- 1Doctorado en Ciencias Biológicas y de la Salud, Universidad Autónoma Metropolitana, Mexico City, Mexico
- 2Laboratorio de Genómica de Enfermedades Psiquiátricas y Neurodegenerativas, Instituto Nacional de Medicina Genómica, Mexico City, Mexico
- 3Departamento de Sistemas Biológicos, Universidad Autónoma Metropolitana, Mexico City, Mexico
- 4Departamento de Genética, Instituto Nacional de Neurología y Neurocirugía Manuel Velasco Suárez, Mexico City, Mexico
- 5Departamento de Psiquiatría, Instituto Nacional de Neurología y Neurocirugía Manuel Velasco Suárez, Mexico City, Mexico
- 6Dirección General, Hospital Psiquiátrico Infantil Juan N Navarro, Mexico City, Mexico
- 7Departamento de Ciencias Naturales, Universidad Autónoma Metropolitana, Unidad Cuajimalpa, Mexico City, Mexico
- 8División Académica de Ciencias de la Salud, Universidad Juárez Autónoma de Tabasco, Villahermosa, Mexico
- 9División Académica Multidisciplinaria de Comalcalco, Universidad Juárez Autónoma de Tabasco, Comalcalco, Mexico
- 10División Académica Multidisciplinaria de Jalpa de Méndez, Universidad Juárez Autónoma de Tabasco, Jalpa de Méndez, Mexico
- 11Grupo de Estudios Médicos y Familiares Carracci, Mexico City, Mexico
Long-term studies have shown significantly lower mortality rates in patients with continuous clozapine (CLZ) treatment than other antipsychotics. We aimed to evaluate epigenetic age and DNA methylome differences between CLZ-treated patients and those without psychopharmacological treatment. The DNA methylome was analyzed using the Infinium MethylationEPIC BeadChip in 31 CLZ-treated patients with psychotic disorders and 56 patients with psychiatric disorders naive to psychopharmacological treatment. Delta age (Δage) was calculated as the difference between predicted epigenetic age and chronological age. CLZ-treated patients were stratified by sex, age, and years of treatment. Differential methylation sites between both groups were determined using linear regression models. The Δage in CLZ-treated patients was on average lower compared with drug-naive patients for the three clocks analyzed; however, after data-stratification, this difference remained only in male patients. Additional differences were observed in Hannum and Horvath clocks when comparing chronological age and years of CLZ treatment. We identified 44,716 differentially methylated sites, of which 87.7% were hypomethylated in CLZ-treated patients, and enriched in the longevity pathway genes. Moreover, by protein–protein interaction, AMPK and insulin signaling pathways were found enriched. CLZ could promote a lower Δage in individuals with long-term treatment and modify the DNA methylome of the longevity-regulating pathways genes.
Introduction
There is evidence of premature mortality and an increase in physical comorbidity in patients with psychiatric disorders (1). On average, their life expectancy is reduced from 12 to 15 years compared with the general population (2, 3). Some authors have proposed that the increased mortality could correlate with increased biological aging (4–6). Various epigenetic age estimators of biological age have been developed based on DNA methylation status across a set of CpG sites in cells/tissues, and these have been shown to correlate with the risk of developing age-related conditions and diseases (7–9). Moreover, the presence of psychotic symptoms is observed in different psychiatric illnesses (mainly schizophrenia and bipolar disorder), contributing to this mortality. A recent study reported a positive correlation between epigenetic aging and psychosis severity (10). Fortunately, psychotic symptoms can be controlled with the use of antipsychotics, which also help in reducing the risk of psychotic relapse (11).
On the other hand, the adverse cardiac and metabolic effects of antipsychotics are well known. However, their use in patients with schizophrenia is associated with lower mortality (12). There is evidence demonstrating a 30–50% lower risk of mortality related to the use of antipsychotics compared with non-use (13, 14). In particular, the long-term use of clozapine (CLZ) has been associated with the lowest mortality (12, 15, 16). CLZ is the antipsychotic of choice for patients with refractory psychosis (17), for reducing suicide, and for improving longevity (18). Epidemiological data (19, 20) have supported this last point. Some studies suggest that CLZ promotes epigenetic changes with pro-longevity effects (21, 22), and a recent work has proposed that CLZ may reduce epigenetic aging (23).
The present work aimed to evaluate epigenetic age and DNA methylome differences between patients treated with CLZ and psychiatric patients without psychopharmacological treatment.
Materials and Methods
Sample Population
In this study, 87 individuals of Mexican ascendancy were included. Of them, 31 were diagnosed with refractory psychosis and treated with CLZ (CLZ-treated patients), and 56 had early onset psychiatric disorders. This last group of patients has not had any previous psychopharmacological treatment and will be called as drug-naive patients. Samples from both groups of patients were recruited from the outpatient clinics. The CLZ-treated patients with refractory psychosis were recruited from the Instituto Nacional de Neurología y Neurocirugía Manuel Velasco Suárez (INNNMVS), and drug-naive patients from the Hospital Psiquiátrico Infantil Juan N. Navarro and the Hospital General Dr. Gustavo Rivorosa Pérez. At least one specialized psychiatrist performed the diagnosis. Individuals with intellectual disabilities, heavy drinkers, smokers, or substance abusers were excluded. In addition, we excluded drug-naive patients who had begun psychopharmacological treatment. The study was conducted according to the guidelines of the Declaration of Helsinki and approved by the Institutional Research and Bioethics Committees of INNNMVS (protocol 38/19), the Ethics Committee of the Children’s Psychiatric Hospital Dr. Juan N. Navarro with approval No. II3/01/0913 (11 October 2017), and by the Ethics Committee of the National Institute of Genomic Medicine (INMEGEN) with approval No. 06/2018/I. Furthermore, all individuals signed informed consents. For those patients under the age of 18 years, an informed assent letter was signed by both parents/guardians and the minor.
Analysis and Quality Control of Microarrays
Blood DNA extraction, bisulfite-conversion (Zymo, Irvine, CA, United States), and microarray hybridization (Infinium MethylationEPIC BeadChip, Illumina, San Diego, CA, United States) were performed according to the manufacturer’s protocol. We used the Genome Reference Consortium Human Build 37 (GRCh37/hg19) as position reference for all the analyses. The fluorescence intensities were measured with the iScan instrument and transformed into idat files with the algorithm implemented in the GenomeStudio software (Illumina, San Diego, CA, United States). Specialized staff carried out microarray analyses in the INMEGEN. The ChAMP (24) package was used for quality control. Briefly, the probes using the following filters were removed: (i) detection p greater than 0.01, (ii) less than three beads in less than 5% of the samples, (iii) all non-CpG sites, (iv) single nucleotide polymorphism (SNP)-associated probes, (v) probes associated with sex chromosomes, and (vi) multi-hit probes. In addition, samples with a ratio greater than 0.1 were removed, resulting in one individual filtered out. After quality control, a matrix of beta values was constructed.
Epigenetic Age Calculation
We normalized the previous beta value matrix by beta-mixture quantile normalization (25) and calculated epigenetic ages with the ENmix package (26). This package allowed us to calculate three epigenetic clocks: Hannum (7), Horvath (8), and DNAm PhenoAge (9). The epigenetic ages calculated with the Horvath, Hannum, and PhenoAge clocks and the chronological age in both groups were compared by correlation analysis. Then, we calculated the delta of age (Δage = epigenetic age − chronological age), subtracting the chronological age from the estimated epigenetic age in all samples and by each clock. Finally, we compared the Δage of each clock between CLZ-treated patients and drug-naive patients. First, stratified analysis by sex and age was carried out, comparing the CLZ-treated patients vs. drug-naive patients. The CLZ-treated patients’ group was later subdivided into subgroups according to the time’s mean of treatment with CLZ (>9 and ≤ 9 years of treatment; i.e., few and many years of treatment, respectively) and according to the mean chronological age (cut-off value = 37 years; i.e., young and older patients). Due to the small sample size, and to achieve subgroups with a similar number of patients (n), the mean values of the years of CLZ treatment and chronological age of patients were used. The student’s t-test was applied for the analysis of these four subgroups.
Differentially Methylation Sites Analysis
We removed the batch effect and adjusted blood cell proportion on the normalized beta values matrix with a single-value deconvolution (champ.SVD and champ.runCombat) and a reference base analysis (champ.refbase), respectively (24, 27). Once adjusted, we analyzed differentially methylated sites analysis by linear models implemented in champ.DMP function of the ChAMP package (28). First, we analyzed differentially methylated sites considering significance at a p lower than 1e-08 (a summary of statistics is available in Supplementary Table 1). Then, after identifying differentially methylated sites, a differentially methylated region analysis was carried out with the ProbeLasso algorithm (29) (a summary statistics of the differential methylation region analysis is available in Supplementary Table 2).
Pathway Enrichment and Protein-Protein Interactions
We performed pathway enrichment after extracting genes resulting from differentially methylated sites and regions through the WebGestalt platform (WEB-based GEne SeT AnaLysis Toolkit) with free access (30) (all pathways of this enrichment are available on Supplementary Table 3). First, we analyzed protein–protein interactions between gene products identified using the web application String (31) and plotted the interactions with Cytoscape 3.8.1 software (32). Then, a sub-enrichment analysis of the longevity regulating pathway was performed in String using the KEGG pathway database (33).
Results
Study Population
In this study, eighty-seven individuals were included and classified into two groups: the CLZ-treated patients with refractory psychosis (n = 31), and the drug-naive patients with psychiatric disorders (n = 56) (Table 1). The following comorbidities were observed in CLZ-treated patients: diabetes (22.6%), epilepsy (16.1%), hyperlipidemia (12.9%), hypercholesterolemia (6.4%), hypothyroidism (6.4%), hypertension (9.7%), and obsessive-compulsive disorder (3.2%). Details about the comorbidities found in the naive patients have been previously reported (34).
Epigenetic Age in Clozapine-Treated Patients vs. Drug-Naive Patients
The variable sex did not show a significant correlation with epigenetic age; however, it was considered in the model as previous reports have demonstrated differences in the epigenetic age between men and women (23). The years of CLZ treatment correlated with epigenetic age in the PhenoAge clock (t = 2.6041, r2 = 0.4415, p = 0.01458); thus, these two variables were included in the stratification of the patients. The chronological age positively correlated with the epigenetic age in all clocks, Hannum (r2 = 0.679, p = 4.96e-13), Horvath (r2 = 0.662, p = 2.85e-12), and PhenoAge (r2 = 0.664, p-value = 2.26e-12) (Figure 1). After obtaining the Δage for all samples, we found that the CLZ-treated patients’ group had a smaller Δage compared with drug-naive patients (Figure 2). This difference was significant for the three analyzed clocks: Horvath (t = −3.23, p = 0.0018), Hannum (t = −4.83, p = 6.0740e-06), and PhenoAge (t = −2.72, p = 0.0079). Hannum’s clock showed the most significant value. The mean Δage in the CLZ-treated patients was 13.64, 9.65, and 1.54 years for Horvath, Hannum, and PhenoAge clocks, respectively. In drug-naive patients, the mean Δage was 20.77, 18.84, and 8.15 years in the same mentioned order for the three clocks.
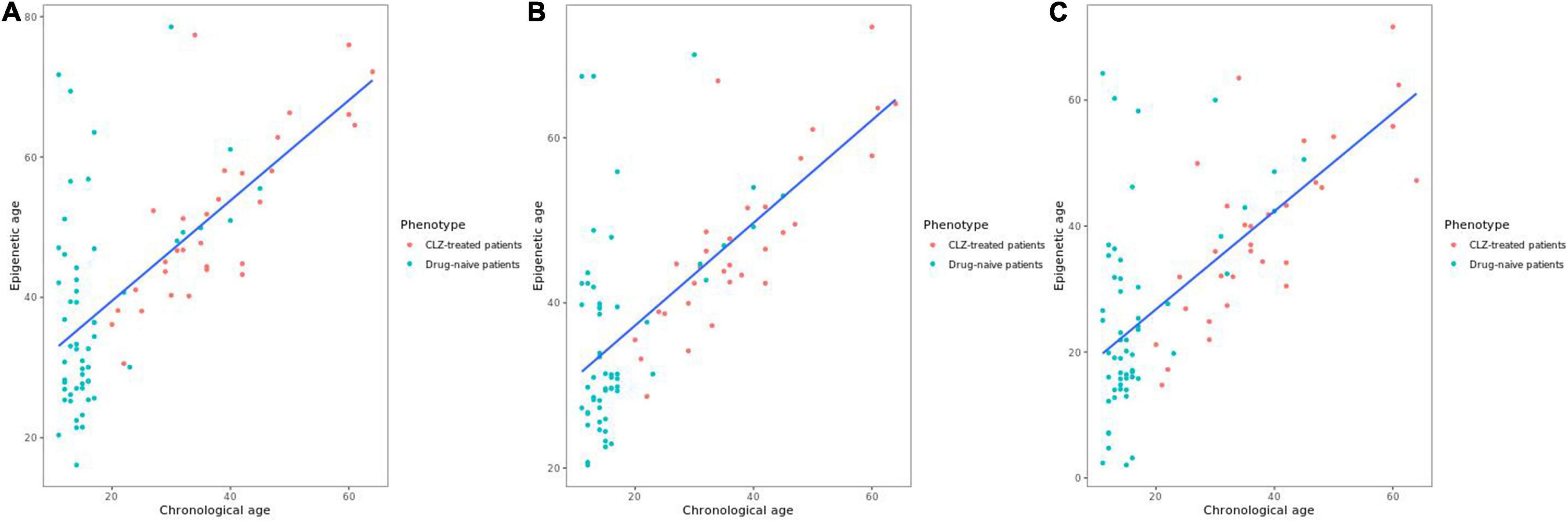
Figure 1. Correlation between chronological age and epigenetic age. (A) Correlation with Horvath’s clock. (B) Correlation with Hannum’s clock. (C) Correlation with PhenoAge’s clock.
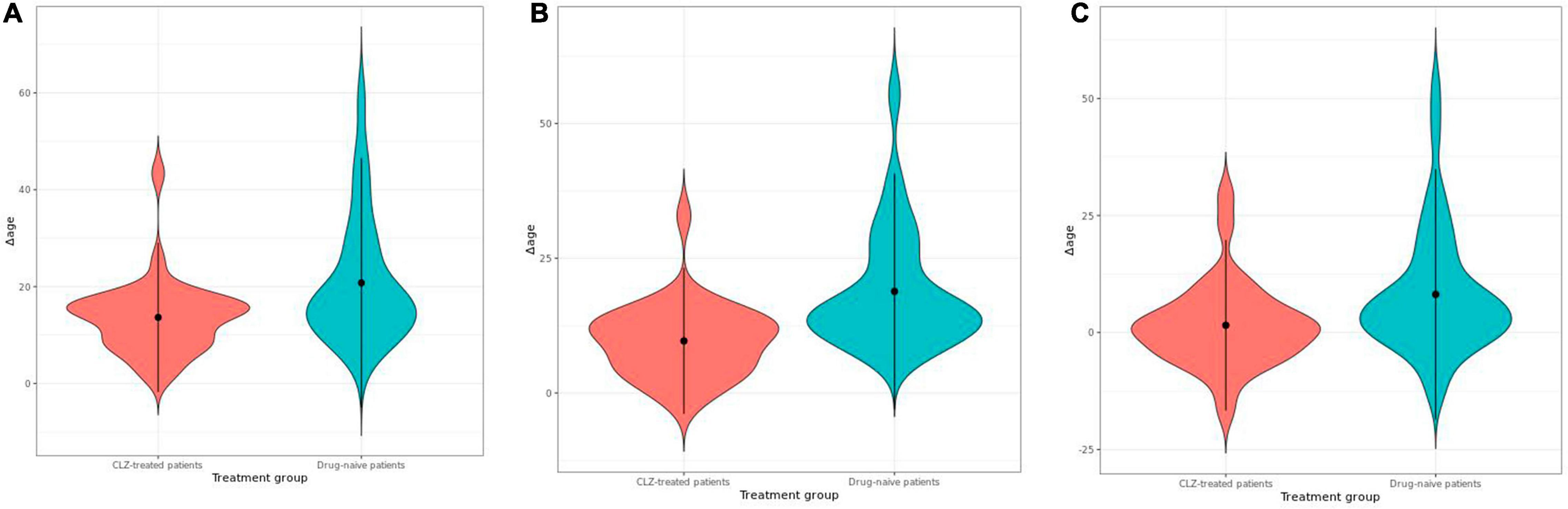
Figure 2. Violin plots showing the comparison of Δage between clozapine (CLZ)-treated patients and drug-naive patients estimated by using epigenetic clocks of (A) Horvath, (B) Hannum, and (C) PhenoAge.
The stratified analysis by sex and age, after comparing CLZ-treated patients vs. drug-naive patients, showed significant differences in the three clocks only for CLZ-treated male patients with mean Δage values of 13.15, 10.07, and 1.60 years for the Horvath, Hannum, and PhenoAge clocks, respectively, showing corresponding values of p of 0.0376, 0.0073, and 0.0370 (Supplementary Figure 1A). The mean Δage in CLZ-treated female patients was 14.30, 9.06, and 1.47 years for the Horvath, Hannum, and PhenoAge clocks, respectively. However, significant differences were only found in Hannum’s clock (t = −3.2253, p = 0.0035). Thus, we did not identify differences between age subgroups (Supplementary Figure 1B).
Comparison of Δage in Older and Younger Patients Treated With Clozapine
We explored Δage differences in CLZ-treated patients, subdivided according to the mean value of chronological age (37.74 years) and the mean treatment time with CLZ (9.22 years). Significant differences of Δage for the Horvath and Hanum clocks were observed between patients by years of CLZ treatment and chronological age (younger vs. older patients, and few years of treatment vs. many years of treatment, respectively). In addition, other significant differences were found for the Hannum clock in the following comparisons: older patients with more years of treatment (>9.22 years) vs. younger patients with more years of treatment (p = 0.0021); older patients with many years of treatment vs. young patients with few years of treatment (p = 0.0263); and older patients with few years of treatment vs. young patients with many years of treatment (p = 0.0236) (Figure 3A). Regarding Horvath’s clock, we only observed differences between the group of older patients with many years of treatment and younger patients with many years of treatment (p = 0.019) (Figure 3B).
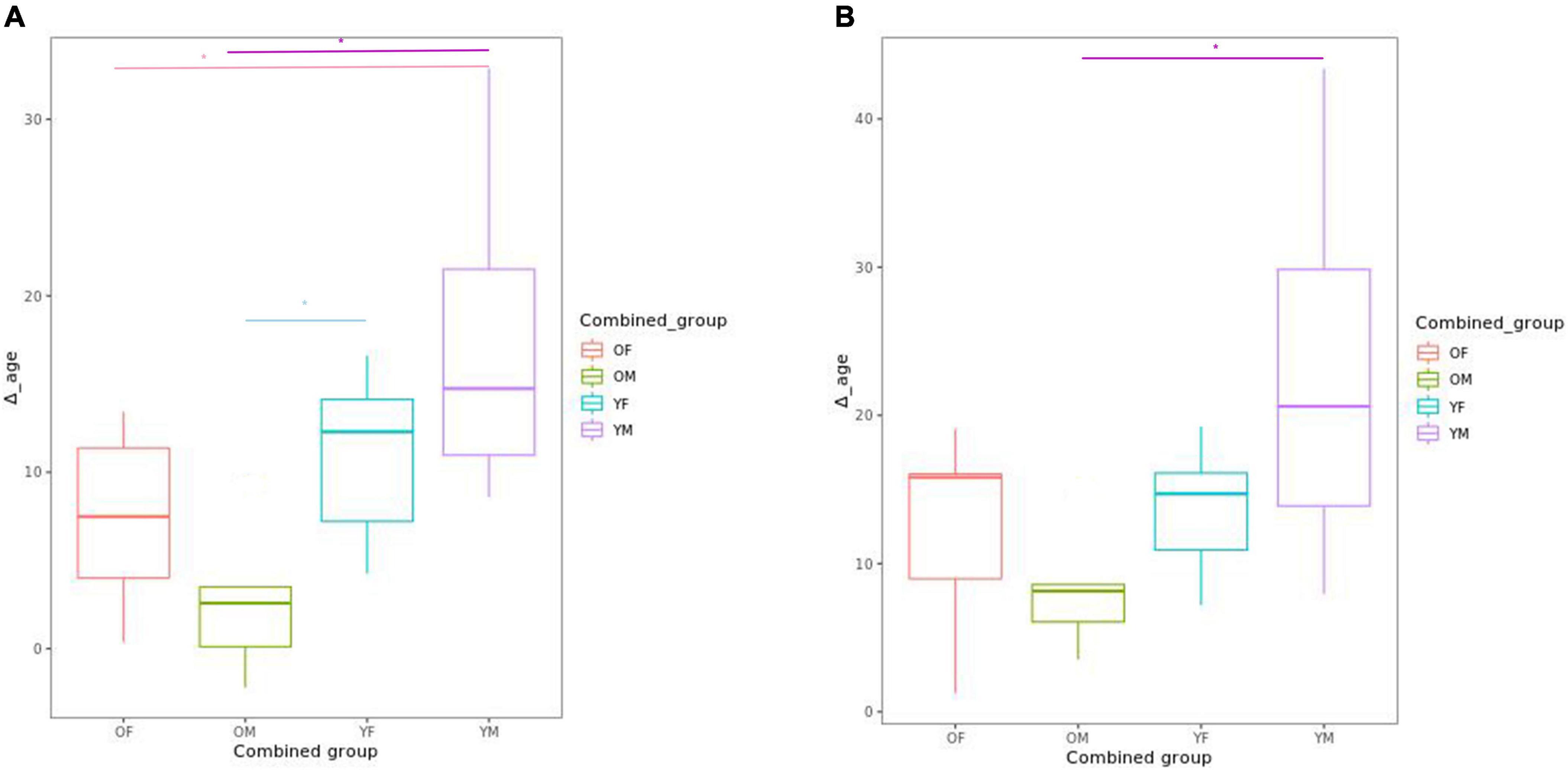
Figure 3. Box plot showing the comparison of delta age (Δage) using (A) Hannum’s clock and (B) Horvath’s clock in the four groups generated according to the mean values of chronological age and years of CLZ treatment. Differences are observed between young patients with more or a few years of treatment vs. older patients with many years of treatment. The four groups analyzed were: young patients with few years of treatment (YF); young patients with many years of treatment (YM); older patients with few years of treatment (OF), and older patients with many years of treatment (OM). The asterisk (*) denotes significant differences.
Differentially Methylated Sites
We identified 44,716 differentially methylated sites between CLZ-treated patients and drug-naive patients. Of note, 39,204 CpG sites (87.70%) were hypomethylated in the CLZ-treated patients, and the remaining (5,512 CpG sites, 12.30%) were hyper-methylated.
According to the position of the differentially methylated sites in relation to the gene structure, the following distribution was observed: 9,573 (24.42%) hypomethylated sites were located at 200 nucleotides of the transcriptional start site (TSS200), 7,247 (18.49%) sites on TSS1500, 7,126 (18.18%) on gene body, 5,332 (13.6%) on 5′-UTR, 4,626 (11.8%) on first exon, 306 (0.78%) on 3′-UTR, and 22 (0.06%) on exon binding sites. The distribution of the hypermethylated sites was as follows: 3,111 (56.44%) sites were annotated to gene body, 266 (4.83%) on 5′-UTR, 265 (4.81%) on TSS1500, 262 (4.75%) on 3′- UTR, 138 (2.5%) were located on the TSS200, 75 (1.36%) on first exon, and 55 (0.1%) on exon binding sites.
The location of differentially methylated sites concerning CpG islands was as follows: 29,895 (66.86%) sites on CpG islands, 7,860 sites (17.56%) on shores, 5,808 sites (12.99%) on the open sea, and 1,153 sites (2.58%) on shelves. Regarding hypomethylated sites, 29,020 of them (74%) were found on CpG islands, 6,822 (17.4%) on shores, 2,892 sites (7.38%) on the open sea, and 470 (1.2%) sites on shelves. The distribution of the hypermethylated sites was: 2,916 (52.9%) sites located on the open sea, 1,038 (18.83%) sites on shores, 875 (15.87%) sites on CpG islands, and 683 (12.39%) sites on shelves.
Enrichment of the Genes Located Within the Differentially Methylated Sites
In total, sixty-five pathways enriched at the hypomethylated sites were identified, and the main ones were involved in the biosynthesis of glycosaminoglycan, glioma, longevity regulatory pathway, VEGF signaling pathway, and circadian rhythm. Additionally, the hypermethylated sites are enriched on ABC transporters and endocytosis pathways (Table 2). Supplementary Table 2 contains the enrichment of all the observed pathways. Among the enriched pathways at the hypomethylated sites, the longevity pathway was identified, which, in turn, interacts with others, such as the AMPK and the insulin signaling pathways. The longevity regulating pathway occupied the 21st place of the ranked list generated by the pathway enrichment; it showed an overlap of 74 genes out of an expected 89 [p = 1.25e-7, false discovery rate (FDR) = 1.14e-6, and enrichment ratio = 1.4578]. We sub-analyzed the longevity regulating pathway because CLZ has been associated with a reduced mortality risk compared with other antipsychotics. For this purpose, a sub-enrichment analysis using protein–protein interaction on this pathway (Figure 4) was performed.
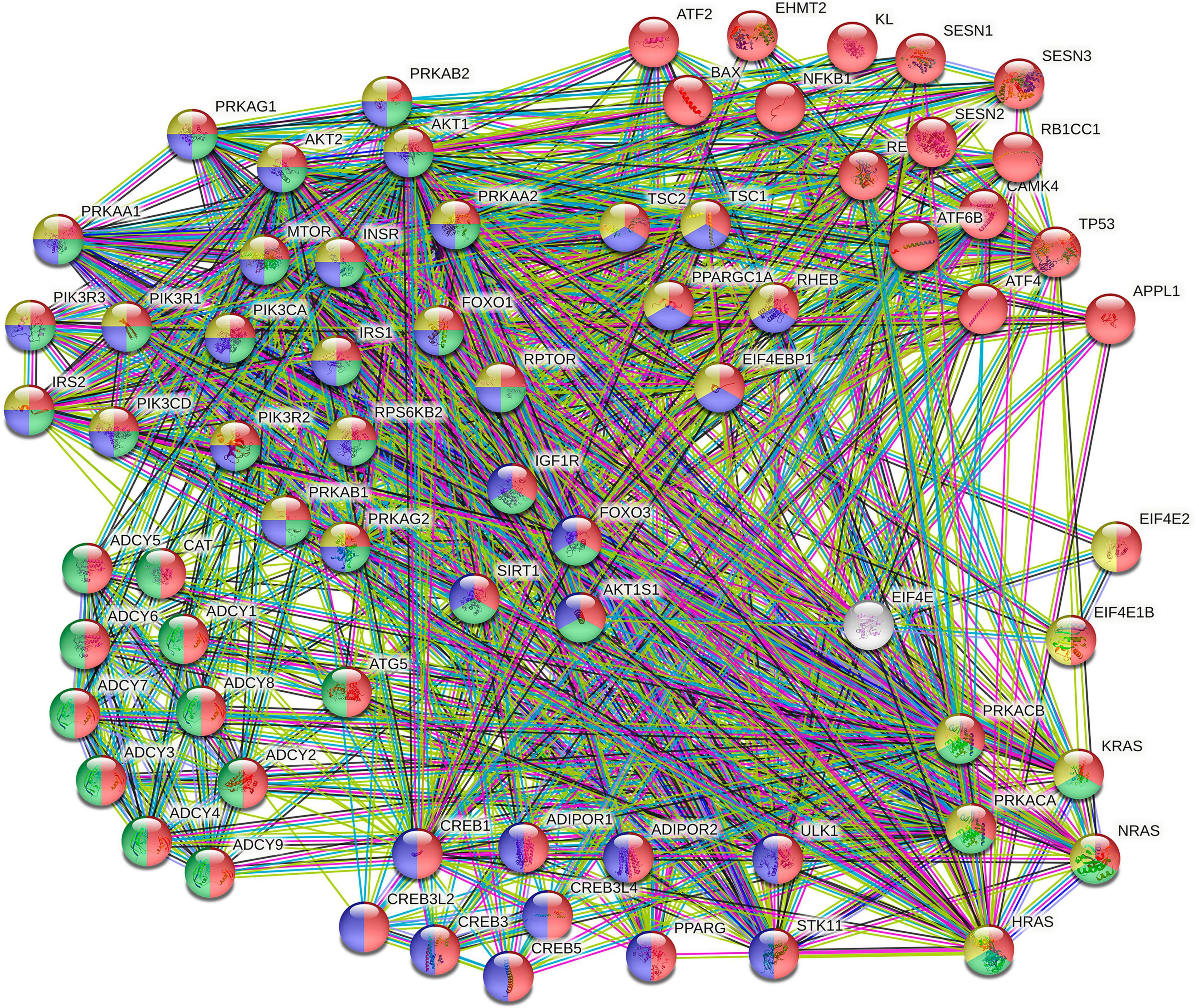
Figure 4. Protein–protein interaction between gene products of the longevity regulating pathway. Clusters are colored based on the Search Tool for Retrieval of Interacting Genes/Proteins (STRING) analysis. The proteins involved in the longevity regulating pathway are highlighted in red and green colors (KEGG pathways: hsa04211 and hsa04213, respectively). In contrast, AMPK signaling proteins (hsa04152) and the insulin signaling pathway proteins (hsa04910) are shown in blue and yellow colors, respectively.
Differentially Methylated Regions
A total of 286 differentially methylated regions were identified, of which 247 regions were hypomethylated and 39 hypermethylated. Then, the FUMA platform [Functional Mapping and Annotation of Genome-Wide Association Studies (GWAS)] (35) was used to prioritize and interpret GWAS results of the 307 annotated genes in this region. This analysis revealed top genes, such as NOTCH4, MICA, TRIM27, PBX2, and FKBPL, as enriched in GWAS of schizophrenia, CLZ-induced agranulocytosis/granulocytopenia in treatment-resistant schizophrenia, and bipolar disorder among other psychiatric and non-psychiatric conditions (Supplementary Figure 2). In this enrichment analysis of the differentially methylated regions, we did not find any pathway with a statistically significant association; nevertheless, in the nominal association, twelve pathways were identified (Supplementary Figure 3).
Discussion
Various analyses of DNA methylome in CLZ-treated patients have been documented in European (36, 37) and Asiatic populations (38, 39). Herein, we report the first differential methylation analysis in a Latin American population between CLZ-treated patients with refractory psychosis and drug-naive patients with psychiatric disorders. Our results showed that the proportion of hypomethylated CpG sites (87.7%) was much higher than hypermethylated ones (12.3%) in the CLZ-treated group. This finding agrees with previous studies that found a higher proportion of hypomethylated sites in patients with refractory schizophrenia treated with CLZ (40), and an increase in the global DNA hypomethylation in leukocytes obtained from patients with schizophrenia who received CLZ (41). Additional studies have reported that CLZ induces DNA demethylation at the level of specific genes; for instance, evidence suggests that CLZ may attenuate the dysregulation of GABAergic and glutamatergic transmission by reducing gene promoter hypermethylation (42, 43). These findings raise CLZ as a potential drug that deserves further investigation and, based on the screening of new potential targets in CLZ, appears to be a promising strategy for drug repurposing.
On the other hand, our results showed that patients with psychosis treated with CLZ had a lower Δage than those without psychopharmacological treatment. In addition, we found a significant reduction in the epigenetic age for the three studied clocks in the group of male patients; in this regard, a previous study observed the same effect of CLZ in reducing epigenetic age in male patients (23). Although the authors studied a larger sample of patients with schizophrenia in that study, they included very few CLZ users and did not perform differential methylation analysis. In this sense, the previous findings are consistent with ours. They suggested that treatment with CLZ could reduce epigenetic aging and directly impact the mortality of individuals treated with this antipsychotic.
Collectively, one of the pathways found to be hypomethylated was the estrogen signaling pathway, including the gene encoding for the estrogen receptor 1 (ESR1) (Figure 3). ESR1 is highly involved in estrogen metabolism, and differences in the frequency of some of its genetic variants have been associated with longevity in humans (44, 45). The DNA methylation levels in this gene may explain the difference in epigenetic age reduction only in men. Estrogen levels in a mouse model treated with 17-alpha estradiol affected metabolic parameters and delayed aging in male mice (46, 47). If CLZ induces DNA hypomethylation, it will increase ESR1 gene expression and estrogen sensitivity, which could fit in a hypothesis behind the significant epigenetic age reduction observed in our study. The longevity regulating pathway also appeared to be potentially involved in CLZ-induced epigenetic age changes. This is in line with epidemiological studies and a meta-analysis by Vermeulen et al. (48), which have reported that the long-term CLZ treatment is associated with an about 40% lower all-cause mortality compared with other antipsychotics (12, 15, 16, 49). In addition, we were able to identify enrichment of the membrane-bound adenylyl cyclase enzymes (also known as adenylate cyclases) in this pathway. Contradictorily, the hypomethylation of ADCYs genes would have a similar action to that reported when these genes are inhibited. For instance, the Adcy5 knockout mouse lives longer and is used as a model of longevity (50–54). Taken together, our findings suggest that the regulation of the longevity pathway by CLZ action may promote epigenetic changes that need to be further explored. Interestingly, another group of genes found in the protein–protein interaction network analysis was related to AMP-activated protein kinases. The activation of this pathway has also been associated with longevity and aging delay (55–59).
Clozapine exhibits unique benefits for ameliorating symptoms in patients with treatment-resistant psychosis, for reducing suicide, and for improving longevity (18). This last characteristic has been observed in some epidemiological studies (14, 19, 20, 49), and in a recent study using age classifiers to drug- induced transcriptomes that identified several geroprotectors, such as CLZ (60). Thus, despite lethal adverse reactions of CLZ, this molecule may have pro-longevity effects that should be further investigated.
Some limitations of this study should be considered. First, our sample size is small, however, this is the first study of CLZ effect on DNA methylome in Latin American patients with psychosis, and the number of CLZ-users included in other reports is still restricted and similar in numbers to ours (which is partly due to the misperception that CLZ is a dangerous therapeutic agent). Despite this, some of our results confirm previous findings from other recent studies. Second, our drug-naive patients had different psychiatric disorders and were younger than CLZ-treated patients’ group, which might influence the observed findings. Third, this is a cross-sectional study performed in a peripheral tissue, in which we did not evaluate other variables that may modulate epigenetic aging, such as prior pharmacological interventions or concomitant to CLZ that might impact DNA methylation. For instance, eight CLZ-treated patients were also receiving valproate, a drug that has been reported to be a potent epigenetic agent, affecting the DNA and histone methylation status (61). Nevertheless, due to a paucity of longitudinal epigenetic data of CLZ-treated patients, we believe that this is a good approach to evaluate the potential CLZ effects on the epigenome. In this study, we only evaluated DNA methylome, future longitudinal studies should confirm our findings and explore whether CLZ may be considered as an epigenetic drug. We could conclude that the long-term CLZ treatment might reduce epigenetic aging in male patients with psychotic disorders. In addition, our findings suggest a hypomethylation of genes of the longevity regulatory pathway as a potential action of CLZ. Thus, CLZ poses an exciting mechanism to be further explored to improve life expectancy and ameliorate the aging effects of patients treated with this antipsychotic.
Data Availability Statement
The data presented in the study are deposited in the GEO online repository, accession numbers GSE197136 and GSE201638.
Ethics Statement
The studies involving human participants were reviewed and approved by the Research and Bioethics Committees of Instituto Nacional de Neurología y Neurocirugía Manuel Velasco Suárez, the Ethics Committee of the Children’s Psychiatric Hospital Dr. Juan N. Navarro with approval No. II3/01/0913, and the Ethics Committee of the National Institute of Genomic Medicine with approval No. 06/2018/I. Furthermore, all adult participants signed informed consent. For those patients under the age of 18 years, an informed assent letter was signed by both parents/guardians and the minor.
Author Contributions
NM-J, ML-L, and HN: conceptualization, resources, and project administration. BP-A, DD-O, AO-V, CA-C, YM-L, ES, IJ-R, CT-Z, and TG-C: methodology. BP-A, JM-M, and AG-M: software. BP-A and JM-M: formal analysis and data curation. BP-A, YM-L, AO-V, DD-O, JM-M, AG-M, and ES-R: investigation. BP-A, JM-M, and NM-J: writing—original draft preparation. ES-R, HN, ML-L, and NM-J: writing—review and editing. ES-R, YM-L, AO-V, DD-O, JM-M, AG-M, BP-A, ES, IJ-R, CT-Z, TG-C, NM-J, ML-L, and HN: visualization. NM-J, ML-L, AG-M, and HN: supervision. NM-J, AG-M, ML-L, and HN: funding acquisition. All authors have read and agreed to the published version of the manuscript.
Funding
This work was supported by the Consejo Nacional de Ciencia y Tecnología (CONACyT) (grant no. 233695 to NM-J), the Fundación Gonzalo Río Arronte (grant no. S591 to AG-M), the Instituto Nacional de Medicina Genómica (INMEGEN) (grant no. 06/2018/I to HN), and Universidad Autónoma Metropolitana, Xochimilco (grant no. 34605034 to ML-L). BP-A was a doctoral student from the Doctorado en Ciencias Biológicas y de la Salud at Universidad Autónoma Metropolitana, Mexico City, Mexico and was supported by CONACyT (grant No. 766012).
Conflict of Interest
The authors declare that the research was conducted in the absence of any commercial or financial relationships that could be construed as a potential conflict of interest.
Publisher’s Note
All claims expressed in this article are solely those of the authors and do not necessarily represent those of their affiliated organizations, or those of the publisher, the editors and the reviewers. Any product that may be evaluated in this article, or claim that may be made by its manufacturer, is not guaranteed or endorsed by the publisher.
Supplementary Material
The Supplementary Material for this article can be found online at: https://www.frontiersin.org/articles/10.3389/fpsyt.2022.870656/full#supplementary-material
References
1. Walker ER, McGee RE, Druss BG. Mortality in mental disorders and global disease burden implications: a systematic review and meta-analysis. JAMA Psychiatry. (2015) 72:334–41. doi: 10.1001/jamapsychiatry.2014.2502
2. Dregan A, McNeill A, Gaughran F, Jones PB, Bazley A, Cross S, et al. Potential gains in life expectancy from reducing amenable mortality among people diagnosed with serious mental illness in the United Kingdom. PLoS One. (2020) 15:e0230674. doi: 10.1371/journal.pone.0230674
3. Hjorthøj C, Stürup AE, McGrath JJ, Nordentoft M. Years of potential life lost and life expectancy in schizophrenia: a systematic review and meta-analysis. Lancet Psychiatry. (2017) 4:295–301. doi: 10.1016/S2215-0366(17)30078-0
4. Han LKM, Verhoeven JE, Tyrka AR, Penninx BWJH, Wolkowitz OM, Månsson KNT, et al. Accelerating research on biological aging and mental health: current challenges and future directions. Psychoneuroendocrinology. (2019) 106:293–311. doi: 10.1016/j.psyneuen.2019.04.004
5. Rutherford BR, Taylor WD, Brown PJ, Sneed JR, Roose SP. Biological aging and the future of geriatric psychiatry. J Gerontol A Biol Sci Med Sci. (2017) 72:343–52. doi: 10.1093/gerona/glw241
6. Wolkowitz OM. Accelerated biological aging in serious mental disorders. World Psychiatry Off. (2018) 17:144–5. doi: 10.1002/wps.20546
7. Hannum G, Guinney J, Zhao L, Zhang L, Hughes G, Sadda S, et al. Genome-wide methylation profiles reveal quantitative views of human aging rates. Mol Cell. (2013) 49:359–67. doi: 10.1016/j.molcel.2012.10.016
8. Horvath S. DNA methylation age of human tissues and cell types. Genome Biol. (2013) 14:R115. doi: 10.1186/gb-2013-14-10-r115
9. Levine ME, Lu AT, Quach A, Chen BH, Assimes TL, Bandinelli S, et al. An epigenetic biomarker of aging for lifespan and healthspan. Aging. (2018) 10:573–91. doi: 10.18632/aging.101414
10. Dada O, Adanty C, Dai N, Jeremian R, Alli S, Gerretsen P, et al. Biological aging in schizophrenia and psychosis severity: DNA methylation analysis. Psychiatry Res. (2021) 296:113646. doi: 10.1016/j.psychres.2020.113646
11. Tandon R, Nasrallah HA, Keshavan MS. Schizophrenia, “just the facts” 5. Treatment and prevention. Past, present, and future. Schizophr Res. (2010) 122:1–23. doi: 10.1016/j.schres.2010.05.025
12. Soontornniyomkij V, Lee EE, Jin H, Martin AS, Daly RE, Liu J, et al. Clinical correlates of insulin resistance in chronic schizophrenia: relationship to negative symptoms. Front Psychiatry. (2019) 10:251. doi: 10.3389/fpsyt.2019.00251
13. Khan SS, Singer BD, Vaughan DE. Molecular and physiological manifestations and measurement of aging in humans. Aging Cell. (2017) 16:624–33. doi: 10.1111/acel.12601
14. Schneider-Thoma J, Efthimiou O, Huhn M, Krause M, Reichelt L, Röder H, et al. Second-generation antipsychotic drugs and short-term mortality: a systematic review and meta-analysis of placebo-controlled randomised controlled trials. Lancet Psychiatry. (2018) 5:653–63. doi: 10.1016/S2215-0366(18)30177-9
15. Meyer JM, Nasrallah HA, McEvoy JP, Goff DC, Davis SM, Chakos M, et al. The clinical antipsychotic trials of intervention effectiveness (CATIE) Schizophrenia trial: clinical comparison of subgroups with and without the metabolic syndrome. Schizophr Res. (2005) 80:9–18. doi: 10.1016/j.schres.2005.07.015
16. Torniainen M, Mittendorfer-Rutz E, Tanskanen A, Björkenstam C, Suvisaari J, Alexanderson K, et al. Antipsychotic treatment and mortality in schizophrenia. Schizophr Bull. (2015) 41:656–63. doi: 10.1093/schbul/sbu164
17. Nucifora FC, Mihaljevic M, Lee BJ, Sawa A. Clozapine as a model for antipsychotic development. Neurotherapeutics. (2017) 14:750–61. doi: 10.1007/s13311-017-0552-9
18. Farooq S, Taylor M. Clozapine: dangerous orphan or neglected friend? Br J Psychiatry. (2011) 198:247–9. doi: 10.1192/bjp.bp.110.088690
19. Cho J, Hayes RD, Jewell A, Kadra G, Shetty H, MacCabe JH, et al. Clozapine and all-cause mortality in treatment-resistant schizophrenia: a historical cohort study. Acta Psychiatr Scand. (2019) 139:237–47. doi: 10.1111/acps.12989
20. Hayes RD, Downs J, Chang CK, Jackson RG, Shetty H, Broadbent M, et al. The effect of clozapine on premature mortality: an assessment of clinical monitoring and other potential confounders. Schizophr Bull. (2015) 41:644–55. doi: 10.1093/schbul/sbu120
21. Alessandro G, Erbo D, Grayson DR. Epigenetic basis of clozapine action. J Drug Des Res. (2017) 4:1055.
22. Dong E, Locci V, Gatta E, Grayson DR, Guidotti A. N-Phthalyl-l-tryptophan (RG108), like clozapine (CLO), induces chromatin remodeling in Brains of prenatally stressed mice. Mol Pharmacol. (2019) 95:62–9. doi: 10.1124/mol.118.113415
23. Higgins-Chen AT, Boks MP, Vinkers CH, Kahn RS, Levine ME. Schizophrenia and epigenetic aging biomarkers: increased mortality, reduced cancer risk, and unique clozapine effects. Biol Psychiatry. (2020) 88:224–35. doi: 10.1016/j.biopsych.2020.01.025
24. Tian Y, Morris TJ, Webster AP, Yang Z, Beck S, Feber A, et al. ChAMP: updated methylation analysis pipeline for illumina beadchips. Bioinforma Oxf Engl. (2017) 33:3982–4. doi: 10.1093/bioinformatics/btx513
25. Teschendorff AE, Marabita F, Lechner M, Bartlett T, Tegner J, Gomez-Cabrero D, et al. A beta-mixture quantile normalization method for correcting probe design bias in illumina infinium 450 k DNA methylation data. Bioinforma Oxf Engl. (2013) 29:189–96. doi: 10.1093/bioinformatics/bts680
26. Xu Z, Niu L, Li L, Taylor JA. ENmix: a novel background correction method for illumina humanmethylation450 beadchip. Nucleic Acids Res. (2016) 44:e20. doi: 10.1093/nar/gkv907
27. Houseman EA, Accomando WP, Koestler DC, Christensen BC, Marsit CJ, Nelson HH, et al. DNA methylation arrays as surrogate measures of cell mixture distribution. BMC Bioinformatics. (2012) 13:86. doi: 10.1186/1471-2105-13-86
28. Ritchie ME, Phipson B, Wu D, Hu Y, Law CW, Shi W, et al. Limma powers differential expression analyses for RNA-sequencing and microarray studies. Nucleic Acids Res. (2015) 43:e47. doi: 10.1093/nar/gkv007
29. Butcher LM, Beck S. Probe lasso: a novel method to rope in differentially methylated regions with 450K DNA methylation data. Methods. (2015) 72:21–8. doi: 10.1016/j.ymeth.2014.10.036
30. Liao Y, Wang J, Jaehnig EJ, Shi Z, Zhang B. WebGestalt 2019: gene set analysis toolkit with revamped UIs and APIs. Nucleic Acids Res. (2019) 47:W199–205. doi: 10.1093/nar/gkz401
31. Szklarczyk D, Gable AL, Lyon D, Junge A, Wyder S, Huerta-Cepas J, et al. STRING v11: protein-protein association networks with increased coverage, supporting functional discovery in genome-wide experimental datasets. Nucleic Acids Res. (2019) 47:D607–13. doi: 10.1093/nar/gky1131
32. Shannon P, Markiel A, Ozier O, Baliga NS, Wang JT, Ramage D, et al. Cytoscape: a software environment for integrated models of biomolecular interaction networks. Genome Res. (2003) 13:2498–504. doi: 10.1101/gr.1239303
33. Kanehisa M, Furumichi M, Tanabe M, Sato Y, Morishima K. KEGG: new perspectives on genomes, pathways, diseases and drugs. Nucleic Acids Res. (2017) 45:D353–61. doi: 10.1093/nar/gkw1092
34. Nolasco-Rosales GA, Martínez-Magaña JJ, Juárez-Rojop IE, González-Castro TB, Tovilla-Zarate CA, García AR, et al. Association study among comethylation modules, genetic polymorphisms and clinical features in mexican teenagers with eating disorders: preliminary results. Nutrients. (2021) 13:3210. doi: 10.3390/nu13093210
35. Watanabe K, Taskesen E, van Bochoven A, Posthuma D. Functional mapping and annotation of genetic associations with FUMA. Nat Commun. (2017) 8:1826. doi: 10.1038/s41467-017-01261-5
36. Gillespie A, Hannon E, Dempster E, Collier D, Egerton A, Mill J, et al. T50 – longitudinal epigenetic analysis of clozapine use in treatment-resistant schizophrenia: data from the crestar consortium. Eur Neuropsychopharmacol. (2017) 27:S463–4. doi: 10.1016/j.euroneuro.2016.09.538
37. Hannon E, Dempster EL, Mansell G, Burrage J, Bass N, Bohlken MM, et al. DNA methylation meta-analysis reveals cellular alterations in psychosis and markers of treatment-resistant schizophrenia. Elife. (2021) 10:e58430. doi: 10.7554/eLife.58430
38. Lee MK, Hong Y, Kim SY, London SJ, Kim WJ. DNA methylation and smoking in Korean adults: epigenome-wide association study. Clin Epigenetics. (2016) 8:103. doi: 10.1186/s13148-016-0266-6
39. Okazaki S, Otsuka I, Numata S, Horai T, Mouri K, Boku S, et al. Epigenetic clock analysis of blood samples from Japanese schizophrenia patients. NPJ Schizophr. (2019) 5:4. doi: 10.1038/s41537-019-0072-1
40. Kinoshita M, Numata S, Tajima A, Yamamori H, Yasuda Y, et al. Effect of clozapine on DNA methylation in peripheral leukocytes from patients with treatment-resistant schizophrenia. Int J Mol Sci. (2017) 18:632. doi: 10.3390/ijms18030632
41. Melas PA, Rogdaki M, Ösby U, Schalling M, Lavebratt C, Ekström TJ. Epigenetic aberrations in leukocytes of patients with schizophrenia: association of global DNA methylation with antipsychotic drug treatment and disease onset. FASEB J. (2012) 26:2712–8. doi: 10.1096/fj.11-202069
42. Dong E, Tueting P, Matrisciano F, Grayson DR, Guidotti A. Behavioral and molecular neuroepigenetic alterations in prenatally stressed mice: relevance for the study of chromatin remodeling properties of antipsychotic drugs. Transl Psychiatry. (2016) 6:e711. doi: 10.1038/tp.2015.191
43. Seo MK, Kim YH, McIntyre RS, Mansur RB, Lee Y, Carmona NE, et al. Effects of antipsychotic drugs on the epigenetic modification of brain-derived neurotrophic factor gene expression in the hippocampi of chronic restraint stress rats. Neural Plast. (2018) 2018:2682037. doi: 10.1155/2018/2682037
44. Corbo RM, Ulizzi L, Positano L, Scacchi R. Association of CYP19 and ESR1 pleiotropic genes with human longevity. J Gerontol A Biol Sci Med Sci. (2011) 66:51–5. doi: 10.1093/gerona/glq160
45. Han B, Chen H, Yao Y, Liu X, Nie C, Min J, et al. Genetic and non-genetic factors associated with the phenotype of exceptional longevity & normal cognition. Sci Rep. (2020) 10:19140. doi: 10.1038/s41598-020-75446-2
46. Garratt M, Lagerborg KA, Tsai YM, Galecki A, Jain M, Miller RA. Male lifespan extension with 17-α estradiol is linked to a sex-specific metabolomic response modulated by gonadal hormones in mice. Aging Cell. (2018) 17:e12786. doi: 10.1111/acel.12786
47. Mann SN, Hadad N, Nelson Holte M, Rothman AR, Sathiaseelan R, Ali Mondal S, et al. Health benefits attributed to 17α-estradiol, a lifespan-extending compound, are mediated through estrogen receptor α. Elife. (2020) 9:e59616. doi: 10.7554/eLife.59616
48. Vermeulen JM, van Rooijen G, van de Kerkhof MPJ, Sutterland AL, Correll CU, de Haan L. Clozapine and long-term mortality risk in patients with schizophrenia: a systematic review and meta-analysis of studies lasting 1.1-12.5 years. Schizophr Bull. (2019) 45:315–29. doi: 10.1093/schbul/sby052
49. Taipale H, Tanskanen A, Mehtälä J, Vattulainen P, Correll CU, Tiihonen J. 20-year follow-up study of physical morbidity and mortality in relationship to antipsychotic treatment in a nationwide cohort of 62,250 patients with schizophrenia (FIN20). World Psychiatry. (2020) 19:61–8. doi: 10.1002/wps.20699
50. De Lorenzo MS, Chen W, Baljinnyam E, Carlini MJ, La Perle K, Bishop SP, et al. Reduced malignancy as a mechanism for longevity in mice with adenylyl cyclase type 5 disruption. Aging Cell. (2014) 13:102–10. doi: 10.1111/acel.12152
51. Vatner SF, Pachon RE, Vatner DE. Inhibition of adenylyl cyclase type 5 increases longevity and healthful aging through oxidative stress protection. Oxid Med Cell Longev. (2015) 2015:250310. doi: 10.1155/2015/250310
52. Vatner SF, Yan L, Ishikawa Y, Vatner DE, Sadoshima J. Adenylyl cyclase type 5 disruption prolongs longevity and protects the heart against stress. Circ J. (2009) 73:195–200. doi: 10.1253/circj.cj-08-0957
53. Yan L, Park JY, Dillinger JG, De Lorenzo MS, Yuan C, Lai L, et al. Common mechanisms for calorie restriction and adenylyl cyclase type 5 knockout models of longevity. Aging Cell. (2012) 11:1110–20. doi: 10.1111/acel.12013
54. Yan L, Vatner DE, O’Connor JP, Ivessa A, Ge H, Chen W, et al. Type 5 adenylyl cyclase disruption increases longevity and protects against stress. Cell. (2007) 130:247–58. doi: 10.1016/j.cell.2007.05.038
55. Apfeld J, O’Connor G, McDonagh T, DiStefano PS, Curtis R. The AMP-activated protein kinase AAK-2 links energy levels and insulin-like signals to lifespan in C. elegans. Genes Dev. (2004) 18:3004–9. doi: 10.1101/gad.1255404
56. Burkewitz K, Weir HJM, Mair WB. AMPK as a pro-longevity target. Exp Suppl. (2016) 107:227–56. doi: 10.1007/978-3-319-43589-3_10
57. Greer EL, Banko MR, Brunet A. AMP-activated protein kinase and FoxO transcription factors in dietary restriction-induced longevity. Ann N Y Acad Sci. (2009) 1170:688–92. doi: 10.1111/j.1749-6632.2009.04019.x
58. Morgunova GV, Klebanov AA. Age-related AMP-activated protein kinase alterations: from cellular energetics to longevity. Cell Biochem Funct. (2019) 37:169–76. doi: 10.1002/cbf.3384
59. Stancu AL. AMPK activation can delay aging. Discov Craiova Rom. (2015) 3:e53. doi: 10.15190/d.2015.45
60. Janssens GE, Lin XX, Millan-Ariño L, Kavšek A, Sen I, Seinstra RI, et al. Transcriptomics-based screening identifies pharmacological inhibition of Hsp90 as a means to defer aging. Cell Rep. (2019) 27:467–80.e. doi: 10.1016/j.celrep.2019.03.044
Keywords: DNA methylome, clozapine, psychotic disorders, epigenetic age, longevity
Citation: Pérez-Aldana BE, Martínez-Magañ JJ, Mayén-Lobo YG, Dávila-Ortiz de Montellano DJ, Aviña-Cervantes CL, Ortega-Vázquez A, Genis-Mendoza AD, Sarmiento E, Soto-Reyes E, Juárez-Rojop IE, Tovilla-Zarate CA, González-Castro TB, Nicolini H, López-López M and Monroy-Jaramillo N (2022) Clozapine Long-Term Treatment Might Reduce Epigenetic Age Through Hypomethylation of Longevity Regulatory Pathways Genes. Front. Psychiatry 13:870656. doi: 10.3389/fpsyt.2022.870656
Received: 07 February 2022; Accepted: 29 March 2022;
Published: 18 May 2022.
Edited by:
Mirko Manchia, University of Cagliari, ItalyReviewed by:
Stefania Schiavone, University of Foggia, ItalyAlessio Squassina, University of Cagliari, Italy
Copyright © 2022 Pérez-Aldana, Martínez-Magaña, Mayén-Lobo, Dávila-Ortiz de Montellano, Aviña-Cervantes, Ortega-Vázquez, Genis-Mendoza, Sarmiento, Soto-Reyes, Juárez-Rojop, Tovilla-Zarate, González-Castro, Nicolini, López-López and Monroy-Jaramillo. This is an open-access article distributed under the terms of the Creative Commons Attribution License (CC BY). The use, distribution or reproduction in other forums is permitted, provided the original author(s) and the copyright owner(s) are credited and that the original publication in this journal is cited, in accordance with accepted academic practice. No use, distribution or reproduction is permitted which does not comply with these terms.
*Correspondence: Humberto Nicolini, aG5pY29saW5pQGlubWVnZW4uZ29iLm14; Nancy Monroy-Jaramillo, bmFuY3kubW9ucm95QGlubm4uZWR1Lm14