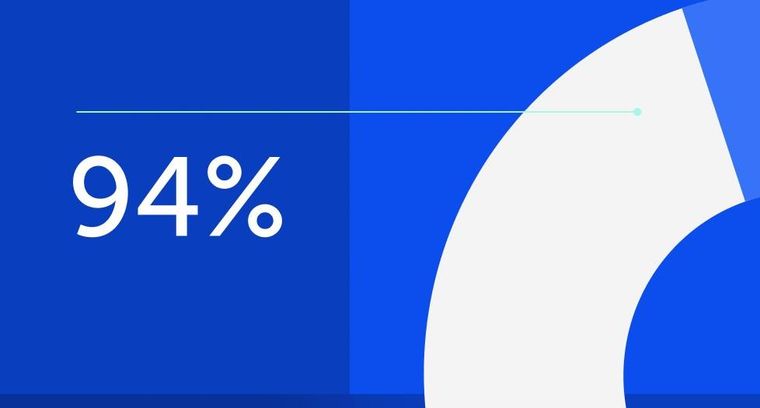
94% of researchers rate our articles as excellent or good
Learn more about the work of our research integrity team to safeguard the quality of each article we publish.
Find out more
REVIEW article
Front. Psychiatry, 05 August 2022
Sec. Public Mental Health
Volume 13 - 2022 | https://doi.org/10.3389/fpsyt.2022.899344
This article is part of the Research TopicMental Health of Disadvantaged ChildrenView all 16 articles
The negative influence on the cognitive ability of schizophrenia is one of the issues widely discussed in recent years. Working memory deficits are thought to be a core cognitive symptom of schizophrenia and lead to poorer social functions and worse academic performance. Previous studies have confirmed that working memory deficits tend to appear in the prodromal phase of schizophrenia. Therefore, considering that children with schizophrenia have better brain plasticity, it is critical to explore the development of their working memory. Although the research in this field developed gradually in recent years, few researchers have summarized these findings. The current study aims to review the recent studies from both behavior and neuroimaging aspects to summarize the working memory deficits of children with schizophrenia and to discuss the pathogenic factors such as genetic susceptibility. In addition, this study put forward some practicable interventions to improve cognitive symptoms of schizophrenia from psychological and neural perspectives.
Schizophrenia is now one of the most prevalent and serious mental illnesses in the world with typical symptoms defined (1, 2): (a) positive symptoms centered on delusions, hallucinations, disorganized speech, and grossly disorganized or catatonic behavior; (b) negative symptoms, i.e., affective flattening, alogia, or avolition; (c) cognitive symptoms including deficits in attention, working memory, and executive functions. Multiple psychiatrists proposed that different aspects of symptoms are not separate. For example, negative symptoms (loss of function), by definition, incorporate cognitive symptoms. However, more complex cognitive impairments have been found over the past years and viewed as one kind of independent symptom by multiple psychiatrists. As a result, we should treat schizophrenia symptoms from a unified perspective (3, 4). In addition, all these symptoms might be linked to the functions of dopamine, serotonin, and glutamatergic neurotransmission systems (5–7).
As shown in surveys, the lifetime prevalence of schizophrenia is estimated to be 0.3% worldwide and 0.83% in China (8, 9) and varies depending on the location, economic conditions, and the diagnostic criteria used. Although the annual cost of schizophrenia for both patients and society is tremendous (10), the therapeutic effect of schizophrenia is still not ideal. A previous investigation showed that only 13.5% of individuals with schizophrenia met the criteria for recovery (11). Furthermore, Phahladira and Luckhoff (12) have found that 70% of patients treated with antipsychotic medication achieved symptom remission, while only 9% achieved both functional remission and good quality of life, implying a large gap between symptom remission and a return to normal life. In a study by Nuechterlein, Subotnik (13), it was highlighted that cognitive functioning is one of the most critical determinants of quality of life for people with schizophrenia compared to symptoms such as delusions or hallucinations. More research provides sufficient support for this statement, for example, the important role of cognitive functioning in socialization has been demonstrated (14) and a longitudinal study has also shown that cognitive impairment predicts slower socialization (15). Therefore, cognitive functioning in patients with schizophrenia has become a key object of research.
People with schizophrenia have deficits in multiple cognitive domains simultaneously (1, 16), A meta-analysis has found that adult patients with schizophrenia perform more poorly than healthy controls in five cognitive domains including IQ, memory, language, executive function, and attention (17). Another systematic review has also indicated worse performance in a broad range of neuropsychological domains like multiple types of memory, intellectual function, sustained attention, as well as set-shifting and response inhibition (18). Specifically, working memory has been considered as one of the most fundamental cognitive functions and is related to a number of core symptoms of schizophrenia (19). Early studies demonstrated that there was a significant correlation between working memory deficits and several schizophrenia symptoms such as affective disorder and disorganized behavior (20, 21).
Working memory is defined as the limited capacity system necessary for short-term storage and manipulation of information in progress (22). In many studies investigating schizophrenia in all age groups, childhood and adolescence have been found to be particularly critical. Cognitive impairments such as working memory deficits tend to present in childhood (age 7–13) and adolescence (age 14–17) before they perform signs and symptoms of schizophrenia (23), so working memory deficits may be one of the predictors of schizophrenia in childhood. In addition, several empirical studies from schools have found that children in the early stages (the pre-psychotic prodromal period and the first 5–10 years after the initial episode) (24) of schizophrenia have poorer educational achievement and impaired social functioning, which may be due to working memory deficits (25–28). Remarkably, schizophrenia, one of the first-episode psychoses (FEP), often develops in childhood and adolescence (29), and patients with FEP tend to show a high remission rate owing to early intervention (30, 31), suggesting that the earlier the intervention was given, the more effective the intervention would be.
Therefore, it is meaningful to study schizophrenia in children from the perspective of cognitive impairments like working memory deficits, however, few reviews have been made. To conclude, the current study aims to summarize studies on working memory deficits in children with schizophrenia from both behavioral and neuroimaging aspects, try to explain deficits with possible susceptible genes and recommend some available interventions.
Childhood and adolescence represent a rapid physical and psychological development. However, it is also a period when neurodevelopmental disorders such as schizophrenia begin to appear, accompanied with cognitive deficits (e.g., working memory deficits), (32). Cognitive Neuroscience Treatment Research to Improve Cognition in Schizophrenia (CNTRICS) has suggested four components of working memory deficits including goal maintenance, interference control, rehearsal (active maintenance over time), and updating (33). Previous studies have found deficits in goal maintenance among schizophrenia patients with several working memory tasks such as the anti-saccade task (34) and Dot Pattern Expectancy task (35). Some other studies also indicate impaired updating function in schizophrenia with updating and maintenance paradigm (36) and auditory target detection task (37). Eye-movement studies have also provided some evidence for abnormal working memory inhibitory processes in ultra-high-risk subjects and siblings (38, 39). These findings indicate that schizophrenia affects the working memory function of patients from multiple dimensions and we suggest that different mechanisms should be distinguished among these effects. In addition, some early field studies demonstrated possible working memory deficits in children with schizophrenia. A meta-analysis also showed that children with schizophrenia had worse general academic and mathematics achievements (40), meanwhile, accompanied by poorer social functions characterized by inadequate emotional reactions, the disorder of thinking, and loss of will (41, 42), which could be considered main markers of working memory deficits. Then, a questionnaire study on a large sample of adolescents and young adults has shown that specific psychotic-like experiences are associated with low working memory capacity (43). Furthermore, previous behavioral studies have found that children with schizophrenia tend to present more slow response time and low accuracy in different types of working memory tasks including visual, auditory, and verbal tasks (44–47). To conclude, sufficient behavioral evidence has told us that various working memory deficits exist in (high-risk) schizophrenia subjects and could be markers of the disease progression.
Over the past few years, an increasing number of neuroscientists have turned to explore the neuromechanism of working memory deficits in patients with schizophrenia. Many functional magnetic resonance imaging (fMRI) studies have reported altered engagement of the cerebral cortex during working memory processing in early-onset (EOS; the age of onset <18 years) schizophrenia, particularly in the dorsolateral prefrontal cortex (DLPFC), ventrolateral prefrontal cortex (VLPFC), and anterior cingulate cortex (ACC) (48–50). These regions have been shown to be closely associated with a wide range of executive dysfunction, especially memory deficits (51). For example, a previous study (52) found the decreased DLPFC connectivity within the working memory network and suggested that patients with EOS schizophrenia showed significant late developmental changes. Nielsen, Madsen (53) found that first-episode schizophrenia had decreased forward connectivity from the left inferior parietal lobe (IPL) to the left inferior frontal gyrus (IFG), which indicated the impaired working memory modulation of the frontoparietal network and the failure of context-sensitive coupling in the early phase of schizophrenia. Moreover, the degree of impact on different working memory functions varies, which perhaps means discrepant neuromechanism. A previous study found the increased activation when patients engaged in the manipulation plus maintenance task. Meanwhile, the changes of brain activation were disproportionately less in the DLPFC and greater in the VLPFC. This result suggested that manipulating function is selectively more affected than storing function in working memory (54). In addition, some researchers have found abnormal hyperactivation in the above-mentioned brain regions, reflecting the use of compensatory cognitive strategy while solving WM tasks (55), which might be another sign of working memory deficits. Furthermore, Loeb et al. (48) also pointed out that decreased activation and functional connectivity in the working memory network in childhood-onset schizophrenia were associated with the severity of the disease. To conclude, although there is no consensus on the neuromechanism of schizophrenia, it's clear that no matter in function or structure, numerous studies have offered enough evidence that children with or at risk of schizophrenia develop with apparent or potential working memory deficits and these deficits tend to present relatively early during schizophrenia.
Previous studies provided sufficient evidence that schizophrenia is a highly heritable disease. An early twin study showed that the estimate of heritability in liability of schizophrenia was nearly 81% (56) and this result was still supported by some recent studies (57, 58). Similarly, working memory deficits in patients with psychosis are genetic with heritability estimates of up to 49% (59). Thus, numerous psychologists and psychiatrists have committed themselves to exploring possible susceptible genes linked with working memory deficits in patients with schizophrenia.
Previous genome-wide association studies (GWAS) have indicated some susceptibility genes are significantly associated with schizophrenia. The most compelling studies came from the psychiatric genomics Association (PGC), which provided the largest sample size for the GWAS study of schizophrenia. With the release of three PGC studies (60–62), researchers further clarified the genetic nature of schizophrenia. In 2014, the landmark GWAS study conducted by the PGC identified 128 independent single nucleotide polymorphisms (SNPs) in 108 gene loci that met genome-wide significance (61). Some associations at DRD2 and several genes involved in glutamatergic neurotransmission were consistent with previous leading pathophysiological hypotheses and highlight molecules with known and potential therapeutic relevance to schizophrenia. While 83 of 128 SNPs have not been reported previously, providing entirely new insights into etiology. In the recent GWAS study conducted by PGC, they identified 120 genes involved 106 protein-coding, of which more gene loci related to neurodevelopmental disorders were found (62). These GWAS studies have identified multiple susceptibility genes significantly related to schizophrenia. However, the specific mechanisms of these genes in schizophrenia are still unclear, which hinders the determination of promising drug molecular targets.
Some researchers chose to focus on the effects of schizophrenia susceptibility genes identified in the GWAS study on cognition and its brain mechanisms, many of which involved the working memory function. For example, the rs1344706 within the zinc finger protein 804A gene (ZNF804A) has been found a compelling candidate single nucleotide polymorphism (SNP) for schizophrenia (63). Recent studies have also provided support for altered brain activities among ZNF804A variant rs1344706 schizophrenia carriers, such as influenced hippocampal-DLPFC functional connectivity during resting-state (64), abnormal prefrontal connectivity (65), and altered cortical network dynamics (66). Some of them also play a significant role in working memory performance and a previous cognitive-measuring study has indicated higher polygenic scores of ZNF804A were associated with poorer working memory (67). Linden, Lancaster (68) has found worse working memory performance for face processing among ZNF804A carriers. Additionally, impaired cognitive control function in working memory has also been found (69, 70).
The dopamine receptor type 2 gene (DRD2) polymorphism was also known as one of the risk variants for schizophrenia (71). The polymorphism of DRD2 (C957T) has been known to be associated with schizophrenia (72), while the result seems inconsistent in different populations (73, 74). Meanwhile, the C957T variant has showed closely connected to working memory performance, especially in goal maintenance function (75) but limited effect on executive function (Working Memory, Response Inhibition, Cognitive Flexibility) (76). Moreover, researchers have also found that the rs1076560 variant of DRD2 significantly contributes to abnormal prefrontal activity and impaired working memory in several task-related studies (77, 78). Braun, Harneit (79) have studied the role of DRD2 dopamine in whole-brain network dynamics and found decreased stability of working memory representations. Pergola, Di Carlo (80) comprehensively studied the DRD2 co-expression network and calculated a related polygenic index which could significantly predict the abnormal prefrontal activity and insufficient working memory processing.
Several GWAS studies have also identified the CACNA1C gene polymorphism (rs1006737) as a susceptibility locus for schizophrenia (81) which often comes with alterations in prefrontal activation and fronto-hippocampal connectivity during emotional processing and executive cognition (82, 83) and finally lead to poor working memory performance (84, 85), especially impact on the encoding phase of working memory (86). Beyond that, some other SNPs in CACNA1C have been identified as risk loci for schizophrenia over the past years (87). However, we are still lacking in profound understanding among them and more evidence is expected.
Moreover, RELN variants have been proved to contribute to the endophenotypes of schizophrenia (88) and regulate synaptic plasticity and cognition in psychotic subjects (89). Reproducible studies have shown multiple RELN variants (7q21-32) are associated with memory impairments (90, 91). In addition, a study in healthy population carried with RELN (rs362691) has shown high cognitive deficits, especially in executive function (92). Although more and more SNPs in RELN are confirmed to be related to schizophrenia recently (93), their effect on working memory awaits deeper studies.
In addition, the transcription factor 4 gene (TCF4) (94, 95), the microRNA-137 gene (MIR-137) (96–98), and the type-3 metabotropic glutamate receptor gene (GRM3) have also been found to be associated with working memory impairment by affecting the functions of brain regions related to working memory processes, including DLPFC, hippocampus, striatum and other regions.
Beyond that, Nicodemus, Kolachana (99) proposed that statistical interactions among multiple susceptibility genes should also be considered. The polygenic risk score (PRS) has become a powerful indicator of GWAS results. Identified SNPs were weighted by their association odds ratio to construct a risk score for each individual. The PRS was positively correlated with the genetic susceptibility of the disease. Wang, Liu (100) calculated the PRS using the formula developed by PGC and found that a higher PRS was significantly associated with impairment in working memory. To sum up, a large number of GWAS studies have shown us that schizophrenia susceptible genes indeed account for working memory deficits. These findings will undoubtedly provide enlightenment for improved treatment for cognitive symptoms in schizophrenia patients in the future. In addition, many newly identified genes are related to neurodevelopmental disorders (62), which further suggests that we need to pay more attention to the children with schizophrenia.
It has been commonly accepted that children, compared to adults, develop with better neural and behavioral plasticity, so early intervention for children with schizophrenia is necessary. In addition, although the main treatment of schizophrenia is antipsychotics, some other therapies have also been put forward to promote the recovery of cognitive function in schizophrenia patients, which in turn help establish adaptive behaviors and have gradually been introduced to children.
Cognitive behavioral therapy (CBT) refers to a class of interventions that share the basic premise that mental disorders and psychological distress are maintained by cognitive factors (101, 102). Therefore, CBT approaches are often used in responding to some cognitive symptoms in a wide range of psychiatric disorders and show a large effect size (103). A review of meta-analysis on the efficacy of CBT has confirmed that the preferential application of CBT in children helped to alleviate behavioral and emotional disorders in various psychosis to some extent (104). In the meantime, a randomized controlled study (105) has found that CBT with treatment as usual (9 months) contributed to better global function and quality of life in adolescents with early-onset schizophrenia, which is considered the external sign of cognitive recovery. Another study on the CBT for adolescents at high risk for serious mental illness (SMI) such as schizophrenia and bipolar disorder indicated that patients asked to engage in several interventions showed reduced difficulties in emotion regulation, better frustration tolerance, and stronger motivation (106). More importantly, CBT is proved to be highly acceptable and feasible with few side effects (104, 107).
Another similar psychopharmacologic treatment commonly used for mental disorders is mindfulness. The definitions of mindfulness are various and have been divided into five dimensions including awareness and attention, present-centeredness, external events, cultivation, and present centeredness (108). An early meta-analysis showed that mindfulness intervention was moderately effective in treating psychotic negative symptoms (109). Recent meta-analyses have provided more evidence for its efficacy and safety (110, 111). Furthermore, mindfulness among children and adolescents has been suggested to have certain clinical effects, while it still needs to be standardized (112). Therefore, Mindfulness-based cognitive therapy for children (MBCT-C) was developed (113) and significant effects were found, including improved cognitive control, better emotional control, and fewer behavioral symptoms (114–116). More importantly mindfulness-based approaches were highly feasible among children and adolescents (117). However, there are few studies on the efficacy of MBCT-C for children, especially schizophrenia. More clinical studies are expected and a comparison could be made between CBT and mindfulness.
However, the tremendous time and human cost of CBT and mindfulness cannot be ignored. To solve this issue, many researchers have been dedicated to intervention with specific cortexes via several neuro-technologies, such as electroconvulsive therapy (ECT). ECT has been proved to be well-tolerated, well-established, and efficacious in multiple moods and psychiatric disorders including depression and schizophrenia (118, 119). However, the majority of results from older studies in children and adolescents were mixed and its effect on them could not be clearly explained. Nowadays, studies and applications of ECT among children and adolescents with FEP become popular and its efficacy has got some verification (120, 121), while more clinic researches are still required. In addition, the side effect of ECT, particularly in cognitive function (e.g., memory loss), has been in heavy discussion for a long time (122, 123). Dossing and Pagsberg (124) suggested that more attention should be paid to cognitive dysfunction under ECT in further studies. To conclude, ECT has been considered a reliable way especially for antipsychotics-resistance patients, while the effect on cognitive functions remains to be vague.
To avoid the argument about safety and enhance the feasibility of neurotechnology, several non-invasive brain stimulation techniques were developed. Transcranial magnetic stimulation (TMS) uses very brief high intensity magnetic fields to induce currents and thus depolarize neurons in small regions of the cortex (125). A review showed that TMS has been applied for the treatment of auditory verbal hallucinations in schizophrenia patients and proved superior efficacy (126). Another repetitive TMS study in EOS patients (127) also reported a significant improvement in the severity of auditory hallucinations and global functioning over the left temporoparietal junction. Additionally, the author appealed to concern about the TMS used in the treatment of several cognitive functions, especially among children. Related studies have shown that TMS in specific cortex like DLPFC contributed to the improvement of inhibitory and executive function in children and early adults (128, 129). However, recent meta-analyses indicate that cognitive enhancement effects caused by TMS in patients with schizophrenia or other neuropsychiatric conditions are moderate (130, 131) and researchers attribute the result to insufficient subjects and lack of long-term TMS intervention. Therefore, more studies in this field are desperately needed.
Another non-invasive brain stimulation technique is transcranial direct current stimulation (tDCS), which has been the subject of great interest owing to its easiness of operation and relatively low cost. tDCS takes effect by applying a weak current over the scalp to modulate cortical excitability by facilitating or inhibiting ongoing neuronal processes. A review of tDCS in psychiatric disorders has demonstrated its high tolerability in patients with schizophrenia and improvement in positive and negative symptoms (132). Meanwhile, one study has provided support that tDCS also played a role in the treatment of cognitive impairments, especially in working memory (133). On this basis, some other researchers focus on the feasibility and safety of tDCS among children and adolescents which is proved to be well-tolerated (134, 135). A recent study of tDCS among children and adolescents with attention-deficit/hyperactivity disorder (ADHD) have indicated positive result in attention and inhibitory control function (136). Another study has reported enhancing working memory performance in children with ADHD under the intervention of tDCS over the left DLPFC (137). In addition, improved reward processing in children with ADHD has also been found through tDCS in specific regions including the left DLPFC and the right ventromedial prefrontal cortex (138). However, a meta-analysis showed little evidence that 1 to 5 sessions of tDCS improve the cognitive measure of children with ADHD (139), which calls for a persistent tDCS study in psychopathic children. Moreover, the study on the clinical effects of tDCS among children with schizophrenia is limited and its real efficacy with cognitive impairments remains to be further examined.
Above all, the current treatments of children with schizophrenia were focused on antipsychotics, which could greatly improve some positive symptoms such as hallucinations and paranoia. In addition, ECT could further enhance the efficiency of treatment in conjunction. However, the above- mentioned therapies are not very effective for negative symptoms (e.g., avolition) and cognitive symptoms (e.g., working memory deficits). Therefore, it is highly recommended to use TMS and tDCS. Moreover, psychopharmacologic treatment including CBT and mindfulness play an important role in helping children with schizophrenia improve cognitive functions and adapt to daily life. Moreover, it is worth emphasizing that persistent treatment is necessary no matter what kind of therapy is chosen.
The current review mainly discusses the cognitive impairments in patients with schizophrenia, especially in children. Previous studies have confirmed that children with schizophrenia are prone to show worse academic grades and more conflicting relationships, accompanied by poor performance in several working memory tasks. In addition, numerous neuroimaging studies have demonstrated altered activation of working memory networks and weak functional connectivity in schizophrenia children. To further explain the pathogenesis of schizophrenia, many susceptible genes have been put forward to take effect in related activities among specific cerebral cortexes which finally accounts for working memory deficits in schizophrenia. Thus, findings of the early presence of cognitive symptoms both in behavior and cerebral cortex offer the opportunity for prevention and treatment of schizophrenia in children. Currently, lots of psychologists and psychiatrists have contributed to the enhancement of cognitive impairments of children with schizophrenia by employing CBT, mindfulness, ECT, TMS, and tDCS.
Although studies on working memory deficits among children with schizophrenia have been constantly emerging, several limitations still exist. Firstly, most working memory tasks used in experiments are mainly designed for adults, and their difficulty and duration might be unsuitable for children. Thus, adapted working memory tasks are required to assess multiple working memory functions more efficiently. Similarly, the treatment for children with schizophrenia is nearly the same as that of adults, and there is also a lack of child intervention studies. Considering the better brain plasticity of children, the brain activity of children might be different from that of adults, so more specific treatment should be studied and developed. Furthermore, the intervention duration of several existing studies on children is not enough which may be one of the reasons for the inconsistent treatment effects. Therefore, more long-term follow-up studies are needed.
In the future, the mechanism of working memory and other cognition impairments in schizophrenia are needed to be explored. On this basis, it is worth looking forward to the more effective and lower-cost therapy for schizophrenia patients, especially for children.
WZ had full access to all the information in this review. WZ, JZ, and JL managed the literature searches and selection. WZ and QZ reviewed the logicality of the manuscript. PO and QZ polished the manuscript in language expression. JZ wrote the first draft of the manuscript. All authors contributed to the article and approved the submitted version.
We would like to thank all the volunteers from all the mentioned studies.
The authors declare that the research was conducted in the absence of any commercial or financial relationships that could be construed as a potential conflict of interest.
All claims expressed in this article are solely those of the authors and do not necessarily represent those of their affiliated organizations, or those of the publisher, the editors and the reviewers. Any product that may be evaluated in this article, or claim that may be made by its manufacturer, is not guaranteed or endorsed by the publisher.
1. Tandon R, Gaebel W, Barch DM, Bustillo J, Gur RE, Heckers S, et al. Definition and description of schizophrenia in the Dsm-5. Schizophr Res. (2013) 150:3–10. doi: 10.1016/j.schres.2013.05.028
2. Simpson EH, Kellendonk C, Kandel E. A Possible role for the striatum in the pathogenesis of the cognitive symptoms of schizophrenia. Neuron. (2010) 65:585–96. doi: 10.1016/j.neuron.2010.02.014
3. Harvey PD, Koren D, Reichenberg A, Bowie CR. Negative symptoms and cognitive deficits: what is the nature of their relationship? Schizophrenia Bull. (2006) 32:250–8. doi: 10.1093/schbul/sbj011
4. Rector NA, Beck AT, Stolar N. The Negative symptoms of schizophrenia: a cognitive perspective. Can J Psychiat. (2005) 50:247–57. doi: 10.1177/070674370505000503
5. McCutcheon RA, Abi-Dargham A, Howes OD. Schizophrenia, dopamine and the striatum: from biology to symptoms. Trends Neurosci. (2019) 42:205–20. doi: 10.1016/j.tins.2018.12.004
6. Kapur S, Remington G. Serotonin-dopamine interaction and its relevance to schizophrenia. Am J Psychiatry. (1996) 153:466–76. doi: 10.1176/ajp.153.4.466
7. Stahl SM. Beyond the dopamine hypothesis of schizophrenia to three neural networks of psychosis: dopamine, serotonin, and glutamate. CNS Spectr. (2018) 23:187–91. doi: 10.1017/S1092852918001013
8. Charlson F, van Ommeren M, Flaxman A, Cornett J, Whiteford H, Saxena S. New who prevalence estimates of mental disorders in conflict settings: a systematic review and meta-analysis. Lancet. (2019) 394:240–8. doi: 10.1016/S0140-6736(19)30934-1
9. Chan KY, Zhao FF, Meng SJ, Demaio AR, Reed C, Theodoratou E, et al. Prevalence of schizophrenia in China between 1990 and 2010. J Glob Health. (2015) 5:142–9. doi: 10.1002/wps.20222
10. Chong HY, Teoh SL, Wu DBC, Kotirum S, Chiou CF, Chaiyakunapruk N. Global economic burden of schizophrenia: a systematic review. Neuropsych Dis Treat. (2016) 12:357–73. doi: 10.2147/NDT.S96649
11. Jaaskelainen E, Juola P, Hirvonen N, McGrath JJ, Saha S, Isohanni M, et al. A systematic review and meta-analysis of recovery in schizophrenia. Schizophrenia Bull. (2013) 39:1296–306. doi: 10.1093/schbul/sbs130
12. Phahladira L, Luckhoff HK, Asmal L, Kilian S, Scheffler F, Plessis SD, et al. Early recovery in the first 24 months of treatment in first-episode schizophrenia-spectrum disorders. Npj Schizophr. (2020) 6:2. doi: 10.1038/s41537-019-0091-y
13. Nuechterlein KH, Subotnik KL, Green MF, Ventura J, Asarnow RF, Gitlin MJ, et al. Neurocognitive predictors of work outcome in recent-onset schizophrenia. Schizophrenia Bull. (2011) 37:S33–40. doi: 10.1093/schbul/sbr084
14. Sigel IE. The psychological distancing model: a study of the socialization of cognition. Cult Psychol. (2002) 8:189–214. doi: 10.1177/1354067X02008002438
15. Jedrziewski MK, Ewbank DC, Wang HD, Trojanowski JQ. The impact of exercise, cognitive activities, and socialization on cognitive function: results from the national long-term care survey. Am J Alzheimers Dis. (2014) 29:372–8. doi: 10.1177/1533317513518646
16. Bora E. Differences in cognitive impairment between schizophrenia and bipolar disorder: considering the role of heterogeneity. Psychiat Clin Neuros. (2016) 70:424–33. doi: 10.1111/pcn.12410
17. Fioravanti M, Carlone O, Vitale B, Cinti ME, Clare L. A Meta-analysis of cognitive deficits in adults with a diagnosis of schizophrenia. Neuropsychol Rev. (2005) 15:73–95. doi: 10.1007/s11065-005-6254-9
18. Bortolato B, Miskowiak KW, Kohler CA, Vieta E, Carvalho AF. Cognitive dysfunction in bipolar disorder and schizophrenia: a systematic review of meta-analyses. Neuropsych Dis Treat. (2015) 11:3111–25. doi: 10.2147/NDT.S76700
19. Forbes NF, Carrick LA, McIntosh AM, Lawrie SM. Working memory in schizophrenia: a meta-analysis. Psychol Med. (2009) 39:889–905. doi: 10.1017/S0033291708004558
20. Cameron AM, Oram J, Geffen GM, Kavanagh DJ, McGrath JJ, Geffen LB. Working memory correlates of three symptom clusters in schizophrenia. Psychiat Res. (2002) 110:49–61. doi: 10.1016/S0165-1781(02)00036-7
21. Perlstein WM, Carter CS, Noll DC, Cohen JD. Relation of prefrontal cortex dysfunction to working memory and symptoms in schizophrenia. Am J Psychiat. (2001) 158:1105–13. doi: 10.1176/appi.ajp.158.7.1105
22. Baddeley A. Working memory: theories, models, and controversies. Annu Rev Psychol. (2012) 63:1–29. doi: 10.1146/annurev-psych-120710-100422
23. Reichenberg A, Caspi A, Harrington H, Houts R, Keefe RSE, Murray RM, et al. Static and dynamic cognitive deficits in childhood preceding adult schizophrenia: a 30-year study. Am J Psychiat. (2010) 167:160–9. doi: 10.1176/appi.ajp.2009.09040574
24. Lieberman JA, Perkins D, Belger A, Chakos M, Jarskog F, Boteva K, et al. The early stages of schizophrenia: speculations on pathogenesis, pathophysiology, and therapeutic approaches. Biol Psychiatry. (2001) 50:884–97. doi: 10.1016/S0006-3223(01)01303-8
25. Jundong J, Kuja-Halkola R, Hultman C, Langstrom N, D'Onofrio BM, Lichtenstein P. Poor school performance in offspring of patients with schizophrenia: what are the mechanisms? Psychol Med. (2012) 42:111–23. doi: 10.1017/S0033291711001127
26. de Nijs J, Pet MA, Investigators G. Metabolic syndrome in schizophrenia patients associated with poor premorbid school performance in early adolescence. Acta Psychiat Scand. (2016) 133:289–97. doi: 10.1111/acps.12528
27. Mutsatsa SH, Joyce EM, Hutton SB, Barnes TRE. Relationship between insight, cognitive function, social function and symptomatology in schizophrenia - the West London first episode study. Eur Arch Psy Clin N. (2006) 256:356–63. doi: 10.1007/s00406-006-0645-7
28. Harvey PD, Silverman JM, Mohs RC, Parrella M, White L, Powchik P, et al. Cognitive decline in late-life schizophrenia: a longitudinal study of geriatric chronically hospitalized patients. Biol Psychiatry. (1999) 45:32–40. doi: 10.1016/S0006-3223(98)00273-X
29. Harrop C, Trower P. Why does schizophrenia develop at late adolescence? Clin Psychol Rev. (2001) 21:241–65. doi: 10.1016/S0272-7358(99)00047-1
30. Lally J, Ajnakina O, Stubbs B, Cullinane M, Murphy KC, Gaughran F, et al. Remission and recovery from first-episode psychosis in adults: systematic review and meta-analysis of long-term outcome studies. Brit J Psychiat. (2017) 211:350. doi: 10.1192/bjp.bp.117.201475
31. Karson C, Duffy RA, Eramo A, Nylander AG, Offord SJ. Long-term outcomes of antipsychotic treatment in patients with first-episode schizophrenia: a systematic review. Neuropsych Dis Treat. (2016) 12:57–67. doi: 10.2147/NDT.S96392
32. Yeo RA, Hodde-Vargas J, Hendren RL, Vargas LA, Brooks WM, Ford CC, et al. Brain abnormalities in schizophrenia-spectrum children: implications for a neurodevelopmental perspective. Psychiatry Res. (1997) 76:1–13. doi: 10.1016/S0925-4927(97)00056-5
33. Barch DM, Smith E. The cognitive neuroscience of working memory: relevance to cntrics and schizophrenia. Biol Psychiat. (2008) 64:11–7. doi: 10.1016/j.biopsych.2008.03.003
34. Ettinger U, Picchioni M, Hall MH, Schulze K, Toulopoulou T, Landau S, et al. Antisaccade performance in monozygotic twins discordant for schizophrenia: the maudsley twin study. Am J Psychiatry. (2006) 163:543–5. doi: 10.1176/appi.ajp.163.3.543
35. Poppe AB, Barch DM, Carter CS, Gold JM, Ragland JD, Silverstein SM, et al. Reduced frontoparietal activity in schizophrenia is linked to a specific deficit in goal maintenance: a multisite functional imaging study. Schizophrenia Bull. (2016) 42:1149–57. doi: 10.1093/schbul/sbw036
36. Gotra MY, Keedy SK, Hill SK. Interactive effects of maintenance decay and interference on working memory updating in schizophrenia. Schizophr Res. (2022) 239:103–10. doi: 10.1016/j.schres.2021.11.028
37. Galletly CA, McFarlane AC, Clark CR. Impaired updating of working memory in schizophrenia. Int J Psychophysiol. (2007) 63:265–74. doi: 10.1016/j.ijpsycho.2006.11.004
38. Caldani S, Bucci MP, Lamy JC, Seassau M, Bendjemaa N, Gadel R, et al. Saccadic eye movements as markers of schizophrenia spectrum: exploration in at-risk mental states. Schizophr Res. (2017) 181:30–7. doi: 10.1016/j.schres.2016.09.003
39. Ross RG, Harris JG, Olincy A, Radant A. Eye Movement task measures inhibition and spatial working memory in adults with schizophrenia, adhd, and a normal comparison group. Psychiat Res. (2000) 95:35–42. doi: 10.1016/S0165-1781(00)00153-0
40. Dickson H, Hedges EP, Ma SY, Cullen AE, MacCabe JH, Kempton MJ, et al. Academic achievement and schizophrenia: a systematic meta-analysis. Psychol Med. (2020) 50:1949–65. doi: 10.1017/S0033291720002354
41. Boada L, Lahera G, Pina-Camacho L, Merchan-Naranjo J, Diaz-Caneja CM, Bellon JM, et al. Social cognition in autism and schizophrenia spectrum disorders: the same but different? J Autism Dev Disord. (2020) 50:3046–59. doi: 10.1007/s10803-020-04408-4
42. Habel U, Krasenbrink I, Bowi U, Ott G, Schneider F. A Special role of negative emotion in children and adolescents with schizophrenia and other psychoses. Psychiatry Res. (2006) 145:9–19. doi: 10.1016/j.psychres.2005.11.001
43. Ziermans TB. Working memory capacity and psychotic-like experiences in a general population sample of adolescents and young adults. Front Psychiatry. (2013) 4:161. doi: 10.3389/fpsyt.2013.00161
44. White T, Schmidt M, Karatekin C. Verbal and visuospatial working memory development and deficits in children and adolescents with schizophrenia. Early Interv Psychiatry. (2010) 4:305–13. doi: 10.1111/j.1751-7893.2010.00204.x
45. Seidman LJ, Meyer EC, Giuliano AJ, Breiter HC, Goldstein JM, Kremen WS, et al. Auditory working memory impairments in individuals at familial high risk for schizophrenia. Neuropsychology. (2012) 26:288–303. doi: 10.1037/a0027970
46. Piskulic D, Olver JS, Norman TR, Maruff P. Behavioural studies of spatial working memory dysfunction in schizophrenia: a quantitative literature review. Psychiat Res. (2007) 150:111–21. doi: 10.1016/j.psychres.2006.03.018
47. Kenny JT, Friedman L, Findling RL, Swales TP, Strauss ME, Jesberger JA, et al. Cognitive impairment in adolescents with schizophrenia. Am J Psychiatry. (1997) 154:1613–5. doi: 10.1176/ajp.154.11.1613
48. Loeb FF, Zhou XP, Craddock KES, Shora L, Broadnax DD, Gochman P, et al. Reduced functional brain activation and connectivity during a working memory task in childhood-onset schizophrenia. J Am Acad Child Psy. (2018) 57:166–74. doi: 10.1016/j.jaac.2017.12.009
49. Bittner RA, Linden DEJ, Roebroeck A, Hartling F, Rotarska-Jagiela A, Maurer K, et al. The when and where of working memory dysfunction in early-onset schizophrenia-a functional magnetic resonance imaging study. Cereb Cortex. (2015) 25:2494–506. doi: 10.1093/cercor/bhu050
50. Schneider F, Habel U, Reske M, Kellermann T, Stocker T, Shah NJ, et al. Neural correlates of working memory dysfunction in first-episode schizophrenia patients: an fmri multi-center study. Schizophr Res. (2007) 89:198–210. doi: 10.1016/j.schres.2006.07.021
51. Green MF. What are the functional consequences of neurocognitive deficits in schizophrenia? Am J Psychiat. (1996) 153:321–30. doi: 10.1176/ajp.153.3.321
52. Kyriakopoulos M, Dima D, Roiser JP, Corrigall R, Barker GJ, Frangou S. Abnormal functional activation and connectivity in the working memory network in early-onset schizophrenia. J Am Acad Child Adolesc Psychiatry. (2012) 51:911–20 e2. doi: 10.1016/j.jaac.2012.06.020
53. Nielsen JD, Madsen KH, Wang Z, Liu Z, Friston KJ, Zhou Y. Working memory modulation of frontoparietal network connectivity in first-episode schizophrenia. Cereb Cortex. (2017) 27:3832–41. doi: 10.1093/cercor/bhx050
54. Tan HY, Choo WC, Fones CSL, Chee MWL. FMRI study of maintenance and manipulation processes within working memory in first-episode schizophrenia. Am J Psychiat. (2005) 162:1849–58. doi: 10.1176/appi.ajp.162.10.1849
55. Thormodsen R, Jensen J, Holmen A, Juuhl-Langseth M, Emblem KE, Andreassen OA, et al. Prefrontal hyperactivation during a working memory task in early-onset schizophrenia spectrum disorders: an FMRI study. Psychiatry Res. (2011) 194:257–62. doi: 10.1016/j.pscychresns.2011.05.011
56. Sullivan PF, Kendler KS, Neale MC. Schizophrenia as a complex trait - evidence from a meta-analysis of twin studies. Arch Gen Psychiat. (2003) 60:1187–92. doi: 10.1001/archpsyc.60.12.1187
57. Hilker R, Helenius D, Fagerlund B, Skytthe A, Christensen K, Werge TM, et al. Heritability of schizophrenia and schizophrenia spectrum based on the nationwide Danish twin register. Biol Psychiat. (2018) 83:492–8. doi: 10.1016/j.biopsych.2017.08.017
58. Lemvigh C, Brouwer R, Pantelis C, Hilker R, Robbins TW, Sahakian B, et al. Heritability of specific cognitive functions and associations with schizophrenia spectrum disorders using cantab: a nation-wide twin study. Schizophrenia Bull. (2019) 45:S171–S. doi: 10.1093/schbul/sbz021.206
59. Adler CM, Holland SK, Schmithorst V, Tuchfarber MJ, Strakowski SM. Changes in neuronal activation in patients with bipolar disorder during performance of a working memory task. Bipolar Disord. (2004) 6:540–9. doi: 10.1111/j.1399-5618.2004.00117.x
60. Schizophrenia Psychiatric Genome-Wide Association Study C. Genome-wide association study identifies five new schizophrenia loci. Nat Genet. (2011) 43:969–76. doi: 10.1038/ng.940
61. Schizophrenia Working Group of the Psychiatric Genomics C. Biological insights from 108 schizophrenia-associated genetic loci. Nature. (2014) 511:421–7. doi: 10.1038/nature13595
62. Schizophrenia Schizophrenia Working Group of the Psychiatric Genomics C, Ripke S, Walters JTR, O'Donovan MC. Mapping genomic loci prioritises genes and implicates synaptic biology in schizophrenia. medRxiv. (2020) 2:2922. doi: 10.1101/2020.09.12.20192922
63. O'Donovan MC, Craddock N, Norton N, Williams H, Peirce T, Moskvina V, et al. Identification of loci associated with schizophrenia by genome-wide association and follow-up. Nat Genet. (2008) 40:1053–5. doi: 10.1038/ng.201
64. Zhang YYA, Yan H, Liao JM Yu H, Jiang SS, Liu Q, et al. Znf804a variation may affect hippocampal-prefrontal resting-state functional connectivity in schizophrenic and healthy individuals. Neurosci Bull. (2018) 34:507–16. doi: 10.1007/s12264-018-0221-y
65. Paulus FM, Krach S, Bedenbender J, Pyka M, Sommer J, Krug A, et al. Partial support for Znf804a genotype-dependent alterations in prefrontal connectivity. Hum Brain Mapp. (2013) 34:304–13. doi: 10.1002/hbm.21434
66. Rasetti R, Sambataro F, Chen Q, Callicott JH, Mattay VS, Weinberger DR. Altered cortical network dynamics: a potential intermediate phenotype for schizophrenia and association with Znf804a. Arch Gen Psychiatry. (2011) 68:1207–17. doi: 10.1001/archgenpsychiatry.2011.103
67. Nicodemus KK, Hargreaves A, Morris D, Anney R, Gill M, Corvin A, et al. Variability in working memory performance explained by epistasis vs polygenic scores in the znf804a pathway. Jama Psychiat. (2014) 71:778–85. doi: 10.1001/jamapsychiatry.2014.528
68. Linden DEJ, Lancaster TM, Wolf C, Baird A, Jackson MC, Johnston SJ, et al. Znf804a genotype modulates neural activity during working memory for faces. Neuropsychobiology. (2013) 67:84–92. doi: 10.1159/000344001
69. Thurin K, Rasetti R, Sambataro F, Safrin M, Chen Q, Callicott JH, et al. Effects of Znf804a on neurophysiologic measures of cognitive control. Mol Psychiatr. (2013) 18:852–4. doi: 10.1038/mp.2012.134
70. Del Re EC, Bergen SE, Mesholam-Gately RI, Niznikiewicz MA, Goldstein JM, Woo TU, et al. Analysis of schizophrenia-related genes and electrophysiological measures reveals Znf804a association with amplitude of P300b elicited by novel sounds. Transl Psychiatry. (2014) 4:e346. doi: 10.1038/tp.2013.117
71. Zheng CM, Shen Y, Xu Q. Rs1076560, a functional variant of the dopamine D2 receptor gene, confers risk of schizophrenia in Han Chinese. Neurosci Lett. (2012) 518:41–4. doi: 10.1016/j.neulet.2012.04.052
72. Monakhov M, Golimbet V, Abramova L, Kaleda V, Karpov V. Association study of three polymorphisms in the dopamine D2 receptor gene and schizophrenia in the Russian population. Schizophr Res. (2008) 100:302–7. doi: 10.1016/j.schres.2008.01.007
73. Gonzalez-Castro TB, Hernandez-Diaz Y, Juarez-Rojop IE, Lopez-Narvaez ML, Tovilla-Zarate CA, Genis-Mendoza A, et al. The role of C957t, Taqi and Ser311cys polymorphisms of the Drd2 gene in schizophrenia: systematic review and meta-analysis. Behav Brain Funct. (2016) 12:114. doi: 10.1186/s12993-016-0114-z
74. Liu L, Fan DZ, Ding N, Hu YT, Cai GQ, Wang L, et al. The relationship between Drd2 gene polymorphisms (C957t and C939t) and schizophrenia: a meta-analysis. Neurosci Lett. (2014) 583:43–8. doi: 10.1016/j.neulet.2014.09.024
75. Persson J, Stenfors C. Superior cognitive goal maintenance in carriers of genetic markers linked to reduced striatal D2 receptor density (C957t and Drd2/Ankk1-Taqia). PLoS ONE. (2018) 13:1837. doi: 10.1371/journal.pone.0201837
76. Klaus K, Butler K, Curtis F, Bridle C, Pennington K. The effect of Ankk1 Taq1a and Drd2 C957t polymorphisms on executive function: a systematic review and meta-analysis. Neurosci Biobehav R. (2019) 100:224–36. doi: 10.1016/j.neubiorev.2019.01.021
77. Gelao B, Fazio L, Selvaggi P, Di Giorgio A, Taurisano P, Quarto T, et al. Drd2 genotype predicts prefrontal activity during working memory after stimulation of D2 receptors with bromocriptine. Psychopharmacology. (2014) 231:2361–70. doi: 10.1007/s00213-013-3398-9
78. Bertolino A, Taurisano P, Pisciotta NM, Blasi G, Fazio L, Romano R, et al. Genetically determined measures of striatal d2 signaling predict prefrontal activity during working memory performance. PLoS ONE. (2010) 5:9348. doi: 10.1371/journal.pone.0009348
79. Braun U, Harneit A, Pergola G, Menara T, Schafer A, Betzel RF, et al. Brain network dynamics during working memory are modulated by dopamine and diminished in schizophrenia. Nat Commun. (2021) 12:694. doi: 10.1038/s41467-021-23694-9
80. Pergola G, Di Carlo P, D'Ambrosio E, Gelao B, Fazio L, Papalino M, et al. Drd2 Co-expression network and a related polygenic index predict imaging, behavioral and clinical phenotypes linked to schizophrenia. Transl Psychiatry. (2017) 7:e1006. doi: 10.1038/tp.2016.253
81. Zhu DJ, Yin JW, Liang CM, Luo XD, Lv D, Dai Z, et al. Cacna1c (Rs1006737) may be a susceptibility gene for schizophrenia: an updated meta-analysis. Brain Behav. (2019) 9:1292. doi: 10.1002/brb3.1292
82. Paulus FM, Bedenbender J, Krach S, Pyka M, Krug A, Sommer J, et al. Association of Rs1006737 in cacna1c with alterations in prefrontal activation and fronto-hippocampal connectivity. Hum Brain Mapp. (2014) 35:1190–200. doi: 10.1002/hbm.22244
83. Bigos KL, Mattay VS, Callicott JH, Straub RE, Vakkalanka R, Kolachana B, et al. Genetic variation in cacna1c affects brain circuitries related to mental illness. Arch Gen Psychiat. (2010) 67:939–45. doi: 10.1001/archgenpsychiatry.2010.96
84. Cosgrove D, Mothersill O, Kendall K, Konte B, Harold D, Giegling I, et al. Cognitive characterization of schizophrenia risk variants involved in synaptic transmission: evidence of cacna1c's role in working memory. Neuropsychopharmacol. (2017) 42:2612–22. doi: 10.1038/npp.2017.123
85. Zhang QM, Shen QG, Xu ZS, Chen M, Cheng LN, Zhai JG, et al. The effects of cacna1c gene polymorphism on spatial working memory in both healthy controls and patients with schizophrenia or bipolar disorder. Neuropsychopharmacol. (2012) 37:677–84. doi: 10.1038/npp.2011.242
86. Spellman T, Rigotti M, Ahmari SE, Fusi S, Gogos JA, Gordon JA. Hippocampal-prefrontal input supports spatial encoding in working memory. Nature. (2015) 522:309. doi: 10.1038/nature14445
87. Moon AL, Haan N, Wilkinson LS, Thomas KL, Hall J. Cacna1c: association with psychiatric disorders, behavior, and neurogenesis. Schizophrenia Bull. (2018) 44:958–65. doi: 10.1093/schbul/sby096
88. Folsom TD, Fatemi SH. The involvement of reelin in neurodevelopmental disorders. Neuropharmacology. (2013) 68:122–35. doi: 10.1016/j.neuropharm.2012.08.015
89. Guidotti A, Grayson DR, Caruncho HJ. Epigenetic Reln dysfunction in schizophrenia and related neuropsychiatric disorders. Front Cell Neurosci. (2016) 10:89. doi: 10.3389/fncel.2016.00089
90. Wedenoja J, Tuulio-Henriksson A, Suvisaari J, Loukola A, Paunio T, Partonen T, et al. Replication of association between working memory and reelin, a potential modifier gene in schizophrenia. Biol Psychiat. (2010) 67:983–91. doi: 10.1016/j.biopsych.2009.09.026
91. Wedenoja J, Loukola A, Tuulio-Henriksson A, Paunio T, Ekelund J, Silander K, et al. Replication of linkage on chromosome 7q22 and association of the regional reelin gene with working memory in schizophrenia families. Mol Psychiatr. (2008) 13:673–84. doi: 10.1038/sj.mp.4002047
92. Baune BT, Konrad C, Suslow T, Domschke K, Birosova E, Sehlmeyer C, et al. The Reelin (Reln) gene is associated with executive function in healthy individuals. Neurobiol Learn Mem. (2010) 94:446–51. doi: 10.1016/j.nlm.2010.08.002
93. Ping J, Zhang J, Wan J, Banerjee A, Huang C, Yu J, et al. Correlation of four single nucleotide polymorphisms of the reln gene with schizophrenia. East Asian Arch Psychiatry. (2021) 31:112–8. doi: 10.12809/eaap2168
94. Alizadeh F, Tavakkoly-Bazzaz J, Bozorgmehr A, Azarnezhad A, Tabrizi M, Ananloo ES. Association of transcription factor 4 (Tcf4) gene Mrna level with schizophrenia, its psychopathology, intelligence and cognitive impairments. J Neurogenet. (2017) 31:344–51. doi: 10.1080/01677063.2017.1396330
95. Badowska DM, Brzozka MM, Kannaiyan N, Thomas C, Dibaj P, Chowdhury A, et al. Modulation of cognition and neuronal plasticity in gain- and loss-of-function mouse models of the schizophrenia risk gene Tcf4. Transl Psychiat. (2020) 10:1026. doi: 10.1038/s41398-020-01026-7
96. Yin JW, Lin JD, Luo XD, Chen YY Li Z, Ma GD, et al. Mir-137: a new player in schizophrenia. Int J Mol Sci. (2014) 15:3262–71. doi: 10.3390/ijms15023262
97. Cosgrove D, Harold D, Mothersill O, Anney R, Hill MJ, Bray NJ, et al. Mir-137-derived polygenic risk: effects on cognitive performance in patients with schizophrenia and controls. Transl Psychiat. (2017) 7:286. doi: 10.1038/tp.2016.286
98. Liu S, Li A, Liu Y, Li J, Wang M, Sun YQ, et al. Mir137polygenic risk is associated with schizophrenia and affects functional connectivity of the dorsolateral prefrontal cortex. Psychol Med. (2020) 50:1510–8. doi: 10.1017/S0033291719001442
99. Nicodemus KK, Kolachana BS, Vakkalanka R, Straub RE, Giegling I, Egan MF, et al. Evidence for statistical epistasis between catechol-o-methyltransferase (Comt) and polymorphisms in Rgs4, G72 (Daoa), Grm3, and Disc1: influence on risk of schizophrenia. Hum Genet. (2007) 120:889–906. doi: 10.1007/s00439-006-0257-3
100. Wang C, Liu B, Zhang XL, Cui Y, Yu CS, Jiang TZ. Multilocus genetic profile in dopaminergic pathway modulates the striatum and working memory. Sci Rep-UK. (2018) 8:2319. doi: 10.1038/s41598-018-23191-y
101. Beck AT. Cognitive therapy: nature and relation to behavior therapy - republished article. Behav Ther. (2016) 47:776–84. doi: 10.1016/j.beth.2016.11.003
102. Dryden W, Bond FW. Reason and emotion in psychotherapy: Albert Ellis. Br J Psychiatry. (1994) 165:131–5. doi: 10.1192/bjp.165.1.131
103. Butler AC, Chapman JE, Forman EM, Beck AT. The empirical status of cognitive-behavioral therapy: a review of meta-analyses. Clin Psychol Rev. (2006) 26:17–31. doi: 10.1016/j.cpr.2005.07.003
104. Hofmann SG, Asnaani A, Vonk IJ, Sawyer AT, Fang A. The efficacy of cognitive behavioral therapy: a review of meta-analyses. Cognit Ther Res. (2012) 36:427–40. doi: 10.1007/s10608-012-9476-1
105. Muller H, Kommescher M, Guttgemanns J, Wessels H, Walger P, Lehmkuhl G, et al. Cognitive behavioral therapy in adolescents with early-onset psychosis: a randomized controlled pilot study. Eur Child Adoles Psy. (2020) 29:1011–22. doi: 10.1007/s00787-019-01415-4
106. Weintraub MJ, Zinberg J, Bearden CE, Miklowitz DJ. Applying a transdiagnostic cognitive-behavioral treatment to adolescents at high risk for serious mental illness: rationale and preliminary findings. Cogn Behav Pract. (2020) 27:202–14. doi: 10.1016/j.cbpra.2019.07.007
107. Aldao A, Nolen-Hoeksema S, Schweizer S. Emotion-regulation strategies across psychopathology: a meta-analytic review. Clin Psychol Rev. (2010) 30:217–37. doi: 10.1016/j.cpr.2009.11.004
108. Nilsson H, Kazemi A. Reconciling and thematizing definitions of mindfulness: the big five of mindfulness. Rev Gen Psychol. (2016) 20:183–93. doi: 10.1037/gpr0000074
109. Khoury B, Lecomte T, Gaudiano BA, Paquin K. Mindfulness interventions for psychosis: a meta-analysis. Schizophr Res. (2013) 150:176–84. doi: 10.1016/j.schres.2013.07.055
110. Louise S, Fitzpatrick M, Strauss C, Rossell SL, Thomas N. Mindfulness- and acceptance-based interventions for psychosis: our current understanding and a meta-analysis. Schizophr Res. (2018) 192:57–63. doi: 10.1016/j.schres.2017.05.023
111. Jansen JE, Gleeson J, Bendall S, Rice S, Alvarez-Jimenez M. Acceptance- and mindfulness-based interventions for persons with psychosis: a systematic review and meta-analysis. Schizophr Res. (2020) 215:25–37. doi: 10.1016/j.schres.2019.11.016
112. Thompson M, Gauntlett-Gilbert J. Mindfulness with children and adolescents: effective clinical application. Clin Child Psychol Psychiatry. (2008) 13:395–407. doi: 10.1177/1359104508090603
113. Randye J, Semple JL. Chapter 8 - Mindfulness-Based Cognitive Therapy for Children. Mindfulness-Based Treatment Approaches (Second Edition). (2014) 2:161–88. doi: 10.1016/B978-0-12-416031-6.00008-6
114. Schonert-Reichl KA, Oberle E, Lawlor MS, Abbott D, Thomson K, Oberlander TF, et al. Enhancing cognitive and social-emotional development through a simple-to-administer mindfulness-based school program for elementary school children: a randomized controlled trial. Dev Psychol. (2015) 51:52–66. doi: 10.1037/a0038454
115. Santonastaso O, Zaccari V, Crescentini C, Fabbro F, Capurso V, Vicari S, et al. Clinical application of mindfulness-oriented meditation: a preliminary study in children with Adhd. Int J Env Res Pub He. (2020) 17:6916. doi: 10.3390/ijerph17186916
116. Bauer CCC, Caballero C, Scherer E, West MR, Mrazek MD, Phillips DT, et al. Mindfulness Training Reduces Stress and Amygdala Reactivity to Fearful Faces in Middle-School Children. Behav Neurosci. (2019) 133:569–85. doi: 10.1037/bne0000337
117. Burke CA. Mindfulness-based approaches with children and adolescents: a preliminary review of current research in an emergent field. J Child Fam Stud. (2010) 19:133–44. doi: 10.1007/s10826-009-9282-x
118. Grover S, Sahoo S, Rabha A, Koirala R. Ect in schizophrenia: a review of the evidence. Acta Neuropsychiatr. (2019) 31:115–27. doi: 10.1017/neu.2018.32
119. Pagnin D, de Queiroz V, Pini S, Cassano GB. Efficacy of ect in depression: a meta-analytic review. J Ect. (2004) 20:13–20. doi: 10.1097/00124509-200403000-00004
120. Zhang ZJ, Chen YC, Wang HN, Wang HH, Xue YY, Feng SF, et al. Electroconvulsive therapy improves antipsychotic and somnographic responses in adolescents with first-episode psychosis - a case-control study. Schizophr Res. (2012) 137:97–103. doi: 10.1016/j.schres.2012.01.037
121. Benson NM, Seiner SJ. Electroconvulsive therapy in children and adolescents: clinical indications and special considerations. Harvard Rev Psychiat. (2019) 27:354–8. doi: 10.1097/HRP.0000000000000236
122. Kaster TS, Daskalakis ZJ, Blumberger DM. Clinical effectiveness and cognitive impact of electroconvulsive therapy for schizophrenia: a large retrospective study. J Clin Psychiat. (2017) 78:E383. doi: 10.4088/JCP.16m10686
123. Porter RJ, Baune BT, Morris G, Hamilton A, Bassett D, Boyce P, et al. Cognitive side-effects of electroconvulsive therapy: what are they, how to monitor them and what to tell patients. Bjpsych Open. (2020) 6:17. doi: 10.1192/bjo.2020.17
124. Dossing E, Pagsberg AK. Electroconvulsive therapy in children and adolescents a systematic review of current literature and guidelines. J Ect. (2021) 37:158–70. doi: 10.1097/YCT.0000000000000761
125. Barker AT, Jalinous R, Freeston IL. Non-invasive magnetic stimulation of human motor cortex. Lancet. (1985) 1:1106–7. doi: 10.1016/S0140-6736(85)92413-4
126. Slotema CW, Blom JD, van Lutterveld R, Hoek HW, Sommer IEC. Review of the efficacy of transcranial magnetic stimulation for auditory verbal hallucinations. Biol Psychiat. (2014) 76:101–10. doi: 10.1016/j.biopsych.2013.09.038
127. Jardri R, Bubrovszky M, Demeulemeester M, Poulet E, Januel D, Cohen D, et al. Repetitive transcranial magnetic stimulation to treat early-onset auditory hallucinations. J Am Acad Child Psy. (2012) 51:947–9. doi: 10.1016/j.jaac.2012.06.010
128. Croarkin PE, Wall CA, Lee J. Applications of Transcranial magnetic stimulation (Tms) in child and adolescent psychiatry. Int Rev Psychiatry. (2011) 23:445–53. doi: 10.3109/09540261.2011.623688
129. Ameis SH, Blumberger DM, Croarkin PE, Mabbott DJ, Lai MC, Desarkar P, et al. Treatment of executive function deficits in autism spectrum disorder with repetitive transcranial magnetic stimulation: a double-blind, sham-controlled, pilot trial. Brain Stimul. (2020) 13:539–47. doi: 10.1016/j.brs.2020.01.007
130. Martin DM, McClintock SM, Forster J, Loo CK. Does Therapeutic repetitive transcranial magnetic stimulation cause cognitive enhancing effects in patients with neuropsychiatric conditions? A systematic review and meta-analysis of randomised controlled trials. Neuropsychol Rev. (2016) 26:295–309. doi: 10.1007/s11065-016-9325-1
131. Sloan NP, Byrne LK, Enticott PG, Lum JAG. Non-invasive brain stimulation does not improve working memory in schizophrenia: a meta-analysis of randomised controlled trials. Neuropsychol Rev. (2021) 31:115–38. doi: 10.1007/s11065-020-09454-4
132. Tortella G, Casati R, Aparicio LV, Mantovani A, Senco N, D'Urso G, et al. Transcranial direct current stimulation in psychiatric disorders. World J Psychiatry. (2015) 5:88–102. doi: 10.5498/wjp.v5.i1.88
133. Jeon DW, Jung DU, Kim SJ, Shim JC, Moon JJ, Seo YS, et al. Adjunct transcranial direct current stimulation improves cognitive function in patients with schizophrenia: a double-blind 12-week study. Schizophr Res. (2018) 197:378–85. doi: 10.1016/j.schres.2017.12.009
134. Rivera-Urbina GN, Nitsche MA, Vicario CM, Molero-Chamizo A. Applications of transcranial direct current stimulation in children and pediatrics. Rev Neurosci. (2017) 28:173–84. doi: 10.1515/revneuro-2016-0045
135. Andrade AC, Magnavita GM, Allegro JVBN, Neto CEBP, Lucena RDS, Fregni F. Feasibility of transcranial direct current stimulation use in children aged 5 to 12 years. J Child Neurol. (2014) 29:1360–5. doi: 10.1177/0883073813503710
136. Guimaraes RSQ, Bandeira ID, Barretto BL, Barretto TL, Wanke T, Alves COC, et al. The Effects of Transcranial direct current stimulation on attention and inhibitory control of children and adolescents with attention-deficit/hyperactivity disorder (Adhd): Study protocol for a randomized, sham-controlled, triple-blind, cross-over trial. Medicine. (2021) 100:e24283. doi: 10.1097/MD.0000000000024283
137. Nejati V, Rasanan AHH, Rad JA, Alavi MM, Haghi S, Nitsche MA. Transcranial direct current stimulation (Tdcs) alters the pattern of information processing in children with adhd: evidence from drift diffusion modeling. Neurophysiol Clin. (2021). doi: 10.1016/j.neucli.2021.11.005
138. Nejati V, Sarraj Khorrami A, Nitsche MA. Transcranial direct current stimulation improves reward processing in children with Adhd. J Atten Disord. (2021) 25:1623–31. doi: 10.1177/1087054720923094
Keywords: schizophrenia, children, working memory, genetic susceptibility, cognitive intervention
Citation: Zhou J, Li J, Zhao Q, Ou P and Zhao W (2022) Working memory deficits in children with schizophrenia and its mechanism, susceptibility genes, and improvement: A literature review. Front. Psychiatry 13:899344. doi: 10.3389/fpsyt.2022.899344
Received: 18 March 2022; Accepted: 20 July 2022;
Published: 05 August 2022.
Edited by:
Xiaoming Li, University of South Carolina, United StatesCopyright © 2022 Zhou, Li, Zhao, Ou and Zhao. This is an open-access article distributed under the terms of the Creative Commons Attribution License (CC BY). The use, distribution or reproduction in other forums is permitted, provided the original author(s) and the copyright owner(s) are credited and that the original publication in this journal is cited, in accordance with accepted academic practice. No use, distribution or reproduction is permitted which does not comply with these terms.
*Correspondence: Wan Zhao, emhhb3dhbjExMDhAaG90bWFpbC5jb20=
Disclaimer: All claims expressed in this article are solely those of the authors and do not necessarily represent those of their affiliated organizations, or those of the publisher, the editors and the reviewers. Any product that may be evaluated in this article or claim that may be made by its manufacturer is not guaranteed or endorsed by the publisher.
Research integrity at Frontiers
Learn more about the work of our research integrity team to safeguard the quality of each article we publish.