- Department of Neuropsychology and Psychopharmacology, Faculty of Psychology and Neuroscience, Maastricht University, Maastricht, Netherlands
Currently, the assessment of the neurobehavioral consequences of repeated cannabis use is restricted to studies in which brain function of chronic cannabis users is compared to that of non-cannabis using controls. The assumption of such studies is that changes in brain function of chronic users are caused by repeated and prolonged exposure to acute cannabis intoxication. However, differences in brain function between chronic cannabis users and non-users might also arise from confounding factors such as polydrug use, alcohol use, withdrawal, economic status, or lifestyle conditions. We propose a methodology that highlights the relevance of acute Δ9-tetrahydrocannabinol (THC) dosing studies for a direct assessment of neuroadaptations in chronic cannabis users. The approach includes quantification of neurochemical, receptor, and functional brain network changes in response to an acute cannabis challenge, as well as stratification of cannabis using groups ranging from occasional to cannabis-dependent individuals. The methodology allows for an evaluation of THC induced neuroadaptive and neurocognitive changes across cannabis use history, that can inform neurobiological models on reward driven, compulsive cannabis use.
Introduction
Cannabis is the most commonly used illicit drug, with 4% of the global population reportedly using the substance (1). The prevalence of cannabis use is expected to increase following recent trends to legalize or decriminalize its use for recreational and therapeutic purposes (2, 3). Thus, as cannabis use increases and the perception of risk of use decreases (4), a pertinent question is how prolonged cannabis use affects the neurocognitive state, and whether there are long-term neurobiological consequences (5). Furthermore, as 10% of those who recreationally consume cannabis develop daily use patterns (6), it is prudent to understand neuroadaptations in the neuro-circuitry which may underlie this increase and persistence of use.
Neuroadaptation in chronic cannabis users has traditionally been evaluated with brain imaging measures in comparison to non-cannabis using controls. Many of these cross-sectional fMRI studies have revealed changes in functional connectivity (7–11), task-related brain activation (12–18) and neurotransmission (19–23) in various brain regions of chronic cannabis users, sometimes in association with cognitive deficits (24–29). However, findings have also been mixed as these studies suffer from the methodological problem that confounding factors (e.g., pre-existing differences, polydrug use, and differences in lifestyle) cannot adequately be controlled and therefore, in addition to cannabis use, might explain observed differences in brain function (30). Cross-sectional studies between chronic cannabis users and non-cannabis users therefore cannot be taken as the sole approach for studying the effects of repeated cannabis use on brain function and its associated neurocognitive state.
Alternatively, we propose that neuroadaptive changes in cannabis users can more selectively be studied in response to an acute challenge with Δ9 -tetrahydrocannabinol (THC). Ideally, such studies would follow a multimodal imaging approach that includes resting state fMRI to assess functional connectivity, PET imaging to profile CB1 receptor densities, and magnetic resonance spectroscopy to quantify neurometabolites in neural circuits in which neuroadaptions are prominent, such as the mesocorticolimbic circuit (31) (Figure 1). Neural changes to an acute THC challenge should be assessed as a function of cannabis use frequency as there is a notion that neural mechanisms underlying acute and long-term cognitive deficits are interrelated and that the latter can be explained as a neuroadaptive response to the former (32). For example, an acute dose of THC has been found to increase glutamate (33–35) and dopamine (36–39) in the striatum of occasional users, whereas sober chronic cannabis users demonstrate a decrease of glutamate and glutamate-related metabolites (19–22), and lower levels of dopamine release (40, 41). Likewise, fMRI studies have repeatedly shown hypoactivation in the mesocorticolimbic circuit during acute THC intoxication (32, 33, 35) in occasional users but hyperactivation in sober, chronic cannabis users (12, 15–17, 26, 42). The intermediate mechanism might be CB1 receptor density that is known to fluctuate with cannabis frequency and represents a neuroadaptive response of the brain to regain homeostasis following sustained and repeated THC exposure (31). It can be hypothesized that this neuroadaptive response in chronic cannabis users is aimed to normalize cognitive function during THC intoxication, but causes underactivation of brain function when sober (32). The dynamics of this antipodal neural response underlying acute and long-term effects of cannabis can typically be studied in placebo-controlled THC studies as a function of cannabis use frequency as shown in Figure 2. This approach could be applied to a number of research issues that are closely associated with cannabis use disorder, as discussed below. These include assessments of neural mechanisms that underlie the progression to compulsive cannabis use, the development of tolerance, and their association to neurocognitive key-elements of addiction such as reward, craving, and cognitive control (43).
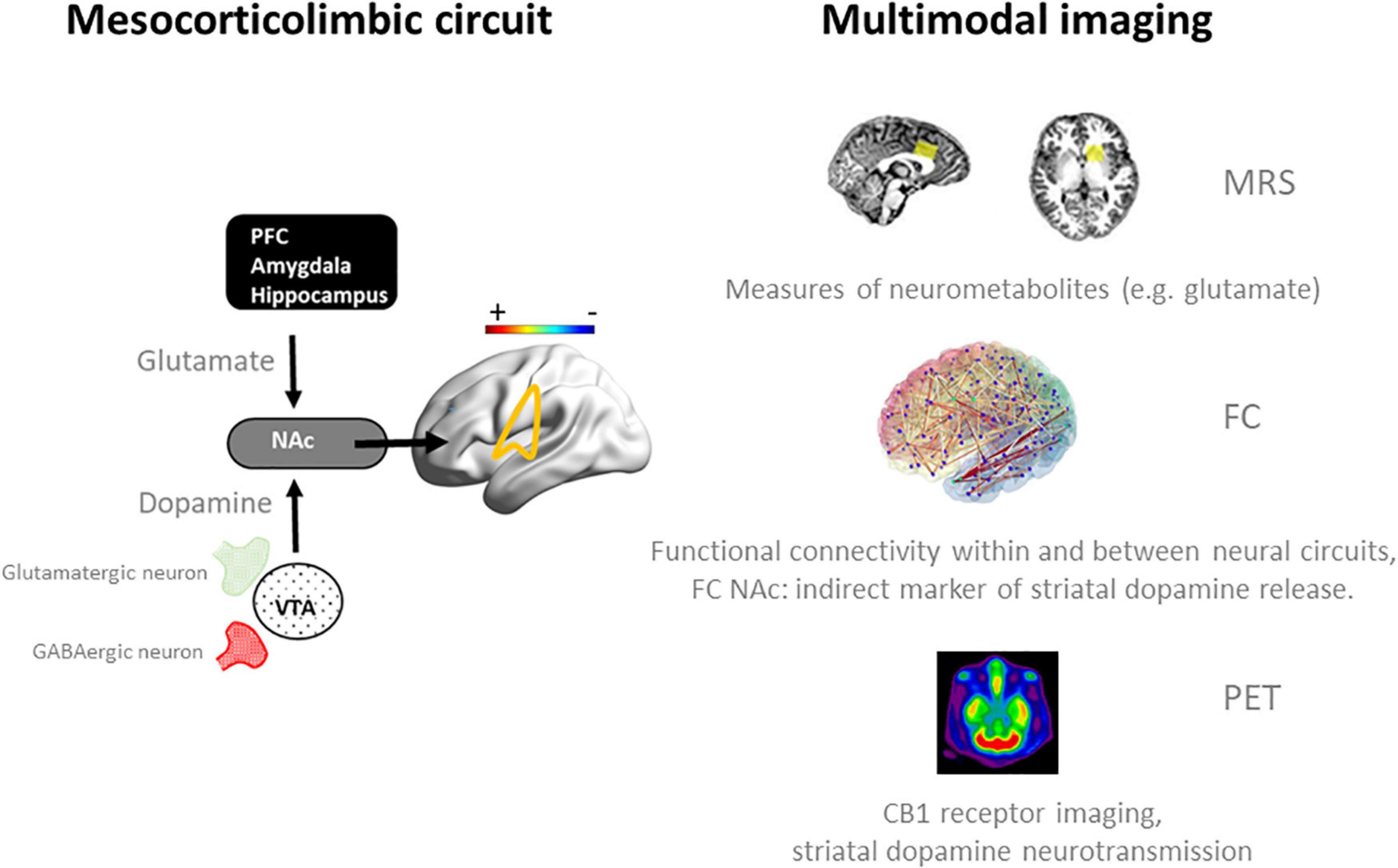
Figure 1. Schematic representation of neurotransmission and functional connectivity in the mesocorticolimbic circuit in the normal brain (32) (left panel) and multimodal imaging measures (right panel) that can be employed to assess dynamics within this circuit during an acute challenge with THC and when sober. Magnetic Resonance Spectroscopy (MRS) has been successfully used to quantify frontal and striatal glutamate concentrations in cannabis users during intoxication and when sober (33, 34). fMRI measures of functional connectivity (FC) have provided functional associations within and between neural networks in cannabis users during intoxication (33, 35, 42) and when sober (15–17, 42), and can be used as an indirect marker of striatal dopamine release during cannabis intoxication (33, 81). Positron emission tomography (PET) can be used to determine CB1 receptor density at glutamatergic and GABAergic neurons in the striatum of cannabis users (60, 62) and to determine dopamine displacement at D2/D3 receptors as a measure of dopamine transmission during cannabis intoxication (36). NAc, nucleus accumbens; PFC, prefrontal cortex.
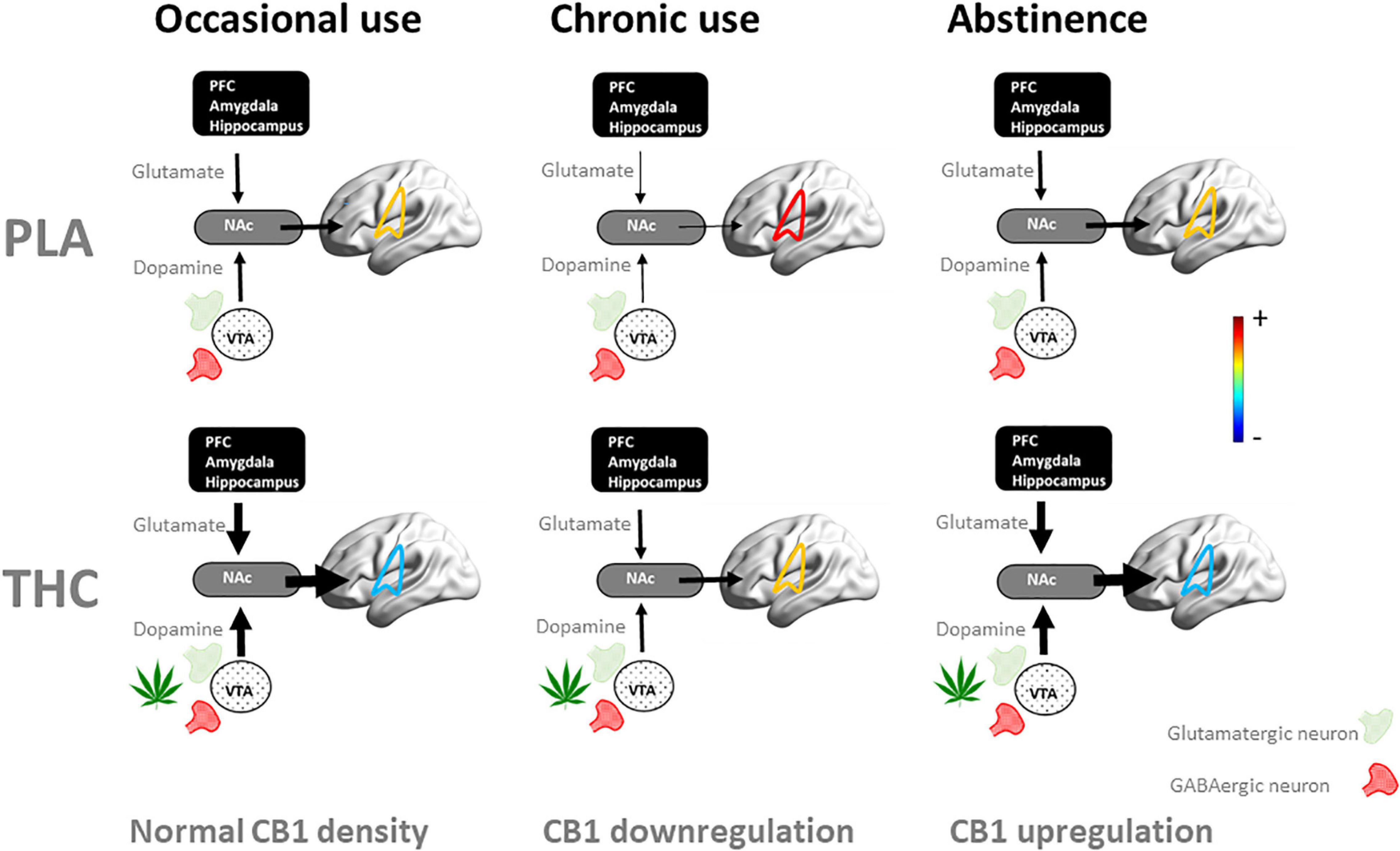
Figure 2. Schematic representation of the dynamics of the neuroadaptive state of the mesocorticolimbic circuit when challenged with placebo (PLA) and THC as users proceed from occasional to chronic cannabis use and into abstinence. In occasional users, an acute THC challenge increases striatal levels of dopamine and glutamate (33–35) through stimulation of CB1 receptors at presynaptic GABAergic and glutamatergic receptors, leading to hypoconnectivity of the mesocorticolimbic circuit (here shown superimposed on brain) and neurocognitive dysfunction (33, 35). With repeated cannabis use, CB1 receptors are downregulated as a neuroadaptive response to CB1 receptor overstimulation (60, 62). In chronic users, this leads to reduced striatal dopamine (40, 41) and glutamate (19–22), as well as hyperconnectivity within the mesocorticolimbic circuit (9, 42, 82) and neurocognitive dysfunction when sober (13, 24, 27, 28). In these users, stimulation of CB1 receptors normalizes striatal dopamine and glutamate concentrations, functional connectivity and neurocognitive function during acute THC intoxication (35). With prolonged abstinence of cannabis, CB1 receptors upregulate (60, 62) and potentially normalize the neuroadaptive and neurocognitive state (63, 64, 66). NAc, nucleus accumbens; PFC, prefrontal cortex.
Transition to Compulsive Cannabis Use
Acute THC studies can be employed to increase or confirm our understanding of the neural and psychological basis of the transition from initial cannabis use to compulsive use. Compulsive cannabis use occurs in parallel to the development of cannabis tolerance but their underlying neural mechanisms may differ. Preclinical studies have suggested that THC-induced dopamine release shifts from the ventral striatum to the dorsal striatum after repeated administration (44), a transition that has been associated with the development of dependence in humans (45). In addiction research, dorsal striatal activation dominance in drug use disorders has been associated with habitual, drug-seeking behavior (43, 46–49), while increased ventral striatal activation has been associated with increased responsiveness to drug-related cues and reward (50–52). Indeed, dependent and non-dependent cannabis users have shown increased responsiveness of the ventral striatum in response to cannabis-related cues (8, 45, 53–56) and increased striatal frontal coupling (45). Dependent users also exhibit increased dorsal striatal reactivity and decreased striatal limbic coupling during cannabis cue exposure (45). Shifts in ventral and dorsal activation as observed in cannabis users are thus intrinsically related to the frequency of acute cannabis intoxications. The development of the striatal response to cannabis cues should therefore also be studied and understood in the context of acute THC challenge studies in groups with varying cannabis use frequencies. Such studies could potentially monitor directly how acute THC intoxication affects ventral-dorsal striatal activation as a function of cannabis use history and further clarify the contributory roles of striatal dopaminergic and glutamatergic neurotransmission to excessive cannabis use and habit formation. The latter is of particular relevance, as a number of acute THC studies have shown that a single dose of THC attenuates the striatal response to rewards such as monetary incentives, cannabis marketing, and music (56–58) suggesting that THC-induced increments in striatal dopamine reduce salience and attentional processing of concurrent rewards (56). Acute THC challenge studies therefore would not only contribute to a fundamental understanding of ventral-dorsal striatal activation with increasing cannabis use, but also provide important pharmacological insights on how shifts in striatal balance can be prevented or controlled in order to avoid progression to compulsive cannabis use.
Dynamics of Tolerance and Neurocognition
Neurocognitive impairments observed during cannabis intoxication are transient and dynamic over time, depending on cannabis use frequency (31). Understanding the dynamics of tolerance can be useful when trying to “tailor” the cannabis experience and develop dosing strategies which promote tolerance in patient populations where they do not want the “high” and neurocognitive impairment. Alternatively, dynamics of tolerance can also inform strategies to promote efficient “tolerance breaks” for individuals who want the “high” and the associated neurocognitive state, but wish to reduce the potential for addiction, as tolerance development promotes addictive behavior (31, 59).
The neurocognitive state of cannabis users is strongly associated with neuroadaptations in the mesocorticolimbic circuit that occur during acute intoxication, chronic use, and abstinence (see Figure 2). Chronic exposure to cannabis produces significant downregulation and desensitization of CB1 receptors in cannabis-dependent users relative to that in controls (60–62). This homeostatic response of the brain also potentially causes a state of underactivation and neurocognitive dysfunction in chronic cannabis users when sober (31, 63–65). Typically, such deficits rapidly decrease during abstinence and do not persist beyond 4–5 weeks (63, 66). It has been hypothesized that neurocognitive impairment in chronic users arise from a state of withdrawal during which CB1 receptors are downregulated and restrained from THC-related receptor stimulation (32). Subsequent CB1 receptor upregulation observed during withdrawal in chronic users (60) was indeed paralleled by an improvement in neurocognitive function (64). Interestingly, the only study to date that investigated the neuroadaptive response to an acute challenge in chronic cannabis users (35) suggested that stimulation of CB1 receptors subsequently normalizes striatal glutamate and dopamine transmission, functional mesocorticolimbic connectivity, and neurocognitive function (31, 32, 35). This neuroadaptive response may also explain the absence of neurocognitive impairment (i.e., tolerance) that is often reported in chronic cannabis users during THC intoxication (31, 67).
Neuroimaging studies can be instrumental in assessing the dynamics of CB1 receptor density and neurocognitive state before or after an acute THC challenge in groups of cannabis users who vary in their frequency of use. Such studies could establish downregulation of CB1 receptor density with increasing cannabis use and determine the impact of CB1 downregulation on mesocorticolimbic function and neurocognitive state, in the presence and absence of an acute THC challenge. Increased knowledge of how temporal changes in cannabis use frequency affect the dynamics of an individual’s response and neuroadaptations to an acute challenge with cannabis, will gain relevance with increasing recreational and medical use of cannabis. Frequency, dose, and duration of use to achieve or reverse tolerance are currently unknown but are important to define recreational use frequencies and medical dosing strategies at which the development of acute tolerance, persistence of neurocognitive deficits, and compulsive use can be avoided.
Markers of the Neurocognitive State
Acute THC studies might also serve to identify neural markers of cognitive function that differentiate compulsive cannabis use from non-problematic cannabis exposure, or the impaired state from the non-impaired state. At present, there is no objective assessment that can classify the neurocognitive state in individual cannabis users. Recent studies have suggested, however, that acute THC intoxication as assessed by subjective ratings of “high” produces a reproducible signature change in brain function that can be detected with neuroimaging techniques (42, 68). The former study (68) conducted functional near-infrared spectroscopy (fNIRS) in cannabis users before and after receiving oral THC and placebo and found increased oxygenated hemoglobin concentration (HbO) in the prefrontal cortex of participants with a clinical rating of subjective intoxication. Machine learning models using fNIRS time course features and connectivity matrices identified the intoxicated state with 76.4% accuracy (68). The latter study (42) used a data-driven independent component methodology to analyze fMRI resting state data to extract a distinct spatial connectivity pattern of hypoconnectivity involving the dorsal attention, limbic, subcortical and cerebellum networks, and of hyperconnectivity between the default mode and ventral attention network, that was associated with the feeling of a subjective “high” during THC intoxication (42). That same study also revealed a broad state of hyperconnectivity within whole-brain networks in chronic cannabis users compared to occasional cannabis users, which might be reflective of an adaptive network reorganization following prolonged cannabis exposure. These acute THC studies suggest that neural fingerprints of cannabis intoxication and cannabis use history can be derived from neuroimaging data. Future studies with acute THC challenges might identify neurobiological features or phenotype characteristics of impaired and maladaptive behaviors that might arise from acute and chronic use of cannabis. Such models might provide unique insights into emerging adaptations of distinct functional networks in users that progress from occasional to chronic cannabis use and underlie the development of a pathological state, such as cannabis use disorder.
Concluding Remarks
While the effects of chronic cannabis exposure on brain function and cognition have become a focal point for research, much remains unknown about neuroadaptive responses to acute THC intoxication, and how these develop over time into chronic cannabis use. This paper posits that pharmaco-imaging studies should be considered to explore how neural responses to an acute THC challenge develop with repeated cannabis use and how such neuroadaptations relate to the development of cannabis tolerance, compulsive cannabis use, and their associated neurocognitive state. Such studies could also target additional factors that are known to moderate the neural response to an acute THC challenge, such a dose, potency, composition, and formulations of cannabis products as well the interaction with underlying pathological states in case of medical use (32). In principle, this approach could also be expanded on to assess neuroadaptations to other drugs of abuse. For example, acute and chronic alterations in neurotransmission and functional connectivity of the mesocorticolimbic circuit have been reported for cocaine (69–71), nicotine (72–76), and alcohol (77–80). The current proposal on how to assess and define neuroadaptations in cannabis users would also call for an international, multi-center research effort in order to include large samples of distinct cannabis user groups, ranging from novice and occasional users at the lowest end of the use frequency spectrum to daily, chronic users at the opposite extreme. It would offer a unique opportunity to develop an integrative, mechanistic view of long-term effects of cannabis on the brain as a neuroadaptive response to acute THC challenges or to the absence thereof.
Author Contributions
All authors contributed to the manuscript and approved its content.
Conflict of Interest
The authors declare that the research was conducted in the absence of any commercial or financial relationships that could be construed as a potential conflict of interest.
Publisher’s Note
All claims expressed in this article are solely those of the authors and do not necessarily represent those of their affiliated organizations, or those of the publisher, the editors and the reviewers. Any product that may be evaluated in this article, or claim that may be made by its manufacturer, is not guaranteed or endorsed by the publisher.
References
2. Hall W, Lynskey M. Evaluating the public health impacts of legalizing recreational cannabis use in the United States. Addiction. (2016) 111:1764–73. doi: 10.1111/add.13428
3. Hasin DS, Shmulewitz D, Sarvet AL. Time trends in US cannabis use and cannabis use disorders overall and by sociodemographic subgroups: a narrative review and new findings. Am J Drug Alcohol Abuse. (2019) 5:623–43. doi: 10.1080/00952990.2019.1569668
4. Johnston LD, Miech RA, O’Malley PM, Bachman JG, Schulenberg JE, Patrick ME. Monitoring the Future National Survey Results on Drug Use, 1975-2017: Overview, Key Findings on Adolescent Drug Use. Ann Arbor, MI: University of Michigan (2018).
5. Zehra A, Burns J, Liu CK, Manza P, Wiers CE, Volkow ND, et al. Cannabis addiction and the brain: a review. J Neuroimmune Pharmacol. (2018) 13:438–52.
6. World Health Organization.Health and Social Effects of Nonmedical Cannabis Use (The). Geneva: World Health Organization (2016).
7. Cheng H, Skosnik PD, Pruce BJ, Brumbaugh MS, Vollmer JM, Fridberg DJ, et al. Resting state functional magnetic resonance imaging reveals distinct brain activity in heavy cannabis users - a multi-voxel pattern analysis. J Psychopharmacol. (2014) 28:1030–40. doi: 10.1177/0269881114550354
8. Filbey FM, Dunlop J. Differential reward network functional connectivity in cannabis dependent and non-dependent users. Drug Alcohol Depend. (2014) 140:101–11. doi: 10.1016/j.drugalcdep.2014.04.002
9. Pujol J, Blanco-Hinojo L, Batalla A, Lopez-Sola M, Harrison BJ, Soriano-Mas C, et al. Functional connectivity alterations in brain networks relevant to self-awareness in chronic cannabis users. J Psychiatr Res. (2014) 51:68–78. doi: 10.1016/j.jpsychires.2013.12.008
10. Lopez-Larson MP, Rogowska J, Yurgelun-Todd D. Aberrant orbitofrontal connectivity in marijuana smoking adolescents. Dev Cogn Neurosci. (2015) 16:54–62. doi: 10.1016/j.dcn.2015.08.002
11. Newman SD, Cheng H, Kim DJ, Schnakenberg-Martin A, Dydak U, Dharmadhikari S, et al. An investigation of the relationship between glutamate and resting state connectivity in chronic cannabis users. Brain Imaging Behav. (2020) 14:2062–71. doi: 10.1007/s11682-019-00165-w
12. Kanayama G, Rogowska J, Pope HG, Gruber SA, Yurgelun-Todd DA. Spatial working memory in heavy cannabis users: a functional magnetic resonance imaging study. Psychopharmacology (Berl). (2004) 176:239–47. doi: 10.1007/s00213-004-1885-8
13. Chang L, Yakupov R, Cloak C, Ernst T. Marijuana use is associated with a reorganized visual-attention network and cerebellar hypoactivation. Brain. (2006) 129:1096–112. doi: 10.1093/brain/awl064
14. Abdullaev Y, Posner MI, Nunnally R, Dishion TJ. Functional MRI evidence for inefficient attentional control in adolescent chronic cannabis abuse. Behav Brain Res. (2010) 215:45–57. doi: 10.1016/j.bbr.2010.06.023
15. Jager G, Block RI, Luijten M, Ramsey NF. Cannabis use and memory brain function in adolescent boys: a cross-sectional multicenter functional magnetic resonance imaging study. J Am Acad Child Adolesc. Psychiatry. (2010) 572:e561–3. doi: 10.1016/j.jaac.2010.02.001
16. Cousijn J, Wiers RW, Ridderinkhof KR, Van Den Brink W, Veltman DJ, Porrino LJ, et al. Individual differences in decision making and reward processing predict changes in cannabis use: a prospective functional magnetic resonance imaging study. Addict Biol. (2013) 18:1013–23. doi: 10.1111/j.1369-1600.2012.00498.x
17. Colizzi M, Fazio L, Ferranti L, Porcelli A, Masellis R, Marvulli D, et al. Functional genetic variation of the cannabinoid receptor 1 and cannabis use interact on prefrontal connectivity and related working memory behavior. Neuropsychopharmacology. (2015) 40:640–9. doi: 10.1038/npp.2014.213
18. Gruber SA, Sagar KA, Dahlgren MK, Gonenc A, Smith RT, Lambros AM, et al. The grass might be greener: medical marijuana patients exhibit altered brain activity and improved executive function after 3 months of treatment. Front Pharmacol. (2017) 8:983. doi: 10.3389/fphar.2017.00983
19. Chang L, Cloak C, Yakupov R, Ernst T. Combined and independent effects of chronic marijuana use and HIV on brain metabolites. J Neuroimmune Pharmacol. (2006) 1:65–76. doi: 10.1007/s11481-005-9005-z
20. Prescot AP, Locatelli AE, Renshaw PF, Yurgelun-Todd DA. Neurochemical alterations in adolescent chronic marijuana smokers: a proton MRS study. Neuroimage. (2011) 57:69–75. doi: 10.1016/j.neuroimage.2011.02.044
21. Muetzel RL, Marjanska M, Collins PF, Becker MP, Valabregue R, Auerbach EJ, et al. In vivo (1)H magnetic resonance spectroscopy in young-adult daily marijuana users. Neuroimage Clin. (2013) 2:581–9. doi: 10.1016/j.nicl.2013.04.011
22. Prescot AP, Renshaw PF, Yurgelun-Todd DA. gamma-Amino butyric acid and glutamate abnormalities in adolescent chronic marijuana smokers. Drug Alcohol Depend. (2013) 129:232–9. doi: 10.1016/j.drugalcdep.2013.02.028
23. Bloomfield MA, Morgan CJ, Kapur S, Curran HV, Howes OD. The link between dopamine function and apathy in cannabis users: an [18F]-DOPA PET imaging study. Psychopharmacology (Berl). (2014) 231:2251–9. doi: 10.1007/s00213-014-3523-4
24. Gruber SA, Yurgelun-Todd DA. Neuroimaging of marijuana smokers during inhibitory processing: a pilot investigation. Brain Res Cogn Brain Res. (2005) 23:107–18. doi: 10.1016/j.cogbrainres.2005.02.016
25. Hester R, Nestor L, Garavan H. Impaired error awareness and anterior cingulate cortex hypoactivity in chronic cannabis users. Neuropsychopharmacology. (2009) 34:2450–8. doi: 10.1038/npp.2009.67
26. Becker B, Wagner D, Gouzoulis-Mayfrank E, Spuentrup E, Daumann J. The impact of early-onset cannabis use on functional brain correlates of working memory. Prog Neuropsychopharmacol Biol Psychiatry. (2010) 34:837–45. doi: 10.1016/j.pnpbp.2010.03.032
27. Wesley MJ, Hanlon CA, Porrino LJ. Poor decision-making by chronic marijuana users is associated with decreased functional responsiveness to negative consequences. Psychiatry Res. (2011) 191:51–9. doi: 10.1016/j.pscychresns.2010.10.002
28. Riba J, Valle M, Sampedro F, Rodriguez-Pujadas A, Martinez-Horta S, Kulisevsky J, et al. Telling true from false: cannabis users show increased susceptibility to false memories. Mol Psychiatry. (2015) 20:772–7. doi: 10.1038/mp.2015.36
29. Rigucci S, Xin L, Klauser P, Baumann PS, Alameda L, Cleusix M, et al. Cannabis use in early psychosis is associated with reduced glutamate levels in the prefrontal cortex. Psychopharmacology (Berl). (2018) 235:13–22. doi: 10.1007/s00213-017-4745-z
30. Curran HV, Freeman TP, Mokrysz C, Lewis DA, Morgan CJ, Parsons LH. Keep off the grass? Cannabis, cognition and addiction. Nat Rev Neurosci. (2016) 17:293–306. doi: 10.1038/nrn.2016.28
31. Ramaekers JG, Mason NL, Theunissen EL. Blunted highs: Pharmacodynamic and behavioral models of cannabis tolerance. Eur Neuropsychopharmacol. (2020) 36:191–205. doi: 10.1016/j.euroneuro.2020.01.006
32. Ramaekers JG, Mason NL, Kloft L, Theunissen EL. The why behind the high: determinants of neurocognition during acute cannabis exposure. Nat Rev Neurosci. (2021) 22:439–54. doi: 10.1038/s41583-021-00466-4
33. Mason NL, Theunissen EL, Hutten N, Tse DHY, Toennes SW, Stiers P, et al. Cannabis induced increase in striatal glutamate associated with loss of functional corticostriatal connectivity. Eur Neuropsychopharmacol. (2019) 29:247–56. doi: 10.1016/j.euroneuro.2018.12.003
34. Colizzi M, Weltens N, Mcguire P, Lythgoe D, Williams S, Van Oudenhove L, et al. Delta-9-tetrahydrocannabinol increases striatal glutamate levels in healthy individuals: implications for psychosis. Mol Psychiatry. (2020) 25:3231–40. doi: 10.1038/s41380-019-0374-8
35. Mason NL, Theunissen EL, Hutten N, Tse DHY, Toennes SW, Jansen JFA, et al. Reduced responsiveness of the reward system is associated with tolerance to cannabis impairment in chronic users. Addict Biol. (2021) 26:e12870. doi: 10.1111/adb.12870
36. Bossong MG, Van Berckel BN, Boellaard R, Zuurman L, Schuit RC, Windhorst AD, et al. Delta 9-tetrahydrocannabinol induces dopamine release in the human striatum. Neuropsychopharmacology. (2009) 34:759–66. doi: 10.1038/npp.2008.138
37. Stokes PR, Mehta MA, Curran HV, Breen G, Grasby PM. Can recreational doses of THC produce significant dopamine release in the human striatum? Neuroimage. (2009) 48:186–90. doi: 10.1016/j.neuroimage.2009.06.029
38. Stokes PR, Egerton A, Watson B, Reid A, Breen G, Lingford-Hughes A, et al. Significant decreases in frontal and temporal [11C]-raclopride binding after THC challenge. Neuroimage. (2010) 52:1521–7. doi: 10.1016/j.neuroimage.2010.04.274
40. Leroy C, Karila L, Martinot JL, Lukasiewicz M, Duchesnay E, Comtat C, et al. Striatal and extrastriatal dopamine transporter in cannabis and tobacco addiction: a high-resolution PET study. Addict Biol. (2012) 17:981–90. doi: 10.1111/j.1369-1600.2011.00356.x
41. Bloomfield MA, Morgan CJ, Egerton A, Kapur S, Curran HV, Howes OD. Dopaminergic function in cannabis users and its relationship to cannabis-induced psychotic symptoms. Biol Psychiatry. (2014) 75:470–8. doi: 10.1016/j.biopsych.2013.05.027
42. Ramaekers JG, Mason NL, Toennes SW, Theunissen EL, Amico E. Functional brain connectomes reflect acute and chronic cannabis use. Sci Rep. (2022) 12:2449. doi: 10.1038/s41598-022-06509-9
43. Everitt BJ, Robbins TW. Neural systems of reinforcement for drug addiction: from actions to habits to compulsion. Nat Neurosci. (2005) 8:1481–9. doi: 10.1038/nn1579
44. Cadoni C, Valentini V, Di Chiara G. Behavioral sensitization to delta 9-tetrahydrocannabinol and cross-sensitization with morphine: differential changes in accumbal shell and core dopamine transmission. J Neurochem. (2008) 106:1586–93. doi: 10.1111/j.1471-4159.2008.05503.x
45. Zhou X, Zimmermann K, Xin F, Zhao W, Derckx RT, Sassmannshausen A, et al. Cue reactivity in the ventral striatum characterizes heavy cannabis use, whereas reactivity in the dorsal striatum mediates dependent use. Biol Psychiatry Cogn Neurosci Neuroimaging. (2019) 4:751–62. doi: 10.1016/j.bpsc.2019.04.006
46. Vollstadt-Klein S, Wichert S, Rabinstein J, Buhler M, Klein O, Ende G, et al. Initial, habitual and compulsive alcohol use is characterized by a shift of cue processing from ventral to dorsal striatum. Addiction. (2010) 105:1741–9. doi: 10.1111/j.1360-0443.2010.03022.x
47. Sjoerds Z, De Wit S, Van Den Brink W, Robbins TW, Beekman AT, Penninx BW, et al. Behavioral and neuroimaging evidence for overreliance on habit learning in alcohol-dependent patients. Transl Psychiatry. (2013) 3:e337. doi: 10.1038/tp.2013.107
48. Everitt BJ, Robbins TW. Drug addiction: updating actions to habits to compulsions ten years on. Annu Rev Psychol. (2016) 67:23–50. doi: 10.1146/annurev-psych-122414-033457
49. Zhukovsky P, Puaud M, Jupp B, Sala-Bayo J, Alsio J, Xia J, et al. Withdrawal from escalated cocaine self-administration impairs reversal learning by disrupting the effects of negative feedback on reward exploitation: a behavioral and computational analysis. Neuropsychopharmacology. (2019) 44:2163–73. doi: 10.1038/s41386-019-0381-0
50. Robinson TE, Berridge KC. The neural basis of drug craving: an incentive-sensitization theory of addiction. Brain Res Brain Res Rev. (1993) 18:247–91. doi: 10.1016/0165-0173(93)90013-p
51. Robinson TE, Berridge KC. Incentive-sensitization and addiction. Addiction. (2001) 96:103–14. doi: 10.1046/j.1360-0443.2001.9611038.x
52. Berridge KC, Robinson TE. Liking, wanting, and the incentive-sensitization theory of addiction. Am Psychol. (2016) 71:670–9. doi: 10.1037/amp0000059
53. Filbey FM, Schacht JP, Myers US, Chavez RS, Hutchison KE. Marijuana craving in the brain. Proc Natl Acad Sci USA. (2009) 106:13016–21. doi: 10.1073/pnas.0903863106
54. Cousijn J, Goudriaan AE, Ridderinkhof KR, Van Den Brink W, Veltman DJ, Wiers RW. Neural responses associated with cue-reactivity in frequent cannabis users. Addict Biol. (2013) 18:570–80. doi: 10.1111/j.1369-1600.2011.00417.x
55. Filbey FM, Dunlop J, Ketcherside A, Baine J, Rhinehardt T, Kuhn B, et al. fMRI study of neural sensitization to hedonic stimuli in long-term, daily cannabis users. Hum Brain Mapp. (2016) 37:3431–43. doi: 10.1002/hbm.23250
56. De Sousa Fernandes Perna EB, Theunissen EL, Kuypers KP, Evers EA, Stiers P, Toennes SW, et al. Brain reactivity to alcohol and cannabis marketing during sobriety and intoxication. Addict Biol. (2017) 22:823–32. doi: 10.1111/adb.12351
57. Jansma JM, Van Hell HH, Vanderschuren LJ, Bossong MG, Jager G, Kahn RS, et al. THC reduces the anticipatory nucleus accumbens response to reward in subjects with a nicotine addiction. Transl Psychiatry. (2013) 3:e234. doi: 10.1038/tp.2013.6
58. Freeman TP, Pope RA, Wall MB, Bisby JA, Luijten M, Hindocha C, et al. Cannabis Dampens the Effects of Music in Brain Regions Sensitive to Reward and Emotion. Int J Neuropsychopharmacol (2018) 21:21–32. doi: 10.1093/ijnp/pyx082
59. Kesner AJ, Lovinger DM. Cannabis use, abuse, and withdrawal: Cannabinergic mechanisms, clinical, and preclinical findings. J Neurochem. (2021) 157:1674–96. doi: 10.1111/jnc.15369
60. Hirvonen J, Goodwin R, Li C-T, Terry G, Zoghbi S, Morse C, et al. Reversible and regionally selective downregulation of brain cannabinoid CB 1 receptors in chronic daily cannabis smokers. Mol Psychiatry. (2012) 17:642–9. doi: 10.1038/mp.2011.82
61. Ceccarini J, Kuepper R, Kemels D, Van Os J, Henquet C, Van Laere K. [18F]MK-9470 PET measurement of cannabinoid CB1 receptor availability in chronic cannabis users. Addict Biol. (2015) 20:357–67. doi: 10.1111/adb.12116
62. D’souza DC, Cortes-Briones JA, Ranganathan M, Thurnauer H, Creatura G, Surti T, et al. Rapid changes in CB1 receptor availability in cannabis dependent males after abstinence from cannabis. Biol Psychiatry Cogn Neurosci Neuroimaging. (2016) 1:60–7. doi: 10.1016/j.bpsc.2015.09.008
63. Pope HG Jr., Gruber AJ, Hudson JI, Huestis MA, Yurgelun-Todd D. Neuropsychological performance in long-term cannabis users. Arch Gen Psychiatry. (2001) 58:909–15. doi: 10.1001/archpsyc.58.10.909
64. Bosker WM, Karschner EL, Lee D, Goodwin RS, Hirvonen J, Innis RB, et al. Psychomotor function in chronic daily cannabis smokers during sustained abstinence. PLoS One. (2013) 8:e53127. doi: 10.1371/journal.pone.0053127
65. Lorenzetti V, Solowij N, Yucel M. The role of cannabinoids in neuroanatomic alterations in cannabis users. Biol Psychiatry. (2016) 79:e17–31. doi: 10.1016/j.biopsych.2015.11.013
66. Schreiner AM, Dunn ME. Residual effects of cannabis use on neurocognitive performance after prolonged abstinence: a meta-analysis. Exp Clin Psychopharmacol. (2012) 20:420–9. doi: 10.1037/a0029117
67. Colizzi M, Bhattacharyya S. Cannabis use and the development of tolerance: a systematic review of human evidence. Neurosci Biobehav Rev. (2018) 93:1–25. doi: 10.1016/j.neubiorev.2018.07.014
68. Gilman JM, Schmitt WA, Potter K, Kendzior B, Pachas GN, Hickey S, et al. Identification of 9-tetrahydrocannabinol (THC) impairment using functional brain imaging. Neuropsychopharmacology. (2022) 47:944–52. doi: 10.1038/s41386-021-01259-0
69. Cox SM, Benkelfat C, Dagher A, Delaney JS, Durand F, Mckenzie SA, et al. Striatal dopamine responses to intranasal cocaine self-administration in humans. Biol Psychiatry. (2009) 65:846–50. doi: 10.1016/j.biopsych.2009.01.021
70. Schmidt HD, Pierce RC. Cocaine-induced neuroadaptations in glutamate transmission: potential therapeutic targets for craving and addiction. Ann N Y Acad Sci. (2010) 1187:35–75. doi: 10.1111/j.1749-6632.2009.05144.x
71. Orsini CA, Colon-Perez LM, Heshmati SC, Setlow B, Febo M. Functional connectivity of chronic cocaine use reveals progressive neuroadaptations in neocortical, striatal, and limbic networks. eNeuro (2018) 5:ENEURO.81–18.
72. Janes AC, Nickerson LD, Frederick Bde B, Kaufman MJ. Prefrontal and limbic resting state brain network functional connectivity differs between nicotine-dependent smokers and non-smoking controls. Drug Alcohol Depend. (2012) 125:252–9. doi: 10.1016/j.drugalcdep.2012.02.020
73. Fedota JR, Stein EA. Resting-state functional connectivity and nicotine addiction: prospects for biomarker development. Ann N Y Acad Sci. (2015) 1349:64–82. doi: 10.1111/nyas.12882
74. Huang W, Tam K, Fernando J, Heffernan M, King J, Difranza JR. Nicotine and resting-state functional connectivity: effects of intermittent doses. Nicotine Tob Res. (2015) 17:1311–7. doi: 10.1093/ntr/ntv009
75. Okita K, Mandelkern MA, London ED. Cigarette use and striatal dopamine D2/3 receptors: possible role in the link between smoking and nicotine dependence. Int J Neuropsychopharmacol. (2016) 19:yw074. doi: 10.1093/ijnp/pyw074
76. Wills L, Kenny PJ. Addiction-related neuroadaptations following chronic nicotine exposure. J Neurochem. (2021) 157:1652–73. doi: 10.1111/jnc.15356
77. Vergara VM, Hutchison K, Calhoun VD. Increased randomness of functional network connectivity in nicotine and alcohol consumers. Annu Int Conf IEEE Eng Med Biol Soc. (2018) 2018:1011–4. doi: 10.1109/EMBC.2018.8512392
78. Han J, Keedy S, Murray CH, Foxley S, De Wit H. Acute effects of alcohol on resting-state functional connectivity in healthy young men. Addict Behav. (2021) 115:106786. doi: 10.1016/j.addbeh.2020.106786
79. Yang X, Meng YJ, Tao YJ, Deng RH, Wang HY, Li XJ, et al. Functional connectivity of nucleus accumbens and medial prefrontal cortex with other brain regions during early-abstinence is associated with alcohol dependence and relapse: a resting-functional magnetic resonance imaging study. Front Psychiatry. (2021) 12:609458. doi: 10.3389/fpsyt.2021.609458
80. Spitta G, Gleich T, Zacharias K, Butler O, Buchert R, Gallinat J. Extrastriatal dopamine D2/3 receptor availability in alcohol use disorder and individuals at high risk. Neuropsychobiology. (2022) 11:1–10. doi: 10.1159/000521103
81. Ramaekers J, Van Wel J, Spronk D, Franke B, Kenis G, Toennes S, et al. Cannabis and cocaine decrease cognitive impulse control and functional corticostriatal connectivity in drug users with low activity DBH genotypes. Brain Imaging Behav. (2016) 10:1254–63. doi: 10.1007/s11682-015-9488-z
Keywords: cannabis, neuroadaptation, neurocognition, mesocorticolimbic circuit, cannabis abuse
Citation: Ramaekers JG, Theunissen EL, van Ruitenbeek P and Mason NL (2022) Cannabis Use and Neuroadaptation: A Call for Δ9-Tetrahydrocannabinol Challenge Studies. Front. Psychiatry 13:870750. doi: 10.3389/fpsyt.2022.870750
Received: 07 February 2022; Accepted: 18 February 2022;
Published: 14 April 2022.
Edited by:
Danilo De Gregorio, Vita-Salute San Raffaele University, ItalyReviewed by:
Jodi M. Gilman, Massachusetts General Hospital and Harvard Medical School, United StatesCopyright © 2022 Ramaekers, Theunissen, van Ruitenbeek and Mason. This is an open-access article distributed under the terms of the Creative Commons Attribution License (CC BY). The use, distribution or reproduction in other forums is permitted, provided the original author(s) and the copyright owner(s) are credited and that the original publication in this journal is cited, in accordance with accepted academic practice. No use, distribution or reproduction is permitted which does not comply with these terms.
*Correspondence: Johannes G. Ramaekers, ai5yYW1hZWtlcnNAbWFhc3RyaWNodHVuaXZlcnNpdHkubmw=