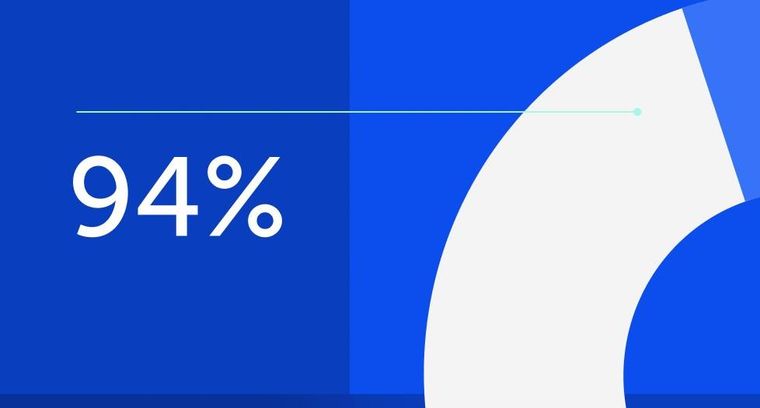
94% of researchers rate our articles as excellent or good
Learn more about the work of our research integrity team to safeguard the quality of each article we publish.
Find out more
ORIGINAL RESEARCH article
Front. Psychiatry, 19 May 2022
Sec. Addictive Disorders
Volume 13 - 2022 | https://doi.org/10.3389/fpsyt.2022.891811
This article is part of the Research TopicMethods and Applications in Addiction Psychiatry Research: 2021View all 13 articles
Due to differences in potency, efficacy, and affinity for CB1 receptors, similarities and differences in psychoactive effect profiles of natural cannabis and synthetic cannabinoids (SCs) cannot reliably be derived from equipotent dose comparisons. Instead, the current study proposes to compare the intrinsic psychoactive effects of natural cannabis (THC) and an SC, JWH-018, at psychotropic dose equivalence. Participants from two placebo-controlled studies were matched for their levels of subjective high to compare neurocognitive and psychotomimetic effects of THC and JWH-018. At equal subjective intoxication levels, both drugs impaired psychomotor, divided attention, and impulse control, with no significant difference between the two drugs. Both drugs also caused significant psychotomimetic effects, but dissociative effects were considerably more pronounced for JWH-018 than THC. We conclude that psychotropic dose equivalence provides a uniform approach for comparing the neurocognitive and psychotomimetic profiles of CB1 agonists, which can also be applied to other drug classes.
The availability of novel psychoactive substances (NPS), which mimic the effects of traditional drugs of abuse, has increased rapidly over the past decades (1). NPS include diverse classes of substances, such as synthetic cannabinoids, synthetic cathinones, phenethylamines, piperazines, tryptamines, aminoindanes, and NPS opioids (2). Although NPS use is less common than the use of other illicit drugs (3), the harm caused to individuals can be quite serious, ranging from acute impairment and adverse effects, to drug addiction (1).
One class of NPS, synthetic cannabinoids (SCs), includes compounds initially designed since the 1970's by researchers to investigate the cannabinoid system and to explore new therapeutic indications, e.g., pain disorders and cancer (4, 5). However, in the early 2000s, these compounds (e.g., JWH-018) appeared in smoking mixtures, such as Spice or K2, which were advertised as “natural” alternatives for cannabis. These smoking mixtures became popular rapidly, especially in countries where recreational use of cannabis was illegal or in situations where users wanted to avoid detection in routine drug screening (6). However, it also became clear from anecdotal reports that these SCs come with serious side effects, such as agitation, psychotomimetic effects, and cardiac events (7–10), as well as fatalities (11, 12). As a result, more and more of these SCs were added to the list of controlled substances in progressively more countries. In response to these bans, the content of the smoking mixtures has changed continuously, with new and more potent SCs being released on the market at an increasing speed (13–15).
SCs bind to the central cannabinoid receptors (CB1 and CB2), which are also the target receptors for Δ9-tetrahydrocannabinol (THC), the main psychoactive component of cannabis. However, the effects elicited by most SCs are more powerful than the effects of natural cannabis (16). The risk of emergency medical treatment is about 30 times greater following the use of SCs than following cannabis (17), with tachycardia, agitation, and nausea as the most frequently reported adverse events (8). Cases of SC intoxication often display cognitive impairment (16). Compared to natural cannabis users and non-cannabis users, frequent SC users were found to perform worse on cognitive tasks, including working memory, inhibition, and long-term memory (18). The most well-known adverse effects linked to SCs are probably psychological symptoms, including agitation, anxiety, and psychosis (16, 19). Psychotomimetic and dissociative effects, often referred to as “zombie effect,” have been noted in up to 28% of the people who admitted using an SC (20, 21). Although the symptoms of SCs overlap with those of cannabis, the stronger and more unpredictable effects of SCs cause the majority of users to express a preference for natural cannabis (22).
Most SCs have a specifically high affinity for CB1 (16, 23–26). Furthermore, they act as agonists with high efficacy (i.e., act as a full agonist) (23, 24, 27). This is in contrast to THC, which has low efficacy, low affinity for CB1, and is less potent, and thus is not able to stimulate cannabinoid receptors to the same degree as SCs (24, 28). Consequently, the behavioral effects of a given dose of an SC are not simply comparable to those of an equivalent dose of THC. In pioneering studies conducted in our lab with an early SC, JWH-018, we demonstrated that a low dose produced significant psychomotor and cognitive impairment, as well as psychotomimetic symptoms (29–32) even at subjective intoxication levels that were lower than what is normally reported for natural cannabis. It seems therefore appropriate to take the level of subjective intoxication into account when establishing the psychotropic dose equivalence between an SC and natural cannabis.
In the present manuscript, we defined psychotropic dose equivalence as the dose at which an identical level of subjective high is achieved with an SC and natural cannabis. Subsequently, we aimed to compare neurocognitive and psychomimetic effects of an SC (i.e., JWH-018) and cannabis in participants from two previous placebo controlled studies (31–34) that were matched for their levels of subjective high. We expected that a comparison at psychotropic dose equivalence would allow for an objective comparison of the neurocognitive and psychotomimetic profiles of JWH-018 and THC.
Subjective and performance data analyzed in this study comes from two studies with a comparable design and setup (31–34). Both studies were placebo-controlled, cross-over studies examining the acute effect of either cannabis (THC) or JWH-018 in healthy cannabis users. The type of cannabis users was comparable in that both studies included occasional users (i.e., cannabis use between 8 and 120 times/year). The studies used the same test battery and questionnaires to measure cognitive and subjective effects that were administered within a similar time window relative to drug dosing. A summary of the subjective and objective tests applied in both studies, and at which time after dosing they were administered, is provided in Figure 1.
Figure 1. Timeline of subjective questionnaires and cognitive tests relative to time of drug administration.
THC data was taken from a previous study from our group (33, 34). In this randomized, double-blind experimental study, 122 cannabis-experienced (at least 8 times/year) participants received 450 μg/kg THC (cannabis plant material, divided into two doses of 300 and 150 μg/kg; i.e., 21 and 11 mg for a 70 kg person), cocaine, and placebo on three separate test days. Cannabis treatment was split into two doses, with the second one given 1 h after the first dose. Cannabis was prepared from batches containing 11–12% THC, while placebo cannabis consisted of Knaster Hemp. Cannabis and placebo were administered using a vaporizer (Volcano) which heated the materials to a temperature of about 225 °C, while storing the vapor in a polythene bag equipped with a valved mouthpiece. Subjects were instructed to inhale according to a standardized manner, inhaling deeply and holding their breath for 10 s after each inhalation. The bag had to be emptied completely, taking about 2–3 min. Subjective and performance measures were taken within 3.5 h after the first administration (see Figure 1). The cocaine condition has been omitted from the current study.
Subjective and performance data after an acute dose of JWH-018 was taken from a previous study performed by our group (31, 32). In this placebo-controlled, cross-over study, 24 healthy occasional cannabis users inhaled the vapor of 75 μg JWH-018/kg body weight and placebo on two separate test days (i.e., 5.25 mg for a 70 kg person). Placebo consisted of Knaster Hemp (Zentauri, Germany), a herbal blend with hemp aroma (0% THC). Both treatments were administered via a vaporizer pen, which heated the materials to ~380°C. Participants inhaled the vapor in five intakes, according to a strict inhalation regimen. A booster dose of 50 μg JWH-018/kg body weight was administered in case participants did not show a subjective response (i.e., a minimum subjective high score of 30% was required) within 15 min after administration of JWH-018. Performance and subjective measures were taken in the 4 h following drug administration (or booster in case needed) (Figure 1). Subjective intoxication was measured regularly after JWH-018 treatment but was maximal at 30 min post drug.
Subjective high was self-rated on a 10 cm Visual Analog Scale (VAS), with 0 indicating “not high at all,” and 10 indicating “extremely high.” Participants reported their highest scores within the first hour after administration for both THC and JWH-018, followed by a return to baseline levels in the following hours (32, 35, 36). In the THC study, subjective high score was determined 5 min after the first administration, when subjective intoxication for THC was maximal in occasional users (36). In the JWH-018 study, subjective intoxication was maximal at 30 min post drug.
To ensure comparable cannabis use patterns across the samples, only participants who used cannabis a maximum of 10 times/month were included from the THC and JWH-018 study. Subsequently, participants with a comparable subjective high score after THC or JWH-018 were selected (max, mean, SD difference was 0.8, 0.28, and 0.36). This resulted in 24 participants of the THC sample who could be matched to the participants in the JWH-018 sample. The characteristics of both samples are shown in Table 1.
The CTT is a psychomotor test that assesses the participant's ability to control a displayed error signal in a first-order compensatory tracking task (37). Error is displayed as a horizontal deviation of a cursor from the midpoint on a horizontal, linear scale. Compensatory joystick movements null the error by returning the cursor to the midpoint. Total duration of the task is ~3 min. The frequency at which the participant loses control is the critical frequency or lambda-c (λc). The test included five trials, of which the lowest and the highest score were removed; the average of the remaining scores is taken as the final CTT-score. This test has repeatedly been shown to be sensitive to the effects of many sedative drugs, including cannabis (36, 38–40).
The DAT measures the ability to divide attention between two tasks performed simultaneously (41). Participants have to perform the same tracking task as described above, but now at a constant difficulty level. As a secondary task, the subject monitors 24 single digits presented in the corners of the computer screen. The participants have to react to the target number “2” by removing their foot as fast as possible from a pedal switch. Duration of the task is 12 min. The mean absolute tracking error (in mm) and the number of control losses are the performance measures of the primary task. The number of misses, false alarms, and mean reaction time (msec) of the responses to the target number, are the performance measures in the secondary task. Performance in this test has proven to be sensitive to the effects of many sedative drugs (36, 38–40).
The SST measures motor impulsivity, which is the inability to inhibit an activated or pre-cued response leading to errors of commission. The current test is adapted from an earlier version (42) and has been validated for stimulant and sedative drug effects (43). The task requires participants to make quick responses to visual go-signals and to inhibit their response if a subsequent visual stop-signal, i.e., “*”, appears in one of the four corners of the screen. Total task duration is ~8 min. Dependent variables are go reaction time (ms), stop reaction time, number of correct responses, omission (not responding on go-trials), and commission errors (not inhibiting a response to a no go trial). Stop reaction time represents the estimated mean time required to inhibit a response. Stop reaction time is calculated by subtracting the stop signal delay from the reaction time on go-trials associated with nth percentile of the reaction time (RT) distribution (44).
The MFFT measures reflection impulsivity, which is the tendency to reflect on the validity of problem-solving under the particular condition of several possible alternatives. The test involves simultaneous presentation of a target figure positioned on the left of the screen and an array of six alternatives on the right half of the screen, all except one differing in one or more details from the target figure. The participants are asked to select from the alternatives the figure that exactly matches the target figure as quickly as possible. Task duration is ~5 min. Two dependent measures, mean latency to first response (ms) and the total number of errors, are automatically recorded. In addition, an impulsivity score (I-score) is calculated by subtracting the standard score of the mean latency to the first response from the standard score of the total number of errors committed. An efficiency score (E-score) is calculated by summing the standard score of the mean latency to the first response with the standard score of the total number of errors committed.
The Clinician Administered Dissociative States Scale (CADSS) (45) comprises of 19 self-rated items, ranging from 0 “not at all” to 4 “extremely.” It is divided into three components: depersonalisation (5 items), derealisation (12 items), and amnesia (2 items). A total dissociative score is achieved by summing all items. The CADSS is designed to be a standardized measure of present-state dissociative symptomatology and was previously found to be sensitive to dissociative effects of psychedelics and drugs of abuse, such as ketamine and THC (46–48).
Psychedelic symptoms are assessed using a 13-item VAS (49). Two scales measure subjective “high” and “drowsiness.” From the other scales, composite scores of “internal perception” (reflecting inner feelings that do not correspond with reality) and “external perception” (reflecting a misperception of an external stimulus or a change in the awareness of the subject's surroundings) are calculated (50).
The POMS is a self-assessment mood questionnaire with 72 items, rated on a 5-point Likert scale, with 0 being “not at all” to 4 “extremely.” Participants have to indicate to what extent these items were representative of their mood at that moment. Eight mood states are classified and quantified by calculating the sum score of associated items for each mood state, i.e., anxiety, depression, anger, vigor, fatigue, confusion, friendliness, and elation. Two composite scales are derived; arousal and positive mood (51).
Participants for both studies were recruited via advertisements. Data collection in the THC study was part of a more extensive study that included fMRI scanning after the booster dose. Participants of both studies were only included if they had prior experience with cannabis. They were medically examined by a physician, who checked for general health and took blood and urine samples for standard chemistry and hematology. Both studies were approved by the standing Medical Ethics Committee of Maastricht University and were carried out in compliance with the revision of the Declaration of Helsinki applicable at that time (i.e., Seoul, 2008; Fortaleza, 2013) and the International Conference on Harmonization guidelines for Good Clinical Practice. A permit for obtaining, storing, and administering cannabis and JWH-018 was obtained from the Dutch drug enforcement administration. All participants gave written informed consent and received financial compensation for their participation. All tests were taken after acute administration of the drug (see Figure 1).
A student's t-test was used to compare differences in monthly cannabis use, while chi-square test was used to compare distributions of sexes between the two studies.
Outcome data that was normally distributed was analyzed using GLM Repeated Measures ANOVA, with Drug (placebo and drug condition (i.e., THC or JWH-018) as within-subject factor and Study (THC and JWH-018 study) as between-subject factor. Partial eta squared (partial η2) is reported to demonstrate the effect's magnitude and is based on Cohen's f, which defines small, medium, and large effect sizes as, respectively 0.10, 0.25, and 0.40, which corresponds to partial η2 values of 0.01, 0.06, and 0.14 (52). Subsequently, difference scores (THC/JWH-018 minus placebo) were analyzed with pairwise comparisons with a t-test for independent samples.
Non-normal distributed data was analyzed with the non-parametric Wilcoxon signed-rank test, to test for significant differences between the drug condition (THC or JWH-018) and placebo. Difference scores (THC or JWH-018–placebo) were tested with a Mann-Whitney test, to assess for differences between THC and JWH-018. A p-value of <0.05 was considered statistically significant. The effect size (r) for non-parametric tests is calculated by dividing the Z-score by the square root of N, indicating a large effect size using Cohen's d criteria of 0.10, 0.30, and 0.50 for defining small, medium, and large effect sizes. All statistical tests were conducted using IBM SPSS statistics, version 26.
There were several missing data due to technical malfunctioning or the participant not being capable of performing the test. For the THC study, CTT data from one participant in the THC condition was missing, as was DAT data from three participants in the placebo and three other participants in the THC condition. In the JWH-018 study, the following was missing: CTT-score for one participant in the JWH-018 condition; DAT for one participant in the placebo and one participant in the JWH-018 condition; SST data for one participant in the JWH-018 condition, and MFFT data for one participant in the placebo and one participant in the JWH-018 condition. Missing data of these participants were replaced by the study and condition's average before entering the RM ANOVA analysis.
Participants in both groups used cannabis <10 times/month, nevertheless, the average consumption in the groups was statistically different [t(46) = 3.17, p < 0.01]. Also, the division of sexes was significantly different according to a Chi-square test [X 2(1, N = 48) = 8.89, p < 0.01].
Individual subjective high scores per treatment condition are presented in Figure 2. Mean subjective high score was 6.46 after THC and 6.41 after JWH-018 administration. Subjective high score demonstrated a significant effect of Drug [F(1, 46) = 329.1; p < 0.001, = 0.88]. No significant effect of Study or Drug x Study was found, confirming that subjective high in both samples was comparable.
Figure 2. Scatter plot with mean and range of subjective high scores in placebo and drug conditions in both samples.
GLM Repeated measures ANOVA showed a significant effect of Drug and Study on CTT score [F(1, 46) = 9.8; p = 0.003, = 0.18; F(1, 46) = 8.88; p = 0.005, = 0.16], indicating that drug conditions impaired performance (Figure 3A), as compared to placebo. Subsequent t-test analysis on the difference scores showed no significant difference between the change in CTT scores caused by THC or JWH-018 (Figure 3B).
Figure 3. (A) Mean (SEM) values for lambda-c in the critical tracking task, and tracking error and reaction time in the divided attention task for placebo and drug conditions, in the THC and JWH-018 study. (B) Lambda-c in the critical tracking task, and Tracking error and reaction time in the divided attention task for both drug conditions shown as difference scores (relative to placebo). *Significant difference between the active condition (THC/JWH-018) and placebo.
GLM Repeated measures ANOVA showed a significant effect of Drug on tracking error [F(1, 46) = 17.66; p < 0.01, = 0.27] and RT [F(1, 46) = 5.25; p = 0.027, = 0.10]. Tracking error and RT increased after the drug conditions (Figure 3A). T-test analysis showed that the change in tracking error and RT did not differ significantly between THC and JWH-018 (Figure 3B).
Control losses and false alarms were analyzed with the non-parametric Wilcoxon signed-rank test, which showed that these were significantly higher after drug conditions compared to the placebo conditions (Z = −4.83; p < 0.01; r = 0.49 and Z = −4.14; p < 0.01; r = −0.42). The change in number of control losses and false alarms was not different between THC and JWH-018, according to Mann-Whitney tests.
Non-parametric testing showed that the number of omission and commission errors increased (Z = −2.50; p = 0.012; r = −0.25 and Z = −2.68; p=0.007; r = −0.27) in the drug conditions compared to placebo (Figure 4A). No effect was found on Stop or Go reaction time. Paired comparison of change scores from placebo demonstrated that the changes in number of omission and commission errors did not differ significantly between THC and JWH-018 (Figure 4B).
Figure 4. (A) Mean (SEM) number of omission and commission errors in the stop signal task and E-score in the matching familiar figures test for placebo and drug conditions, in the THC and JWH-018 study. *Significant difference between the active condition (THC/JWH-018) and placebo. (B) E-score in the matching familiar figures test for both drug conditions shown as difference scores (relative to placebo).
GLM Repeated measures ANOVA demonstrated a significant effect of Study on Latency (F(1, 46) = 9.27; p = 0.004) with participants in the JWH-018 study showing slower responses. GLM did not show an effect on I-score.
Non-normally distributed E-score and errors were analyzed using Wilcoxon signed-rank test, and this showed that the E-score significantly decreased after the drug conditions compared to placebo (Z = −2.17; p = 0.03; r = −0.22) (Figure 4A). No significant difference was found in the number of errors. Pairwise comparison of change scores from placebo indicated no significant difference on E-scores between THC and JWH-018 (Figure 4B).
All scales of the POMS were analyzed using non-parametric testing. Wilcoxon signed-rank test showed that all scales of the POMS, except anger, were significantly different in the drug conditions compared to placebo (see Supplementary Material for Z and p-values). While scores on the anxiety, depression, fatigue, confusion scale increased, scores on vigor, friendliness, elation, arousal, and positive mood decreased after treatment (Figure 5A).
Figure 5. (A) Mean (SEM) scores on the POMS scales vigor, friendliness, elation, arousal, and positive mood, for placebo and drug conditions, in the THC and JWH-018 study. *Significant difference between the active condition (THC/JWH-018) and placebo. (B) Scores on the POMS scales vigor, friendliness, elation, arousal, and positive mood for both drug conditions shown as difference scores (relative to placebo). *Significant differences between THC and JWH-018.
Pairwise comparison showed that scores on vigor, friendliness, elation, and positive mood were significantly different between THC and JWH-018 (see Supplementary Material for U and p-values) (Figure 5B).
All scales of the CADSS were analyzed non-parametrically, and responses were significantly higher in the drug conditions than in placebo (see Supplementary Material for Z and p-values) (Figure 6A). Pairwise comparison of change scores from placebo demonstrated that the scores in the JWH-018 condition were significantly higher than in the THC condition (see Supplementary Material for U and p-values) (Figure 6B).
Figure 6. (A) Mean (SEM) scores on the CADSS derealisation, depersonalisation amnesia, and total score for placebo and drug conditions, in the THC and JWH-018 study. *Significant difference between the drug condition (THC or JWH-018) and placebo. (B) scores on the CADSS derealisation, depersonalisation amnesia, and total score for both drug conditions shown as difference scores (relative to placebo); significant differences between THC and JWH-018.
Wilcoxon signed-rank tests showed that all scales of the Bowdle were significantly increased after drug treatment compared to placebo (see Supplementary Material for Z and p-values). Pairwise comparison of change scores from placebo showed that there was no difference in the scores on the Bowdle scales between THC and JWH-018.
Although SCs act on the same receptors as THC, it is not straightforward to compare these compounds based on equipotent doses due to the additional differences in affinity, and efficacy. Instead, in this study, the effects of an SC were compared with cannabis (THC), based on psychotropic dose equivalence as represented by subjective intoxication levels. We selected a group of occasional cannabis users who took part in a large THC study and, based on their acute subjective intoxication score, matched these to a group of participants who took part in a JWH-018 study. The design and measures used in both studies were similar, making these datasets ideal for conducting this comparative analysis.
When using equipotent doses in our earlier study [i.e., with JWH-018 being 4–5 times as potent as THC, a dose 5 times as low as a standard THC dose was selected for JWH-018 (i.e., 3 mg JWH-018)], levels of subjective intoxication produced by JWH-018 were much lower than what has been shown previously for typical THC doses (29). In the current study, using psychotropic dose equivalence, i.e., at similar levels of subjective intoxication after both cannabinoids (i.e., 6.46 and 6.41 cm), comparable effects were demonstrated on cognitive performance tests. Both drugs impaired performance on psychomotor, divided attention, and impulsivity tasks with similar magnitudes. Likewise, no significant differences between THC and JWH-018-induced levels of cognitive impairment were found. Subjective questionnaires demonstrated that both drugs caused significant psychotomimetic effects but that the dissociative effects were considerably stronger for JWH-018 than for THC, while positive mood states were more affected by THC. Psychotomimetic effects of JWH-018 at psychotropic dose equivalence in the present study were higher than those observed at equipotent dose equivalence in a previous study (30), which underscores the relevance of distinguishing between these comparative approaches.
Dissociative symptoms, known to occur in psychiatric disorders such as schizophrenia, have been reported during cannabis intoxication (53), and the link between the use of cannabis and psychosis has been reported recurrently (54–58). The risk of an adverse psychotomimetic experience after cannabis use is dose-related, with higher doses increasing the risk (59–61). The current finding that synthetic cannabinoids induce stronger psychotomimetic effects is in line with the many case reports and epidemiological data showing SCs to elicit psychotomimetic effects in vulnerable populations or patients with schizophrenia and healthy individuals (19, 55, 56, 62–64). The lack of cannabidiol (CBD), the non-psychoactive constituent of cannabis, might explain the increased risk of experiencing psychotomimetic effects after SC use. CBD, which is present in different ratios in natural cannabis, has been found to protect against THC's psychotic effect (65–69). It has also been suggested that, due to the higher efficacy, SCs alter the function of the neurotransmitter systems involved in schizophrenia, such as dopamine and glutamate, to a considerable extent (70). In addition, SCs yield metabolites with partial to full agonist activity (24), which again can interact with these neurotransmitter systems, leading to an increased effect. For THC, acute administration has been found to increase glutamate and dopamine levels (71), and increases in striatal glutamate levels were found to underlie the acute psychotomimetic effects of intravenously administered THC (72). It can be hypothesized that the association between striatal glutamate and psychotomimetic symptoms is even more prominent for SC due to their higher levels of efficacy. Studies into the effect of SCs on the neurotransmitter systems involved in schizophrenia are needed to determine the role of these systems in explaining the strong psychotomimetic effects compared to natural cannabis.
As in our previous studies (32, 34), the current analysis demonstrated that drug treatment (THC and JWH-018) affected mood states, with the negative states (except anger) increasing and the positive states decreasing. The decrease in friendliness, vigor, elation, and positive mood was apparent after THC, while both drugs decreased arousal. The effects of THC on mood have not been consistent in previous studies. Some studies showed no effect of THC on mood (73–75), while others demonstrated negative effects (76–78), whereas a more recent study showed elevated scores on positive states (79, 80). These inconsistencies in the effect of THC on mood can possibly be explained by essential differences in study designs, such as differences in dose, route of administration, time of assessment after dosing, and participants' drug use history. SCs users also often report negative mood changes (81) when intoxicated. This negative effect on mood was confirmed for JWH-018 in the current comparative analysis and in the previous studies (30, 32). However, in the current study, the changes in mood after JWH-018 appeared to be less prominent than after THC. This could be due to baseline differences in mood states, which were incorporated in the previous JWH-018 study (30, 32). However, no baseline measurement of mood was taken in the THC-study, hence no correction for baseline differences could be applied in the current study.
Participants in the current study were matched on subjective intoxication levels to accomplish psychotropic dose equivalence. However, there were significant differences in the average monthly consumption of cannabis and the males/females ratio in the two groups (83.3% males in the THC study vs. 41.7% in the JWH-018 study). With respect to cannabis use history, an earlier study comparing a large sample of infrequent and daily cannabis users demonstrated that the acute effects on neurocognitive performance were similar across users irrespective of their cannabis use history (33). With the current participants' use history falling in a much narrower range of cannabis use (i.e., excluding using more than 3 times/week), it is unlikely that this small difference in cannabis use history might have contributed to different findings for THC and JWH-018. With regard to sexes, there have been suggestions that women are more sensitive to the effects of THC than men (82). However, several studies were unable to confirm this, showing no differences between men and women in the acute effect of THC on neurocognitive function (80, 83). In the current study, it is also unlikely that sex differences significantly affected the study results. Men were overly represented in the THC study, but nevertheless, the effects of THC were apparent on a wide range of measures. Overall, by using the subjective high scores as the matching factor between our groups, potential confounding of differences in sex and cannabis use history between participants of the two studies were eliminated.
The proposed method of comparing drugs at psychotropic dose equivalence can also be applied to other drugs of abuse. Especially with the large number of NPS entering the market since the early 2000s, it has been challenging to determine the risks associated with these new compounds. The EMCDDA (84) has, nevertheless, recommended that individual health risks of NPS be assessed in pharmacological studies in humans and include psychological and behavioral measures. Moreover, the EMCDDA guidelines advise evaluating the risk of novel NPS relative to traditional drugs of abuse. However, when comparing different compounds within various drug classes, different neurotransmitter binding profiles are typical. Psychedelic drugs, for example, all act as agonists at the serotonin 2a receptor. Nonetheless, there is a wide variety in the subjective effects they can elicit (85), which is attributed to, among others, the binding affinities for serotonin receptor subtypes. Stimulants, on the other hand, typically act on multiple neurotransmitter systems, such as dopamine, noradrenaline, and serotonin (86, 87). However, based on their binding profiles, it is difficult to predict and compare effects (88). Therefore, to make a fair judgment on the neurocognitive and psychotomimetic effects and addictive potential of different drugs, it is advised to compare them based on psychotropic dose equivalence. When using psychotropic dose equivalence in other drug classes, it is advised to use the primary subjective motive for that class of drugs, e.g., feeling energetic with stimulants, or experiencing broadened consciousness with psychedelics (89). In the current study, subjective intoxication levels were used to determine psychotropic dose equivalence, as feeling high is the primary goal for recreational users of cannabinoids.
Up until now, more than 190 SCs have been reported by the EMCDDA, and only for a few of them the acute effects have been studied in humans (29–32, 90, 91). In addition, the most recent generations of SCs, which show increased potency and efficacy at CB1 receptors (92, 93), have not yet been tested in controlled studies. Whether using equipotent dose or psychotropic dose equivalence, controlled clinical studies with SCs and other NPS are challenging for ethical and safety reasons. A safe starting dose should be determined using all available preclinical data on pharmacology, toxicology, pharmacokinetics, and pharmacodynamics, including information retrieved from recreational users. It is vital to find a balance between minimizing the risks and maximizing the benefits (i.e., eliciting a pharmacological response). Furthermore, first-in-human studies with NPS should be conducted as phase 1 studies and implement the guidelines and regulations associated with this type of research (94).
Findings in the present study suggest that the method of psychotropic dose equivalence is more accurate to predict drug outcome as compared to the method of dose equivalence (i.e., based on differences in potency). At psychotropic dose equivalence, THC and JWH-018 impaired cognitive performance to a similar extent, while dissociative effects were more pronounced for JWH-018 than for THC, and positive mood states were more affected by THC. The effects of JWH-018 that we reported in previous laboratory studies may have underestimated the neurocognitive and psychotomimetic effects that SC users may experience in real life when they achieve their maximal desired state of subjective high. In addition, with the high potency of SCs and the inconsistent content of smoking mixtures, it is very difficult for users to predict the maximal subjective high, resulting in very unpredictable neurocognitive outcomes and common overdosing.
The original contributions presented in the study are included in the article/Supplementary Material, further inquiries can be directed to the corresponding author.
The studies involving human participants were reviewed and approved by Medisch-Ethische Toetsingscommissie azM/UM. The patients/participants provided their written informed consent to participate in this study.
ET: analysis of data and writing manuscript. JR, NM, and ET: interpretation of data. All authors: conceptualization and reviewing the manuscript. All authors approved the submitted version of the manuscript and agreed to be accountable for all aspects of the work.
The authors declare that the research was conducted in the absence of any commercial or financial relationships that could be construed as a potential conflict of interest.
All claims expressed in this article are solely those of the authors and do not necessarily represent those of their affiliated organizations, or those of the publisher, the editors and the reviewers. Any product that may be evaluated in this article, or claim that may be made by its manufacturer, is not guaranteed or endorsed by the publisher.
The Supplementary Material for this article can be found online at: https://www.frontiersin.org/articles/10.3389/fpsyt.2022.891811/full#supplementary-material
1. United Nations Office on Drugs and Crime (UNODC). Current NPS Threats. Volume II. Vienna: UNODC Laboratory and Scientific Section (2020).
2. United Nations Office on Drugs and Crime. World Drug Report 2021. Vienna: United Nations Publications (2021).
3. Peacock A, Bruno R, Gisev N, Degenhardt L, Hall W, Sedefov R, et al. New psychoactive substances: challenges for drug surveillance, control, and public health responses. Lancet. (2019) 394:1668–84. doi: 10.1016/S0140-6736(19)32231-7
4. Hudson S, Ramsey J. The emergence and analysis of synthetic cannabinoids. Drug Test Anal. (2011) 3:466–78. doi: 10.1002/dta.268
5. European Monitoring Centre for Drugs and Drug Addiction. Perspectives on Drugs: Synthetic Cannabinoids in Europe. Luxembourg: Publications Office of the European Union (2017).
6. Spaderna M, Addy PH, D'Souza DC. Spicing things up: synthetic cannabinoids. Psychopharmacology. (2013) 228:525–40. doi: 10.1007/s00213-013-3188-4
7. Hermanns-Clausen M, Kneisel S, Szabo B, Auwärter V. Acute toxicity due to the confirmed consumption of synthetic cannabinoids: clinical and laboratory findings. Addiction. (2013) 108:534–44. doi: 10.1111/j.1360-0443.2012.04078.x
8. Tait RJ, Caldicott D, Mountain D, Hill SL, Lenton S. A systematic review of adverse events arising from the use of synthetic cannabinoids and their associated treatment. Clin Toxicol. (2016) 54:1–13. doi: 10.3109/15563650.2015.1110590
9. Pacher P, Steffens S, Haskó G, Schindler TH, Kunos G. Cardiovascular effects of marijuana and synthetic cannabinoids: the good, the bad, and the ugly. Nat Rev Cardiol. (2018) 15:151–66. doi: 10.1038/nrcardio.2017.130
10. Deng H, Verrico CD, Kosten TR, Nielsen DA. Psychosis and synthetic cannabinoids. Psychiatry Res. (2018) 268:400–12. doi: 10.1016/j.psychres.2018.08.012
11. Kraemer M, Boehmer A, Madea B, Maas A. Death cases involving certain new psychoactive substances: a review of the literature. Forensic Sci Int. (2019) 298:186–267. doi: 10.1016/j.forsciint.2019.02.021
12. Giorgetti A, Busardò FP, Tittarelli R, Auwärter V, Giorgetti R. Post-mortem toxicology: a systematic review of death cases involving synthetic cannabinoid receptor agonists. Front Psychiatry. (2020) 11:464. doi: 10.3389/fpsyt.2020.00464
13. European Monitoring Centre for Drugs and Drug Addiction. EU Drug Markets Report 2019. Luxembourg: Publications Office of the European Union (2019).
14. Alam RM, Keating JJ. Adding more “spice” to the pot; a review of the chemistry and pharmacology of newly emerging heterocyclic synthetic cannabinoid receptor agonists. Drug Test Anal. (2020) 12:297–315. doi: 10.1002/dta.2752
15. Malaca S, Busardò FP, Nittari G, Sirignano A, Ricci G. Fourth generation of synthetic cannabinoid receptor agonists: a review on the latest insights. Curr Pharm Des. (2022). doi: 10.2174/1381612827666211115170521. [Epub ahead of print].
16. Castaneto MS, Gorelick DA, Desrosiers NA, Hartman RL, Pirard S, Huestis MA, et al. Synthetic cannabinoids: epidemiology, pharmacodynamics, and clinical implications. Drug Alcohol Depend. (2014) 144:12–41. doi: 10.1016/j.drugalcdep.2014.08.005
17. Winstock A, Lynskey M, Borschmann R, Waldron J. Risk of emergency medical treatment following consumption of cannabis or synthetic cannabinoids in a large global sample. J Psychopharmacol. (2015) 29:698–703. doi: 10.1177/0269881115574493
18. Cohen K, Kapitány-Fövény M, Mama Y, Arieli M, Rosca P, Demetrovics Z, et al. The effects of synthetic cannabinoids on executive function. Psychopharmacology. (2017) 234:1121–34. doi: 10.1007/s00213-017-4546-4
19. Every-Palmer S. Synthetic cannabinoid JWH-018 and psychosis: an explorative study. Drug Alcohol Depend. (2011) 117:152–7. doi: 10.1016/j.drugalcdep.2011.01.012
20. Vandrey R, Dunn KE, Fry JA, Girling ER. A survey study to characterize use of spice products (synthetic cannabinoids). Drug Alcohol Depend. (2012) 120:238–41. doi: 10.1016/j.drugalcdep.2011.07.011
21. Forrester MB, Kleinschmidt K, Schwarz E, Young A. Synthetic cannabinoid and marijuana exposures reported to poison centers. Hum Exp Toxicol. (2012) 31:1006–11. doi: 10.1177/0960327111421945
22. Winstock AR, Barratt MJ. Synthetic cannabis: a comparison of patterns of use and effect profile with natural cannabis in a large global sample. Drug Alcohol Depend. (2013) 131:106–11. doi: 10.1016/j.drugalcdep.2012.12.011
23. Atwood BK, Huffman J, Straiker A, Mackie K. JWH018, a common constituent of 'spice' herbal blends, is a potent and efficacious cannabinoid CB receptor agonist. Br J Pharmacol. (2010) 160:585–93. doi: 10.1111/j.1476-5381.2009.00582.x
24. Brents LK, Reichard EE, Zimmerman SM, Moran JH, Fantegrossi WE, Prather PL, et al. Phase I hydroxylated metabolites of the K2 synthetic cannabinoid JWH-018 retain in vitro and in vivo cannabinoid 1 receptor affinity and activity. PLoS ONE. (2011) 6:e21917. doi: 10.1371/journal.pone.0021917
25. Uttl L, Szczurowska E, Hájková K, Horsley RR, Štefková K, HloŽek T, et al. Behavioral and pharmacokinetic profile of indole-derived synthetic cannabinoids JWH-073 and JWH-210 as compared to the phytocannabinoid Δ9-THC in rats. Front Neurosci. (2018) 12:703. doi: 10.3389/fnins.2018.00703
26. Gurney SM, Scott K, Kacinko S, Presley B, Logan B. Pharmacology, toxicology, and adverse effects of synthetic cannabinoid drugs. Forensic Sci Rev. (2014) 26:53–78.
27. Hess C, Schoeder CT, Pillaiyar T, Madea B, Müller CE. Pharmacological evaluation of synthetic cannabinoids identified as constituents of spice. Forensic Toxicol. (2016) 34:329–43. doi: 10.1007/s11419-016-0320-2
28. Tai S, Fantegrossi WE. Synthetic cannabinoids: pharmacology, behavioral effects, and abuse potential. Curr Addict Rep. (2014) 1:129–36. doi: 10.1007/s40429-014-0014-y
29. Theunissen EL, Hutten NR, Mason NL, Toennes SW, Kuypers KP, Sousa Fernandes Perna EB, et al. Neurocognition and subjective experience following acute doses of the synthetic cannabinoid JWH-018: a phase 1, placebo-controlled, pilot study. Br J Pharmacol. (2018) 175:18–28. doi: 10.1111/bph.14066
30. Theunissen EL, Hutten NR, Mason NL, Toennes SW, Kuypers KP, Ramaekers JG, et al. Neurocognition and subjective experience following acute doses of the synthetic cannabinoid JWH-018: responders versus non-responders. Cannabis Cannabinoid Res. (2019) 4:51–61. doi: 10.1089/can.2018.0047
31. Theunissen EL, Reckweg JT, Hutten NR, Kuypers KP, Toennes SW, Neukamm MA, et al. Intoxication by a synthetic cannabinoid (JWH-018) causes cognitive and psychomotor impairment in recreational cannabis users. Pharmacol Biochem Behav. (2021) 202:173118. doi: 10.1016/j.pbb.2021.173118
32. Theunissen EL, Reckweg JT, Hutten NRPW, Kuypers KP, Toennes SW, Neukamm MA, et al. Psychotomimetic symptoms after a moderate dose of a synthetic cannabinoid (JWH-018): implications for psychosis. Psychopharmacology. (2021) 1–11. doi: 10.1007/s00213-021-05768-0
33. Ramaekers J, Van Wel J, Spronk D, Toennes S, Kuypers K, Theunissen E, et al. Cannabis and tolerance: acute drug impairment as a function of cannabis use history. Sci Rep. (2016) 6:26843. doi: 10.1038/srep26843
34. Van Wel J, Spronk D, Kuypers K, Theunissen E, Toennes S, Verkes R, et al. Psychedelic symptoms of cannabis and cocaine use as a function of trait impulsivity. J Psychopharmacol. (2015) 29:324–34. doi: 10.1177/0269881114563633
35. Ramaekers JG, Kauert G, van Ruitenbeek P, Theunissen EL, Schneider E, Moeller MR, et al. High-potency marijuana impairs executive function and inhibitory motor control. Neuropsychopharmacology. (2006) 31:2296–303. doi: 10.1038/sj.npp.1301068
36. Ramaekers JG, Kauert GF, Theunissen EL, Toennes SW, Moeller MR. Neurocognitive performance during acute THC intoxication in heavy and occasional cannabis users. J Psychopharmacol. (2009) 23:266–77. doi: 10.1177/0269881108092393
37. Jex HR, McDonnell JD, Phatak AV. A “Critical” Tracking Task for Man-Machine Research Related to the Operator's Effective Delay Time. I. Theory and Experiments With a First- Order Divergent Controlled Element. NASA CR-616. NASA CR (1966).
38. Robbe HW, O'Hanlon JF. Acute and subchronic effects of paroxetine 20 and 40 mg on actual driving, psychomotor performance and subjective assessments in healthy volunteers. Eur Neuropsychopharmacol. (1995) 5:35–42. doi: 10.1016/0924-977X(94)00130-4
39. Jongen S, Vuurman E, Ramaekers J, Vermeeren A. Alcohol calibration of tests measuring skills related to car driving. Psychopharmacology. (2014) 231:2435–47. doi: 10.1007/s00213-013-3408-y
40. Vermeeren A, Riedel WJ, van Boxtel MP, Darwish M, Paty I, Patat A. Differential residual effects of zaleplon and zopiclone on actual driving: a comparison with a low dose of alcohol. Sleep. (2002) 25:224–31. doi: 10.1093/sleep/25.2.224
41. Moskowitz H. Laboratory studies of the effects of alcohol on some variables related to driving. J Safety Res. (1973) 5:185–92.
42. Fillmore MT, Rush CR, Hays L. Acute effects of oral cocaine on inhibitory control of behavior in humans. Drug Alcohol Depend. (2002) 67:157–67. doi: 10.1016/S0376-8716(02)00062-5
43. Ramaekers JG, Kuypers KPC. Acute effects of 3,4-methylenedioxymethamphetamine (MDMA) on behavioral measures of impulsivity: alone and in combination with alcohol. Neuropsychopharmacology. (2006) 31:1048–55. doi: 10.1038/sj.npp.1300894
44. Logan GD. On the ability to inhibit thought and action. In: Dagenbach D, Carr TH, editors. Inhibitory Processes in Attention, Memory and Language. San Diego, CA: Academic Press (1994). p. 189–239.
45. Bremner JD, Krystal JH, Putnam FW, Southwick SM, Marmar C, Charney DS, et al. Measurement of dissociative states with the Clinician-Administered Dissociative States Scale (CADSS). J Trauma Stress. (1998) 11:125–36. doi: 10.1023/A:1024465317902
46. Derntl B, Hornung J, Sen ZD, Colic L, Li M, Walter M, et al. Interaction of sex and age on the dissociative effects of ketamine action in young healthy participants. Front Neurosci. (2019) 13:616. doi: 10.3389/fnins.2019.00616
47. van Heugten-Van der Kloet D, Giesbrecht T, van Wel J, Bosker WM, Kuypers KP, Theunissen EL, et al. MDMA, cannabis, and cocaine produce acute dissociative symptoms. Psychiatry Res. (2015) 228:907–12. doi: 10.1016/j.psychres.2015.04.028
48. D'Souza DC, Perry E, MacDougall L, Ammerman Y, Cooper T, Braley G, et al. The psychotomimetic effects of intravenous delta-9-tetrahydrocannabinol in healthy individuals: implications for psychosis. Neuropsychopharmacology. (2004) 29:1558. doi: 10.1038/sj.npp.1300496
49. Bowdle AT, Radant AD, Cowley DS, Kharasch ED, Strassman RJ, Roy-Byrne PP, et al. Psychedelic effects of ketamine in healthy volunteers: relationship to steady-state plasma concentrations. Anesthesiology. (1998) 88:82–8. doi: 10.1097/00000542-199801000-00015
50. Zuurman L, Roy C, Schoemaker R, Hazekamp A, Den Hartigh J, Bender J, et al. Effect of intrapulmonary tetrahydrocannabinol administration in humans. J Psychopharmacol. (2008) 22:707–16. doi: 10.1177/0269881108089581
51. de Wit H, Enggasser JL, Richards JB. Acute administration of d-amphetamine decreases impulsivity in healthy volunteers. Neuropsychopharmacology. (2002) 27:813–25. doi: 10.1016/S0893-133X(02)00343-3
52. Richardson JT. Eta squared and partial eta squared as measures of effect size in educational research. Educ Res Rev. (2011) 6:135–47. doi: 10.1016/j.edurev.2010.12.001
53. Hunter EC, Sierra M, David AS. The epidemiology of depersonalisation and derealisation. Soc Psychiatry Psychiatr Epidemiol. (2004) 39:9–18. doi: 10.1007/s00127-004-0701-4
54. Stark T, Di Martino S, Drago F, Wotjak CT, Micale V. Phytocannabinoids and schizophrenia: focus on adolescence as a critical window of enhanced vulnerability and opportunity for treatment. Pharmacol Res. (2021) 174:105938. doi: 10.1016/j.phrs.2021.105938
55. Every-Palmer S. Warning: legal synthetic cannabinoid-receptor agonists such as JWH-018 may precipitate psychosis in vulnerable individuals. Addiction. (2010) 105:1859–60. doi: 10.1111/j.1360-0443.2010.03119.x
56. Papanti D, Schifano F, Botteon G, Bertossi F, Mannix J, Vidoni D, et al. “Spiceophrenia”: a systematic overview of “spice”-related psychopathological issues and a case report. Hum Psychopharmacol Clin Exp. (2013) 28:379–89. doi: 10.1002/hup.2312
57. Peglow S, Buchner J, Briscoe G. Synthetic cannabinoid induced psychosis in a previously nonpsychotic patient. Am J Addict. (2012) 21:287–8. doi: 10.1111/j.1521-0391.2012.00222.x
58. Fattore L. Synthetic cannabinoids—further evidence supporting the relationship between cannabinoids and psychosis. Biol Psychiatry. (2016) 79:539–48. doi: 10.1016/j.biopsych.2016.02.001
59. Radhakrishnan R, Wilkinson ST, D'Souza DC. Gone to pot—a review of the association between cannabis and psychosis. Front Psychiatry. (2014) 5:54. doi: 10.3389/fpsyt.2014.00054
60. D'Souza DC, Abi-Saab WM, Madonick S, Forselius-Bielen K, Doersch A, Braley G, et al. Delta-9-tetrahydrocannabinol effects in schizophrenia: implications for cognition, psychosis, and addiction. Biol Psychiatry. (2005) 57:594–608. doi: 10.1016/j.biopsych.2004.12.006
61. Di Forti M, Marconi A, Carra E, Fraietta S, Trotta A, Bonomo M, et al. Proportion of patients in south London with first-episode psychosis attributable to use of high potency cannabis: a case-control study. Lancet Psychiatry. (2015) 2:233–8. doi: 10.1016/S2215-0366(14)00117-5
62. Vallersnes OM, Dines AM, Wood DM, Yates C, Heyerdahl F, Hovda KE, et al. Psychosis associated with acute recreational drug toxicity: a European case series. BMC Psychiatry. (2016) 16:1–8. doi: 10.1186/s12888-016-1002-7
63. Hermanns-Clausen M, Müller D, Kithinji J, Angerer V, Franz F, Eyer F, et al. Acute side effects after consumption of the new synthetic cannabinoids AB-CHMINACA and MDMB-CHMICA. Clin Toxicol. (2018) 56:404–11. doi: 10.1080/15563650.2017.1393082
64. van Amsterdam J, Brunt T, van den Brink W. The adverse health effects of synthetic cannabinoids with emphasis on psychosis-like effects. J Psychopharmacol. (2015) 29:254–63. doi: 10.1177/0269881114565142
65. Di Bartolomeo M, Stark T, Maurel OM, Iannotti FA, Kuchar M, Ruda-Kucerova J, et al. Crosstalk between the transcriptional regulation of dopamine D2 and cannabinoid CB1 receptors in schizophrenia: analyses in patients and in perinatal Δ9-tetrahydrocannabinol-exposed rats. Pharmacol Res. (2021) 164:105357. doi: 10.1016/j.phrs.2020.105357
66. Kucerova J, Tabiova K, Drago F, Micale V. Therapeutic potential of cannabinoids in schizophrenia. Recent Pat CNS Drug Discov. (2014) 9:13–25. doi: 10.2174/1574889809666140307115532
67. Di Forti M, Morgan C, Dazzan P, Pariante C, Mondelli V, Marques TR, et al. High-potency cannabis and the risk of psychosis. Br J Psychiatry. (2009) 195:488–91. doi: 10.1192/bjp.bp.109.064220
68. Iseger TA, Bossong MG. A systematic review of the antipsychotic properties of cannabidiol in humans. Schizophr Res. (2015) 162:153–61. doi: 10.1016/j.schres.2015.01.033
69. Zuardi AW, Crippa JA, Hallak J, Bhattacharyya S, Atakan Z, Martín-Santos R, et al. A critical review of the antipsychotic effects of cannabidiol: 30 years of a translational investigation. Curr Pharm Des. (2012) 18:5131–40. doi: 10.2174/138161212802884681
70. Fantegrossi WE, Wilson CD, Berquist MD. Pro-psychotic effects of synthetic cannabinoids: interactions with central dopamine, serotonin, and glutamate systems. Drug Metab Rev. (2018) 50:65–73. doi: 10.1080/03602532.2018.1428343
71. Mason NL, Theunissen EL, Hutten N, Tse DHY, Toennes SW, Stiers P, et al. Cannabis induced increase in striatal glutamate associated with loss of functional corticostriatal connectivity. Eur Neuropsychopharmacol. (2019) 29:247–56. doi: 10.1016/j.euroneuro.2018.12.003
72. Colizzi M, Weltens N, McGuire P, Lythgoe D, Williams S, Van Oudenhove L, et al. Delta-9-tetrahydrocannabinol increases striatal glutamate levels in healthy individuals: implications for psychosis. Mol Psychiatry. (2020) 25:3231–40. doi: 10.1038/s41380-019-0374-8
73. Chait LD, Perry JL. Acute and residual effects of alcohol and marijuana, alone and in combination, on mood and performance. Psychopharmacology. (1994) 115:340–9. doi: 10.1007/BF02245075
74. Chait L, Zacny JP. Reinforcing and subjective effects of oral Δ9-THC and smoked marijuana in humans. Psychopharmacology. (1992) 107:255–62. doi: 10.1007/BF02245145
75. Lile JA, Kelly TH, Hays LR. The reinforcing, self-reported, performance and physiological effects of Δ9-tetrahydrocannabinol, triazolam, hydromorphone and methylphenidate in cannabis users. Behav Pharmacol. (2010) 21:29. doi: 10.1097/FBP.0b013e32833470d7
76. Chait L, Evans SM, Grant K, Kamien J, Johanson C, Schuster C. Discriminative stimulus and subjective effects of smoked marijuana in humans. Psychopharmacology. (1988) 94:206–12. doi: 10.1007/BF00176846
77. Childs E, Lutz JA, de Wit H. Dose-related effects of delta-9-THC on emotional responses to acute psychosocial stress. Drug Alcohol Depend. (2017) 177:136–44. doi: 10.1016/j.drugalcdep.2017.03.030
78. McDonald J, Schleifer L, Richards JB, de Wit H. Effects of THC on behavioral measures of impulsivity in humans. Neuropsychopharmacology. (2003) 28:1356–65. doi: 10.1038/sj.npp.1300176
79. Matheson J, Mann RE, Sproule B, Huestis MA, Wickens CM, Stoduto G, et al. Acute and residual mood and cognitive performance of young adults following smoked cannabis. Pharmacol Biochem Behav. (2020) 194:172937. doi: 10.1016/j.pbb.2020.172937
80. Matheson J, Sproule B, Di Ciano P, Fares A, Le Foll B, Mann RE, et al. Sex differences in the acute effects of smoked cannabis: evidence from a human laboratory study of young adults. Psychopharmacology. (2020) 237:305–16. doi: 10.1007/s00213-019-05369-y
81. Castellanos D, Thornton G. Synthetic cannabinoid use: recognition and management. J Psychiatr Pract. (2012) 18:86–93. doi: 10.1097/01.pra.0000413274.09305.9c
82. Sholler DJ, Strickland JC, Spindle TR, Weerts EM, Vandrey R. Sex differences in the acute effects of oral and vaporized cannabis among healthy adults. Addict Biol. (2021) 26:e12968. doi: 10.1111/adb.12968
83. Arkell TR, Kevin RC, Vinckenbosch F, Lintzeris N, Theunissen E, Ramaekers JG, et al. Sex differences in acute cannabis effects revisited: results from two randomized, controlled trials. Addict Biol. (2022) 27:e13125. doi: 10.1111/adb.13125
84. EMCDDA. Risk Assessment of New Psychoactive Substances: Operating Guidelines. Luxemburg: European Monitoring Centre for Drugs and Drug addiction (2009).
85. Zamberlan F, Sanz C, Martínez Vivot R, Pallavicini C, Erowid E, Tagliazucchi E, et al. The varieties of the psychedelic experience: a preliminary study of the association between the reported subjective effects and the binding affinity profiles of substituted phenethylamines and tryptamines. Front Integr Neurosci. (2018) 12:54. doi: 10.3389/fnint.2018.00054
86. Baumann MH, Walters HM, Niello M, Sitte HH. Neuropharmacology of synthetic cathinones. Handb Exp Pharmacol. (2018) 252:113–42. doi: 10.1007/164_2018_178
87. Liechti ME. Novel psychoactive substances (designer drugs): overview and pharmacology of modulators of monoamine signalling. Swiss Med Wkly. (2015) 145:w14043. doi: 10.4414/smw.2015.14043
88. Romach MK, Schoedel KA, Sellers EM. Human abuse liability evaluation of CNS stimulant drugs. Neuropharmacology. (2014) 87:81–90. doi: 10.1016/j.neuropharm.2014.04.014
89. Kettner H, Mason NL, Kuypers KP. Motives for classical and novel psychoactive substances use in psychedelic polydrug users. Contemp Drug Probl. (2019) 46:304–20. doi: 10.1177/0091450919863899
90. Maida NL, Papaseit E, Martínez L, Pérez-Mañá C, Poyatos L, Pellegrini M, et al. Acute pharmacological effects and oral fluid biomarkers of the synthetic cannabinoid ur-144 and thc in recreational users. Biology. (2021) 10:257. doi: 10.3390/biology10040257
91. Martínez L, La Maida N, Papaseit E, Pérez-Mañá C, Poyatos L, Pellegrini M, et al. Acute pharmacological effects and oral fluid concentrations of the synthetic cannabinoids JWH-122 and JWH-210 in humans after self-administration: an observational study. Front Pharmacol. (2021) 12:705643. doi: 10.3389/fphar.2021.705643
92. Krotulski AJ, Cannaert A, Stove C, Logan BK. The next generation of synthetic cannabinoids: detection, activity, and potential toxicity of pent-4en and but-3en analogues including MDMB-4en-PINACA. Drug Test Anal. (2021) 13:427–38. doi: 10.1002/dta.2935
93. Cannaert A, Sparkes E, Pike E, Luo JL, Fang A, Kevin RC, et al. Synthesis and in vitro cannabinoid receptor 1 activity of recently detected synthetic cannabinoids 4F-MDMB-BICA, 5F-MPP-PICA, MMB-4en-PICA, CUMYL-CBMICA, ADB-BINACA, APP-BINACA, 4F-MDMB-BINACA, MDMB-4en-PINACA, A-CHMINACA, 5F-AB-P7AICA, 5F-MDMB-P7AICA, and 5F-AP7AICA. ACS Chem Neurosci. (2020) 11:4434–46. doi: 10.1021/acschemneuro.0c00644
Keywords: cannabinoids, THC, JWH-018, psychotropic dose equivalence, neurocognitive effects, psychotomimetic effects
Citation: Theunissen EL, Kuypers KPC, Mason NL and Ramaekers JG (2022) A Comparison of Acute Neurocognitive and Psychotomimetic Effects of a Synthetic Cannabinoid and Natural Cannabis at Psychotropic Dose Equivalence. Front. Psychiatry 13:891811. doi: 10.3389/fpsyt.2022.891811
Received: 08 March 2022; Accepted: 26 April 2022;
Published: 19 May 2022.
Edited by:
Danilo De Gregorio, Vita-Salute San Raffaele University, ItalyReviewed by:
Vincenzo Micale, Università di Catania, ItalyCopyright © 2022 Theunissen, Kuypers, Mason and Ramaekers. This is an open-access article distributed under the terms of the Creative Commons Attribution License (CC BY). The use, distribution or reproduction in other forums is permitted, provided the original author(s) and the copyright owner(s) are credited and that the original publication in this journal is cited, in accordance with accepted academic practice. No use, distribution or reproduction is permitted which does not comply with these terms.
*Correspondence: Eef Lien Theunissen, ZS50aGV1bmlzc2VuQG1hYXN0cmljaHR1bml2ZXJzaXR5Lm5s
Disclaimer: All claims expressed in this article are solely those of the authors and do not necessarily represent those of their affiliated organizations, or those of the publisher, the editors and the reviewers. Any product that may be evaluated in this article or claim that may be made by its manufacturer is not guaranteed or endorsed by the publisher.
Research integrity at Frontiers
Learn more about the work of our research integrity team to safeguard the quality of each article we publish.