these two brain regions, therefore, further attention and research on these two brain regions are needed in the future.
Some limitations should be considered. First, although the patients in this study were included strictly according to the inclusion criteria, there were still some factors that potentially influenced the results of this study, such as the time of onset, the use of antidepressants and the duration of the disease. Second, this study focused on only one scale, the HAMD-17, and more scales need to be used in the future to focus in more detail on the correlation between cognitive, anxiety, insomnia, and somatic subtypes of symptoms with TRD and nTRD in patients with MDD, thus improving the scientific value of this study. Third, we did not clarify the gender differences between the different groups of the sample in this study, so this is an important direction for future research. Finally, Setting a GRF correction threshold voxel level of P < 0.001 is more statistically valid (69, 70), but we did not find significant cluster. Setting a threshold of P < 0.005 in this study may be slightly statistically weak, and in future studies, we will further expand the sample size and use more stringent statistical validity to enhance the scientific value of this study.
Conclusion
In conclusion, we used ALFF and ReHo based on rs-fMRI technique to preliminarily analyze the differences in local neurological functional activity between TRD and nTRD brains. We found that although the clinical symptoms were similar in the TRD and nTRD groups, there was abnormal neurofunctional activity in some of the same brain regions, and ALFF and ReHo were more extensively altered in the TRD group with more complex neuropathological mechanisms, especially in the inferior frontal triangular gyrus of the frontal lobe and the middle temporal gyrus of the temporal lobe.
Data Availability Statement
The raw data supporting the conclusions of this article will be made available by the authors, without undue reservation.
Ethics Statement
The studies involving human participants were reviewed and approved by the Ethics Committee of Guang'anmen Hospital, Chinese Academy of Traditional Chinese Medicine (NO. 2017-021-SQ). The patients/participants provided their written informed consent to participate in this study.
Author Contributions
JF conceived and designed this experiment. JS drafted the manuscript and participated in data collection and analysis. YM drew diagrams and made statistical analysis of data. LC, ZW, CG, YL, DG, XL, and KX involved in data analysis and data collection. YH performed fMRI on the subjects. XH, JT, XY, HW, and XX involved in case collection and symptom assessment of patients. All authors contributed to the article and approved the submitted version.
Funding
This research was supported by the China Academy of Chinese Medical Sciences Innovation Fund (CI2021A03301), National Natural Science Foundation of China (82174282 and 81774433), National Key Research and Development Program of China (2018YFC1705802), and Clinical Efficacy and Brain Mechanism of Transcutaneous Auricular Vagus Nerve Stimulation for patients With Mild to Moderate Depression (QYSF-2020-02).
Conflict of Interest
The authors declare that the research was conducted in the absence of any commercial or financial relationships that could be construed as a potential conflict of interest.
Publisher's Note
All claims expressed in this article are solely those of the authors and do not necessarily represent those of their affiliated organizations, or those of the publisher, the editors and the reviewers. Any product that may be evaluated in this article, or claim that may be made by its manufacturer, is not guaranteed or endorsed by the publisher.
Acknowledgments
We thank all the subjects who participated in the experiment for their support.
References
1. Hasin DS, Sarvet AL, Meyers JL, Saha TD, Ruan WJ, Stohl M, et al. Epidemiology of adult DSM-5 major depressive disorder and its specifiers in the United States. JAMA Psychiatry. (2018) 75:336–46. doi: 10.1001/jamapsychiatry.2017.4602
2. Ahmed HU, Hossain MD, Aftab A, Soron TR, Alam MT, Chowdhury MWA, et al. Suicide and depression in the world health organization South-East Asia Region: a systematic review. WHO South East Asia J Public Health. (2017) 6:60–6. doi: 10.4103/2224-3151.206167
3. Ionescu DF, Rosenbaum JF, Alpert JE. Pharmacological approaches to the challenge of treatment-resistant depression. Dialogues Clin Neurosci. (2015) 17:111–26. doi: 10.31887/DCNS.2015.17.2/dionescu
4. Gaynes BN, Lux L, Gartlehner G, Asher G, Forman-Hoffman V, Green J, et al. Defining treatment-resistant depression. Depress Anxiety. (2020) 37:134–45. doi: 10.1002/da.22968
5. Pandarakalam JP. Challenges of treatment-resistant depression. Psychiatr Danub. (2018) 30:273–84. doi: 10.24869/psyd.2018.273
6. Berlim MT, Fleck MP, Turecki G. Current trends in the assessment and somatic treatment of resistant/refractory major depression: an overview. Ann Med. (2008) 40:149–59. doi: 10.1080/07853890701769728
7. Jaffe DH, Rive B, Denee TR. The humanistic and economic burden of treatment-resistant depression in Europe: a cross-sectional study. BMC Psychiatry. (2019) 19:247. doi: 10.1186/s12888-019-2222-4
8. Ivanova JI, Birnbaum HG, Kidolezi Y, Subramanian G, Khan SA, Stensland MD. Direct and indirect costs of employees with treatment-resistant and non-treatment-resistant major depressive disorder. Curr Med Res Opin. (2010) 26:2475–84. doi: 10.1185/03007995.2010.517716
9. Crown WH, Finkelstein S, Berndt ER, Ling D, Poret AW, Rush AJ, et al. The impact of treatment-resistant depression on health care utilization and costs. J Clin Psychiatry. (2002) 63:963–71. doi: 10.4088/JCP.v63n1102
10. DiBernardo A, Lin X, Zhang Q, Xiang J, Lu L, Jamieson C, et al. Humanistic outcomes in treatment resistant depression: a secondary analysis of the STAR*D study. BMC Psychiatry. (2018) 18:352. doi: 10.1186/s12888-018-1920-7
11. Li QS, Tian C, McIntyre MH, Sun Y; 23andMe Research Team, Hinds DA, Narayan VA. Phenotypic analysis of 23andMe survey data: treatment-resistant depression from participants' perspective. Psychiatry Res. (2019) 278:173–9. doi: 10.1016/j.psychres.2019.06.011
12. Qiao J, Geng D, Qian L, Zhu X, Zhao H. Correlation of clinical features with hs-CRP in TRD patients. Exp Ther Med. (2019) 17:344–8. doi: 10.3892/etm.2018.6914
13. Enache D, Pariante CM, Mondelli V. Markers of central inflammation in major depressive disorder: a systematic review and meta-analysis of studies examining cerebrospinal fluid, positron emission tomography and post-mortem brain tissue. Brain Behav Immun. (2019) 81:24–40. doi: 10.1016/j.bbi.2019.06.015
14. Liu JJ, Wei YB, Strawbridge R, Bao Y, Chang S, Shi L, et al. Peripheral cytokine levels and response to antidepressant treatment in depression: a systematic review and meta-analysis. Mol Psychiatry. (2020) 25:339–50. doi: 10.1038/s41380-019-0474-5
15. Gong J, Wang J, Qiu S, et al. Common and distinct patterns of intrinsic brain activity alterations in major depression and bipolar disorder: voxel-based meta-analysis. Transl Psychiatry. (2020) 10:353. doi: 10.1038/s41398-020-01036-5
16. Cui P, Kong K, Yao Y, Huang Z, Shi S, Liu P, et al. Brain functional alterations in MDD patients with somatic symptoms: a resting-state fMRI study. J Affect Disord. (2021) 295:788–96. doi: 10.1016/j.jad.2021.08.143
17. Mwansisya TE, Hu A, Li Y, Chen X, Wu G, Huang X, et al. Task and resting-state fMRI studies in first-episode schizophrenia: A systematic review. Schizophr Res. (2017) 189:9–18. doi: 10.1016/j.schres.2017.02.026
18. Dapretto M, Davies MS, Pfeifer JH, Scott AA, Sigman M, Bookheimer SY, et al. Understanding emotions in others: mirror neuron dysfunction in children with autism spectrum disorders. Nat Neurosci. (2006) 9:28–30. doi: 10.1038/nn1611
19. Drysdale AT, Grosenick L, Downar J, Dunlop K, Mansouri F, Meng Y, et al. Resting-state connectivity biomarkers define neurophysiological subtypes of depression. Nat Med. (2017) 23:264. doi: 10.1038/nm.4246
20. Borserio BJ, Sharpley CF, Bitsika V, Sarmukadam K, Fourie PJ, Agnew LL. Default mode network activity in depression subtypes. Rev Neurosci. (2021) 32:597–613. doi: 10.1515/revneuro-2020-0132
21. Guo CC, Hyett MP, Nguyen VT, Parker GB, Breakspear MJ. Distinct neurobiological signatures of brain connectivity in depression subtypes during natural viewing of emotionally salient films. Psychol Med. (2016) 46:1535–45. doi: 10.1017/S0033291716000179
22. Fagiolini A, Kupfer DJ. Is treatment-resistant depression a unique subtype of depression? Biol Psychiatry. (2003) 53:640–8. doi: 10.1016/S0006-3223(02)01670-0
23. Zang Y, Jiang T, Lu Y, He Y, Tian L. Regional homogeneity approach to fMRI data analysis. Neuroimage. (2004) 22:394–400. doi: 10.1016/j.neuroimage.2003.12.030
24. Zang YF, He Y, Zhu CZ, Cao QJ, Sui MQ, Liang M, et al. Altered baseline brain activity in children with ADHD revealed by resting-state functional MRI. Brain Dev. (2007) 29:83–91. doi: 10.1016/j.braindev.2006.07.002
25. Shen Z, Zhu J, Ren L, Qian M, Shao Y, Yuan Y, et al. Aberrant amplitude low-frequency fluctuation (ALFF) and regional homogeneity (ReHo) in generalized anxiety disorder (GAD) and their roles in predicting treatment remission. Ann Transl Med. (2020) 8:1319. doi: 10.21037/atm-20-6448
26. Yue Y, Jiang Y, Shen T, Pu J, Lai HY, Zhang B. ALFF and ReHo mapping reveals different functional patterns in early- and late-onset parkinson's disease. Front Neurosci. (2020) 14:141. doi: 10.3389/fnins.2020.00141
27. Chrobak AA, Bohaterewicz B, Sobczak AM, Marszał-Wiśniewska M, Tereszko A, Krupa A, et al. Time-Frequency characterization of resting brain in bipolar disorder during Euthymia-a preliminary study. Brain Sci. (2021) 11:599. doi: 10.3390/brainsci11050599
28. Wei HL, Tian T, Zhou GP, et al. Disrupted dynamic functional connectivity of the visual network in episodic patients with migraine without aura. Neural Plast. (2022) 2022:9941832. doi: 10.1155/2022/9941832
29. Guo WB, Sun XL, Liu L, Xu Q, Wu RR, Liu ZN, et al. Disrupted regional homogeneity in treatment-resistant depression: a resting-state fMRI study. Prog Neuropsychopharmacol Biol Psychiatry. (2011) 35:1297–302. doi: 10.1016/j.pnpbp.2011.02.006
30. Zhang A, Li G, Yang C, Liu P, Wang Y, Kang L, et al. Alterations of amplitude of low-frequency fluctuation in treatment-resistant versus non-treatment-resistant depression patients. Neuropsychiatr Dis Treat. (2019) 15:2119–28. doi: 10.2147/NDT.S199456
31. Wu QZ, Li DM, Kuang WH, Zhang TJ, Lui S, Huang XQ, et al. Abnormal regional spontaneous neural activity in treatment-refractory depression revealed by resting-state fMRI. Hum Brain Mapp. (2011) 32:1290–9. doi: 10.1002/hbm.21108
32. Guo WB, Liu F, Xue ZM, Xu XJ, Wu RR, Ma CQ, et al. Alterations of the amplitude of low-frequency fluctuations in treatment-resistant and treatment-response depression: a resting-state fMRI study. Prog Neuropsychopharmacol Biol Psychiatry. (2012) 37:153–60. doi: 10.1016/j.pnpbp.2012.01.011
33. Suo N, He B, Cui S, Yang Y, Wang M, Yuan Q, et al. Convergent functional changes of default mode network in mild cognitive impairment using activation likelihood estimation. Front Aging Neurosci. (2021) 13:708687. doi: 10.3389/fnagi.2021.708687
34. Li CT, Lin CP, Chou KH, Chen IY, Hsieh JC, Wu CL, et al. Structural and cognitive deficits in remitting and non-remitting recurrent depression: a voxel-based morphometric study. Neuroimage. (2010) 50:347–56. doi: 10.1016/j.neuroimage.2009.11.021
35. Raichle ME, MacLeod AM, Snyder AZ, Powers WJ, Gusnard DA, Shulman GL, et al. default mode of brain function. Proc Natl Acad Sci U S A. (2001) 98:676–82. doi: 10.1073/pnas.98.2.676
36. Raichle ME, Snyder AZ. A default mode of brain function: a brief history of an evolving idea. Neuroimage. (2007) 37:1083–99. doi: 10.1016/j.neuroimage.2007.02.041
37. Depping MS, Wolf ND, Vasic N, Sosic-Vasic Z, Schmitgen MM, Sambataro F, et al. Aberrant resting-state cerebellar blood flow in major depression. J Affect Disord. (2018) 226:227–31. doi: 10.1016/j.jad.2017.09.028
38. Pan F, Xu Y, Zhou W, Chen J, Wei N, Lu S, et al. Disrupted intrinsic functional connectivity of the cognitive control network underlies disease severity and executive dysfunction in first-episode, treatment-naive adolescent depression. J Affect Disord. (2020) 264:455–63. doi: 10.1016/j.jad.2019.11.076
39. Du J, Rolls ET, Cheng W, et al. Functional connectivity of the orbitofrontal cortex, anterior cingulate cortex, and inferior frontal gyrus in humans. Cortex. (2020) 123:185–99. doi: 10.1016/j.cortex.2019.10.012
40. Briggs RG, Lin YH, Dadario NB, Kim SJ, Young IM, Bai MY, et al. Anatomy and white matter connections of the middle frontal Gyrus. World Neurosurg. (2021) 150:e520–9. doi: 10.1016/j.wneu.2021.03.045
41. Badre D, Nee DE. Frontal cortex and the hierarchical control of behavior. Trends Cogn Sci. (2018) 22:170–88. doi: 10.1016/j.tics.2017.11.005
42. Greenlee JD, Oya H, Kawasaki H, Volkov IO, Severson MA 3rd, Howard MA 3rd, et al. Functional connections within the human inferior frontal gyrus. J Comp Neurol. (2007) 503:550–9. doi: 10.1002/cne.21405
43. Zhuo C, Zhou C, Lin X, Tian H, Wang L, Chen C, et al. Common and distinct global functional connectivity density alterations in drug-naïve patients with first-episode major depressive disorder with and without auditory verbal hallucination. Prog Neuropsychopharmacol Biol Psychiatry. (2020) 96:109738. doi: 10.1016/j.pnpbp.2019.109738
44. Li W, Kutas M, Gray JA, Hagerman RH, Olichney JM. The role of glutamate in language and language disorders - evidence from ERP and pharmacologic studies. Neurosci Biobehav Rev. (2020) 119:217–41. doi: 10.1016/j.neubiorev.2020.09.023
45. Bernard R, Kerman IA, Thompson RC, Jones EG, Bunney WE, Barchas JD, et al. Altered expression of glutamate signaling, growth factor, and glia genes in the locus coeruleus of patients with major depression. Mol Psychiatry. (2011) 16:634–46. doi: 10.1038/mp.2010.44
46. Pham TH, Gardier AM. Fast-acting antidepressant activity of ketamine: highlights on brain serotonin, glutamate, and GABA neurotransmission in preclinical studies. Pharmacol Ther. (2019) 199:58–90. doi: 10.1016/j.pharmthera.2019.02.017
47. Su H, Zuo C, Zhang H, Jiao F, Zhang B, Tang W, et al. Regional cerebral metabolism alterations affect resting-state functional connectivity in major depressive disorder. Quant Imaging Med Surg. (2018) 8:910–24. doi: 10.21037/qims.2018.10.05
48. Zhang B, Qi S, Liu S, Liu X, Wei X, Ming D. Altered spontaneous neural activity in the precuneus, middle and superior frontal gyri, and hippocampus in college students with subclinical depression. BMC Psychiatry. (2021) 21:280. doi: 10.1186/s12888-021-03292-1
49. Li W, Li Y, Yang W, Zhang Q, Wei D, Li W, et al. Brain structures and functional connectivity associated with individual differences in Internet tendency in healthy young adults. Neuropsychologia. (2015) 70:134–44. doi: 10.1016/j.neuropsychologia.2015.02.019
50. Amidfar M, Ko YH, Kim YK. Neuromodulation and cognitive control of emotion. Adv Exp Med Biol. (2019) 1192:545–64. doi: 10.1007/978-981-32-9721-0_27
51. Briggs RG, Tanglay O, Dadario NB, Young IM, Fonseka RD, Hormovas J, et al. The unique fiber anatomy of middle temporal gyrus default mode connectivity. Oper Neurosurg (Hagerstown). (2021) 21:E8–E14. doi: 10.1093/ons/opab109
52. Xu J, Wang J, Fan L, Li H, Zhang W, Hu Q, et al. Tractography-based parcellation of the human middle temporal gyrus. Sci Rep. (2015) 5:18883. doi: 10.1038/srep18883
53. Jiang J, Chen X, Qiu Y, Wang B, Yu Y, Zhu ZZ, et al. Hyperconnectivity between the posterior cingulate and middle frontal and temporal gyrus in depression: Based on functional connectivity meta-analyses. Brain Imaging Behav. (2022) 23:61. doi: 10.1007/s11682-022-00628-7
54. Joo SW, Chon MW, Rathi Y, Shenton ME, Kubicki M, Lee J. Abnormal asymmetry of white matter tracts between ventral posterior cingulate cortex and middle temporal gyrus in recent-onset schizophrenia. Schizophr Res. (2018) 192:159–66. doi: 10.1016/j.schres.2017.05.008
55. Subramanian S, Lopez R, Zorumski CF, Cristancho P. Electroconvulsive therapy in treatment resistant depression. J Neurol Sci. (2022) 434:120095. doi: 10.1016/j.jns.2021.120095
56. Buckner RL, Andrews-Hanna JR, Schacter DL. The brain's default network: anatomy, function, and relevance to disease. Ann N Y Acad Sci. (2008) 1124:1–38. doi: 10.1196/annals.1440.011
57. McLellan Q, Wilkes TC, Swansburg R, Jaworska N, Langevin LM, MacMaster FP. History of suicide attempt and right superior temporal gyrus volume in youth with treatment-resistant major depressive disorder. J Affect Disord. (2018) 239:291–4. doi: 10.1016/j.jad.2018.07.030
58. Fusar-Poli P, Placentino A, Carletti F, et al. Functional atlas of emotional faces processing: a voxel-based meta-analysis of 105 functional magnetic resonance imaging studies. J Psychiatry Neurosci. (2009) 34:418–32. doi: 10.1111/j.1365-2850.2009.01434.x
59. Parker JG, Zalusky EJ, Kirbas C. Functional MRI mapping of visual function and selective attention for performance assessment and presurgical planning using conjunctive visual search. Brain Behav. (2014) 4:227–37. doi: 10.1002/brb3.213
60. Groves SJ, Pitcher TL, Melzer TR, et al. Brain activation during processing of genuine facial emotion in depression: Preliminary findings. J Affect Disord. (2018) 225:91–6. doi: 10.1016/j.jad.2017.07.049
61. Farah R, Greenwood P, Dudley J, Hutton J, Ammerman RT, Phelan K„ et al. Maternal depression is associated with altered functional connectivity between neural circuits related to visual, auditory, and cognitive processing during stories listening in preschoolers. Behav Brain Funct. (2020) 16:5. doi: 10.1186/s12993-020-00167-5
62. Fischer AS, Ellwood-Lowe ME, Colich NL, Cichocki A, Ho TC, Gotlib IH. Reward-circuit biomarkers of risk and resilience in adolescent depression. J Affect Disord. (2019) 246:902–9. doi: 10.1016/j.jad.2018.12.104
63. de Kwaasteniet BP, Rive MM, Ruhé HG, Schene AH, Veltman DJ, Fellinger L, et al. Decreased resting-state connectivity between neurocognitive networks in treatment resistant depression. Front Psychiatry. (2015) 6:28. doi: 10.3389/fpsyt.2015.00028
64. Depping MS, Schmitgen MM, Kubera KM, Wolf RC. Cerebellar contributions to major depression. Front Psychiatry. (2018) 9:634. doi: 10.3389/fpsyt.2018.00634
65. Schmahmann JD, Weilburg JB, Sherman JC. The neuropsychiatry of the cerebellum - insights from the clinic. Cerebellum. (2007) 6:254–67. doi: 10.1080/14734220701490995
66. Hoche F, Guell X, Vangel MG, Sherman JC, Schmahmann JD. The cerebellar cognitive affective/Schmahmann syndrome scale. Brain. (2018) 141:248–70. doi: 10.1093/brain/awx317
67. Huguet G, Kadar E, Temel Y, Lim LW. Electrical stimulation normalizes c-Fos expression in the deep cerebellar nuclei of depressive-like rats: implication of antidepressant activity. Cerebellum. (2017) 16:398–410. doi: 10.1007/s12311-016-0812-y
68. Guo WB, Liu F, Chen JD, Gao K, Xue ZM, Xu XJ, et al. Abnormal neural activity of brain regions in treatment-resistant and treatment-sensitive major depressive disorder: a resting-state fMRI study. J Psychiatr Res. (2012) 46:1366–73. doi: 10.1016/j.jpsychires.2012.07.003
69. Roiser JP, Linden DE, Gorno-Tempinin ML, Moran RJ, Dickerson BC, Grafton ST. Minimum statistical standards for submissions to neuroimage: clinical. Neuroimage Clin. (2016) 12:1045–47. doi: 10.1016/j.nicl.2016.08.002
Keywords: treatment-resistant depression, non-treatment-resistant depression, fMRI, amplitude of low-frequency fluctuation, regional homogeneity
Citation: Sun J, Ma Y, Chen L, Wang Z, Guo C, Luo Y, Gao D, Li X, Xu K, Hong Y, Hou X, Tian J, Yu X, Wang H, Fang J and Xiao X (2022) Altered Brain Function in Treatment-Resistant and Non-treatment-resistant Depression Patients: A Resting-State Functional Magnetic Resonance Imaging Study. Front. Psychiatry 13:904139. doi: 10.3389/fpsyt.2022.904139
Received: 25 March 2022; Accepted: 23 June 2022;
Published: 22 July 2022.
Edited by:
Masahiro Takamura, Shimane University, JapanReviewed by:
Thomas Frodl, University Hospital RWTH Aachen, GermanyYifeng Rang, South China Agricultural University, China
Copyright © 2022 Sun, Ma, Chen, Wang, Guo, Luo, Gao, Li, Xu, Hong, Hou, Tian, Yu, Wang, Fang and Xiao. This is an open-access article distributed under the terms of the Creative Commons Attribution License (CC BY). The use, distribution or reproduction in other forums is permitted, provided the original author(s) and the copyright owner(s) are credited and that the original publication in this journal is cited, in accordance with accepted academic practice. No use, distribution or reproduction is permitted which does not comply with these terms.
*Correspondence: Jiliang Fang, RmFuZ21naEAxNjMuY29t; Xue Xiao, eGlhb3h1ZXBzeUBzaW5hLmNvbQ==
†These authors have contributed equally to this work and share first authorship