- 1Shanghai Key Laboratory of Forensic Medicine, Key Lab of Forensic Science, Ministry of Justice, Shanghai Forensic Service Platform, Academy of Forensic Science, Shanghai, China
- 2Sichuan Provincial Center for Mental Health, Sichuan Academy of Medical Science, Sichuan Provincial People's Hospital, Chengdu, China
- 3Hongkou Mental Health Center, Shanghai, China
Most patients with neurocognitive disorders after traumatic brain injury (TBI) show executive dysfunction, in which the pre-frontal cortex (PFC) plays an important role. However, less objective evaluation technique could be used to assess the executive dysfunction in these patients. Functional near-infrared spectroscopy (fNIRS), which is a non-invasive technique, has been widely used in the study of psychiatric disorders, cognitive dysfunction, etc. The present study aimed to explore whether fNIRS could be a technique to assess the damage degree of executive function in patients with neurocognitive disorders after TBI by using the Stroop and N-back tasks in PFC areas. We enrolled 37 patients with neurocognitive disorders after TBI and 60 healthy controls. A 22-channel fNIRS device was used to record HbO during Stroop, 1-back and 2-back tasks. The results showed that patients made significantly more errors and had longer response times than healthy controls. There were statistically significant differences in HbO level variation in bilateral frontopolar, bilateral inferior frontal gyrus and left middle temporal gyrus during Stroop color word consistency tasks and in left frontopolar during Stroop color word inconsistency tasks. During 2-back tasks, there were also statistically significant differences in HbO level variation in bilateral frontopolar, bilateral inferior frontal gyrus, bilateral dorsolateral pre-frontal cortex. According to brain activation maps, the patients exhibited lower but more widespread activation during the 2-back and Stroop color word consistency tasks. The fNIRS could identify executive dysfunction in patients with neurocognitive disorders after TBI by detecting HbO levels, which suggested that fNIRS could be a potential objective evaluation technique in neurocognitive disorders after TBI.
Introduction
Executive function (EF) refers to the cognitive neural mechanism used by humans in the process of completing a target task (1). As part of cognitive function, EF represents the ability to control the processing of advanced behavior. The impairment of EF leads to neuropsychological defects, such as difficulties in making plans, forming concepts, decision-makings, cognitive flexibility and controlling one's own actions (2). Previous studies have found that the pre-frontal cortex (PFC) plays an important role in EF, and the initial discoveries about EF arose from the exploration of patients with damage to the PFC (3–6). The number of patients with traumatic brain injury (TBI) is increasing yearly for various reasons, such as car accidents and falls. Indeed, it has been found that 77% of patients with craniocerebral injury caused by traffic accidents experience different degrees of neurocognitive disorders, including post-traumatic brain syndrome (PTS) and organic injury (7). Epidemiological evidence indicates that ~14–30% of patients with TBI have varying degrees of cognitive impairment, and ~90% may suffer spontaneous sequelae remission within 2 weeks post-injury, but some impairments may last for several weeks (8). In the weeks to months post-injury, even in mild TBI, some patients still experience symptoms, such as persistent headaches, dizziness, attention deficits, hypomnesia and cognitive impairment, and the most common symptom in patients with TBI is executive dysfunction (9). However, patients tend to focus on the physical trauma and ignore the impairment of EF in the early stages. Furthermore, the TBI patients with cognitive impairments are more likely to develop Alzheimer's disease (AD) (10), which suggests that effective cognitive interventions and imaging tools to identify the patients with impairments in cognitive function, especially mild cognitive impairment (MCI) should be developed.
In recent years, the field of functional near-infrared spectroscopy (fNIRS) has grown (11). The fNIRS is a non-invasive technique with superior temporal and spatial resolution, which provides the real-time measurement of haemodynamic changes in neural regions under the surface of the brain. The fNIRS has been widely applied in the field of cognitive processing, such as in relation to memory (12), attention processing (13), thinking (14), and social cognition (15). Patients with TBI can also be tested using fNIRS to elucidate deficits in memory or attention within the frontal and temporal lobes. According to the literature, selective attention, inhibitory control, memory, and problem-solving are commonly affected in these patients (16, 17). However, the recent literature also highlights contradictory findings regarding brain activation in TBI patients based on various neuroimaging tools, including functional magnetic resonance imaging (fMRI), electroencephalography (EEG), and event-related potentials (ERPs). Khetani et al. (18) examined cerebral activation using fMRI during a working memory task in the first month following pediatric mild TBI and observed that children with pediatric post-concussional syndrome (PPCS) had decreased activation in the posterior cingulate and pre-cuneus during the one-back task. Westfall et al. (19) reported increased brain activation during most difficult working memory load condition in adolescents after TBI. Moreover, using fMRI, Chen et al. (20) reported task-related activation in a subset of regions during a working memory task in symptomatic concussed athletes. Problematically, studies in the field of TBI have mainly focused on deficits in sustained attention or working memory (20–22). A limited number of studies have examined both memory and sustained attention together, but these failed to address changes in neural activity (23). Additionally, previous studies have explored post-injury cognitive function by means of techniques such as ERPs, eye movement (EM), and EEG (12), but these techniques have poor spatial resolution. Therefore, the advantages of fNIRS in the study of cognitive function and its good spatial and temporal resolution warrant further discussion. Moreover, although some researchers have used fNIRS in patients with TBI in the past and focused on the clinical treatment stage, including comparisons during the rehabilitation process. Few studies about fNIRS in neurocognitive disorder after TBI have been conducted when patients seemed to achieve clinical cure, but still suffered persistent executive dysfunction for more than 6 months after TBI.
The study uses a combination of fNIRS with the Stroop and N-back tasks which are considered as effective methods to detect the damage degree of EF in patients with MCI (24). The present study aimed to explore whether fNIRS could be a technique to assess the damage degree of executive function in patients with neurocognitive disorders after TBI by using the Stroop and N-back tasks in PFC areas.
Materials and methods
Participants
In total, 60 healthy controls and 37 patients with neurocognitive disorders after TBI were enrolled in the study. Patients were enrolled from September 2019 to November 2020 in the Academy of Forensic Science. Inclusion criteria were as follows: (1) diagnosis of neurocognitive disorder after TBI according to the International Statistical Classification of Diseases and Related Health Problems, Tenth Revision (ICD-10), criterion F07 and Z87.820; (2) mild to moderate traumatic brain injury; (3) conform to the clinical treatment completion criteria 6 months or more after traumatic brain injury; (4) aged between 18 and 60 years old; (5) right-handedness. Mild or moderate injury was defined as an initial Glasgow coma scale (GCS) score between 12 and 15. Patients with a history of chronic disease, mental illness, and substance addictions were excluded. The healthy controls were made up of staff and students in the Academy of Forensic Science. For healthy controls, the following inclusion and exclusion criteria were utilized: (1) aged between 18 and 60 years old; (2) right-handed; (3) no mental disorders; (4) no family history of mental disorders; (5) no organic brain diseases; (6) no alcohol or substance use; (7) no significant medical history; and (8) no use of psychotropic drugs.
The study was approved by the ethics committee of the Academy of Forensic Science. All the methods and procedures of the study were performed in accordance with the criteria laid out by the Declaration of Helsinki and other relevant national and international requirements for human research. All participants signed written informed consent prior to engaging in the study.
Scales and intelligence quotient (IQ) assessment
Self-designed research forms were used to collect the demographic and clinical characteristics of the participants. The activity of daily living (ADL) scale and social disability screening schedule (SDSS) scale were completed according to the patients' medical records and interviews conducted by the researchers. Patients with total ADL scores >16 are deemed to have impairments in their daily life activities, with higher scores indicating more severe impairment. Patients with total SDSS scores >2 are deemed to have social dysfunction, with higher scores indicating more severe impairment of social function. The Wechsler Intelligence Test (Chinese version) was used to collect the IQ levels of the participants.
Stroop tasks
The classic color word Stroop experiment was fully computer-controlled and programmed with E-Prime experimental software. Each trial started with the presentation of a red fixation cross for 500 ms and a guide with information about the tasks for 2,000 ms. The Stroop task was revised for our study, to include color word consistency tests and color word inconsistency tests (25). The rest time was used as the baseline. During the task, a color word presented in colored ink was shown on a computer screen. The participants were instructed to press a key (left-handed F when the color word was the same as the ink color or right-handed J when the color word was inconsistent with the ink color) while simultaneously stating the ink color. In color word consistency tests, the color word was the same as the ink color in 91% of the trials. In the color word inconsistency tests, the color word was different from the ink color in 89% of the trials. After the key press action, the word on the screen changed. The two tests were presented in a block design and conducted three times, each with a break period of 30 s between blocks. A red fixation cross was presented on the computer screen during the break period, and the participant was guided to relax and focus on the screen. All responses were digitally recorded.
N-back tasks
A combined 1-back task and 2-back task were used to quantify working memory (26) in this study. The task was fully computer-controlled and programmed with E-Prime experimental software. Each trial started with the presentation of a red fixation cross for 500 ms and a guide with information about the tasks for 2,000 ms. During the N-back task, a series of stimuli were presented, and the participant was instructed to respond to the stimuli that they saw “N” items-back (1-back or 2-back) (27). In the 1-back condition, the participants were required to memorize the numbers and determine whether the number was the same as the previous one. They were instructed to press the left-handed F key when the number was the same as the previous one, and right-handed J key when the number was different from the previous one. In the 2-back conditions, the participants were instructed to judge whether the number was consistent with the number presented two items before. A 30 s period was inserted between the trials. The stimuli were numbers (1–9), which were pseudo-randomly ordered to ensure that there were no repeats (9–9) and no ordered series (1–2–3). The numbers 1–9 randomly appeared 23 times in the 1-back condition and 25 times in the 2-back condition. As an example, in the 1-back version, the series “1–3–6–3……” may be shown to a participant. For this list of numbers, when they saw 3, they were required to press the right-handed J and when they saw 6, they were required to press the right-handed J, etc. In the 2-back version with the same list of numbers “1–3–6–3 ……,” when the participant saw 6, they were required to press the right-handed J and when they saw 3, they were required to press the left-handed F, always keeping in mind the number presented two numbers previously. All responses were digitally recorded.
The FNIRS tests
The tests included Stroop or N-back tasks, and each task appeared three times. All participants took part in both types of tasks. No fNIRS data was acquired during the practice phases. At the beginning, the 10 s baselines were used to normalize the data. Additionally, 30 s rest blocks were added between stimuli and during this time, the fNIRS data were saved, and the participants were instructed to rest.
The HbO data were acquired using the LABNIRS (Shimazu company, Japan). The 16 probes combined into 22 channels and the 22-channels optical array was placed across the forehead providing bilateral pre-frontal lobe and dorsolateral pre-frontal lobe coverage. The lower side of the probe was flush with the upper side of the eyebrow. The locations of the 22 channels are shown in Figure 1. The FASTRAK three dimensions magnetic digitizer (Polhemus companies in the United States) and the three dimensions position measurement system software (version 4.1.0.0 Shimadzu Corporation, Japan) were used to determine the three-dimensional spatial position of each probe, according to the Montreal Neurological Institute (MNI) reference coordinate system (28).
Statistical analyzes
The fNIRS data were collected by LABNIRS and pre-processed by denoising algorithms. Heartbeat and respiration noise signals were removed by using a 0.14–0.17 Hz finite impulse response low-pass filter. The instrumental noise and physiological interference from the body were processed by denoising algorithms (29). The concentration change of HbO was calculated by the modified Beer-lambert law. The HbO data were processed and analyzed by NIRS-SPM, a near-infrared data processing package in Matlab software (The MathWorks, USA). Spatial maps were estimated by β values in following general linear model (GLM).
Y = observed fNIRS data; X = convolution of design matrix and hemodynamic response function; ε = residuals
Spatial maps were created by three contrast-model-matrices in NIRS-SPM. A one sample t-test was used to create spatial maps of significant activation for the patients and controls, respectively. Threshold images for the resulting group data used an alpha of 0.05. Areas of significant task-based activity are described in terms of three-dimensional spatial position data.
The data were analyzed by IBM Statistical Product and Service Solutions version 22.0 (IBM SPSS 22.0) and Matlab software. The statistical significance level was set at P < 0.05 correction thresholds (corrected by FDR). All data were presented as mean ± standard deviation (SD). Categorical data were compared using chi-square tests. The Student's t-test was used to compare the normally distributed variables between patients and healthy controls. The Mann-Whitney U-test was used to compare the non-normally distributed variables between patients and healthy controls.
Results
Demographic characteristics
A total of 37 patients and 60 healthy controls were enrolled. During the study, four patients and five healthy controls did not complete the tasks. In total, 30 patients and 55 healthy controls completed the study. There were no significant differences between the healthy controls and the patients in age, sex and education. The IQ levels of patients were significantly lower than the healthy controls. The ADL and SDSS scores of patients were significantly higher than the healthy controls (Table 1).
Behavioral data during Stroop and N-back tests
The response times of patients were significantly longer than healthy controls during the Stroop color word consistency and color word inconsistency tests (color word consistency: 2,301.03 ± 1,035.62 vs. 1,298.19 ± 559.72 ms, t = 5.051, P = 0.001; color word inconsistency: 3,150.44 ± 1,241.66 vs. 1,964.98 ± 727.85 ms, t = 4.902, P = 0.001). The error numbers of the patients were significantly higher than the healthy controls during both the color word consistency and color word inconsistency tests (color word consistency: 10.00 ± 7.59 vs. 3.19 ± 6.74, t = 4.125, P = 0.001; color word inconsistency: 14.15 ± 9.44 vs. 5.43 ± 8.28, t = 4.362, P = 0.001).
The response times of both patients and healthy controls were significantly shorter during the color word consistency tests compared to the color word inconsistency tests (patients: t = −7.820, P = 0.001; controls: t = −10.599, P = 0.001). Additionally, the error numbers of patients and healthy controls were significantly lower during the color word consistency tests compared to the color word inconsistency tests (patients: t = −4.414, P = 0.001; controls: t = −5.529, P = 0.001).
The response times of the patients were significantly longer than the healthy controls during the 1-back and 2-back tests (1-back: 1,825.12 ± 891.01 vs. 1,337.42 ± 904.97 ms, t = 2.520, P = 0.014; 2-back: 3,074.63 ± 143.65 vs. 2,426.06 ± 1,829.00 ms, t = 2.564, P = 0.012). The error numbers of the patients were significantly higher than the healthy controls during the 1-back and 2-back tests (1-back: 9.03 ± 6.14 vs. 6.08 ± 4.94, t = 3.343, P = 0.001; 2-back: 14.19 ± 9.11 vs. 7.10 ± 5.95, t = 3.730, P = 0.001).
No significant differences in error number between the 1-back and 2-back tests were observed for healthy controls (t = −1.903, P = 0.056), but the error numbers of patients were significantly lower during the 1-back tests compared to 2-back tests (t = −4.632, P < 0.001). The response times of both patients and healthy controls were significantly shorter during the 1-back tests compared to 2-back tests (patients: t = −6.387, P = 0.001; controls: t = −7.139, P = 0.001).
The relationships between channels and brain areas
The relationships between channels and brain areas according to the FASTRAK three dimensions magnetic digitizer and the three dimensions position measurement system software were shown in Table 2.
The FNIRS results during Stroop and N-back tasks
In the Stroop tasks, there were significant differences in ΔHbO levels in channels 3, 4, 8, 9, 11, 12, 14, 15, and 21 between patients and healthy controls during the color word consistency tests. However, there were only significant differences in channels 3, 4, and 12 during the color word inconsistency tests (Table 3). There were no significant differences in other channels during the Stroop tasks.
In the N-back tasks, no significant differences were found between patients and healthy controls during the 1-back tasks. During 2-back tasks, there were significant differences in ΔHbO levels in channels 2, 3, 7, 9, 10, 11, 14, 15, 18, 21, and 22 between patients and healthy controls (Table 4).
The brain activation maps during Stroop and N-back tasks for patients and healthy controls are shown in Figures 2–5.
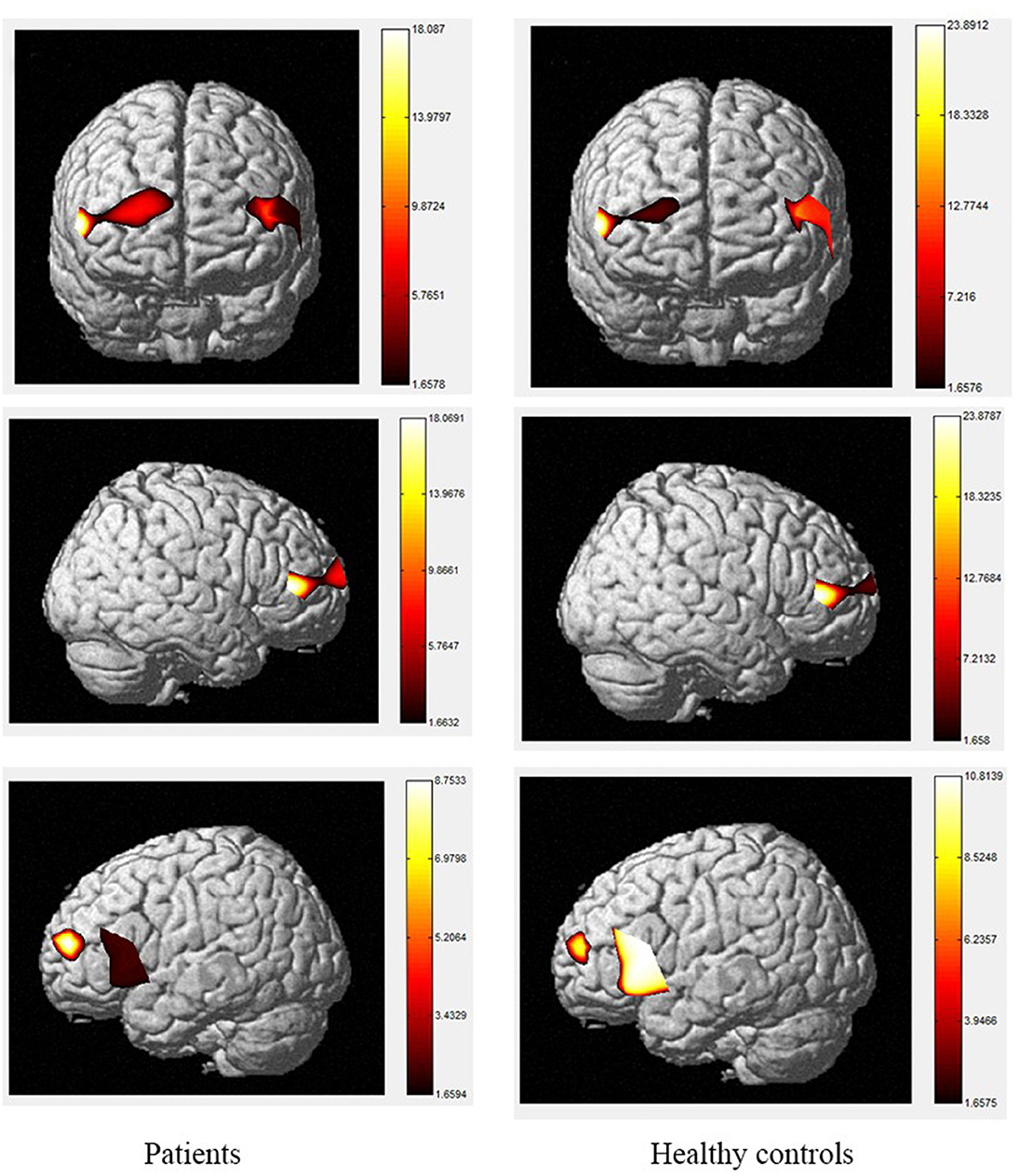
Figure 2. The activation of brain areas during the color word consistency Stroop tasks between patients and healthy controls. The color bars in right sides mean the activated strength of brain areas.
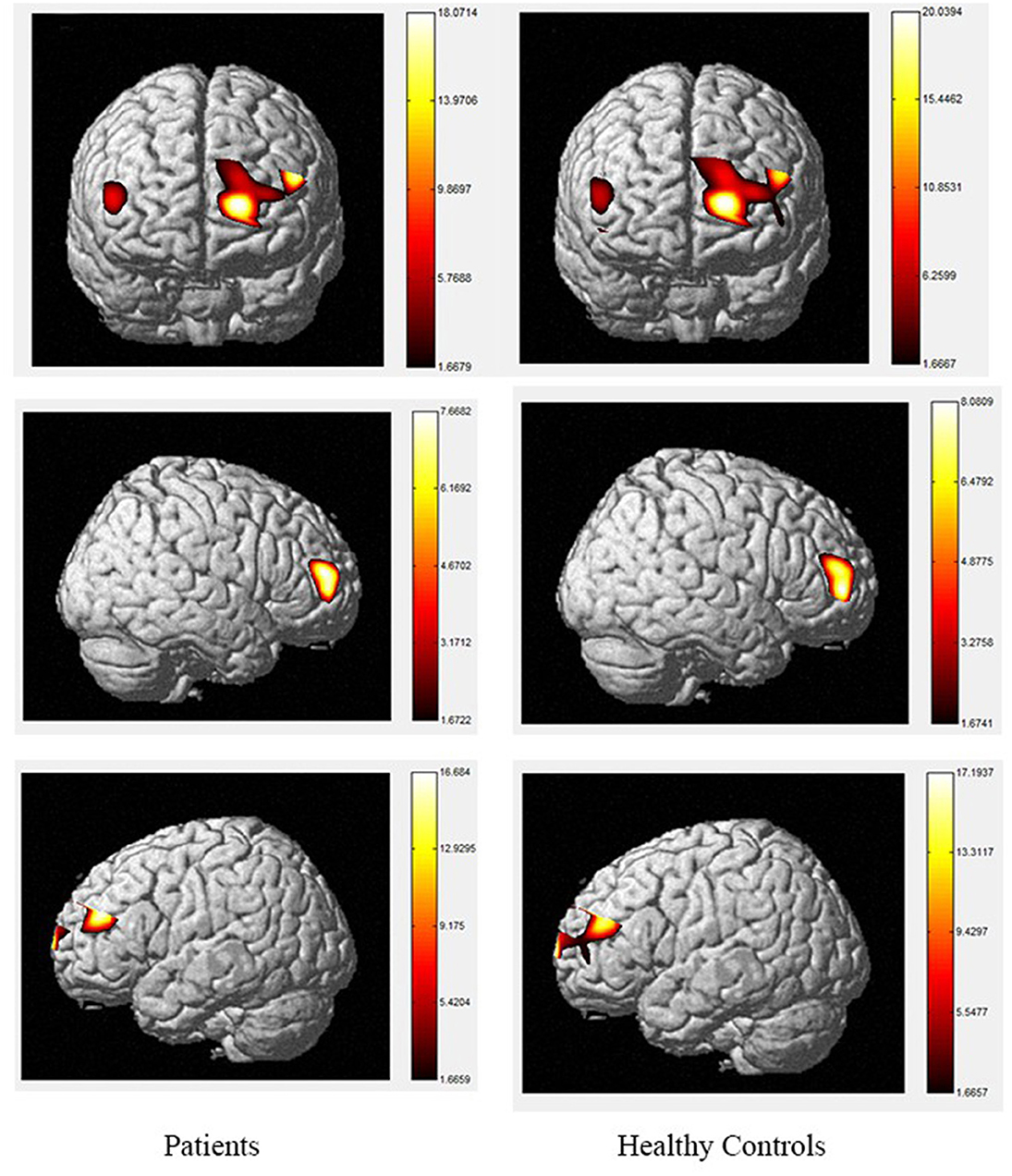
Figure 3. The activation of brain areas during the color word inconsistency Stroop tasks between patients and healthy controls. The color bars in right sides mean the activated strength of brain areas.
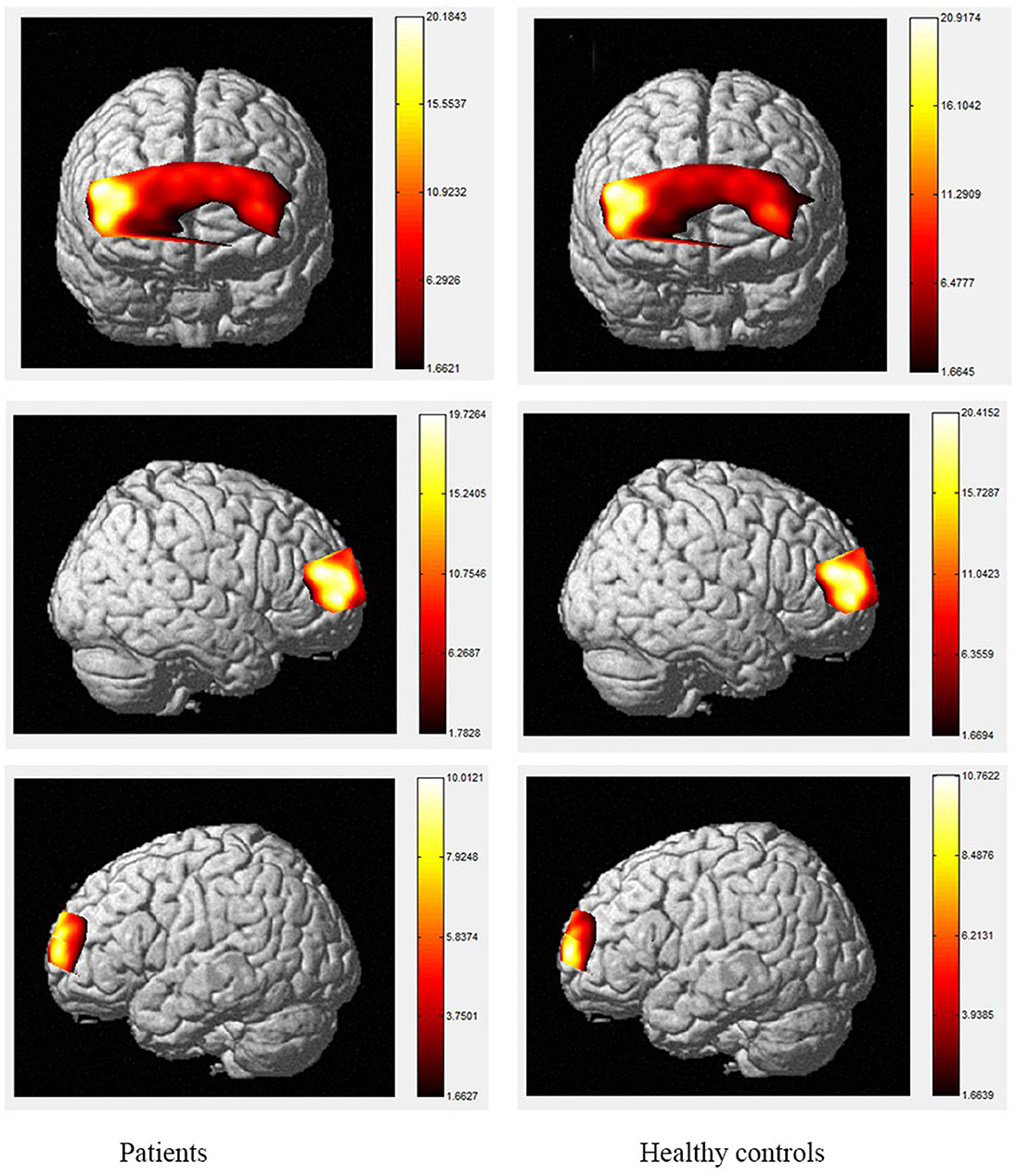
Figure 4. The activation of brain areas during 1-back tasks between patients and healthy controls. The color bars in right sides mean the activated strength of brain areas.
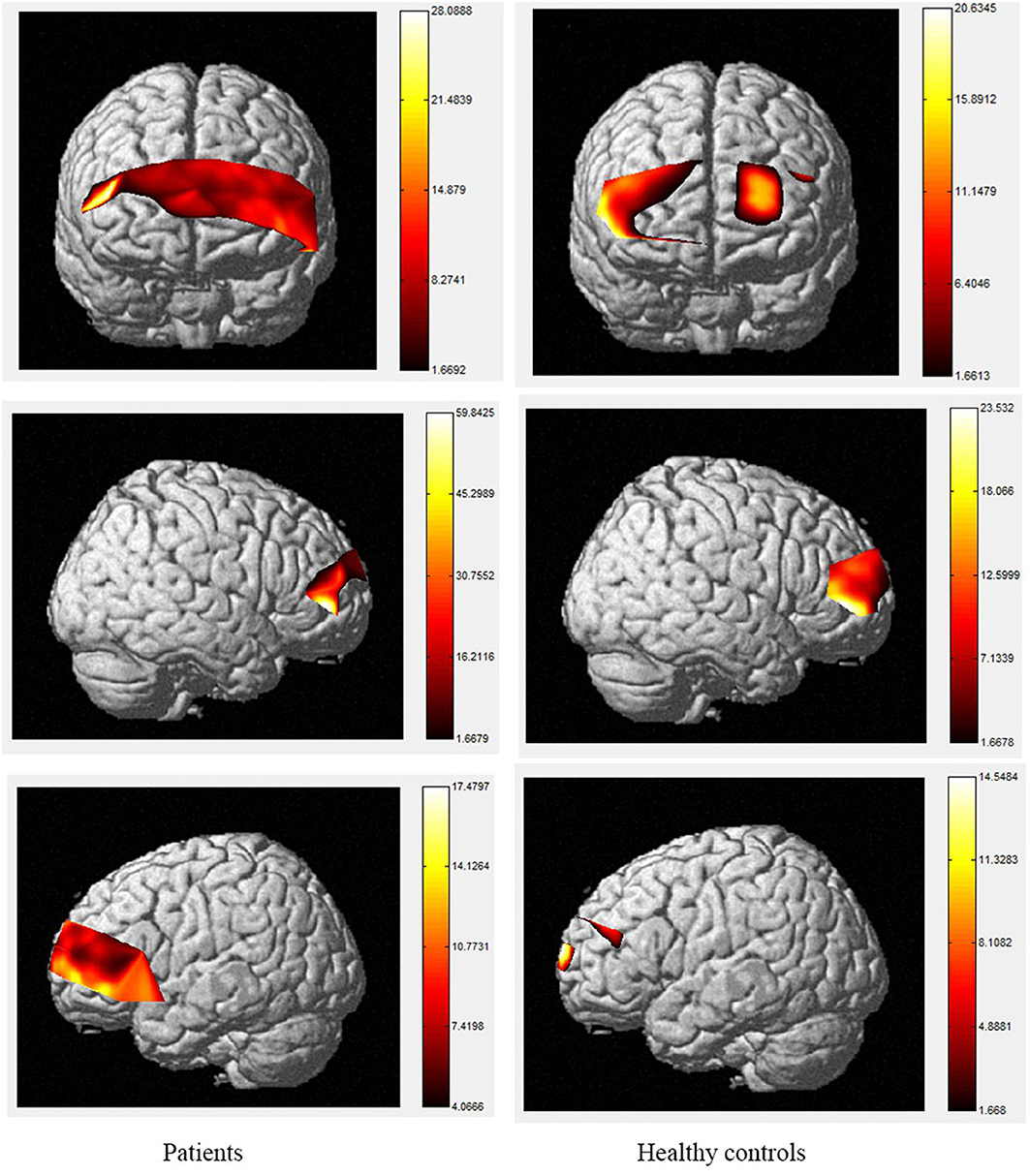
Figure 5. The activation of brain areas during 2-back tasks between patients and healthy controls. The color bars in right sides mean the activated strength of brain areas.
Discussion
Deficits in memory, selective attention, and response inhibition are common after TBI and fMRI studies showed that inefficient pre-frontal lobe function were associated with these deficits (30–32). To address the poor temporal resolution and limitations of fMRI (33), Wijeakumar et al. (34) observed a significant voxel-wise correlation between fNIRS and fMRI measurements, which were applicable to all experimental conditions in the frontal temporal cortex of the brain. The fNIRS is a good technique for studying cognitive function, but the simultaneous evaluation of memory and attention using fNIRS in patients has not previously been performed. This study aimed to address this gap in the literature by assessing changes in cerebral oxygenation, especially HbO, and cognitive accuracy during N-back and Stroop tasks in patients with neurocognitive disorders after TBI using fNIRS.
The fNIRS is a non-invasive monitoring modality, which might be helpful in detecting brain's condition during the acute phase of TBI. Therefore, numerous studies focused on the NIRS-based data and their association with patient functional outcome and a high proportion of studies concluded that fNIRS could be a potential non-invasive technique for assessing TBI (35, 36). However, most studies paid more attention to fNIRS as an continuous monitoring during acute phase of TBI and few studies focused on residual psychiatric symptoms which existed more than 6 months after TBI. The executive dysfunction is a prominent and persistent problem for patients with neurocognitive disorders after TBI, but few fNIRS studies on working memory and sustained attention have been conducted when patients discharged from hospitals for more than 6 months. Meanwhile, fNIRS analysis has not been used to examine changes in the frontal lobes and, especially, frontopolar areas of patients during EF-related N-back and Stroop tasks. Indeed, a study on patients with MCI observed that the combination of Stroop and N-back tasks provided superior resolution for identifying MCI in an elderly group. An initial objective of the study was to identify the differences in memory and sustained attention between patients with persistent symptoms, for whom memory problems were most prominent, and controls using fNIRS (37). The inability to inhibit pre-potent responses and selectively focus on salient information can be measured using the Stroop task, while the N-back task a useful tool for assessing memory. Ultimately, the results revealed significant differences between patients and controls for both behavioral and cerebral oxygenation data during these tasks.
The Stroop and N-back tasks can be modified into two conditions, including simple and more complex, and the subjects are instructed to respond as quickly as possible. These tasks allow us to determine the neural responses to both a simple task and to a more complex task that requires memory, selective attention, and response inhibition. Behaviorally, both the patients and healthy controls exhibited the Stroop effect in this study. Although the 2-back task was complex, and the healthy controls required longer processing time, the number of errors they made was no different from the 1-back task. However, in the patient group, reaction times were longer and the number of errors significantly increased in the 2-back task. A possible explanation for this difference may be that the complexity of the task did not affect the process of storing and processing information in healthy controls. Regardless of the task load, the corresponding neural areas remained effective. Conversely, in the same task, the reaction time of patients was significantly longer and the number of errors was significantly higher, indicating memory and attention impairments in the patient group, which are consistent with previous findings regarding performance in complex executive tasks (38).
Moreover, the patients were limited in complex tasks, and the differences in activated areas increased accordingly. During the 1-back task, there was greater left-sided than right-sided activation in the rostral pre-frontal cortex (rPFC) among controls, and the activation area of the patients was also mainly the rPFC on the left side. Currently, it is believed that working memory includes at least two parts, which are responsible for processing words and spatial information, respectively. Word working memory mainly activates the left hemisphere (dominant hemisphere), whereas spatial working memory mainly activates the right hemisphere. The N-back task is one of the classical models of working memory, and its related brain activation is in line with the neural circuits of working memory, this is relatively consistent with the activation area of word working memory (39, 40) and supports the theory that numbers are also processed as a kind of word information in the left frontal hemisphere. Under the 2-back condition, the activation area of the controls was in the left rPFC and the left dorsolateral pre-frontal cortex (DLPFC). However, the activation area of the patients covered most of the left and right rPFC, in a scattered manner, and the right-side region of the ventrolateral pre-frontal cortex (VLPFC) was also activated. Therefore, the present study demonstrates the involvement of the DLPFC, VLPFC, and rPFC during the 2-back task in TBI patients.
The increased activity during the 2-back task relative to the 1-back task observed in this study is similar to fMRI findings in controls, as tasks with higher memory demands are associated with increased PFC activity (40–47). The spatial locations activated by the 2-back task in this study are also consistent with the areas identified using fMRI, including the bilateral PFC (48, 49). However, our results contradict the studies that reported the anterior cingulate cortex (ACC) was activated during the 2-back task in patients with TBI using fMRI (50–54). A possible explanation for this may be differences in methodology, as fNIRS data cannot be received from subcortical regions such as the ACC, and the number of channels is restricted. In terms of the activation of localized brain regions based on channel differences, both groups exhibited activation in BA46/BA10, including the rPFC, VLPFC, and DLPFC. In contrast, significant differences between the two groups were observed during the 2-back task. Specifically, during this task, the patients exhibited decreased activity compared to controls in channels 2, 3, 9, 10, 11, 14, 18, 19, 21, and 22 which were located in the bilateral DLPFC and rPFC. Previous researches has found that the VLPFC, especially BA44 and BA45, plays an important role in silent reading and retelling, meaning that this area, as well as the parietal cortex, belongs to the phonetic ring and participate in the smooth maintenance of working memory (55, 56). Activation of the bilateral VLPFC was observed during the digital 2-back task in controls, which may reflect neuronal responses generated by extracting stored information and comparing it with current information or may be related to silent reading and retelling. This area was strongly activated in the control group, and we hypothesize that the group had a higher tolerance for silent reading and recitation, allowing them to perform the task better than patients. The differences in these areas suggest that the strategies used by the two groups to complete the task differed significantly.
The color word Stroop test is commonly used to assess selective attention in TBI. The latency difference between the two tasks is referred to as Stroop Interference (SI). In accordance with the present results, previous studies have demonstrated that larger SIs are observed in TBI patients than in healthy controls. The TBI-related increase in SI is typically considered to reflect the decrease in selective-attention after TBI. The current study observed that greater difficulty in both the consistent and inconsistent conditions in TBI patients. These outcomes are contrary to those of Plenger et al. (13), who noted that greater difficulty in color naming after TBI was not reflected in their behavioral data. These differences could have arisen due to the contexts of the paradigm. Indeed, studies using the Stroop paradigm in China have all reported SI in the behavioral data of healthy controls. Furthermore, a recent meta-analysis proposed that TBI-related changes in sensory processing, specifically color vision, could at least partly explain this increase in SI after TBI (57). They proposed that increased difficulty in color vision processing after TBI could be the source of inflated SI, beyond any changes in selective attention. There are several possible explanations for differences in SI results, such as using error rates, lacking mandatory control time, changing the stimuli only after the subject pressed the key, and recording every key response, which may make the task not sensitive enough to gauge small-scale differences in baseline color-naming. During the consistent color condition, the control group activated a large area of the right rPFC, as well as a small area of left rPFC, whereas, among the TBI group, the consistent condition elicited a greater spatial extent of significant activation bilaterally. During the inconsistent task, activation was observed in the same regions in the control and patient groups with the patient group showing a small amount of additional activation in part of the left rPFC, VLPFC and DLPFC. The spatial locations activated by the inconsistent condition in this study are consistent with the regions involved during the Stroop inconsistent task using fMRI, as previous literature has demonstrated significant bilateral rPFC involvement in that task (58, 59). Increased activity in the left hemisphere was expected given the verbalizations required during this task (60). More specifically, activation in the VLPFC was expected given its role in naming and word retrieval, which has been repeatedly demonstrated in various neuroimaging studies (61–65).
Increased activity during the inconsistent condition of the Stroop task compared to the consistent condition in this study is in line with the results of fMRI studies with normal controls, as tasks requiring increased attentional demand are associated with increased pre-frontal cortex activity. Comparisons of the cerebral oxygenation patterns of patients and controls revealed Stroop effects in certain regions of interest (ROIs; BA10, BA46, rPFC, and left DLPFC) for HbO. The accuracy rates of TBI patients were lower than those of controls, and both groups responded more accurately to the consistent condition than the inconsistent condition. These effects support our difficulty manipulation, as the participants made more errors and took longer reflection times in the more difficult inconsistent condition. In addition, the increased difficulty resulted in more response errors and longer response times for the TBIs than for the controls. Moreover, the patient group activated a greater spatial extent, bilaterally extending from the anterior frontal lobes to the left VLPFC regions. Activations in dorsal regions during the simple visual attention task support attention and orientation toward visual stimuli (66–68). Furthermore, between-group analysis of the extent of activity changes from baseline in certain channels (4, 8, 9, 11, 14, 15, and 22, located in BA9, BA21, BA10, and BA45) during consistent condition demonstrates that the patient group had significantly lower HbO responses in bilateral frontal regions during this condition compared controls. These results suggest that patients with TBI generally activate the anterior frontal lobes less efficiently than controls, but the activation was more extensive in the VLPFC, as reported by Plenger et al. (13), who found a similar phenomenon during a dot color-naming task. It is possible that less efficient activity represents damage to an inhibitory area of the brain in the patient group, and more extensive activation reflects neural compensation in these individuals, as a greater degree of processing may be required to accomplish the same task. The fNIRS data supports this sensory theory (65, 69–73). Indeed, in the inconsistent condition, the patients activated the same regions as those active by controls. For controls, there were additional loci of brain activity when performing the color word inconsistent task compared to the color word consistent task; specifically, additional activation was observed in the bilateral PFC.
Limitations
This study has several limitations. Firstly, the subjects may have demonstrated uncooperative behavior during task execution, thus making examination challenging. Secondly, the sample size was relatively small, which may have affected the statistical power. Thirdly, the study focused on fNIRS as a potential objective evaluation technique in neurocognitive disorders after TBI, and did not investigate the underlying mechanisms. Therefore, larger sample sizes and more mechanism research will be carried out in the.
Conclusion
In conclusion, based on fNIRS technology, the results of this study find that patients with neurocognitive disorders after TBI show decreased activation strength and increased activation range in brain activation maps during Stroop and n-back tasks, which means these patients may suffer executive dysfunction. Therefore, the fNIRS could be a potential objective evaluation technique in neurocognitive disorders after TBI.
Data availability statement
The datasets generated for this study are available on request to the corresponding author.
Ethics statement
The studies involving human participants were reviewed and approved by Academy of Forensic Science. The patients/participants provided their written informed consent to participate in this study. Written informed consent was obtained from the individual(s) for the publication of any potentially identifiable images or data included in this article.
Author contributions
FC and HL participated in the design of the study and drafted the manuscript. FC, HL, NL, SZ, and CL enrolled patients and healthy volunteers, performed the experiments, and performed statistical analyses. QZ participated in the design of the study and helped with proofreading. WC and HL conceived the study protocol and participated in the design and co-ordination of the study. All authors contributed to the final text and approved it.
Funding
This study was supported by the National Natural Science Foundation of China (81801881), Science and Technology Committee of Shanghai Municipality (20DZ1200300, 21DZ2270800, and 19DZ2292700).
Acknowledgments
We would like to acknowledge all participants from the Academy of Forensic Science, Sichuan Provincial People's Hospital and Shanghai Hongkou Mental Health Center, who provided help in this study.
Conflict of interest
The authors declare that the research was conducted in the absence of any commercial or financial relationships that could be construed as a potential conflict of interest.
Publisher's note
All claims expressed in this article are solely those of the authors and do not necessarily represent those of their affiliated organizations, or those of the publisher, the editors and the reviewers. Any product that may be evaluated in this article, or claim that may be made by its manufacturer, is not guaranteed or endorsed by the publisher.
References
1. Benoit RG, Gilbert SJ, Frith CD, Burgess PW. Rostral pre-frontal cortex and the focus of attention in prospective memory. Cerebral Cortex. (2012) 22:1876–86. doi: 10.1093/cercor/bhr264
2. Davranche K, Casini L, Arnal PJ, Rupp T, Perrey S, Verges S. Cognitive functions and cerebral oxygenation changes during acute and prolonged hypoxic exposure. Physiol Behav. (2016) 164:189–97. doi: 10.1016/j.physbeh.2016.06.001
3. Ehlis AC, Haeussinger FB, Gastel A, Fallgatter AJ, Plewnia C. Task-dependent and polarity-specific effects of pre-frontal transcranial direct current stimulation on cortical activation during word fluency. Neuroimage. (2016) 140:134–40. doi: 10.1016/j.neuroimage.2015.12.047
4. Guhn A, Domschke K, Muller LD, Dresler T, Eff F, Kopf J, et al. Neuropeptide S receptor gene variation and neural correlates of cognitive emotion regulation. Soc Cogn Affect Neurosci. (2015) 10:1730–7. doi: 10.1093/scan/nsv061
5. Koike S, Takizawa R, Nishimura Y, Takano Y, Takayanagi Y, Kinou M, et al. Different hemodynamic response patterns in the pre-frontal cortical sub-regions according to the clinical stages of psychosis. Schizophr Res. (2011) 132:54–61. doi: 10.1016/j.schres.2011.07.014
6. Kroczek AM, Haussinger FB, Rohe T, Schneider S, Plewnia C, Batra A, et al. Effects of transcranial direct current stimulation on craving, heart-rate variability and pre-frontal hemodynamics during smoking cue exposure. Drug Alcohol Depend. (2016) 168:123–7. doi: 10.1016/j.drugalcdep.2016.09.006
7. Wu Z, Mazzola CA, Catania L, Owoeye O, Yaramothu C, Alvarez T, et al. Altered cortical activation and connectivity patterns for visual attention processing in young adults post-traumatic brain injury: a functional near infrared spectroscopy study. CNS Neurosci Ther. (2018) 24:539–48. doi: 10.1111/cns.12811
8. Dean PJ, Sterr A. Long-term effects of mild traumatic brain injury on cognitive performance. Front Hum Neurosci. (2013) 7:30. doi: 10.3389/fnhum.2013.00030
9. Arciniegas DB, Frey KL, Newman J, Wortzel HS. Evaluation and management of post-traumatic cognitive impairments. Psychiatr Ann. (2010) 40:540–52. doi: 10.3928/00485713-20101022-05
10. Zysk M, Clausen F, Aguilar X, Sehlin D, Syvanen S, Erlandsson A. Long-term effects of traumatic brain injury in a mouse model of Alzheimer's disease. J Alzheimers Dis. (2019) 72:161–80. doi: 10.3233/JAD-190572
11. Ehlis AC, Schneider S, Dresler T, Fallgatter AJ. Application of functional near-infrared spectroscopy in psychiatry. NeuroImage. (2014) 85 Pt 1:478–88. doi: 10.1016/j.neuroimage.2013.03.067
12. Lianyang L, Arakaki X, Thao T, Harrington M, Padhye N, Zouridakis G. Brain activation profiles in mTBI: evidence from ERP activity of working memory response. Conf Proc IEEE Eng Med Biol Soc. (2016) 2016:1862–5. doi: 10.1109/EMBC.2016.7591083
13. Plenger P, Krishnan K, Cloud M, Bosworth C, Qualls D, Marquez de la Plata C. fNIRS-based investigation of the Stroop task after TBI. Brain Imaging Behav. (2016) 10:357–66. doi: 10.1007/s11682-015-9401-9
14. Nishizawa Y, Kanazawa T, Kawabata Y, Matsubara T, Maruyama S, Kawano M, et al. fNIRS assessment during an emotional Stroop task among patients with depression: replication and extension. Psychiatry Investig. (2019) 16:80–6. doi: 10.30773/pi.2018.11.12.2
15. Tak S, Uga M, Flandin G, Dan I, Penny WD. Sensor space group analysis for fNIRS data. J Neurosci Methods. (2016) 264:103–12. doi: 10.1016/j.jneumeth.2016.03.003
16. Chernomordik V, Amyot F, Kenney K, Wassermann E, Diaz-Arrastia R, Gandjbakhche A. Abnormality of low frequency cerebral hemodynamics oscillations in TBI population. Brain Res. (2016) 1639:194–9. doi: 10.1016/j.brainres.2016.02.018
17. Hibino S, Mase M, Shirataki T, Nagano Y, Fukagawa K, Abe A, et al. Oxyhemoglobin changes during cognitive rehabilitation after traumatic brain injury using near infrared spectroscopy. Neurol Med Chir. (2013) 53:299–303. doi: 10.2176/nmc.53.299
18. Khetani A, Rohr CS, Sojoudi A, Bray S, Barlow KM. Alteration in cerebral activation during a working memory task after pediatric mild traumatic brain injury: a prospective controlled cohort study. J Neurotrauma. (2019). doi: 10.1089/neu.2018.6117
19. Westfall DR, West JD, Bailey JN, Arnold TW, Kersey PA, Saykin AJ, et al. Increased brain activation during working memory processing after pediatric mild traumatic brain injury (mTBI). J Pediatr Rehabil Med. (2015) 8:297–308. doi: 10.3233/PRM-150348
20. Chen JK, Johnston KM, Frey S, Petrides M, Worsley K, Ptito A. Functional abnormalities in symptomatic concussed athletes: an fMRI study. Neuroimage. (2004) 22:68–82. doi: 10.1016/j.neuroimage.2003.12.032
21. Chen DY, Hsu HL, Kuo YS, Wu CW, Chiu WT, Yan FX, et al. Effect of age on working memory performance and cerebral activation after mild traumatic brain injury: a functional MR imaging study. Radiology. (2016) 278:854–62. doi: 10.1148/radiol.2015150612
22. Palacios EM, Fernandez-Espejo D, Junque C, Sanchez-Carrion R, Roig T, Tormos JM, et al. Diffusion tensor imaging differences relate to memory deficits in diffuse traumatic brain injury. BMC Neurol. (2011) 11:24. doi: 10.1186/1471-2377-11-24
23. Slovarp L, Azuma T, Lapointe L. The effect of traumatic brain injury on sustained attention and working memory. Brain Inj. (2012) 26:48–57. doi: 10.3109/02699052.2011.635355
24. Yang D, Hong KS, Yoo SH, Kim CS. Evaluation of neural degeneration biomarkers in the pre-frontal cortex for early identification of patients with mild cognitive impairment: an fNIRS study. Front Hum Neurosci. (2019) 13:317. doi: 10.3389/fnhum.2019.00317
25. Logan DM, Hill KR, Larson MJ. Cognitive control of conscious error awareness: error awareness and error positivity (Pe) amplitude in moderate-to-severe traumatic brain injury (TBI). Front Hum Neurosci. (2015) 9:397. doi: 10.3389/fnhum.2015.00397
26. Owens JA, Spitz G, Ponsford JL, Dymowski AR, Willmott C. An investigation of white matter integrity and attention deficits following traumatic brain injury. Brain injury. (2018) 32:776–83. doi: 10.1080/02699052.2018.1451656
27. Chen Y, Spagna A, Wu T, Kim TH, Wu Q, Chen C, et al. Testing a cognitive control model of human intelligence. Sci Rep. (2019) 9:2898. doi: 10.1038/s41598-019-39685-2
28. Tsuzuki D, Jurcak V, Singh AK, Okamoto M, Watanabe E, Dan I. Virtual spatial registration of stand-alone fNIRS data to MNI space. Neuroimage. (2007) 34:1506–18. doi: 10.1016/j.neuroimage.2006.10.043
29. Zhang Y, Li Z. Review of noise sources and denoising methods based on functional near-infrared spectroscopy for brain imaging. Infrared. (2019) 40:35–46. doi: 10.3969/j.issn.1672-8785.2019.07.005
30. Huppert T, Barker J, Schmidt B, Walls S, Ghuman A. Comparison of group-level, source localized activity for simultaneous functional near-infrared spectroscopy-magnetoencephalography and simultaneous fNIRS-fMRI during parametric median nerve stimulation. Neurophotonics. (2017) 4:015001. doi: 10.1117/1.NPh.4.1.015001
31. Kenney K, Amyot F, Haber M, Pronger A, Bogoslovsky T, Moore C, et al. Cerebral vascular injury in traumatic brain injury. Exp Neurol. (2016) 275 Pt 3:353–66. doi: 10.1016/j.expneurol.2015.05.019
32. Yuan Z, Ye J. Fusion of fNIRS and fMRI data: identifying when and where hemodynamic signals are changing in human brains. Front Hum Neurosci. (2013) 7:676. doi: 10.3389/fnhum.2013.00676
33. Steinbrink J, Villringer A, Kempf F, Haux D, Boden S, Obrig H. Illuminating the BOLD signal: combined fMRI-fNIRS studies. Magn Reson Imaging. (2006) 24:495–505. doi: 10.1016/j.mri.2005.12.034
34. Wijeakumar S, Huppert TJ, Magnotta VA, Buss AT, Spencer JP. Validating an image-based fNIRS approach with fMRI and a working memory task. Neuroimage. (2017) 147:204–18. doi: 10.1016/j.neuroimage.2016.12.007
35. Mathieu F, Khellaf A, Ku JC, Donnelly J, Thelin EP, Zeiler FA. Continuous near-infrared spectroscopy monitoring in adult traumatic brain injury: a systematic review. J Neurosurg Anesthesiol. (2020) 32:288–99. doi: 10.1097/ANA.0000000000000620
36. Roldán M, Kyriacou PA. Near-infrared spectroscopy (NIRS) in traumatic brain injury (TBI). Sensors. (2021) 21:1586. doi: 10.3390/s21051586
37. Mihara M, Yagura H, Hatakenaka M, Hattori N, Miyai I. Clinical application of functional near-infrared spectroscopy in rehabilitation medicine. Brain Nerve. (2010) 62:125–32. doi: 10.11477/mf.1416100628
38. Bediz CS, Oniz A, Guducu C, Ural Demirci E, Ogut H, Gunay E, et al. Acute supramaximal exercise increases the brain oxygenation in relation to cognitive workload. Front Hum Neurosci. (2016) 10:174. doi: 10.3389/fnhum.2016.00174
39. Lilienthal L, Tamez E, Shelton JT, Myerson J, Hale S. Dual n-back training increases the capacity of the focus of attention. Psychon Bull Rev. (2013) 20:135–41. doi: 10.3758/s13423-012-0335-6
40. Leon-Dominguez U, Martin-Rodriguez JF, Leon-Carrion J. Executive n-back tasks for the neuropsychological assessment of working memory. Behav Brain Res. (2015) 292:167–73. doi: 10.1016/j.bbr.2015.06.002
41. Kopf J, Dresler T, Reicherts P, Herrmann MJ, Reif A. The effect of emotional content on brain activation and the late positive potential in a word n-back task. PLoS ONE. (2013) 8:e75598. doi: 10.1371/journal.pone.0075598
42. Vermeij A, van Beek AH, Olde Rikkert MG, Claassen JA, Kessels RP. Effects of aging on cerebral oxygenation during working-memory performance: a functional near-infrared spectroscopy study. PLoS ONE. (2012) 7:e46210. doi: 10.1371/journal.pone.0046210
43. Vermeij A, van Beek AH, Reijs BL, Claassen JA, Kessels RP. An exploratory study of the effects of spatial working-memory load on pre-frontal activation in low- and high-performing elderly. Front Aging Neurosci. (2014) 6:303. doi: 10.3389/fnagi.2014.00303
44. Aghajani H, Garbey M, Omurtag A. Measuring mental workload with EEG+fNIRS. Front Hum Neurosci. (2017) 11:359. doi: 10.3389/fnhum.2017.00359
45. Herff C, Heger D, Fortmann O, Hennrich J, Putze F, Schultz T. Mental workload during n-back task-quantified in the pre-frontal cortex using fNIRS. Front Hum Neurosci. (2013) 7:935. doi: 10.3389/fnhum.2013.00935
46. Kuruvilla MS, Green JR, Ayaz H, Murman DL. Neural correlates of cognitive decline in ALS: an fNIRS study of the pre-frontal cortex. Cogn Neurosci. (2013) 4:115–21. doi: 10.1080/17588928.2013.797889
47. Stojanovic-Radic J, Wylie G, Voelbel G, Chiaravalloti N, DeLuca J. Neuroimaging and cognition using functional near infrared spectroscopy (fNIRS) in multiple sclerosis. Brain Imaging Behav. (2015) 9:302–11. doi: 10.1007/s11682-014-9307-y
48. Fishburn FA, Norr ME, Medvedev AV, Vaidya CJ. Sensitivity of fNIRS to cognitive state and load. Front Hum Neurosci. (2014) 8:76. doi: 10.3389/fnhum.2014.00076
49. Choe J, Coffman BA, Bergstedt DT, Ziegler MD, Phillips ME. Transcranial direct current stimulation modulates neuronal activity and learning in pilot training. Front Hum Neurosci. (2016) 10:34. doi: 10.3389/fnhum.2016.00034
50. Huang MX, Nichols S, Robb-Swan A, Angeles-Quinto A, Harrington DL, Drake A, et al. MEG Working memory N-back task reveals functional deficits in combat-related mild traumatic brain injury. Cereb Cortex. (2019) 29:1953–68. doi: 10.1093/cercor/bhy075
51. Redick TS, Shipstead Z, Harrison TL, Hicks KL, Fried DE, Hambrick DZ, et al. No evidence of intelligence improvement after working memory training: a randomized, placebo-controlled study. J Exp Psychol Gen. (2013) 142:359–79. doi: 10.1037/a0029082
52. Le AS, Aoki H, Murase F, Ishida K. A novel method for classifying driver mental workload under naturalistic conditions with information from near-infrared spectroscopy. Front Hum Neurosci. (2018) 12:431. doi: 10.3389/fnhum.2018.00431
53. van der Horn HJ, Liemburg EJ, Scheenen ME, de Koning ME, Spikman JM, van der Naalt J. Post-concussive complaints after mild traumatic brain injury associated with altered brain networks during working memory performance. Brain Imaging Behav. (2016) 10:1243–53. doi: 10.1007/s11682-015-9489-y
54. Hocke LM, Duszynski CC, Debert CT, Dleikan D, Dunn JF. Reduced functional connectivity in adults with persistent post-concussion symptoms: a functional near-infrared spectroscopy study. J Neurotrauma. (2018) 35:1224–32. doi: 10.1089/neu.2017.5365
55. Araki A, Ikegami M, Okayama A, Matsumoto N, Takahashi S, Azuma H, et al. Improved pre-frontal activity in AD/HD children treated with atomoxetine: a NIRS study. Brain Dev. (2015) 37:76–87. doi: 10.1016/j.braindev.2014.03.011
56. Huhn AS, Meyer RE, Harris JD, Ayaz H, Deneke E, Stankoski DM, et al. Evidence of anhedonia and differential reward processing in pre-frontal cortex among post-withdrawal patients with prescription opiate dependence. Brain Res Bull. (2016) 123:102–9. doi: 10.1016/j.brainresbull.2015.12.004
57. Ben-David BM, van Lieshout PH, Shakuf V. Sensory source for Stroop effects in persons after TBI: support from fNIRS-based investigation. Brain Imaging Behav. (2016) 10:1135–6. doi: 10.1007/s11682-015-9477-2
58. Jia-cheng L, Xing C, Jiong X. The defects of executive function in attentional deficit and hyperaction disorder: a study of Stroop effects by fMRI. China Clin Med Imaging. (2018) 29:556–9. doi: 10.12117/jccmi.2018.08.007
59. Tlustos SJ, Chiu CY, Walz NC, Holland SK, Bernard L, Wade SL. Neural correlates of interference control in adolescents with traumatic brain injury: functional magnetic resonance imaging study of the counting Stroop task. J Int Neuropsychol Soc. (2011) 17:181–9. doi: 10.1017/S1355617710001414
60. Kopitzki K, Oldag A, Sweeney-Reed CM, Machts J, Veit M, Kaufmann J, et al. Interhemispheric connectivity in amyotrophic lateral sclerosis: a near-infrared spectroscopy and diffusion tensor imaging study. Neuroimage Clin. (2016) 12:666–72. doi: 10.1016/j.nicl.2016.09.020
61. Sato H, Obata AN, Moda I, Ozaki K, Yasuhara T, Yamamoto Y, et al. Application of near-infrared spectroscopy to measurement of hemodynamic signals accompanying stimulated saliva secretion. J Biomed Opt. (2011) 16:047002. doi: 10.1117/1.3565048
62. Romero K, Lobaugh NJ, Black SE, Ehrlich L, Feinstein A. Old wine in new bottles: validating the clinical utility of SPECT in predicting cognitive performance in mild traumatic brain injury. Psychiatry Res. (2015) 231:15–24. doi: 10.1016/j.pscychresns.2014.11.003
63. Rousseaux M, Verigneaux C, Kozlowski O. An analysis of communication in conversation after severe traumatic brain injury. Eur J Neurol. (2010) 17:922–9. doi: 10.1111/j.1468-1331.2009.02945.x
64. Suchy Y, Leahy B, Sweet JJ, Lam CS. Behavioral dyscontrol scale deficits among traumatic brain injury patients, part II: comparison to other measures of executive functioning. Clin Neuropsychol. (2003) 17:492–506. doi: 10.1076/clin.17.4.492.27949
65. Soeda A, Nakashima T, Okumura A, Kuwata K, Shinoda J, Iwama T. Cognitive impairment after traumatic brain injury: a functional magnetic resonance imaging study using the Stroop task. Neuroradiology. (2005) 47:501–6. doi: 10.1007/s00234-005-1372-x
66. Sliwinska MW, James A, Devlin JT. Inferior parietal lobule contributions to visual word recognition. J Cogn Neurosci. (2015) 27:593–604. doi: 10.1162/jocn_a_00721
67. Cabeza R, Nyberg L. Neural bases of learning and memory: functional neuroimaging evidence. Curr Opin Neurol. (2000) 13:415–21. doi: 10.1097/00019052-200008000-00008
68. Devlin JT, Raley J, Tunbridge E, Lanary K, Floyer-Lea A, Narain C, et al. Functional asymmetry for auditory processing in human primary auditory cortex. J Neurosci. (2003) 23:11516–22. doi: 10.1523/JNEUROSCI.23-37-11516.2003
69. Sozda CN, Larson MJ, Kaufman DA, Schmalfuss IM, Perlstein WM. Error-related processing following severe traumatic brain injury: an event-related functional magnetic resonance imaging (fMRI) study. Int J Psychophysiol. (2011) 82:97–106. doi: 10.1016/j.ijpsycho.2011.06.019
70. Hendry K, Ownsworth T, Beadle E, Chevignard MP, Fleming J, Griffin J, et al. Cognitive deficits underlying error behavior on a naturalistic task after severe traumatic brain injury. Front Behav Neurosci. (2016) 10:190. doi: 10.3389/fnbeh.2016.00190
71. Crowe SF, Crowe LM. Does the presence of post-traumatic anosmia mean that you will be disinhibited? J Clin Exp Neuropsychol. (2013) 35:298–308. doi: 10.1080/13803395.2013.771616
72. Adjorlolo S. Ecological validity of executive function tests in moderate traumatic brain injury in Ghana. Clin Neuropsychol. (2016) 30:1517–37. doi: 10.1080/13854046.2016.1172667
Keywords: neurocognitive disorders, traumatic brain injury, executive function, Stroop task, n-back task, fNIRS (functional near infrared spectroscopy)
Citation: Chang F, Li H, Li N, Zhang S, Liu C, Zhang Q and Cai W (2022) Functional near-infrared spectroscopy as a potential objective evaluation technique in neurocognitive disorders after traumatic brain injury. Front. Psychiatry 13:903756. doi: 10.3389/fpsyt.2022.903756
Received: 24 March 2022; Accepted: 27 June 2022;
Published: 22 July 2022.
Edited by:
Murat Ilhan Atagün, Çanakkale Onsekiz Mart University, TurkeyReviewed by:
Dalin Yang, Pusan National University, South KoreaBerker Duman, Ankara University, Turkey
Copyright © 2022 Chang, Li, Li, Zhang, Liu, Zhang and Cai. This is an open-access article distributed under the terms of the Creative Commons Attribution License (CC BY). The use, distribution or reproduction in other forums is permitted, provided the original author(s) and the copyright owner(s) are credited and that the original publication in this journal is cited, in accordance with accepted academic practice. No use, distribution or reproduction is permitted which does not comply with these terms.
*Correspondence: Weixiong Cai, dHNhaXNlQDE2My5jb20=; Haozhe Li, bGloYW96aGVfa2lyYUBmb3htYWlsLmNvbQ==
†These authors have contributed equally to this work