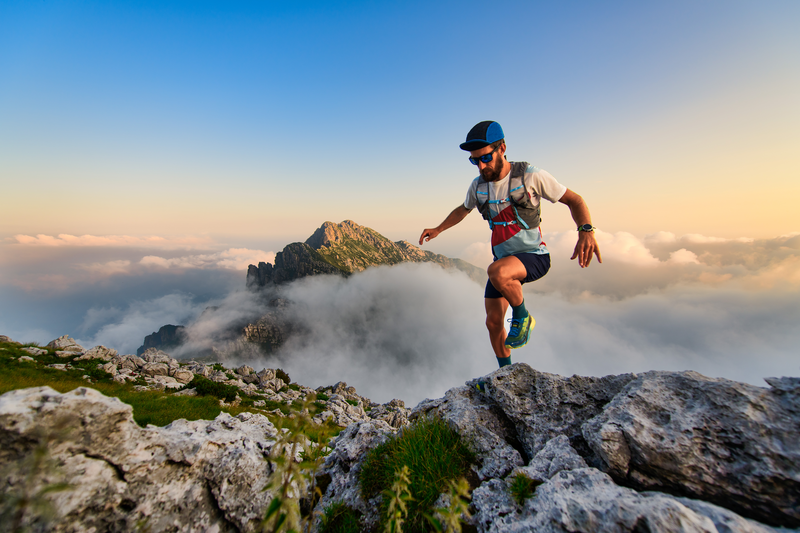
95% of researchers rate our articles as excellent or good
Learn more about the work of our research integrity team to safeguard the quality of each article we publish.
Find out more
ORIGINAL RESEARCH article
Front. Psychiatry , 24 June 2022
Sec. Molecular Psychiatry
Volume 13 - 2022 | https://doi.org/10.3389/fpsyt.2022.895852
This article is part of the Research Topic Behavioral Dysfunctions in Neuropsychiatric Diseases: Exploring New Therapeutic Targets View all 6 articles
Background: The underlying biological mechanisms for neurological symptoms following a traumatic brain injury (TBI) remain poorly understood. This study investigated the associations between serum inflammatory biomarkers and neurological symptoms in the chronic phase following moderate to severe TBI.
Methods: The serum interleukin [IL]-1β, IL-4, IL-5, IL-6, IL-7, IL-8, IL-10, IL-12p70, and the tumor necrosis factor [TNF]-α in 72 TBI patients 6 months to 2 years post injury were measured. Neurological symptoms including depression, chronic headache, sleep disturbance, irritability, anxiety, and global neurological disability was assessed. The associations between the biomarkers and the neurological symptoms were assessed using correlation and regression analysis.
Results: It was found that the most common post-injury symptom was sleep disturbance (84.7%), followed by chronic headaches (59.7%), irritability (55.6%), and depression (54.2%). TNF-α was a protective factor for chronic headache (OR = 0.473, 95% CI = 0.235–0.952). IL-6 was positively associated with sleep disturbance (r = 0.274, p = 0.021), while IL-5 and IL-12p70 were negatively associated with the degree of global neurological disability (r = −0.325, p = 0.006; r = −0.319, p = 0.007).
Conclusion: This study provides preliminary evidence for the association between chronic inflammation with neurological symptoms following a TBI, which suggests that anti-inflammatory could be a potential target for post-TBI neurological rehabilitation. Further research with larger sample sizes and more related biomarkers are still needed, however, to elucidate the inflammatory mechanisms for this association.
Traumatic brain injuries (TBIs) are one of the leading causes of death and disability worldwide (1), with around 5.3 million people in the United States and nearly 7.7 million people in Europe living with a TBI-related disability (2). Post-TBI neurological symptoms, such as poor executive function, impaired attention, depression, sleep disturbance, chronic headaches, emotional dysregulation, poor decision-making, and aggressive behavior (3–5), can be a heavy burden on individuals, families, and society. TBIs are often described as a “silent epidemic” because the patients and family members know how devastating the effects can be; however, awareness is low and research into TBI-related neurological symptoms has been sparse, with the underlying mechanisms still being largely unknown (1).
Inflammation is an important secondary injury mechanisms after TBI (6) and has been reported to be associated with many neurological symptoms, such as depression, sleep disturbance, and headaches. Many studies have documented the associations between depression and immune dysregulation (7–9), and it has been found that higher concentrations of pro-inflammatory cytokines can directly contribute to the development of depressive symptoms (10). Dowlati et al. found that TNF-α and IL-6 concentrations were significantly higher in patients with depression compared with healthy control subjects, and suggested that depression was accompanied by an activation of the inflammatory response system (11). Review studies have also found that inflammation, and especially neuroinflammation, was one of the mechanisms for chronic post-traumatic headaches (12–14). Moreover, multiple line of evidence showed a reciprocal connection between the immune system and sleep, which suggested that the dysregulation of inflammation could be contributing to sleep disturbances, and vice versa (15, 16). Specifically, Irwin et al. reported that sleep disturbance was associated with increases in systemic inflammation (higher levels of CRP and IL-6) (16).
Based on these surmised associations between inflammation, TBI, and neurological symptoms, it is plausible to suggest that inflammation may be connected with post-TBI neurological disabilities. Several studies have investigated the associations between acute inflammation with the long-term outcome following TBI. For example, Nwachuku et al. reported that the cerebrospinal fluid (CSF) concentrations of inflammatory biomarkers (IL-1, IL-6, TNF-α, IFN-γ, IL-12p70, IL-10, and IL-8) in the first 5 days after a severe TBI had a negative association with the 6-month Glasgow Outcome Scale (GOS) scores (17). Juengst et al. found that higher levels of acute CSF cytokine surface markers (sVCAM-1, sICAM-1, and sFAS) were associated with a 3.92-fold increase in the odds of developing depression 6 months after a moderate to severe TBI (18). Kumar et al. reported that IL-1β, IL-6, IL-8, and IL-10 serum levels, and TNF-α were elevated 3 months after a severe TBI, and increased cytokine was, respectively, associated with 1.21 and 1.18 increases in the odds of an unfavorable GOS score at 6 and 12 months post-TBI (19). Similarly, Devoto et al. reported that acute inflammation after a TBI was related to chronic behavioral and neurological symptoms in military personnel (20). These results, therefore, preliminarily linked acute inflammation to long-term post-TBI outcomes; however, it is not clear whether chronic neurological and neurological symptoms in TBI patients are related to chronic inflammation is not clear.
Recent years, emerging researchers proposed that the acute post -TBI inflammation may transit from an adaptive immune response to a chronic, maladaptive response, leading to post-trauma depression and more significant disability (21–23). Several studies have reported that chronic neuroinflammation may exist long-term after a central nervous system (CNS) injury. For example, Ramlackhansingh found that increased microglial activation can be present up to 17 years post-TBI (24), and Johnson et al. found persistent inflammation and ongoing white matter degeneration up to 18 years after a single TBI (25). Studies have also found long-term microglial activation in TBI animals, particularly in subcortical areas remote from the focal injury (26), which was also evident in a postmortem study in people (27). Moreover, Witcher et al. found that microglia activation promotes persistent neuropathology and long-term functional impairments in neuronal homeostasis after TBI (28). On the other side, animal studies demonstrated that microglial depletion during chronic phase of experimental TBI reduced neurodegeneration and neurological deficits, such as cognitive and memory impairments (29–32). These findings provide preliminary evidence that neuroinflammation may persist for years and cause negative outcomes after a TBI; however, whether the systemic inflammation in the long-term phase post-TBI is associated with neurological symptoms is still unclear and needs further study.
To explore the neurological symptoms in the chronic post-TBI phase and their relationship with systemic inflammatory biomarkers, this study assessed the serum cytokines in patients with neurological symptoms more than 6 months after a moderate to severe TBI. The common neurological symptoms and global functions were evaluated using psychological scales. This study's exploration of the inflammation basis for neurological symptoms following a TBI could provide guidance on the provision of treatment and rehabilitation for people with chronic TBI-related neurological disabilities.
TBI patients referred for forensic evaluations for their neurological disability from May 2019 to May 2020 in a forensic center in Sichuan Province, China, were invited to participate. Seventy-two TBI patients were enrolled in the current study. All participants were of Chinese Han ethnicity, the average age was 47.7 ± 12.0 years, and there were 58 males (80.6%, average age was 46.6 ± 12.5 years) and 14 females (19.4%, average age was 52.5 ± 9.1 years). Eighty-nine percent of the injuries had been the result of a traffic accident, while the remaining had been the result of falls. The time interval from the injury to the forensic assessment ranged from 182 to 718 days, with the average interval being 334 ± 163 days (details are presented in Table 1). The enrollment criteria for participants were as follows: (a) 18–60 years old; (b) had a moderate to severe TBI indicated by symptoms and a head computed tomographic scan or magnetic resonance image; and (c) had neurological complaints for more than 6 months post-TBI. Participants were excluded if they: (a) had a cold in the previous month; (b) had a history of an inflammatory disorder, such as rheumatoid arthritis, multiple sclerosis, systemic lupus erythematosus and so on, because patients with these inflammatory diseases may have elevation of systemic inflammatory biomarkers (33–35), which would preclude the independent analysis of TBI-related inflammation; (c) had bleeding disorder because the venipuncture was used in this study; (d) have a history of cigarette smoking in the previous 6 months, because studies indicated that cigarette smoke may induce chronic inflammation and increase expression of inflammatory cytokines, such as IL-6, IL-8 and TNF-α (36–38); (e) had pre-injury neurological or psychiatric disorders (headache, anxiety, depression, sleep disturbance, intelligence deficits and so on), because these pre-injury symptoms may contribute to more severe neuropsychiatric symptoms after traumatic injury (39–41), which would influence the evaluation of the post-injury neurological symptoms; (f) had been dosed with anti-inflammatory or antidepressant drugs in the previous month. This study was approved by the Medical Ethics Committee of Sichuan University and written informed consent was obtained from all participants and their legal guardians.
Peripheral blood samples (2 ml) were collected in sterile tubes with EDTA-Na2 anticoagulants by standard venipuncture from each participant, after which they were centrifuged (3,000 rpm, 15 min) to extract the serum. The serum was then aliquoted and stored at −80°C until the batch analysis. There were nine inflammatory markers measured in the serum: IL-1β, IL-4, IL-5, IL-6, IL-7, IL-8, IL-10, IL-12p70, and TNF-α. The serum levels of these biomarkers were measured using a commercially available electrochemiluminescence immunoassay named U-PLEX Biomarker Group 1 (Human) Multiplex Assay (K15067L-1) (Meso Scale Discovery, Maryland, United States) according to the manufacturer's instructions (https://www.mesoscale.com/~/media/files/product%20inserts/u−plex%20biomarker%20group%201%20human%20insert−multiplex.pdf).A MESO QuickPlex SQ 120 machine and MSD Discovery Workbench software version 4.0.12 were respectively used for the detection and data analysis.
Depression was assessed based on the International Classification of Diseases-10th revision (ICD-10) criteria, with the Patient Health Questionnaire-9 (PHQ-9) (42) employed to quantify the depression severity. The PHQ-9 comprises nine items that describe depressive symptoms, with participants required to rate their feelings in the previous 2 weeks on a 4-point Likert scale from 0 = “not at all” to 3 = “nearly every day.” The total score ranges from 0 to 27, with higher scores indicating increased symptom severity. We used a cut-off score of 5 and above for depression in Chinese population (43).
The Hamilton Anxiety Scale (HAMA) (44) was used to evaluate anxiety severity, which comprises 14 symptom-defined items. Participants were required to rate their feelings on a basic numeric scoring from 0 (not at all) to 4 (severe). The total scale score ranges from 0 to 56, with a cut-off score of 14 and above indicating anxiety (45), and higher score indicating higher severity.
The Pittsburgh sleep quality index (PSQI) was used to measure sleep quality and sleep patterns in the previous month, which analyzes seven factors: subjective sleep quality; sleep latency; sleep duration; habitual sleep efficiency; sleep disturbances; use of sleeping medications; and daytime dysfunction (46). The questions are rated on a 4-point Likert scale (0–3), with the score for each component then added to give a total score ranging from 0 to 21, with higher scores indicating poorer sleep quality. We created a categorical measure using a cut-off of 6 and above for sleep disturbance (47).
Irritability was measured with 7 items derived from the Neuropsychiatry Inventory (NPI) (48). The items are yes/no questions about behavioral manifestations of irritability. Patients' caregivers were required to rate the frequencies (1–4, 1 = occasionally, 2 = often, 3 = frequently, 4 = very frequently) and severity (1–3, 1 = mild, 2 = moderate, 3 = severe) of the irritability symptoms is. The total score is the frequency score multiplied by the severity score, which ranges from 1 to 12, with higher scores indicating higher severity. This inventory has been validated in Chinese and TBI populations (49, 50).
Chronic headache was rated using a visual analog scale (score ranges from 0 to 100, higher scores indicate higher severity). Global intelligence was measured using the Chinese version of Wechsler Adult Intelligence Scale Revised (WAIS-RC, higher scores indicate higher IQ) (51).
Global neurological disability was evaluated based on the Classification of Severity of Disability by Physical Injuries (National standard issued by the Supreme People's Court, the Supreme People's Procuratorate, the Ministry of Public Security, 2016) criteria. The neurological disability degree ranges from 1 to 10, in which 10 is the lowest and 1 is the highest degree of disability. Multiple aspects are considered in the evaluation; intelligence, memory, concentration, cognition, depression, psychotic symptoms, daily life function, and vocational and social functions; which are similar to the factors in the Glasgow Outcome Scale-Extended (52) and the International Classification of Functioning, Disability, and Health (ICF) (53).
The statistical analyses were performed using IBM SPSS Statistics Version 15 (IBM, Armonk, NY). The means and standard deviations were computed to describe the continuous variables, and frequencies were used to describe the categorical variables. Chi-Square test was used to examine the difference of prevalence of neurological symptoms in subgroups. Shapiro-Wilk test was used to examine the distribution of data. Two-independent sample t-test (normally distributed data) or non-parametric tests (Mann-Whitney U-test for non-normally distributed data) were used to explore the differences in scores of scales and inflammatory biomarkers between the TBI patients with and without neurological symptoms, such as depression, headaches, sleep disturbance, and irritability. Bivariate correlation analysis (Pearson correlation test for continuous data and Spearman correlation test for categorical data) were conducted to explore the correlations between the inflammatory biomarkers and the neurological symptoms, and binary logistic regression analysis was used to identify the risk factors for the neurological symptoms.
Of the 72 patients, nine were unable to complete the IQ test because of cognitive impairment, feelings of discomfort, refusal, and being non-communicative. The average IQ for the remaining 63 patients was 60.2 ± 9.7 (ranging from 33 to 83). The most common symptom was sleep disturbance (84.7%), followed by chronic headache (59.7%), irritability (55.6%), and depression (54.2%). The prevalence of sleep disturbance, headache, and irritability did not differ in female and male patients (P > 0.05). However, the prevalence of depression (85.7% vs. 46.6%) and anxiety (35.7% vs. 6.9%) in females was significantly higher than that in males (P = 0.008). Thirty-nine patients were categorized as depressed, and the mean PHQ score was 12.1 ± 4.0, which indicated a mild to moderate degree of depression. Notably, the chronic headache was the most common symptom complained by the patients; however, the irritability was reported by the caregivers as the most common symptom that caused greatest distress to the family members. Compared to the above symptoms, anxiety (12.5%) was less common. The global neurological disability level for the sample ranged from 4 to 10. The details are given in Table 2.
In the patients with neurological symptoms, males scored significantly higher in chronic headache (p = 0.039) and irritability (p = 0.028) than females, which indicates more severity of these two symptoms in males. Besides, no difference was observed in the severity of other symptoms between males and females (Supplementary Table 1).
According to the result of Shapiro-Wilk test, the concentrations of inflammatory biomarkers were non-normally distributed (P < 0.05). The Mann-Whitney U test showed no male and female differences for all the investigated inflammatory biomarkers (Supplementary Table 2), and no inflammatory biomarker differences were found between the TBI patients with and without the investigated neurological symptoms (Figure 1).
Figure 1. Comparisons of the cytokine levels between TBI-patients with and without related neurological symptom. 1 pg (picogram) = 10−12 g. The X-axis indicates the type of cytokines. The Y-axis indicates the median of the cytokine concentrations, while boxes represent lower quartile, median and upper quartile of the concentrations. Boxplots above the X-axis represent the concentrations of cytokines for patients with related neurological symptoms, while those below the X-axis represent the concentrations of cytokines for patients without related neurological symptom. The concentration of cytokines did not differ significantly between patients with and without each neurological symptom (All P > 0.05).
The results of the bivariate Spearman correlation analysis showed that age was positively associated with IL-5 (r = 0.306, P = 0.009), TNF-α (r = 0.350, P = 0.003), IL-6 (r = 0.263, P = 0.025), sleep disturbance (r = 0.257, P = 0.029), and depression (r = 0.340, P = 0.004), but negatively associated with irritability (r = −0.321, P = 0.006). Considering the effect of age on the symptoms and biomarkers, a partial correlation analysis was conducted to evaluate the associations between the inflammatory biomarkers and the neurological symptoms independent of age. Of the neurological symptoms, chronic headache was positively associated with both depression (r = 0.251, P = 0.035) and irritability (r = 0.250, P = 0.036). Inflammatory biomarkers IL-5, IL-6, and IL-8 were positively associated with sleep disturbance (r = 0.242, P = 0.041; r = 0.309, P = 0.008; r = 0.236, P = 0.046); however, only the association with IL-6 persisted after adjusting the effect of age. The bivariate correlation analysis also revealed that IL-6 was positively associated with depression (r = 0.275, P = 0.019); however, the association was absent after controlling for the age effect. IL-5 and IL12p70 were negatively associated with WAIS IQ (r = −0.237, P = 0.047; r = −0.260, P = 0.028) and the global neurological disability level (r = −0.325, P = 0.006; r = −0.319, P = 0.007) even after adjusting for the age effect. Details are presented in Table 3.
To investigate the risk factors for the neurological symptoms, a binary logistic regression analysis was conducted on the participants with and without specific headache, sleep disturbance, depression, and irritability symptoms. TNF-α was identified as a protective factor for post-TBI chronic headache (OR = 0.473). IQ (OR = 1.041) and headache (OR = 1.024) was a risk factor for depression. Age was a protective factor for irritability (OR = 0.941) and headache was a risk factor (OR = 1.018) (Table 4). More information about the regression results is presented in Supplementary Table 3.
Table 4. Risk and protective factors for neuropsychiatric symptoms post-TBI identified from the binary logistic regression.
This study investigated the serum concentrations of nine inflammatory biomarkers in 72 TBI patients in the post-TBI chronic phase (from 6 months to 2 years) and analyzed the association of these biomarkers with neurological symptoms including depression, chronic headache, sleep disturbance, irritability, anxiety, WAIS IQ, and global neuropsychiatric disability.
Of the 72 TBI patients, the most common symptom was sleep disturbance (84.7%), followed by chronic headache (59.7%), irritability (55.6%), and depression (54.2%). The high prevalence of post-TBI sleep disturbance was in line with the results of a previous review study that reported the sleep disturbances were the most common TBI patient complaints that contributed to morbidity and long-term sequelae (54). A meta-analysis comprising 21 studies reported that about 50% of TBI patients suffered from sleep-wake disturbances (55), and Makley et al. found that up to 68% of patients had sleep disturbances in TBI rehabilitation units (56).
The current study also found that IL-5, IL-6, and IL-8 were positively associated with sleep disturbance; however, only the association with IL-6 persisted after controlling for age, which indicated that a higher circulation IL-6 level was related to more severity of sleep disturbance. Although the association has no causality property, a bidirectional relationship between sleep and inflammation has been verified in TBI patients. IL-6 has been recognized as one of the most important biomarkers for chronic or systemic low-grade inflammation and has the most non-immunological effects on tissues (57). Malik et al. noted in a review study that IL-6 was one of the most common cytokines associated with poor psychological outcomes in a chronic mTBI population (23). Specifically, it has been proposed that IL-6 is the “sleep factor” that mediates sleep continuity and sleep macrostructure (58, 59). Several studies have reported that sleep disturbances can increase systemic IL-6 concentrations (60, 61). For example, Nemeth et al. reported that a reduction in sleep duration and quality led to a significant elevation in IL-6 serum levels (61). Preliminary evidence has also suggested that IL-6 may modify circadian rhythms by activating period gene 1 (Per 1) transcription (62). Furthermore, another study found that sleep can modify immune system functions by inducing changes in the hypothalamus-pituitary-adrenal axis and the sympathetic nervous system (63).
In addition to the aforementioned studies based on non-TBI population, Gottshall et al. also investigated the association between sleep quality and cytokines (IL-10, IL-6, and TNF-α) in the plasma and plasma-derived extracellular vesicles (EVs) in chronic mTBI (64). Same to the current study, they also found that plasma IL-6 was positively correlated with sleep quality; however, this association was absent in their study after adjusting for the effect of age, sex, and BMI. The difference in participants may account for this different result. Gottshall et al. examined the US military servicemembers and veterans who had lifetime mTBI history with an average of 9 years interval post injury; however, the current study was based on Chinese population who suffered moderate to severe TBI 6 months to 2 years ago (the average interval was 334 ± 163 days). In addition, the difference in the age of the study population (the mean age was 40 years in Gottshall et al.'s study and 48 years in the current study) may also account for the difference results. Future studies based on larger sample with higher homogeneity are warranted in verifying the association between IL-6 and sleep quality in TBI patients. In addition, as TBI patients face long-term psychological stress, this may be a critical factor for chronic inflammation and sleep disturbance. Therefore, the interactions between inflammation, the HPA-axis, and sleep disturbance post TBI is worthy of further study. Sleep disturbances after a TBI, particularly in the chronic phase, contribute to an exacerbation of neurocognitive and neurobehavioral deficits and prolong the recovery phase after the injury (65, 66). Therefore, early recognition and intervention may limit the secondary negative TBI effects and improve patient outcomes (67).
Chronic headache was the second most common symptoms in the post-TBI chronic phase, with a prevalence of 59.7%. In line with this, a review study that examined data from more than 23 studies covering 4,200 TBI patients found that 51.5% of TBI patients experienced chronic pain (68). In the current study, persistent headache was the most common symptom complained by the patients, which was reported to significantly influence their daily lives and cause extreme emotional distress. Furthermore, chronic headache was identified as a significant risk factor both for depression and irritability, which highlights the contribution chronic headache makes to emotional disturbance and related cognitive-behavioral problems. This study highlighted that chronic headache may be a mediator for emotional and behavioral problems in TBI patients and should be an important target for post-TBI neurological symptom interventions. In further, the interactions between chronic headache, depression, irritability, and sleep disturbance post-TBI should receive more attention (54).
Although chronic headache is common and appears to play a crucial role in TBI-related disabilities, the exact mechanisms are still unclear. Neuroinflammation has been reported as an important mechanism for chronic headache (12, 14, 69). For example, Irvine et al. found that several neuroinflammation and pain-related cytokines such as TNF-α and IL-1βincreased in the thalamus, hippocampus, substantia nigra, and hypothalamus of TBI patients (12). Aydin et al. reported the serum TNF-α was significant higher in patients with migraine with aura during migraine attacks (70). Perini et al. found that circulating TNF-α levels were higher in patients with migraine studied soon after attacks onset and lower over time (71). The difference in the direction of the association between cytokines and headaches are varied in different studies, because of the difference in the study population, the type of headaches and the time interval between the headache attacks and the sample collection. In the current study, TNF-α was found to be a protective factor for post-TBI chronic headache, which was supported by a number of previous studies. Previous studies have shown that in addition to its pro-inflammatory activity, TNF-α also acts as a neurotrophic factor that is vital for neuroprotection and repair (72, 73). Genetic studies have also found that the function of TNF-α differs in the acute and delayed post-TBI phases. Immediately after the injury, TNF-α seems to act as a potent inflammatory mediator; however, later it has been found to be the neurotrophic factor required for neuroprotection and repair (74). Another study found that when the TNF-α receptor gene was knocked out, post-TBI mice had exacerbated tissue and blood brain barrier (BBB) damage and neurological impairment, suggesting that TNF-α may have neuroprotective effects (75).
Of the 72 TBI participants, 54.2% reported depression in the chronic post-TBI phase. This prevalence was similar to Bombardier et al., which reported that 53.1% of 559 TBI patients met the criteria for a major depressive disorder (MDD) in the first year after the TBI (76). Major depression has been reported to be the most prevalent neuropsychiatric disorder following a TBI, with a period prevalence of 33% to 42% within the first year and 61% in the first 7 years following the injury (77). Review study has reported that the best current evidence suggests that depressed mood in persons with a TBI is characterized more by irritability, anger, and aggression than by sadness and tearfulness (78), which was also verified in the current study. In the current study, irritability was reported as the most common emotional problem that caused the most distressing burden to caregivers. In line with this, Kersel et al. also reported that emotional control problems were the most distressing issue for patients and caregivers at 1 year post-injury in a cohort of severe TBI patients (79). Therefore, neurological rehabilitation for TBI patients should focus greater attention on psychological and neurological management and treatment for irritability.
There are many potential mechanisms to explain post-TBI depression and the related emotional dysregulation, such as brain area and related circuit damage (e.g., pre-frontal, hippocampus, and frontal lobe-basal ganglia circuit) (80), neuroinflammation, axonal degeneration, and psychosocial factors (disruption to family and social relationships, functional impairment, loneliness, loss of employment, and financial worries) (81). An interesting finding in the current study was that IQ was identified as a risk factor for depression. One explanation for this could be that patients with higher IQs have a greater focus on their future, and therefore feel more depressed when confronted with their negative TBI-related consequences. On the contrary, patients with lower IQ may have more severe executive ability impairments and would possibly not pay attention to thinking about their future life and the psychosocial factors. This result highlights that psychosocial factors may play crucial role in the development of depression in TBI patients; therefore, greater attention should be focused on TBI patients with higher IQs and executive functions in preventing depression post TBI.
Some studies have documented an association between inflammation and depression and reported that higher concentrations of pro-inflammatory cytokines possibly directly contribute to the development of depressive symptoms (11, 82, 83). The current study found that the serum IL-6 level was positively associated with depression; however, this association was not significant after controlling for the age effect. Age is positively associated with depression and IL-6, which indicates that older TBI patients are more likely to develop post-injury depression and IL-6 may have a greater impact in older populations. Given the relatively small sample size of the current study, further studies with larger sample sizes and with more subgroups are needed.
IL-5 and IL12p70 were negatively associated with WAIS IQ and the global neurological disability level of the TBI patients, which suggested that IL-5 and IL-12p70 were associated with the global neurological function in the chronic post-TBI phase. IL-12p70 is the active form of IL-12, which is an important type 1 immune cytokines and is mainly produced by macrophages and dendritic cells (84). Several studies have concluded that IL-12p70 is associated with cognitive impairment and neurodegenerative disorders such as Alzheimer's disease (AD) because of its role in the neuro-inflammatory processes (85, 86). Trollor et al. reported that IL-12 contributed to cognitive decline such as reduced processing speed performances in elderly individuals aged 70–90 years in an Australian population (87), and older individuals with mild cognitive impairment have also been found to have higher levels of IL-12 (88). A study in Taiwan also found that the IL12 receptor (β2) gene played a vital role in modulating cognitive aging (89). A meta-analysis study also found significantly higher levels of IL-12 in peripheral blood in AD patients compared with the healthy controls (90). Additionally, a recent study used a machine learning prediction model to assess 242 blood proteins in 80 older adults and found that IL-12 was one of the three proteins that could predict cognitive impairment (91). Papenberg et al. also found that IL-12 was associated with Mini-Mental State Examination (MMSE) test scores and the gray-matter volumes in the lateral prefrontal cortex and hippocampus in older adults (92).
The IL-12 was simultaneously associated with the global neurological disability in the chronic phase after TBI, and the neurogenerative deficits in elder adults, indicating that there may be some common pathogenesis for TBI and elder neurodegenerative deficits. Accumulated epidemiological studies have identified TBI as a risk factor for delayed neurodegenerative disorders including Alzheimer's disease, dementia and chronic traumatic encephalopathy (93–95). However, the underpin pathogenesis for this link is still not clear. Given the emerging studies reported that neuroinflammation may persist for decades after TBI, it may play a critical role in the link between TBI and the delayed neurodegenerative disorders. Preclinical studies have demonstrated that persistent neuroinflammation characterized by microglia activation would reduce neurogenesis and synaptogenesis, and further to reduce cortical dendritic complexity and neuronal connectivity (30, 96, 97), which would contribute to neurological deficits and on-going neurodegenerative processes after TBI (98–100). Moreover, animal studies have showed that microglial inhibition or depletion during chronic phase of experimental TBI could reduce neurodegeneration and neurological deficits, such as cognitive and memory impairments (98, 101), which indicates that neuroinflammation may also serve as a therapeutic target for the rehabilitation in TBI patients. The current study only examined the serum levels of cytokines, and did not investigate the cytokine levels in brain areas. Given that the cytokines could travel across disrupted Blood Brain Barrier (BBB) after TBI (102), future studies could simultaneously measure cytokines in brain areas and blood so as to provide more information about the inflammatory mechanisms of the long-term neurological deficits after TBI.
There were some weaknesses in the current study that need to be considered. First, the sample size was relatively small, which may have limited the statistical ability to detect small differences between the groups. The current study was a preliminary exploration of the association between inflammatory biomarkers and neurological symptoms in the chronic phase of TBI patients. Based on the preliminary evidence for this association, future studies with larger sample sizes are warranted. Second, this study only investigated nine inflammatory markers. further studies are needed to assess a broader array of markers involving oxidative stress and HPA axis, from which a more general and informative picture of the pathogenesis of TBI-related neurological symptoms and the inflammatory mechanisms could be obtained. Third, the current study only compared the inflammation in different TBI subgroups with and without specific neurological symptoms, and investigated the association of inflammatory cytokines with different neurological symptoms. Future studies could include healthy controls and depressive patients without TBI as comparison subgroups, by which we could get a more comprehensive understanding of the inflammation profiles for post-TBI neurological symptoms. Fourth, the menstrual status of female participants was not collected in the current study. Hormonal levels of women in different menstrual status may affect mental and behavioral symptoms and may affect the levels of biomarkers of inflammation, which should be considered as an influencing factor when investigating the association between inflammatory biomarkers and psychiatric symptoms in the future. Last but not least, body mass index (BMI) has been reported to be positively associated with the level of inflammatory biomarkers such as CRP and IL-6 (103), which should be included as a factor in the future studies on inflammation.
Inflammation is a shared neurophysiologic underpinning for TBI and various neurological symptoms, which provides a promising framework to investigate the mechanisms of the persistent neurological disabilities following a TBI. However, few studies have focused on the association between chronic inflammation and neurological symptoms in TBI patients. This is one of the few studies that has focused on chronic cytokine profiles and their relationships with neurological symptoms post-TBI. The results showed that inflammation cytokines were related to sleep disturbance, headaches, and the global neurological function in the post-TBI chronic phase, which adds evidence to the reciprocal relationship between inflammation and neurological symptoms following a TBI. The results highlighted the potential role of anti-inflammatory for the TBI patient's neurological function rehabilitation. The present study is just a preliminary investigation of the association between inflammation and neurological symptoms following a TBI. Given the small sample size and relatively small correlation coefficients, further research with larger sample sizes and more related biomarkers are needed to more thoroughly elucidate this association and the underlying mechanisms.
The original contributions presented in the study are included in the article/Supplementary Material, further inquiries can be directed to the corresponding author/s.
The studies involving human participants were reviewed and approved by Medical Ethical Committee of Sichuan University. The patients/participants provided their written informed consent to participate in this study.
GL, YY, and JH: conceptualization. GL: data analysis, funding acquisition, and writing-original draft. GL, HL, YH, ZH, YL, YG, and JH: data collection and experiments. YY and JH: methodology, supervision, writing-review, and editing. All authors have read and agreed to the published version of the manuscript.
This work was supported by the China Postdoctoral Science Foundation (2018M643488) and the National Natural Science Foundation of China (81901928).
The authors declare that the research was conducted in the absence of any commercial or financial relationships that could be construed as a potential conflict of interest.
All claims expressed in this article are solely those of the authors and do not necessarily represent those of their affiliated organizations, or those of the publisher, the editors and the reviewers. Any product that may be evaluated in this article, or claim that may be made by its manufacturer, is not guaranteed or endorsed by the publisher.
We are much thankful to those who supported this project.
The Supplementary Material for this article can be found online at: https://www.frontiersin.org/articles/10.3389/fpsyt.2022.895852/full#supplementary-material
1. Editorial. Traumatic brain injury-time to end the silence. Lancet Neurol. (2010) 9:331. doi: 10.1016/S1474-4422(10)70069-7
2. Rubiano AM, Carney N, Chesnut R, Puyana JC. Global neurotrauma research challenges and opportunities. Nature. (2015) 527:S193–7. doi: 10.1038/nature16035
3. Wilson L, Stewart W, Dams-O'Connor K, Diaz-Arrastia R, Horton L, Menon DK, et al. The chronic and evolving neurological consequences of traumatic brain injury. Lancet Neurol. (2017) 16:813–25. doi: 10.1016/S1474-4422(17)30279-X
4. Jourdan C, Bayen E, Pradat-Diehl P, Ghout I, Darnoux E, Azerad S, et al. A comprehensive picture of 4-year outcome of severe brain injuries. Results from the Paris-Tbi Study. Ann Phys Rehabil Med. (2016) 59:100–6. doi: 10.1016/j.rehab.2015.10.009
5. Vallat-Azouvi C, Paillat C, Bercovici S, Morin B, Paquereau J, Charanton J, et al. Subjective complaints after acquired brain injury: presentation of the Brain Injury Complaint Questionnaire (BICOQ). J Neurosci Res. (2018) 96:601–11. doi: 10.1002/jnr.24180
6. Das M, Mohapatra S, Mohapatra SS. New perspectives on central and peripheral immune responses to acute traumatic brain injury. J Neuroinflammation. (2012) 9:236. doi: 10.1186/1742-2094-9-236
7. Kim YK, Na KS, Myint AM, Leonard BE. The role of pro-inflammatory cytokines in neuroinflammation, neurogenesis and the neuroendocrine system in major depression. Prog Neuropsychopharmacol Biol Psychiatry. (2016) 64:277–84. doi: 10.1016/j.pnpbp.2015.06.008
8. Miller AH, Raison CL. The role of inflammation in depression: from evolutionary imperative to modern treatment target. Nat Rev Immunol. (2016) 16:22–34. doi: 10.1038/nri.2015.5
9. Krogh J, Benros ME, Jorgensen MB, Vesterager L, Elfving B, Nordentoft M. The association between depressive symptoms, cognitive function, and inflammation in major depression. Brain Behav Immun. (2014) 35:70–6. doi: 10.1016/j.bbi.2013.08.014
10. Anisman H, Hayley S, Turrin N, Merali Z. Cytokines as a stressor: implications for depressive illness. Int J Neuropsychoph. (2002) 5:357–73. doi: 10.1017/S1461145702003097
11. Dowlati Y, Herrmann N, Swardfager W, Liu H, Sham L, Reim EK, et al. A meta-analysis of cytokines in major depression. Biol Psychiatry. (2010) 67:446–57. doi: 10.1016/j.biopsych.2009.09.033
12. Irvine KA, Clark JD. Chronic pain after traumatic brain injury: pathophysiology and pain mechanisms. Pain Med. (2018) 19:1315–33. doi: 10.1093/pm/pnx153
13. Ruff RL, Blake K. Pathophysiological links between traumatic brain injury and post-traumatic headaches. F1000Res. (2016) 5: F1000 Faculty Rev-2116. doi: 10.12688/f1000research.9017.1
14. Kamins J, Charles A. Posttraumatic headache: basic mechanisms and therapeutic targets. Headache. (2018) 58:811–26. doi: 10.1111/head.13312
15. Irwin MR. Sleep and inflammation: partners in sickness and in health. Nat Rev Immunol. (2019) 19:702–15. doi: 10.1038/s41577-019-0190-z
16. Irwin MR. Why sleep is important for health: a psychoneuroimmunology perspective. Ann Rev Psychol. (2015) 66:143–72. doi: 10.1146/annurev-psych-010213-115205
17. Nwachuku EL, Puccio AM, Adeboye A, Chang YF, Kim J, Okonkwo DO. Time course of cerebrospinal fluid inflammatory biomarkers and relationship to 6-month neurologic outcome in adult severe traumatic brain injury. Clin Neurol Neurosurg. (2016) 149:1–5. doi: 10.1016/j.clineuro.2016.06.009
18. Juengst SB, Kumar RG, Failla MD, Goyal A, Wagner AK. Acute inflammatory biomarker profiles predict depression risk following moderate to severe traumatic brain injury. J Head Trauma Rehabil. (2015) 30:207–18. doi: 10.1097/HTR.0000000000000031
19. Kumar RG, Boles JA, Wagner AK. Chronic inflammation after severe traumatic brain injury: characterization and associations with outcome at 6 and 12 months postinjury. J Head Trauma Rehabil. (2015) 30:369–81. doi: 10.1097/HTR.0000000000000067
20. Devoto C, Arcurio L, Fetta J, Ley M, Rodney T, Kanefsky R, et al. Inflammation relates to chronic behavioral and neurological symptoms in military personnel with traumatic brain injuries. Cell Transplant. (2017) 26:1169–77. doi: 10.1177/0963689717714098
21. Juengst SB, Kumar RG, Wagner AK. A narrative literature review of depression following traumatic brain injury: prevalence, impact, and management challenges. Psychol Res Behav Manag. (2017) 10:175–86. doi: 10.2147/PRBM.S113264
22. McKee CA, Lukens JR. Emerging roles for the immune system in traumatic brain injury. Front Immunol. (2016) 7:556. doi: 10.3389/fimmu.2016.00556
23. Malik S, Alnaji O, Malik M, Gambale T, Rathbone MP. Correlation between mild traumatic brain injury-induced inflammatory cytokines and emotional symptom traits: a systematic review. Brain Sci. (2022) 12:102. doi: 10.3390/brainsci12010102
24. Ramlackhansingh AF, Brooks DJ, Greenwood RJ, Bose SK, Turkheimer FE, Kinnunen KM, et al. Inflammation after trauma: microglial activation and traumatic brain injury. Ann Neurol. (2011) 70:374–83. doi: 10.1002/ana.22455
25. Johnson VE, Stewart JE, Begbie FD, Trojanowski JQ, Smith DH, Stewart W. Inflammation and white matter degeneration persist for years after a single traumatic brain injury. Brain. (2013) 136(Pt. 1):28–42. doi: 10.1093/brain/aws322
26. Toyama H, Hatano K, Suzuki H, Ichise M, Momosaki S, Kudo G, et al. In vivo imaging of microglial activation using a peripheral benzodiazepine receptor ligand: [11c]pk-11195 and animal pet following ethanol injury in rat striatum. Ann Nucl Med. (2008) 22:417–24. doi: 10.1007/s12149-008-0136-1
27. Gentleman SM, Leclercq PD, Moyes L, Graham DI, Smith C, Griffin WS, et al. Long-term intracerebral inflammatory response after traumatic brain injury. Forensic Sci Int. (2004) 146:97–104. doi: 10.1016/j.forsciint.2004.06.027
28. Witcher KG, Eiferman DS, Godbout JP. Priming the inflammatory pump of the cns after traumatic brain injury. Trends Neurosci. (2015) 38:609–20. doi: 10.1016/j.tins.2015.08.002
29. Henry RJ, Ritzel R, Barrett JP, Doran S, Jiao Y, Szeto GL, et al. Microglial depletion with csf1r inhibitor during chronic phase of experimental traumatic brain injury reduces neurodegeneration and neurological deficits. Anesth Analg. (2020) 130:1000. doi: 10.1101/791871
30. Witcher KG, Bray CE, Chunchai T, Zhao F, O'Neil SM, Gordillo AJ, et al. Traumatic brain injury causes chronic cortical inflammation and neuronal dysfunction mediated by microglia. J Neurosci. (2021) 41:1597–616. doi: 10.1523/JNEUROSCI.2469-20.2020
31. Manivannan S, Marei O, Elalfy O, Zaben M. Neurogenesis after traumatic brain injury - the complex role of hmgb1 and neuroinflammation. Neuropharmacology. (2021) 183:108400. doi: 10.1016/j.neuropharm.2020.108400
32. Witcher KG, Bray CE, Dziabis JE, McKim DB, Benner BN, Rowe RK, et al. Traumatic brain injury-induced neuronal damage in the somatosensory cortex causes formation of rod-shaped microglia that promote astrogliosis and persistent neuroinflammation. Glia. (2018) 66:2719–36. doi: 10.1002/glia.23523
33. Kondo N, Kuroda T, Kobayashi D. Cytokine networks in the pathogenesis of rheumatoid arthritis. Int J Mol Sci. (2021) 22:10922. doi: 10.3390/ijms222010922
34. Lu H, Wu PF, Zhang W, Liao X. Circulating interleukins and risk of multiple sclerosis: a mendelian randomization study. Front Immunol. (2021) 12:647588. doi: 10.3389/fimmu.2021.647588
35. Aringer M. Inflammatory markers in systemic lupus erythematosus. J Autoimmun. (2020) 110:102374. doi: 10.1016/j.jaut.2019.102374
36. Furman D, Campisi J, Verdin E, Carrera-Bastos P, Targ S, Franceschi C, et al. Chronic inflammation in the etiology of disease across the life span. Nat Med. (2019) 25:1822–32. doi: 10.1038/s41591-019-0675-0
37. Strzelak A, Ratajczak A, Adamiec A, Feleszko W. Tobacco smoke induces and alters immune responses in the lung triggering inflammation, allergy, asthma and other lung diseases: a mechanistic review. Int J Environ Res Public Health. (2018) 15:1033. doi: 10.3390/ijerph15051033
38. Arnson Y, Shoenfeld Y, Amital H. Effects of tobacco smoke on immunity, inflammation and autoimmunity. J Autoimmun. (2010) 34:J258–65. doi: 10.1016/j.jaut.2009.12.003
39. Yue JK, Cnossen MC, Winkler EA, Deng HS, Phelps RRL, Coss NA, et al. Pre-injury comorbidities are associated with functional impairment and post-concussive symptoms at 3-and 6-months after mild traumatic brain injury: a track-tbi study. Front Neurol. (2019) 10:343. doi: 10.3389/fneur.2019.00343
40. Silverberg ND, Gardner AJ, Brubacher JR, Panenka WJ, Li JJ, Iverson GL. Systematic review of multivariable prognostic models for mild traumatic brain injury. J Neurotrauma. (2015) 32:517–26. doi: 10.1089/neu.2014.3600
41. Cassidy JD, Cancelliere C, Carroll LJ, Cote P, Hincapie CA, Holm LW, et al. Systematic review of self-reported prognosis in adults after mild traumatic brain injury: results of the international collaboration on mild traumatic brain injury prognosis. Arch Phys Med Rehabil. (2014) 95:S132–51. doi: 10.1016/j.apmr.2013.08.299
42. Kroenke K, Spitzer RL. The Phq-9: a new depression diagnostic and severity measure. Psychiat Ann. (2002) 32:509–15. doi: 10.3928/0048-5713-20020901-06
43. Zhu C, Wu W, Zhang M. Handbook of Psychiatric Rating Scales. In: Zhang M, He Y, editors. Changsha: Hunan Science and Technology Press (2015) 172.
44. Hamilton M. The assessment of anxiety states by rating. Br J Med Psychol. (1959) 32:50–5. doi: 10.1111/j.2044-8341.1959.tb00467.x
45. Wang X, Wang X, Ma H. Handbook of Mental Health Assessment Scale. In: Wang X, Wang X, Ma H, editors. Beijing: Chinese Journal of Mental Health. (1999) 181.
46. Buysse DJ, Reynolds CF III, Monk TH, Berman SR, Kupfer DJ. The pittsburgh sleep quality index: a new instrument for psychiatric practice and research. Psychiatry Res. (1989) 28:193–213. doi: 10.1016/0165-1781(89)90047-4
47. Okun ML, Kravitz HM, Sowers MF, Moul DE, Buysse DJ, Hall M. Psychometric evaluation of the insomnia symptom questionnaire: a self-report measure to identify chronic insomnia. J Clin Sleep Med. (2009) 5:41–51. doi: 10.5664/jcsm.27391
48. Cummings JL, Mega M, Gray K, Rosenberg-Thompson S, Carusi DA, Gornbein J. The neuropsychiatric inventory: comprehensive assessment of psychopathology in dementia. Neurology. (1994) 44:2308–14. doi: 10.1212/WNL.44.12.2308
49. Wang T, Xiao S, Li X, Wang H, Liu Y, Su N, et al. Reliability and validity of the Chinese version of the neuropsychiatric inventory in mainland china. Int J Geriatr Psychiatry. (2012) 27:539–44. doi: 10.1002/gps.2757
50. Kilmer RP, Demakis GJ, Hammond FM, Grattan KE, Cook JR, Kornev AA. Use of the neuropsychiatric inventory in traumatic brain injury: a pilot investigation. Rehabil Psychol. (2006) 51:232–8. doi: 10.1037/0090-5550.51.3.232
51. Gong Y. Manual for the Wechsler Adult Intelligence Scale: Chinese Revision. Changhu: Hunan Medical College (1982).
52. Sander A. The Extended Glasgow Outcome Scale. The Center for Outcome Measurement in Brain Injury. (2002). Available online at: http://www.tbims.org/combi/gose
53. (WHO) WHO. International Classification of Functioning, Disability and Health (Icf). Geneva: World Health Organization (2001).
54. Wickwire EM, Williams SG, Roth T, Capaldi VF, Jaffe M, Moline M, et al. Sleep, sleep disorders, and mild traumatic brain injury. What we know and what we need to know: findings from a National Working Group. Neurotherapeutics. (2016) 13:403–17. doi: 10.1007/s13311-016-0429-3
55. Mathias JL, Alvaro PK. Prevalence of sleep disturbances, disorders, and problems following traumatic brain injury: a meta-analysis. Sleep Med. (2012) 13:898–905. doi: 10.1016/j.sleep.2012.04.006
56. Makley MJ, English JB, Drubach DA, Kreuz AJ, Celnik PA, Tarwater PM. Prevalence of sleep disturbance in closed head injury patients in a rehabilitation unit. Neurorehabil Neural Repair. (2008) 22:341–7. doi: 10.1177/1545968308315598
57. Papanicolaou DA, Vgontzas AN. Interleukin-6: the endocrine cytokine. J Clin Endocrinol Metab. (2000) 85:1331–3. doi: 10.1210/jcem.85.3.6582
58. Vgontzas AN, Papanicolaou DA, Bixler EO, Lotsikas A, Zachman K, Kales A, et al. Circadian interleukin-6 secretion and quantity and depth of sleep. J Clin Endocrinol Metab. (1999) 84:2603–7. doi: 10.1210/jcem.84.8.5894
59. Vgontzas AN, Bixler EO, Lin HM, Prolo P, Trakada G, Chrousos GP. Il-6 and its circadian secretion in humans. Neuroimmunomodulation. (2005) 12:131–40. doi: 10.1159/000084844
60. Abedelmalek S, Chtourou H, Aloui A, Aouichaoui C, Souissi N, Tabka Z. Effect of time of day and partial sleep deprivation on plasma concentrations of Il-6 during a short-term maximal performance. Eur J Appl Physiol. (2013) 113:241–8. doi: 10.1007/s00421-012-2432-7
61. Vgontzas AN, Zoumakis E, Bixler EO, Lin HM, Follett H, Kales A, et al. Adverse effects of modest sleep restriction on sleepiness, performance, and inflammatory cytokines. J Clin Endocrinol Metab. (2004) 89:2119–26. doi: 10.1210/jc.2003-031562
62. Motzkus D, Albrecht U, Maronde E. The human Per1 gene is inducible by interleukin-6. J Mol Neurosci. (2002) 18:105–9. doi: 10.1385/JMN:18:1-2:105
63. Rico-Rosillo MG, Vega-Robledo GB. Sleep and immune system. Rev Alerg Mex. (2018) 65:160–70. doi: 10.29262/ram.v65i2.359
64. Gottshall JL, Guedes VA, Pucci JU, Brooks D, Watson N, Sheth P, et al. Poor sleep quality is linked to elevated extracellular vesicle-associated inflammatory cytokines in warfighters with chronic mild traumatic brain injuries. Front Pharmacol. (2022) 12:762077. doi: 10.3389/fphar.2021.762077
65. Terrio H, Brenner LA, Ivins BJ, Cho JM, Helmick K, Schwab K, et al. Traumatic brain injury screening: preliminary findings in a us army brigade combat team. J Head Trauma Rehabil. (2009) 24:14–23. doi: 10.1097/HTR.0b013e31819581d8
66. Farrell-Carnahan L, Franke L, Graham C, McNamee S. Subjective sleep disturbance in veterans receiving care in the veterans affairs polytrauma system following blast-related mild traumatic brain injury. Mil Med. (2013) 178:951–6. doi: 10.7205/MILMED-D-13-00037
67. Wolfe LF, Sahni AS, Attarian H. Sleep disorders in traumatic brain injury. Neurorehabilitation. (2018) 43:257–66. doi: 10.3233/NRE-182583
68. Alfano DP. Emotional and pain-related factors in neuropsychological assessment following mild traumatic brain injury. Brain Cogn. (2006) 60:194–6.
69. Mayer CL, Huber BR, Peskind E. Traumatic brain injury, neuroinflammation, and post-traumatic headaches. Headache. (2013) 53:1523–30. doi: 10.1111/head.12173
70. Aydin M, Demir CF, Arikanoglu A, Bulut S, Ilhan N. Plasma cytokine levels in migraineurs during and outside of attacks. Eur J Gen Med. (2015) 12:307–12. doi: 10.15197/ejgm.01255
71. Perini F, D'Andrea G, Galloni E, Pignatelli F, Billo G, Alba S, et al. Plasma cytokine levels in migraineurs and controls. Headache. (2005) 45:926–31. doi: 10.1111/j.1526-4610.2005.05135.x
72. Figiel I. Pro-inflammatory cytokine tnf-alpha as a neuroprotective agent in the brain. Acta Neurobiol Exp. (2008) 68:526–34.
73. Kim M, Jung K, Ko Y, Kim IS, Hwang K, Jang JH, et al. TNF-alpha pretreatment improves the survival and function of transplanted human neural progenitor cells following hypoxic-ischemic brain injury. Cells. (2020) 9:1195. doi: 10.3390/cells9051195
74. Scherbel U, Raghupathi R, Nakamura M, Saatman KE, Trojanowski JQ, Neugebauer E, et al. Differential acute and chronic responses of tumor necrosis factor-deficient mice to experimental brain injury. Proc Natl Acad Sci USA. (1999) 96:8721–6. doi: 10.1073/pnas.96.15.8721
75. Sullivan PG, Bruce-Keller AJ, Rabchevsky AG, Christakos S, Clair DK, Mattson MP, et al. Exacerbation of damage and altered nf-kappab activation in mice lacking tumor necrosis factor receptors after traumatic brain injury. J Neurosci. (1999) 19:6248–56. doi: 10.1523/JNEUROSCI.19-15-06248.1999
76. Bombardier CH, Fann JR, Temkin NR, Esselman PC, Barber J, Dikmen SS. Rates of major depressive disorder and clinical outcomes following traumatic brain injury. JAMA. (2010) 303:1938–45. doi: 10.1001/jama.2010.599
77. Fann JR, Hart T, Schomer KG. Treatment for depression after traumatic brain injury: a systematic review. J Neurotrauma. (2009) 26:2383–402. doi: 10.1089/neu.2009.1091
78. Seel RT, Macciocchi S, Kreutzer JS. Clinical considerations for the diagnosis of major depression after moderate to severe TBI. J Head Trauma Rehab. (2010) 25:99–112. doi: 10.1097/HTR.0b013e3181ce3966
79. Kersel DA, Marsh NV, Havill JH, Sleigh JW. Psychosocial functioning during the year following severe traumatic brain injury. Brain Inj. (2001) 15:683–96. doi: 10.1080/02699050010013662
80. Vattakatuchery JJ, Lathif N, Joy J, Cavanna A, Rickards H. Pharmacological interventions for depression in people with traumatic brain injury: systematic review. J Neurol Neurosur Ps. (2014) 85. doi: 10.1136/jnnp-2014-308883.18
81. Whelan-Goodinson R, Ponsford JL, Schonberger M, Johnston L. Predictors of psychiatric disorders following traumatic brain injury. J Head Trauma Rehab. (2010) 25:320–9. doi: 10.1097/HTR.0b013e3181c8f8e7
82. Toenders YJ, Laskaris L, Davey CG, Berk M, Milaneschi Y, Lamers F, et al. Inflammation and depression in young people: a systematic review and proposed inflammatory pathways. Mol Psychiatry. (2021) 27:315–27. doi: 10.1038/s41380-021-01306-8
83. Han KM, Ham BJ. How inflammation affects the brain in depression: a review of functional and structural Mri studies. J Clin Neurol. (2021) 17:503–15. doi: 10.3988/jcn.2021.17.4.503
84. Zhang S, Wang Q. Factors determining the formation and release of bioactive Il-12: regulatory mechanisms for Il-12p70 synthesis and inhibition. Biochem Biophys Res Commun. (2008) 372:509–12. doi: 10.1016/j.bbrc.2008.05.081
85. Griffin WS. Neuroinflammatory cytokine signaling and Alzheimer's disease. N Engl J Med. (2013) 368:770–1. doi: 10.1056/NEJMcibr1214546
86. Lee DR, Kwon CH, Park JY, Kim YK, Woo JS. 15-Deoxy-Δ12,14-prostaglandin J2 induces mitochondrial-dependent apoptosis through inhibition of Pka/Nf-?b in renal proximal epithelial cells. Toxicology. (2009) 258:17–24. doi: 10.1016/j.tox.2009.01.001
87. Trollor JN, Smith E, Agars E, Kuan SA, Baune BT, Campbell L, et al. The association between systemic inflammation and cognitive performance in the elderly: the Sydney memory and ageing study. Age. (2012) 34:1295–308. doi: 10.1007/s11357-011-9301-x
88. Trollor JN, Smith E, Baune BT, Kochan NA, Campbell L, Samaras K, et al. Systemic inflammation is associated with mci and its subtypes: the Sydney memory and aging study. Dement Geriatr Cogn. (2010) 30:569–78. doi: 10.1159/000322092
89. Lin E, Kuo PH, Liu YL, Yang AC, Tsai SJ. Association and interaction effects of interleukin-12 related genes and physical activity on cognitive aging in old adults in the taiwanese population. Front Neurol. (2019) 10:1065. doi: 10.3389/fneur.2019.01065
90. Swardfager W, Lanctot K, Rothenburg L, Wong A, Cappell J, Herrmann N. A meta-analysis of cytokines in Alzheimer's disease. Biol Psychiat. (2010) 68:930–41. doi: 10.1016/j.biopsych.2010.06.012
91. Diniz BS, Sibille E, Ding Y, Tseng G, Aizenstein HJ, Lotrich F, et al. Plasma biosignature and brain pathology related to persistent cognitive impairment in late-life depression. Mol Psychiatr. (2015) 20:594–601. doi: 10.1038/mp.2014.76
92. Papenberg G, Ferencz B, Mangialasche F, Mecocci P, Cecchetti R, Kalpouzos G, et al. Physical activity and inflammation: effects on gray-matter volume and cognitive decline in aging. Hum Brain Mapp. (2016) 37:3462–73. doi: 10.1002/hbm.23252
93. Stocchetti N, Zanier ER. Chronic impact of traumatic brain injury on outcome and quality of life: a narrative review. Crit Care. (2016) 20:148. doi: 10.1186/s13054-016-1318-1
94. Smith DH, Johnson VE, Stewart W. Chronic neuropathologies of single and repetitive TBI: substrates of dementia? Nat Rev Neurol. (2013) 9:211–21. doi: 10.1038/nrneurol.2013.29
95. Fleminger S, Oliver DL, Lovestone S, Rabe-Hesketh S, Giora A. Head injury as a risk factor for Alzheimer's disease: the evidence 10 years on; a partial replication. J Neurol Neurosur Ps. (2003) 74:857–62. doi: 10.1136/jnnp.74.7.857
96. Simon DW, McGeachy M, Bayir H, Clark RSB, Loane DJ, Kochanek PM. Neuroinflammation in the evolution of secondary injury, repair, and chronic neurodegeneration after traumatic brain injury. Nat Rev Neurol. (2017) 13:171–91. doi: 10.1038/nrneurol.2017.13
97. Smitha JA, Dasa A, Rayb SK, Banika NL. Role of pro-inflammatory cytokines released from microglia in neurodegenerative disease. Brain Res Bull. (2012) 87:10–20. doi: 10.1016/j.brainresbull.2011.10.004
98. Cho HJ, Sajja VS, Vandevord PJ, Lee YW. Blast induces oxidative stress, inflammation, neuronal loss and subsequent short-term memory impairment in rats. Neuroscience. (2013) 253:9–20. doi: 10.1016/j.neuroscience.2013.08.037
99. Zafonte RD. Traumatic brain injury: an enduring challenge. Lancet Neurol. (2017) 16:766–8. doi: 10.1016/S1474-4422(17)30300-9
100. Loane DJ, Kumar A, Stoica BA, Cabatbat R, Faden AI. Progressive neurodegeneration after experimental brain trauma: association with chronic microglial activation. J Neuropathol Exp Neurol. (2014) 73:14–29. doi: 10.1097/NEN.0000000000000021
101. Kumar A, Loane DJ. Neuroinflammation after traumatic brain injury: opportunities for therapeutic intervention. Brain Behav Immun. (2012) 26:1191–201. doi: 10.1016/j.bbi.2012.06.008
102. van Vliet EA, Ndode-Ekane XE, Lehto LJ, Gorter JA, Andrade P, Aronica E, et al. Long-lasting blood-brain barrier dysfunction and neuroinflammation after traumatic brain injury. Neurobiol Dis. (2020) 145:105080. doi: 10.1016/j.nbd.2020.105080
Keywords: traumatic brain injury, interleukin, chronic inflammation, neuropsychiatric disability, sleep disorder, headache
Citation: Li G, Liu H, He Y, Hu Z, Gu Y, Li Y, Ye Y and Hu J (2022) Neurological Symptoms and Their Associations With Inflammatory Biomarkers in the Chronic Phase Following Traumatic Brain Injuries. Front. Psychiatry 13:895852. doi: 10.3389/fpsyt.2022.895852
Received: 14 March 2022; Accepted: 31 May 2022;
Published: 24 June 2022.
Edited by:
Antonia Manduca, Roma Tre University, ItalyReviewed by:
Alessandro Rava, Roma Tre University, ItalyCopyright © 2022 Li, Liu, He, Hu, Gu, Li, Ye and Hu. This is an open-access article distributed under the terms of the Creative Commons Attribution License (CC BY). The use, distribution or reproduction in other forums is permitted, provided the original author(s) and the copyright owner(s) are credited and that the original publication in this journal is cited, in accordance with accepted academic practice. No use, distribution or reproduction is permitted which does not comply with these terms.
*Correspondence: Yi Ye, eWV5aUBzY3UuZWR1LmNu; Junmei Hu, aHVqdW5tZWlAc2N1LmVkdS5jbg==
Disclaimer: All claims expressed in this article are solely those of the authors and do not necessarily represent those of their affiliated organizations, or those of the publisher, the editors and the reviewers. Any product that may be evaluated in this article or claim that may be made by its manufacturer is not guaranteed or endorsed by the publisher.
Research integrity at Frontiers
Learn more about the work of our research integrity team to safeguard the quality of each article we publish.