- Department of Psychology, University of Wisconsin-Milwaukee, Milwaukee, WI, United States
Cannabis use has been associated with deficits in self-regulation, including inhibitory control. Cannabis users have previously exhibited both structural and functional deficits in the rostral anterior cingulate cortex (rACC), a region involved in self-regulation of emotional response and inhibitory control. The present study aimed to examine whether abstinent cannabis users demonstrated abnormal functional activation and connectivity of the bilateral rACC during an emotional inhibitory processing task, and whether gender moderated these relationships. Cannabis-using (N = 34) and non-using (N = 32) participants ages 16–25 underwent at least 2-weeks of monitored substance use abstinence (excluding tobacco) and fMRI scanning while completing a Go/No-go task using fearful and calm emotional faces as non-targets. Multiple linear regression and ANCOVA were used to determine if cannabis group status was related to rACC activation and context-dependent functional connectivity, and whether gender moderated these relationships. Results showed decreased bilateral rACC activation in cannabis users during fearful response inhibition, although groups did not show any context-dependent connectivity differences between the left or right rACC during calm or fearful inhibition. Gender findings revealed that cannabis-using females compared to males did show aberrant connectivity between the right rACC and right cerebellum. These results are consistent with literature demonstrating aberrant structural and functional rACC findings and suggest that chronic cannabis use may disrupt typical rACC development—even after abstinence—potentially conferring risk for later development of mood disorders. Marginal gender-specific connectivity findings bolster continued findings regarding female vulnerability to effects of cannabis on cognition and affect. Findings should be assessed in longitudinal studies to determine causality and timing effects.
Introduction
Cannabis use is becoming more common, with 42% of young adults (aged 19–30) using during 2020 in the United States (1). Adolescents and young adults may be particularly vulnerable to the neurocognitive impact of cannabis use due to ongoing neurodevelopment (2, 3). The frontal executive system is one of the last to develop, taking longer to mature than limbic regions involved in emotion (4). Thus, adolescents and young adults may have an increased likelihood of participating in risky activities, such as substance use (2, 4). In turn, the protracted neurodevelopment occurring during adolescence and young adulthood may leave them more susceptible to the neurocognitive effects of substance use (4).
Δ-9-Tetrahydrocannabinol (THC)—the primary psychoactive component of cannabis—binds receptors in the endogenous cannabinoid (eCB) system (5), namely, the cannabinoid receptor-1 (CB1) in the brain. CB1 receptor density is high in the prefrontal cortex (PFC), cingulate cortex, hippocampus, amygdala, basal ganglia, and cerebellum (6–9). The eCB system modulates a variety of functions (e.g., sleep, pain, inflammation, and energy intake), including stress and emotional regulation (10, 11), and—from a psychological perspective—the eCB system is involved in executive functioning, affective processing, and mood disorders (11, 12). Therefore, it is posited long-term cannabis use has the potential to disrupt eCB activity and in turn, impacting these domains.
Inhibitory control, or response inhibition, is conceptualized as withholding a prepotent response (13–16). With few exceptions (17), chronic cannabis exposure has been associated with inhibitory control deficits such that, cannabis users perform more poorly than non-users on behavioral and neuropsychological inhibitory control tasks (18–24); additionally, functional magnetic resonance imaging (fMRI) studies have revealed greater activation in cannabis users versus non-users within inhibitory control regions (25–27). While completing inhibitory control tasks (e.g., Go/No-go or Stop-signal), cannabis users demonstrate hyperactivity of dorsolateral prefrontal, medial frontal, inferior frontal gyrus, cingulate, inferior, and superior parietal, suggesting increased brain processing effort was necessary to achieve inhibition (25–27). Along with task-based fMRI investigations, chronic cannabis use has also been associated with greater functional connectivity during inhibitory control tasks. Cannabis-dependent individuals demonstrated hyperconnectivity between frontal control and substantia nigra/STN network during response inhibition (28) and had greater connectivity between the PFC and occipitoparietal cortex related to task difficulty despite no performance differences (29). Therefore, prior research has suggested that regular cannabis use is linked with increased connectivity across the cognitive control network, including prefrontal regions.
One posited mediator for the impact of cannabis use on inhibitory control is the anterior cingulate cortex (ACC). Cannabis users exhibit ACC hypoactivity associated with reduced error awareness (30) and during response inhibition (i.e., Stroop)—despite similar behavioral performance (31, 32)—along with dispersed ACC activity during behavioral inhibition (33); these findings are evident after minimal to no periods of cannabis abstinence. Specifically, the ACC is critical in automatic emotion regulation (34, 35), which has connections to both the amygdala and the PFC (36–38). Notably, two functionally distinct subdivisions of the ACC exist (36, 37) which each serve unique roles in emotion processing (39). Specifically, the rostral-ventral ACC (rACC) plays a role in regulating emotional conflict, while the caudal-dorsal ACC (dACC) plays a more significant role in emotional appraisal and expression (39, 40). As it relates to cannabis use, acute THC administration demonstrates aberrant limbic, frontal, temporal, ACC, and parietal activity elicited by emotional faces (41–44), and increased connectivity between the rACC/medial PFC and the amygdala (45). Among chronic users, reduced amygdala activation (46–48) and attenuated ACC activation is observed when viewing negative faces and scenes (46, 48). Further, aberrant frontolimbic connectivity (49) and hyperconnectivity between the ACC and parietal, post-central, and precuneus cortices has been demonstrated when responding to cannabis-cues (50). Correspondingly, bilateral rACC hyperconnectivity at rest is associated with increased depressive mood symptoms in cannabis users (51). Moreover, these activation and connectivity findings are demonstrated across varying lengths of sustained abstinence (no abstinence to 7-days) and thus, clarifying the role of longer lengths abstinence in the relationship between ACC and repeated cannabis use is warranted.
Interestingly, cannabis use is associated with structural abnormalities in the ACC among ROI-specific analyses: smaller volumes in individuals with (52, 53) and without (54) psychosis, and reduced cortical thickness in those with concurrent psychopathology (55, 56) and alcohol use (57). Though, whole-brain morphometry findings tend to find null or mixed structural differences in the ACC (58–60). Notably, these varied structural differences in the ACC may be better explained by underlying functional aberrations. Within cannabis users, we previously reported that smaller rACC volumes were linked with deficits in discriminating differences in facial emotions (54), aligning with previous reports (40). Taken together, research indicates that within the ACC, cannabis users generally exhibit reduced gray matter volume and abnormal activation during cognitive control and emotion processing tasks. Additionally, cannabis and other substances have been associated with increased functional connectivity between the ACC and other cortices, though the literature is not consistent, possible indicating increased connectivity in cannabis users is necessary in order to achieve a behavioral response commensurate with controls.
Investigating brain-behavior associations within fMRI investigations can also shed light on applicability of neuroimaging findings on behavior. Cannabis use has been related to increased levels of perceived stress, and on the flip side, lower distress tolerance (61–64). Notably, emotion regulation is a protective factor against the initiation of substance use (65) and can be dysregulated in substance users (66, 67) which, in turn, mediates the relationship between stress and using cannabis as a coping mechanism (68). Therefore, the literature suggests a relationship between cannabis use, increased perception of stress, poor emotional inhibition, blunted rACC activation, aberrant rACC frontolimbic connectivity, and increased rACC connectivity diffusely across cortices.
There is also a question on whether gender may moderate this relationship, as indicated by studies highlighting how females typically outperform males in response inhibition in healthy populations (69–71). Moreover, males and females also differ in basic emotional processing; each gender demonstrates unique neural activation patterns while viewing emotional faces (72–75). Specifically, males exhibit increased frontal and parietal recruitment while females demonstrate greater temporal, ACC, and limbic activation to emotional faces (75–78). Specific to cannabis, preclinical studies indicate eCB activity varies by sex in a regionally specific manner (79) and density is modulated by estrogen activity (79–81). Importantly, females may be more vulnerable to the effects of cannabis on affective circuitry. Female, relative to male, rats are more susceptible to the anxiety and depression producing properties of THC (82) and exhibit greater CB1 desensitization to THC in frontolimbic regions (82, 83). In humans, female cannabis users have volumetric differences in the PFC, amygdala, and ACC relative to non-using females, that are related to subtle but significant behavioral outcomes (54, 84–86) and demonstrate more pronounced differences in left rACC volumes, which is associated with poorer facial emotion processing (54). These findings remain even after variable periods of sustained abstinence (up to one month) and further reinforce investigating potential long-term effects after a period of sustained abstinence. Additionally, given the aforementioned gender differences, there may be neural differences in the left rACC during emotional response inhibition in cannabis using males versus females.
The aim of the present study is to examine rACC activation during inhibitory processing while viewing negative affective stimuli among abstinent adolescent and young adult regular cannabis users and non-users and investigate functional connectivity differences between the rACC and the rest of the brain during this task. We expect to see decreased rACC activation during correct Fearful No-go trials (46, 48) and increased left and right rACC connectivity with frontal and limbic regions (28, 29, 50, 87, 88) among cannabis users, despite abstinence status. In addition, gender differences on these associations will be examined, with females hypothesized to show pronounced differences in left and right rACC activity and connectivity (54, 82–85). Exploratory analyses will examine the correlation between significant findings and perceived stress.
Materials and Methods
Participants
All study protocol was approved by university IRB and in accordance with the Declaration of Helsinki. Participants were individuals ages 16–25, recruited from a larger parent study (R01DA030354; PI: Lisdahl). Exclusionary criteria consisted of magnetic resonance imaging (MRI) contraindications, pregnancy, left-handedness, birth complications, traumatic head injury, neurological disorders, learning and intellectual disabilities, vision or hearing impairments, current psychotropic medication use, independent Axis I DSM-IV-TR diagnosis, ≥10 cigarettes per day, and excessive other drug use (>25 lifetime uses of non-cannabis or non-alcohol substance use).
To be included in the cannabis-using group, individuals were required to have used at least 40 times in the past year and have at least 50 lifetime uses. To be included as a non-using control, individuals were required to have fewer than 5 past year and 20 lifetime cannabis uses (86).
Procedure
Individuals were recruited through flyers posted in the community. Phone screening was conducted to determine eligibility of interested individuals. During screening, demographics, lifetime substance use history was gathered using the Customary Drinking and Drug Use Record (CDDR) (89, 90), and a DSM-IV-TR semi-structured interview, the Mini International Psychiatric Interview (MINI) (91) was administered.
Eligible participants completed an informed consent/assent process. For those under age 18, parent permission and minor assent were obtained. Prior to MRI scanning, participants underwent at least 2-weeks of monitored abstinence of all substances except nicotine (including alcohol and cannabis) using urine toxicology (One Step Drug Screen Test Dip Card Panel; Innovacon, Inc., San Diego, CA, United States) and continuous sweat toxicology (PharmChek Drugs of Abuse Patch; PharmChem Inc., Fort Worth, TX, United States). Participants also underwent repeated breathalyzer testing (Alco-Sensor IV; Intoximeters, Inc., St. Louis, MO, United States) for recent alcohol use. Abstinence was verified at weekly sessions during the 3 weeks preceding the MRI scan.
Measures
Substance Use
Participants were administered the Timeline Follow Back (TLFB) (19, 92), a measure of past year substance use that uses holidays and other memory cues. Substance use was measured in standard units [alcohol (standard drinks), nicotine (number of cigarettes and hits of chew/snuff/pipe/cigar/hookah), cannabis (smoked/vaped flower and concentrates were measured and dosing was converted to joints based grams)] and assessed for each day during the past year. The CDDR was used to measure lifetime and past 3-month substance use (90).
Perceived Stress
The Perceived Stress Scale-14 (PSS-14) (93) was used to measure level of perceived stress. The PSS-14 is a 14-item measure of the degree to which individuals perceive situations as stressful and measures levels of distress and ability to cope (94).
Magnetic Resonance Imaging Data Acquisition
High-resolution anatomical images were collected using a T1-weighted spoiled gradient-recalled at steady-state pulse sequence (TR = 8.2 ms, TE = 3.4 s, TI = 450, and flip angle of 12°). The in-plane resolution of the anatomical images was 256 × 256 with a square field of view (FOV) of 240 mm. One hundred fifty slices were acquired at 1 mm thickness. Echo planar images (EPI) were acquired while performing the emotional Go/No-go task using T2 × weighted gradient-echo EPI pulse sequence (TR/TE = 2500 ms/30 ms, FOV = 200 cm, matrix 64 × 64 voxels, slice thickness = 3.2 mm., flip angle = 90 degrees, 44 contiguous axial slices) with 117 TRs of volume data acquired per run.
Functional Magnetic Resonance Imaging Task
Participants completed an emotional Go/No-go task previously used with healthy adolescents (95, 96). Task stimuli included fearful, happy, and calm faces from the NimStim set of facial expressions (97). The task included six functional runs, counterbalanced for order, one for each combination of emotion (fearful, happy, calm) and Go/No-go. At the beginning of each run, participants were instructed to press a button (“Go”) for a particular emotional face and to withhold a button press (“No-go”) for a different type of face. On each trial, a face was presented for 500 milliseconds, followed by a jittered intertrial interval ranging from 2 to 14.5 s (mean = 5.2 s). In sum, 48 trials appeared in a pseudorandomized order (35 “Go” and 13 “No-go”).
Magnetic Resonance Imaging Preprocessing
All images underwent standard preprocessing steps using the Analysis of Functional Neuroimages software package (AFNI) (98). Preprocessing included slice time alignment, motion correction, and co-registration of EPI data to T1 scan with the aid of a cost function. Each voxel’s time series was despiked and a spatial smoothing kernel of 4 mm was used. Each individual’s anatomy was warped to standard (Montreal Neurological Institute; MNI) space and the resulting registration matrix was applied to the EPI data. Then a brain mask was created from the EPI data, which was aligned to the volume with the fewest outliers. Each individual’s activation data was scaled to percent signal change. The first three TRs of volume data were removed from each run. To account for motion, TRs of volume data with greater than 0.4 mm of motion were censored from the analysis. Subjects with >18% of volumes exceeding the 0.4 mm motion threshold on any individual run were removed from the analysis (N = 3; cannabis-using male N = 1, non-using male N = 1, non-using female N = 1) (25, 27). Additionally, TRs of volume data with intensity outliers greater than 10% of voxels in the automasked brain were censored. For each fearful and calm No-go stimuli, two functional runs were concatenated together (happy Go/fearful No-go and calm Go/fearful No-go for “fearful No-go”; happy Go/calm No-go and fearful Go/calm No-go for “calm No-go”). Then a gamma function was used for convolution of the stimuli timing to create a Hemodynamic Response Function (HRF). Finally, the HRF was deconvolved with the acquired MRI signal on a voxel-by-voxel basis. Images were visually inspected for accuracy and manually edited when appropriate.
Region of Interest (Rostral Anterior Cingulate Cortex) Measurement
FreeSurfer’s Desikan-Killiany atlas (99) was used to define the left and right rACC for each participant and was visually inspected for accuracy. The left and right rACC were then used as a seed region for (1) task-based ROI activation analysis and (2) task-based generalized psychophysiological interaction (gPPI) functional connectivity analysis to examine context-dependent connectivity between rACC (left and right) and whole brain.
Data Analysis
Preliminary Analysis
Demographic variables were examined with ANOVA and chi-square analyses. Variables that differed between groups were included in the primary analysis as additional covariates (see Table 1).
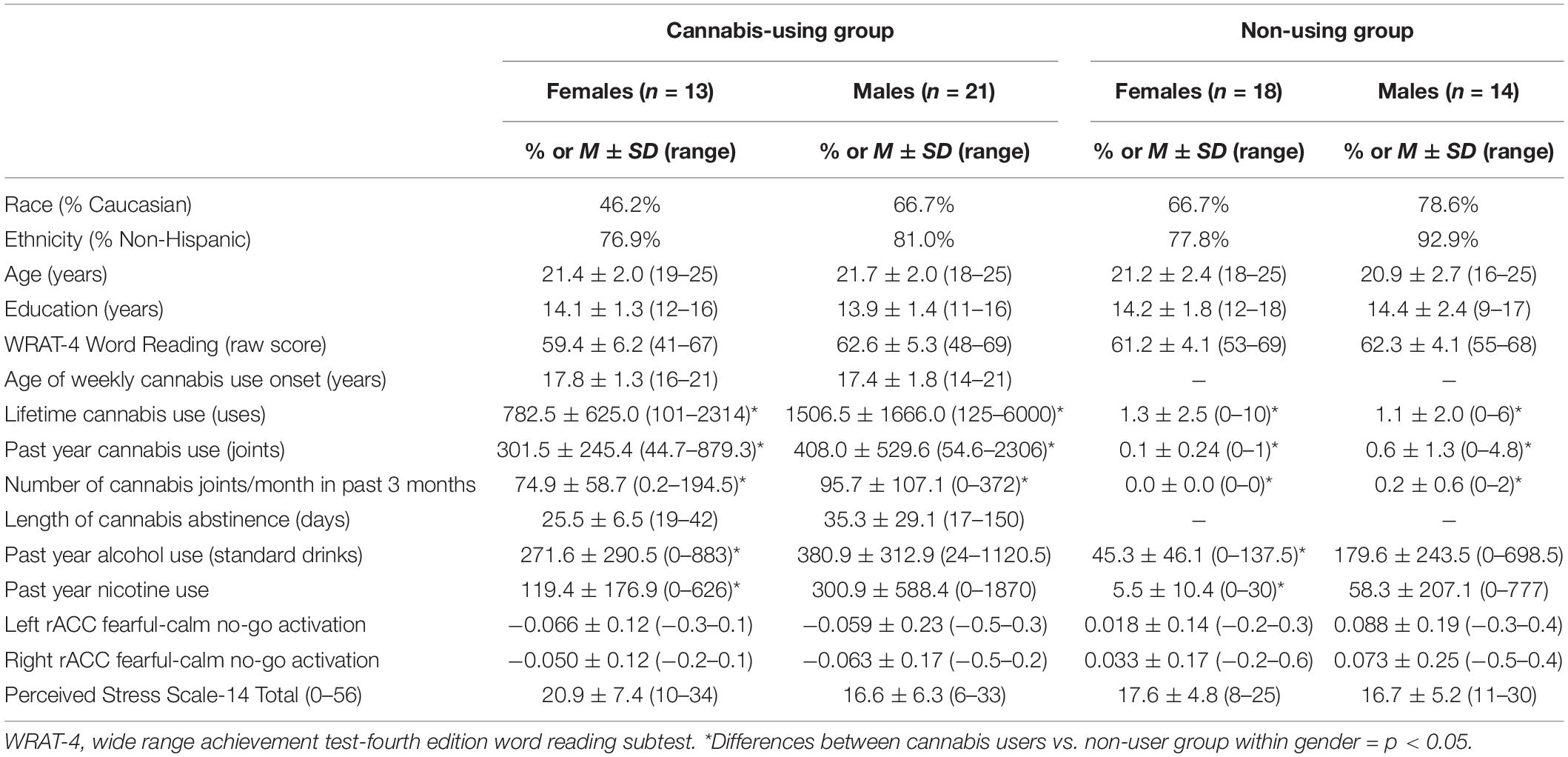
Table 1. Participant demographics, substance use variables, BOLD activation in rACC, and PSS-14 scores.
Behavioral Performance
Independent samples t-tests were used to compare differences in fMRI task performance between cannabis users and non-users. Participants who achieved <66% accurate “Go” trials for any given run (e.g., happy Go/fearful No-go) were excluded from the analysis due to performance validity concerns (N = 4; cannabis-using male N = 1, non-using male N = 1, non-using female N = 2) (100).
Primary Analysis
Single Subjects Analysis (Activation)
Using AFNI’s 3dROIstats, mean calm No-go activation was subtracted from mean fearful No-go activation for each the left and right rACC, yielding individual fearful-calm No-go activation values.
Group Analysis (Activation)
Individual subject fearful-calm No-go activation values for each left and right rACC were entered into SPSS. Multiple regressions were used to determine the relationship between cannabis group status and left and right rACC fearful-calm No-go activation. Main effects and covariates were added into the first block and cannabis × gender interaction was entered into the second block. Gender, past year alcohol use, and past year nicotine use were included as covariates in all analyses.
Single Subjects Analysis (Connectivity)
Single subject analysis included linear modeling consistent with gPPI analysis in AFNI. For each subject, four interaction regressors were created (for each combination of left/right rACC and fearful/calm) representing the interaction between changes in the blood oxygen level-dependent (BOLD) response to correct No-go trials and rACC activation. These interaction regressors were each entered into respective linear deconvolution models along with regressors of interest (correct No-go trials, rACC activation) and no interest (motion, drift effect, go trials, inaccurate No-go trials). The deconvolution models were used to determine connectivity within whole brain, yielding regression coefficients for each subject, which were entered into group analysis.
Group Analysis (Connectivity)
ANCOVA via AFNI’s “3dMVM” was used to perform four group analyses (for each combination of left/right rACC and fearful/calm) on cannabis × gender. Gender, past year alcohol use, and past year nicotine use were included as covariates.
Multiple Comparisons
Monte Carlo simulations using AFNI’s 3dClustSim were used to correct for multiple comparisons based on cluster extent (family-wise alpha = 0.05; voxelwise threshold of p = 0.005) (101).
Secondary Analysis
Pearson correlations were run to investigate whether left or right rACC activation during emotional inhibition was related to perceived stress scale (PSS) total scores. In addition, Pearson correlations were run to investigate whether functional connectivity (regression coefficients) in clusters that significantly differed according to only group and/or group × gender were significantly associated with perceived stress scale (PSS) total scores. Analyses were conducted in SPSS and statistical decisions were made if p < 0.05.
Results
Preliminary Analysis
A total of 66 individuals (34 cannabis users and 32 non-users) were included in the final analysis. ANOVAs and chi-square tests revealed no significant difference between cannabis users and non-users in age (p = 0.37), race (p = 0.47), ethnicity (p = 0.42), gender (p = 0.14), education (p = 0.51), reading ability (p = 0.81), and PSS-14 total scores (p = 0.49). As expected, cannabis users and non-users significantly differed on measures of lifetime cannabis use (p < 0.001), past year cannabis use (p < 0.001), past year alcohol use (p < 0.001), and past year nicotine use (p = 0.02). Follow-up demographic analyses examined differences in these variables by gender and by cannabis-group status within gender. Broadly, males and females significantly differed on measures of lifetime cannabis use (p = 0.05) and past year alcohol use (p = 0.02), such that males reported greater use relative to females. Consistent with full group findings, cannabis-using and non-using females significantly differed in lifetime cannabis use (p < 0.001), past year cannabis use (p < 0.001), past year alcohol use (p < 0.01), and past year nicotine use (p = 0.01). In addition, cannabis-using and non-using males significantly differed in lifetime cannabis use (p < 0.01) and past year cannabis use (p = 0.001) (see Table 1).
Behavioral Performance
There were no group differences in performance during Go trials paired with fearful No-go (p = 0.31), Go trials paired with calm No-go (p = 0.40), fearful No-go trials (p = 0.21), or calm No-go trials (p = 0.07).
Primary Analysis
Rostral Anterior Cingulate Cortex Region of Interest Activation
Cannabis users, relative to controls, demonstrated significantly less left [t(61) = −3.08, beta = −0.42, p = 0.003] and right [t(61) = −3.07, beta = −0.42, p = 0.003] rACC activation during fearful-calm No-go (Figure 1). Gender did not moderate the relationship between cannabis group and left [t(60) = 0.75, beta = 0.09, p = 0.46] or right [t(60) = 0.55, beta = 0.07, p = 0.58] rACC activation. Greater past year alcohol use significantly predicted stronger right rACC activation [t(61) = 2.13, beta = 0.29, p = 0.04] (see Table 2).
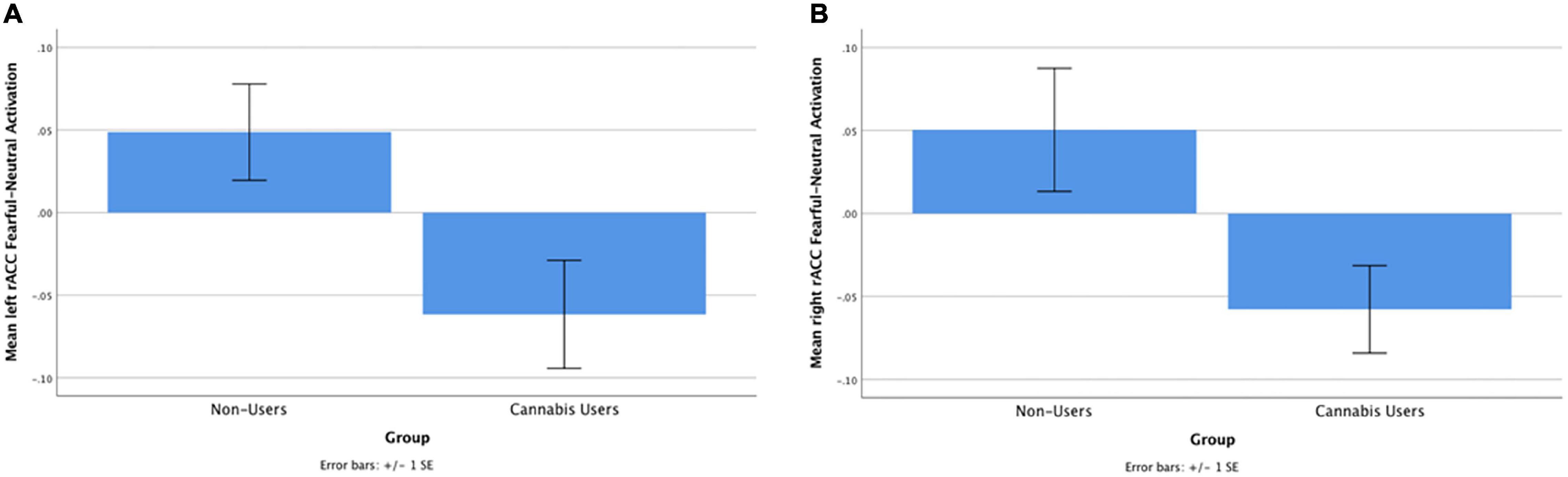
Figure 1. (A) Cannabis users, compared with controls, demonstrated significantly less left rACC activation during fearful-calm No-go trials [t(48) = –3.08, beta = –0.42, p = 0.003]. (B) Cannabis users, compared with controls, demonstrated significantly less right rACC activation during fearful-calm No-go trials [t(48) = –3.07, beta = –0.42, p = 0.003].
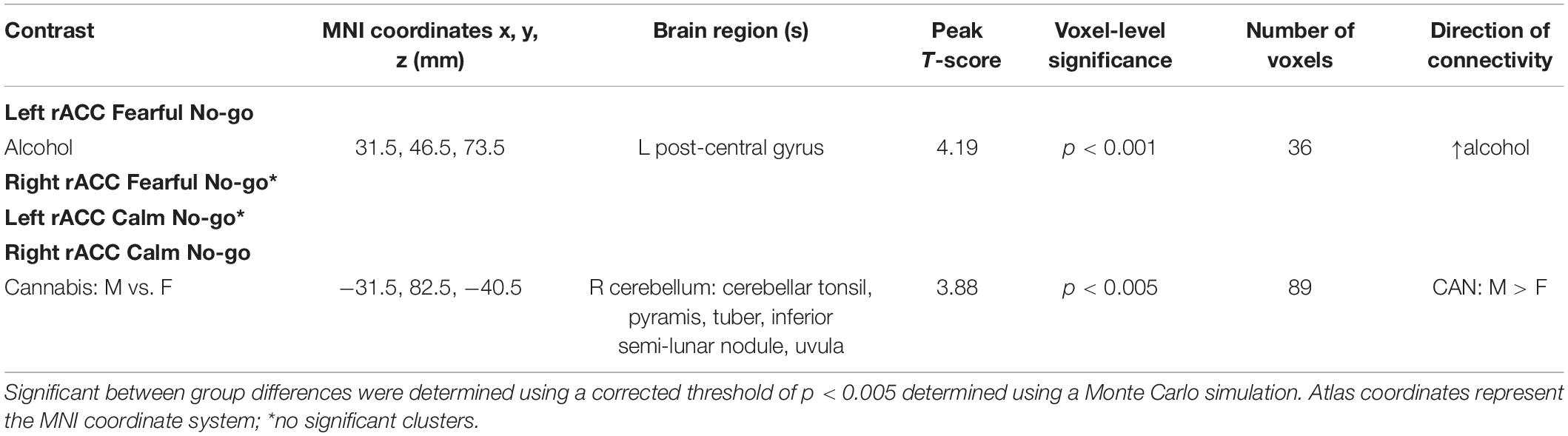
Table 2. Regions with significant differences in functional connectivity with the rACC for various contrasts.
Rostral Anterior Cingulate Cortex Connectivity
Left Rostral Anterior Cingulate Cortex Fearful No-Go
Cannabis and cannabis × gender did not significantly predict clusters functionally connected to the left rACC during successful Fearful No-go trials. However, greater past year alcohol use was significantly related to greater left rACC connectivity with one cluster located in the post-central gyrus (Table 2).
Left Rostral Anterior Cingulate Cortex Calm No-Go
Cannabis and cannabis × gender did not significantly predict clusters functionally connected to the left rACC during successful Calm No-go trials.
Right Rostral Anterior Cingulate Cortex Fearful No-Go
Cannabis and cannabis × gender did not significantly predict clusters functionally connected to the right rACC during successful Fearful No-go trials.
Right Rostral Anterior Cingulate Cortex Calm No-Go
Cannabis and cannabis × gender did not significantly predict clusters functionally connected to the right rACC during successful Calm No-go trials. Despite no cannabis × gender interaction, within a contrast of cannabis-using males compared to cannabis-using females, males demonstrated significantly greater connectivity relative to females between the right rACC and a cluster in the right cerebellum (Table 2 and Figures 2, 3). Within non-users, gender was not related to right rACC connectivity.
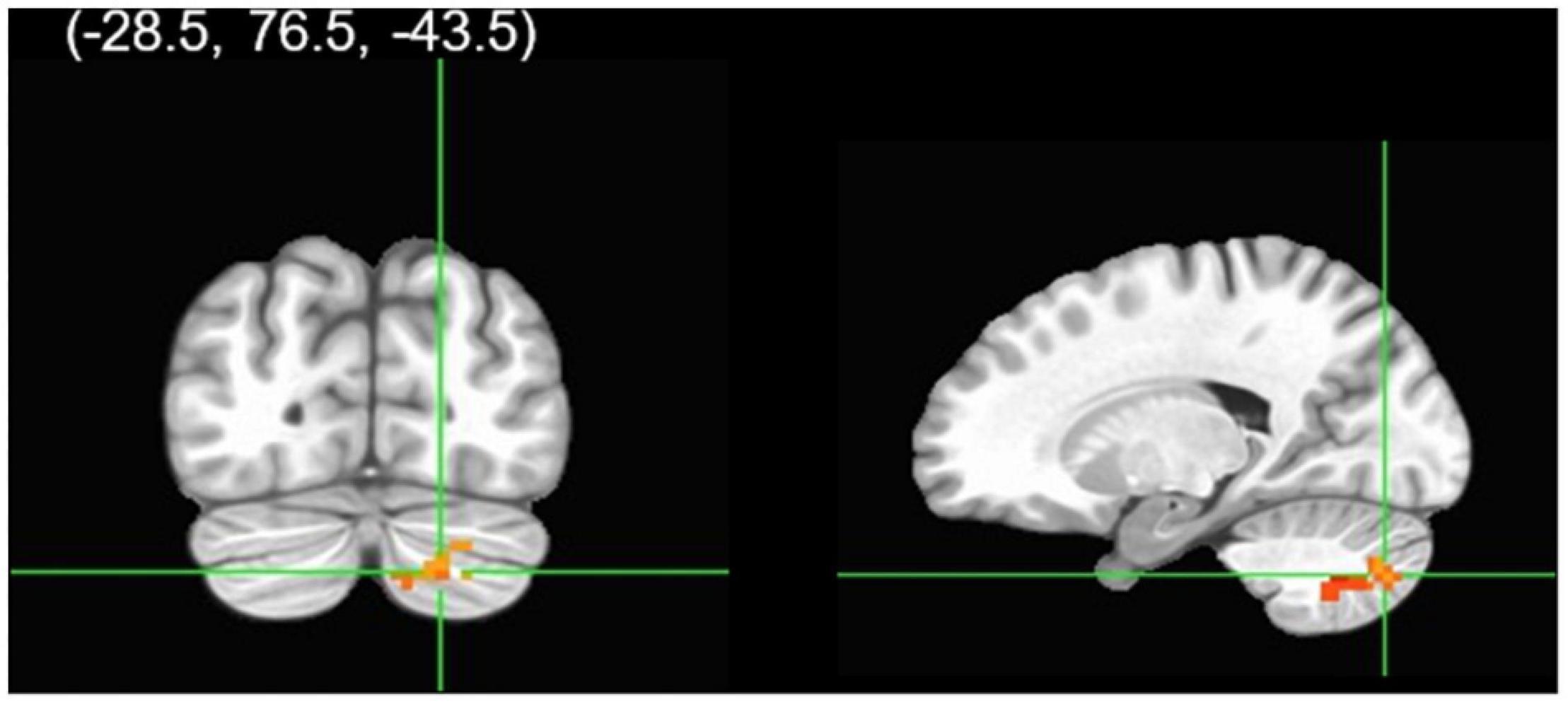
Figure 2. Group analysis of connectivity with the right rACC during correct calm No-go trials. A gender group contrast revealed the right rACC had greater functional connectivity with a cluster in the right cerebellum in males relative to females. The colors represent areas of significant connectivity; warm colors indicate increased connectivity (voxelwise threshold p < 0.005; family-wise correction p < 0.05).
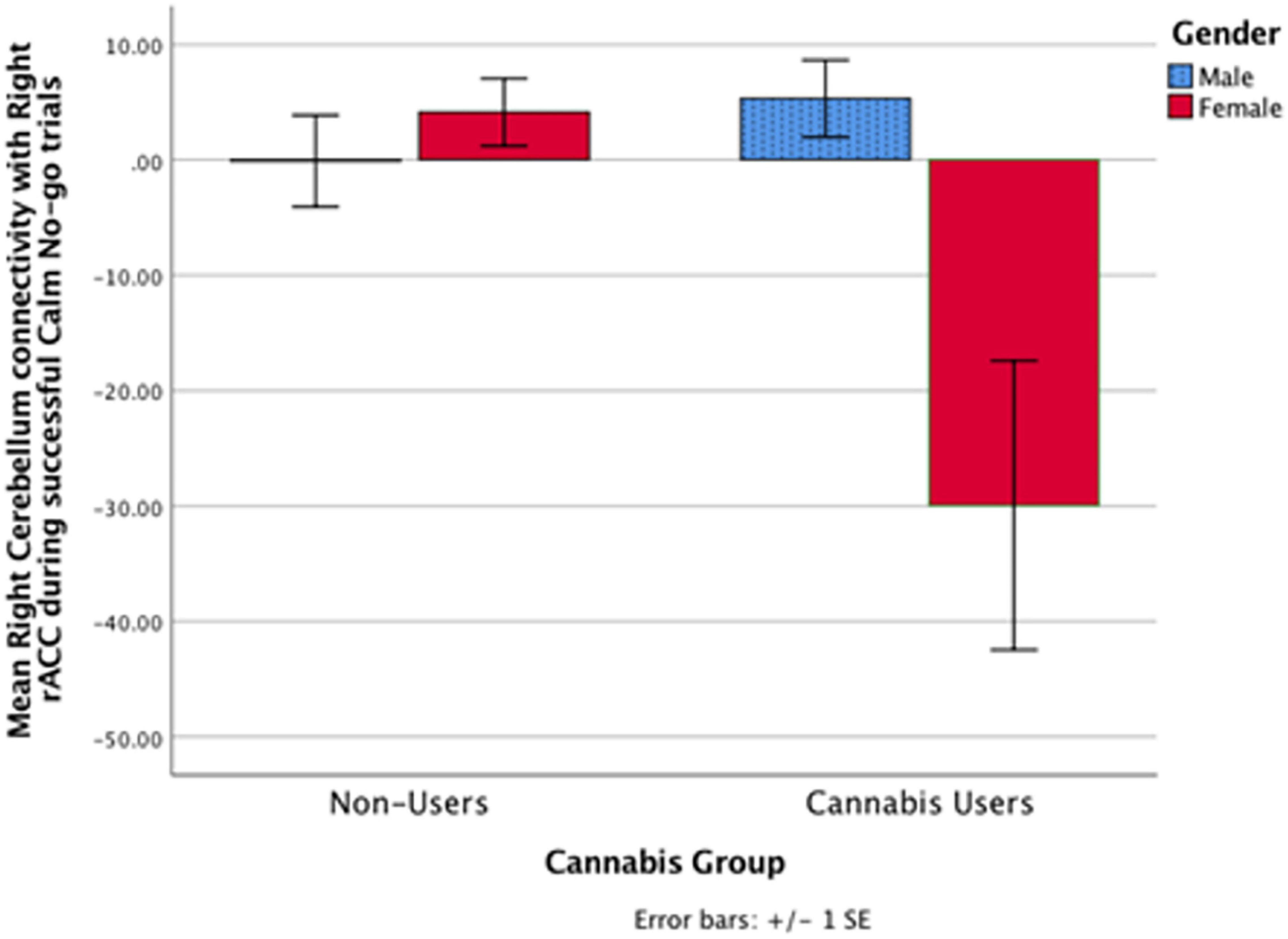
Figure 3. In the cannabis-using group, the right rACC had greater functional connectivity with a cluster in the right cerebellum in males relative to females during successful calm No-go trials (p < 0.005). Non-users did not exhibit a significant gender relationship. Notably, there was no significant cannabis × gender interaction.
Secondary Analysis
Rostral Anterior Cingulate Cortex Activation and Perceived Stress
Within cannabis users, neither left [r = −0.001, p = 0.996] nor right [r = −0.006, p = 0.97] rACC was associated with Perceived Stress Scale total scores.
Rostral Anterior Cingulate Cortex Connectivity and Perceived Stress
Right cerebellum and right rACC connectivity were not significantly related to perceived stress within the cannabis-using group [r = −0.20, p = 0.25], in neither males [r = −0.17, p = 0.45] nor females [r = −0.01, p = 0.97] for rACC calm No-go connectivity finding.
Discussion
The current study is the first to assess relationships between cannabis use, gender, and rACC activation and functional connectivity during an emotional response inhibition task. It was found that abstinent cannabis users, relative to non-using controls, had significantly decreased left and right rACC activation during successful response inhibition upon presentation of fearful faces. Regarding connectivity results, there were no significant differences between abstinent cannabis users and controls in rACC connectivity during fearful or calm response inhibition. Further, group level moderation by gender was non-significant. However, follow-up contrasts revealed that abstinent cannabis-using males, relative to cannabis-using females, demonstrated greater right rACC and right cerebellum connectivity during successful calm No-go trials. Within non-users, gender was not related to rACC connectivity. Interestingly, findings were unrelated to current levels of perceived stress.
The finding that abstinent cannabis users had decreased activation in the right and left rACC during emotional response inhibition complements the ACC structural literature, which has shown relationships between cannabis use and reduced volume and thickness in the ACC (52–57, 102). In conjunction, previous functional studies have also found relationships between cannabis use and reduced ACC activation in response to emotional content and stress (46, 48, 103). Contrarily, Wetherill et al. (104) showed that cannabis users have greater perigenual ACC activation in response to backward masked aversive stimuli. Notably, participants were slightly older, non-abstinent (mean = 1.5 days abstinence), treatment-seeking cannabis users (104), which differs from the abstinent adolescent-young adult users in the current study. Studies have also demonstrated ACC hypoactivation or different distribution of ACC activation in cannabis users during response inhibition tasks without an emotional component (30–33). The present study builds upon these two bodies of literature by showing that cannabis users, following a monitored at least 2-week abstinence period, had rACC hypoactivity during fearful response inhibition after controlling for calm response inhibition.
Similarly, Dreyfuss et al. (78) found that in healthy individuals, right ACC recruitment was associated with successful inhibition of response to fearful non-targets; consistent with the present study’s finding. The current findings extend this literature by demonstrating that controls—compared to cannabis users—recruit the rACC more during fearful relative to calm response inhibition. This suggests that cannabis users do not recruit the rACC to the same extent as controls when successfully inhibiting a motor response when processing a fearful face. Thus, cannabis users may rely on other networks during emotional response inhibition that are hyperactive relative to controls during response inhibition or emotion tasks (25–27, 49, 105), which may serve as a consequence of or a risk for problematic use (106, 107). It is possible that rather than recruiting the rACC during emotional response inhibition, cannabis users recruit areas (e.g., right dorsolateral prefrontal, medial frontal, superior and inferior parietal, right occipital, posterior cingulate, precuneus) that are hyperactive during neutral response inhibition and emotional processing paradigms. A whole-brain activation study would be necessary to address which regions cannabis users recruit during emotional response inhibition. Further, longitudinal studies on ACC development in conjunction with cannabis use are needed to disentangle directionality.
During adolescence and young adulthood, the ACC undergoes significant structural and functional development (108–111). Given the current study’s findings, in conjunction with previous literature showing ACC structural and functional differences, chronic cannabis use may interfere with normal ACC development. The proposed mechanism behind this is a reduction in CB1 receptors within the cingulate cortex associated with chronic cannabis use (112), positing the ACC as particularly vulnerable due to its high density of CB1 receptors (6, 7). Moreover, endocannabinoid signaling via CB1 receptors in the mPFC (including the rACC) regulates the HPA stress response (10, 113). Therefore, damage to the endocannabinoid system via long-term cannabis use may result in abnormal or decreased neural response to aversive stimuli. This is consistent with research suggesting that abnormal adolescent development of ACC structure and function is associated with depression and anxiety (111, 114–116). In the current study, contrary to prediction, decreased rACC activation in cannabis users was not associated with perceived stress and coping ability. It is possible that the neural differences observed in cannabis users during functional MRI precede differences in perceived stress that have yet to emerge, particularly given the study’s exclusion for mood disorders. A longitudinal study would be necessary to address whether decreased rACC activation in abstinent cannabis users predicts emergence into mood disorders.
While decreased rACC activation was observed in abstinent cannabis users, no relationship was then found between rACC connectivity with other cortical regions by cannabis-group status for either fearful or calm response inhibition. Based on previous findings of greater functional connectivity in cannabis users during response inhibition tasks (28, 29, 50, 87, 88), it was hypothesized that greater connectivity in cannabis users would be necessary to achieve successful response inhibition. Perhaps cannabis users had decreased activation in other areas in addition to the rACC, but to different extents, such that no increase or decrease in rACC connectivity relative to controls was apparent. Additionally, in abstinent cannabis users, other regions might compensate for aberrant rACC activation in a manner that results in similar connectivity and performance across both cannabis users and controls. A whole brain activation study would provide insight into the behavior of other regions in abstinent cannabis users during emotional response inhibition. Regions with significant activation in a whole brain analysis could be more appropriate than the rACC as seeds for an ROI connectivity analysis.
While gender did not significantly moderate the relationship between cannabis use and rACC connectivity during either fearful or calm response inhibition, within a contrast of only the cannabis-using group, gender was related to rACC connectivity. Specifically, cannabis-using males, relative to females, demonstrated greater connectivity between the right rACC and right cerebellum during Calm No-go response inhibition. Because no gender interaction was observed, this is considered a marginal finding useful for future directions and should be replicated. On inspection (Figure 3), males’ right rACC and right cerebellum connectivity during Calm response inhibition more closely resembles connectivity of male and female controls. This marginal finding suggests that females may be more vulnerable to the effects of cannabis on cognition and affect, consistent with previous research (54, 82, 84–86). This may potentially be due to differences in gender-specific use patterns (117), though, warrants future investigation in larger sample sizes that can conduct gender-specific analyses. Related to the present findings, the cerebellum has a particularly high density of CB1 receptors and female rats have exhibited increased vulnerability to reduced CB1 receptor expression in the cerebellum under repeated stress (6, 7, 118). Alternatively, it is possible that increased rACC and cerebellum connectivity in cannabis-using males indicates an over-reliance on the cerebellum, which has been observed in substance users during other cognitive tasks (119–122). Thus, cannabis use may differentially impact male and female rACC-cerebellar circuitry during response inhibition. Again, this finding should be replicated in a larger sample.
There are important limitations that possibly contribute to the null connectivity findings. Length of abstinence was relatively long in the current study compared with most studies in the extant literature [mean = 31 days vs. 3 days in most studies of emotion and neurocognition in cannabis users, e.g., Gruber et al. (46); Wesley et al. (48); Zimmermann et al. (49)], thus previously reported findings may reflect fairly acute effects that recover with sustained abstinence. Then, a more lenient decision on individual voxel thresholding (set at p < 0.005) was made to detect more subtle effects (e.g., small to medium effect sizes), yet, research has shown that thresholding at p < 0.001 decreases the likelihood of false positives and bolsters fMRI finding interpretation (123). Thus, findings will need to be replicated in a larger sample in order to detect small effect sizes. Notably, the current study demonstrated decreased rACC activation during emotional response inhibition, even after controlling for calm response inhibition. Thus, the activation results in the current study are consistent with each the emotion processing and response inhibition literatures (30–32), and in turn, may generalize to passively viewing fearful faces or other negatively valenced stimuli (46, 48, 104). Further, as response inhibition impairments may be a risk factor for problematic substance use (106, 107, 124), these findings bolster this literature, yet, the current study is cross-sectional and cannot determine causality of the relationship between cannabis use and inhibitory control. Therefore, longitudinal research is warranted on the causal relationship between cannabis use and inhibitory control, as well as the underlying neural mechanisms.
In the current study, cannabis users exhibited decreased right and left rACC recruitment during successful response inhibition to fearful faces compared to calm faces. These findings build upon previous studies demonstrating ACC hypoactivation during emotion processing and inhibitory control tasks (30–32, 46, 48, 104). Chronic cannabis use during adolescence may interfere with typical development of the rACC and other brain regions important for emotion regulation or may pose as a risk-factor for subsequent use; prospective, longitudinal studies are best-suited to disentangle this relationship. Abnormal ACC development is related to depression and anxiety (111, 114–116). Therefore, rACC functional differences may subsequently emerge into mood differences, predisposing cannabis users to later development of affective disorders. Given the significant development of the rACC during adolescence and potential impact of chronic cannabis use, this region may be important to track structurally and functionally over time in prospective longitudinal studies.
Data Availability Statement
The raw data supporting the conclusions of this article will be made available by the authors, without undue reservation.
Ethics Statement
The studies involving human participants were reviewed and approved by University of Wisconsin-Milwaukee Institutional Review Board. Written informed consent to participate in this study was provided by the participants’ legal guardian/next of kin.
Author Contributions
RS: conceptualization, formal analysis, data curation, writing-original draft, and writing-review and editing. KM: conceptualization, investigation, and writing-review and editing. AW: software, data curation, and writing-review and editing. AT: methodology, software, data curation, and investigation. KL: conceptualization, methodology, investigation, resources, writing-review and editing, project administration, and funding acquisition. All authors contributed to the article and approved the submitted version.
Funding
This work was supported by the National Institute on Drug Abuse [R01 DA030354 (KL) and F31 DA054761 (RS)].
Conflict of Interest
The authors declare that the research was conducted in the absence of any commercial or financial relationships that could be construed as a potential conflict of interest.
Publisher’s Note
All claims expressed in this article are solely those of the authors and do not necessarily represent those of their affiliated organizations, or those of the publisher, the editors and the reviewers. Any product that may be evaluated in this article, or claim that may be made by its manufacturer, is not guaranteed or endorsed by the publisher.
Acknowledgments
We thank the study participants, BraIN lab team, and our funding agencies. Content of this manuscript appear in a thesis conducted at the University of Wisconsin-Milwaukee (125).
References
1. Schulenberg JE, Johnston LD, O’malley PM, Bachman JG, Miech RA, Patrick ME. Monitoring the Future National Survey Results on Drug Use, 1975-2019: Volume II, College Students and Adults Ages 19-60. Ann Arbor, MI: Institute for Social Research, The University of Michigan (2021).
2. Casey BJ, Jones RM, Hare TA. The adolescent brain. Ann N Y Acad Sci. (2008) 1124:111–26. doi: 10.1038/s41582-020-0364-0
3. Blest-Hopley G, Colizzi M, Giampietro V, Bhattacharyya S. Is the adolescent brain at greater vulnerability to the effects of Cannabis? A narrative review of the evidence. Front Psychiatry. (2020) 11:859. doi: 10.3389/fpsyt.2020.00859
4. Bava S, Tapert SF. Adolescent brain development and the risk for alcohol and other drug problems. Neuropsychol Rev. (2010) 20:398–413. doi: 10.1007/s11065-010-9146-6
6. Herkenham M, Lynn AB, Little MD, Johnson MR, Melvin LS, De Costa BR, et al. Cannabinoid receptor localization in brain. Proc Natl Acad Sci USA. (1990) 87:1932–6.
7. Glass M, Dragunow M, Faull RL. Cannabinoid receptors in the human brain: a detailed anatomical and quantitative autoradiographic study in the fetal, neonatal and adult human brain. Neuroscience. (1997) 77:299–318. doi: 10.1016/s0306-4522(96)00428-9
8. Mackie K. Distribution of cannabinoid receptors in the central and peripheral nervous system. In: Pertwee RG editor. Cannabinoids. Berlin, Heidelberg: Springer Berlin Heidelberg (2005). p. 299–325. doi: 10.1007/3-540-26573-2_10
9. Svizenska I, Dubovy P, Sulcova A. Cannabinoid receptors 1 and 2 (CB1 and CB2), their distribution, ligands and functional involvement in nervous system structures–a short review. Pharmacol Biochem Behav. (2008) 90:501–11. doi: 10.1016/j.pbb.2008.05.010
10. McLaughlin RJ, Hill MN, Gorzalka BB. A critical role for prefrontocortical endocannabinoid signaling in the regulation of stress and emotional behavior. Neurosci Biobehav Rev. (2014) 42:116–31. doi: 10.1016/j.neubiorev.2014.02.006
11. Hillard CJ. Circulating endocannabinoids: from whence do they come and where are they going? Neuropsychopharmacology. (2018) 43:155–72. doi: 10.1038/npp.2017.130
12. Gorzalka BB, Hill MN, Hillard CJ. Regulation of endocannabinoid signaling by stress: implications for stress-related affective disorders. Neurosci Biobehav Rev. (2008) 32:1152–60. doi: 10.1016/j.neubiorev.2008.03.004
13. Nigg JT. On inhibition/disinhibition in developmental psychopathology: views from cognitive and personality psychology and a working inhibition taxonomy. Psychol Bull. (2000) 126:220–46. doi: 10.1037/0033-2909.126.2.220
14. Aron AR, Robbins TW, Poldrack RA. Inhibition and the right inferior frontal cortex. Trends Cogn Sci. (2004) 8:170–7. doi: 10.1016/j.tics.2004.02.010
15. Aron AR, Robbins TW, Poldrack RA. Inhibition and the right inferior frontal cortex: one decade on. Trends Cogn Sci. (2014) 18:177–85. doi: 10.1016/j.tics.2013.12.003
16. Nigg JT. Annual research review: on the relations among self-regulation, self-control, executive functioning, effortful control, cognitive control, impulsivity, risk-taking, and inhibition for developmental psychopathology. J Child Psychol Psychiatry. (2017) 58:361–83. doi: 10.1111/jcpp.12675
17. Takagi M, Lubman DI, Cotton S, Fornito A, Baliz Y, Tucker A, et al. Executive control among adolescent inhalant and cannabis users. Drug Alcohol Rev. (2011) 30:629–37. doi: 10.1111/j.1465-3362.2010.00256.x
18. Griffith-Lendering MF, Huijbregts SC, Vollebergh WA, Swaab H. Motivational and cognitive inhibitory control in recreational cannabis users. J Clin Exp Neuropsychol. (2012) 34:688–97. doi: 10.1080/13803395.2012.668874
19. Lisdahl KM, Price JS. Increased marijuana use and gender predict poorer cognitive functioning in adolescents and emerging adults. J Int Neuropsychol Soc. (2012) 18:678–88. doi: 10.1017/S1355617712000276
20. Cousijn J, Watson P, Koenders L, Vingerhoets WA, Goudriaan AE, Wiers RW. Cannabis dependence, cognitive control and attentional bias for cannabis words. Addict Behav. (2013) 38:2825–32. doi: 10.1016/j.addbeh.2013.08.011
21. Dougherty DM, Mathias CW, Dawes MA, Furr RM, Charles NE, Liguori A, et al. Impulsivity, attention, memory, and decision-making among adolescent marijuana users. Psychopharmacology (Berl). (2013) 226:307–19. doi: 10.1007/s00213-012-2908-5
22. Martinez-Loredo V, Fernandez-Hermida JR, Fernandez-Artamendi S, Carballo JL, Garcia-Cueto E, Garcia-Rodriguez O. The association of both self-reported and behavioral impulsivity with the annual prevalence of substance use among early adolescents. Subst Abuse Treat Prev Policy. (2015) 10:23.
23. Scott JC, Wolf DH, Calkins ME, Bach EC, Weidner J, Ruparel K, et al. Cognitive functioning of adolescent and young adult cannabis users in the Philadelphia neurodevelopmental cohort. Psychol Addict Behav. (2017) 31:423–34. doi: 10.1037/adb0000268
24. Wallace AL, Wade NE, Hatcher KF, Lisdahl KM. Effects of cannabis use and subclinical ADHD symptomology on attention based tasks in adolescents and young adults. Arch Clin Neuropsychol. (2019) 34:700–5. doi: 10.1093/arclin/acy080
25. Tapert SF, Schweinsburg AD, Drummond SP, Paulus MP, Brown SA, Yang TT, et al. Functional MRI of inhibitory processing in abstinent adolescent marijuana users. Psychopharmacology (Berl). (2007) 194:173–83. doi: 10.1007/s00213-007-0823-y
26. Roberts GM, Garavan H. Evidence of increased activation underlying cognitive control in ecstasy and cannabis users. Neuroimage. (2010) 52:429–35. doi: 10.1016/j.neuroimage.2010.04.192
27. Wallace AL, Maple KE, Barr AT, Lisdahl KM. BOLD responses to inhibition in cannabis-using adolescents and emerging adults after 2 weeks of monitored cannabis abstinence. Psychopharmacology (Berl). (2020) 237:3259–68. doi: 10.1007/s00213-020-05608-7
28. Filbey FM, Yezhuvath U. Functional connectivity in inhibitory control networks and severity of cannabis use disorder. Am J Drug Alcohol Abuse. (2013) 39:382–91. doi: 10.3109/00952990.2013.841710
29. Harding IH, Solowij N, Harrison BJ, Takagi M, Lorenzetti V, Lubman DI, et al. Functional connectivity in brain networks underlying cognitive control in chronic cannabis users. Neuropsychopharmacology. (2012) 37:1923–33. doi: 10.1038/npp.2012.39
30. Hester R, Nestor L, Garavan H. Impaired error awareness and anterior cingulate cortex hypoactivity in chronic cannabis users. Neuropsychopharmacology. (2009) 34:2450–8. doi: 10.1038/npp.2009.67
31. Eldreth DA, Matochik JA, Cadet JL, Bolla KI. Abnormal brain activity in prefrontal brain regions in abstinent marijuana users. Neuroimage. (2004) 23:914–20. doi: 10.1016/j.neuroimage.2004.07.032
32. Gruber SA, Yurgelun-Todd DA. Neuroimaging of marijuana smokers during inhibitory processing: a pilot investigation. Brain Res Cogn Brain Res. (2005) 23:107–18. doi: 10.1016/j.cogbrainres.2005.02.016
33. Gruber SA, Dahlgren MK, Sagar KA, Gonenc A, Killgore WD. Age of onset of marijuana use impacts inhibitory processing. Neurosci Lett. (2012) 511:89–94. doi: 10.1016/j.neulet.2012.01.039
34. Schmahl CG, Mcglashan TH, Bremner JD. Neurobiological correlates of borderline personality disorder. Psychopharmacol Bull. (2002) 36:69–87.
35. Wingenfeld K, Rullkoetter N, Mensebach C, Beblo T, Mertens M, Kreisel S, et al. Neural correlates of the individual emotional Stroop in borderline personality disorder. Psychoneuroendocrinology. (2009) 34:571–86. doi: 10.1016/j.psyneuen.2008.10.024
36. Devinsky O, Morrell MJ, Vogt BA. Contributions of anterior cingulate cortex to behaviour. Brain. (1995) 118(Pt 1):279–306. doi: 10.1093/brain/118.1.279
37. Bush G, Luu P, Posner MI. Cognitive and emotional influences in anterior cingulate cortex. Trends Cogn Sci. (2000) 4:215–22. doi: 10.1016/s1364-6613(00)01483-2
38. Gasquoine PG. Localization of function in anterior cingulate cortex: from psychosurgery to functional neuroimaging. Neurosci Biobehav Rev. (2013) 37:340–8. doi: 10.1016/j.neubiorev.2013.01.002
39. Etkin A, Egner T, Kalisch R. Emotional processing in anterior cingulate and medial prefrontal cortex. Trends Cogn Sci. (2011) 15:85–93. doi: 10.1016/j.tics.2010.11.004
40. Etkin A, Buchel C, Gross JJ. The neural bases of emotion regulation. Nat Rev Neurosci. (2015) 16:693–700. doi: 10.1038/nrn4044
41. Phan KL, Angstadt M, Golden J, Onyewuenyi I, Popovska A, De Wit H. Cannabinoid modulation of amygdala reactivity to social signals of threat in humans. J Neurosci. (2008) 28:2313–9. doi: 10.1523/JNEUROSCI.5603-07.2008
42. Fusar-Poli P, Placentino A, Carletti F, Landi P, Allen P, Surguladze S, et al. Functional atlas of emotional faces processing: a voxel-based meta-analysis of 105 functional magnetic resonance imaging studies. J Psychiatry Neurosci. (2009) 34:418–32.
43. Bhattacharyya S, Morrison PD, Fusar-Poli P, Martin-Santos R, Borgwardt S, Winton-Brown T, et al. Opposite effects of delta-9-tetrahydrocannabinol and cannabidiol on human brain function and psychopathology. Neuropsychopharmacology. (2010) 35:764–74. doi: 10.1038/npp.2009.184
44. Bossong MG, Van Hell HH, Jager G, Kahn RS, Ramsey NF, Jansma JM. The endocannabinoid system and emotional processing: a pharmacological fMRI study with 9-tetrahydrocannabinol. Eur Neuropsychopharmacol. (2013) 23:1687–97. doi: 10.1016/j.euroneuro.2013.06.009
45. Gorka SM, Fitzgerald DA, De Wit H, Phan KL. Cannabinoid modulation of amygdala subregion functional connectivity to social signals of threat. Int J Neuropsychopharmacol. (2014) 18:yu104. doi: 10.1093/ijnp/pyu104
46. Gruber SA, Rogowska J, Yurgelun-Todd DA. Altered affective response in marijuana smokers: an FMRI study. Drug Alcohol Depend. (2009) 105:139–53. doi: 10.1016/j.drugalcdep.2009.06.019
47. Cornelius JR, Aizenstein HJ, Hariri AR. Amygdala reactivity is inversely related to level of cannabis use in individuals with comorbid cannabis dependence and major depression. Addict Behav. (2010) 35:644–6. doi: 10.1016/j.addbeh.2010.02.004
48. Wesley MJ, Lile JA, Hanlon CA, Porrino LJ. Abnormal medial prefrontal cortex activity in heavy cannabis users during conscious emotional evaluation. Psychopharmacology (Berl). (2016) 233:1035–44. doi: 10.1007/s00213-015-4180-y
49. Zimmermann K, Walz C, Derckx RT, Kendrick KM, Weber B, Dore B, et al. Emotion regulation deficits in regular marijuana users. Hum Brain Mapp. (2017) 38:4270–9. doi: 10.1002/hbm.23671
50. Filbey FM, Dunlop J. Differential reward network functional connectivity in cannabis dependent and non-dependent users. Drug Alcohol Depend. (2014) 140:101–11. doi: 10.1016/j.drugalcdep.2014.04.002
51. Shollenbarger S, Thomas AM, Wade NE, Gruber SA, Tapert SF, Filbey FM, et al. Intrinsic frontolimbic connectivity and mood symptoms in young adult Cannabis users. Front Public Health. (2019) 7:311. doi: 10.3389/fpubh.2019.00311
52. Szeszko PR, Robinson DG, Sevy S, Kumra S, Rupp CI, Betensky JD, et al. Anterior cingulate grey-matter deficits and cannabis use in first-episode schizophrenia. Br J Psychiatry. (2007) 190:230–6. doi: 10.1192/bjp.bp.106.024521
53. Rapp C, Walter A, Studerus E, Bugra H, Tamagni C, Rothlisberger M, et al. Cannabis use and brain structural alterations of the cingulate cortex in early psychosis. Psychiatry Res. (2013) 214:102–8. doi: 10.1016/j.pscychresns.2013.06.006
54. Maple KE, Thomas AM, Kangiser MM, Lisdahl KM. Anterior cingulate volume reductions in abstinent adolescent and young adult cannabis users: association with affective processing deficits. Psychiatry Res Neuroimaging. (2019) 288:51–9. doi: 10.1016/j.pscychresns.2019.04.011
55. Rais M, Van Haren NE, Cahn W, Schnack HG, Lepage C, Collins L, et al. Cannabis use and progressive cortical thickness loss in areas rich in CB1 receptors during the first five years of schizophrenia. Eur Neuropsychopharmacol. (2010) 20:855–65. doi: 10.1016/j.euroneuro.2010.08.008
56. Lisdahl KM, Tamm L, Epstein JN, Jernigan T, Molina BS, Hinshaw SP, et al. The impact of ADHD persistence, recent cannabis use, and age of regular cannabis use onset on subcortical volume and cortical thickness in young adults. Drug Alcohol Depend. (2016) 161:135–46. doi: 10.1016/j.drugalcdep.2016.01.032
57. Jacobus J, Squeglia LM, Sorg SF, Nguyen-Louie TT, Tapert SF. Cortical thickness and neurocognition in adolescent marijuana and alcohol users following 28 days of monitored abstinence. J Stud Alcohol Drugs. (2014) 75:729–43. doi: 10.15288/jsad.2014.75.729
58. Chye Y, Suo C, Lorenzetti V, Batalla A, Cousijn J, Goudriaan AE, et al. Cortical surface morphology in long-term cannabis users: a multi-site MRI study. Eur Neuropsychopharmacol. (2019) 29:257–65. doi: 10.1016/j.euroneuro.2018.11.1110
59. Lorenzetti V, Chye Y, Silva P, Solowij N, Roberts CA. Does regular cannabis use affect neuroanatomy? An updated systematic review and meta-analysis of structural neuroimaging studies. Eur Arch Psychiatry Clin Neurosci. (2019) 269:59–71. doi: 10.1007/s00406-019-00979-1
60. Rossetti MG, Mackey S, Patalay P, Allen NB, Batalla A, Bellani M, et al. Sex and dependence related neuroanatomical differences in regular cannabis users: findings from the ENIGMA addiction working group. Transl Psychiatry. (2021) 11:272. doi: 10.1038/s41398-021-01382-y
61. Hasan NS, Babson KA, Banducci AN, Bonn-Miller MO. The prospective effects of perceived and laboratory indices of distress tolerance on cannabis use following a self-guided quit attempt. Psychol Addict Behav. (2015) 29:933–40. doi: 10.1037/adb0000132
62. Ketcherside A, Filbey FM. Mediating processes between stress and problematic marijuana use. Addict Behav. (2015) 45:113–8. doi: 10.1016/j.addbeh.2015.01.015
63. Moitra E, Christopher PP, Anderson BJ, Stein MD. Coping-motivated marijuana use correlates with DSM-5 cannabis use disorder and psychological distress among emerging adults. Psychol Addict Behav. (2015) 29:627–32. doi: 10.1037/adb0000083
64. Farris SG, Metrik J, Bonn-Miller MO, Kahler CW, Zvolensky MJ. Anxiety sensitivity and distress intolerance as predictors of cannabis dependence symptoms, problems, and craving: the mediating role of coping motives. J Stud Alcohol Drugs. (2016) 77:889–97. doi: 10.15288/jsad.2016.77.889
65. Quinn PD, Fromme K. Self-regulation as a protective factor against risky drinking and sexual behavior. Psychol Addict Behav. (2010) 24:376–85. doi: 10.1037/a0018547
66. Wilcox CE, Pommy JM, Adinoff B. Neural circuitry of impaired emotion regulation in substance use disorders. Am J Psychiatry. (2016) 173:344–61. doi: 10.1176/appi.ajp.2015.15060710
67. Russell BS, Heller AT, Hutchison M. Differences in adolescent emotion regulation and impulsivity: a group comparison study of school-based recovery students. Subst Use Misuse. (2017) 52:1085–97. doi: 10.1080/10826084.2016.1272612
68. Bonn-Miller MO, Vujanovic AA, Boden MT, Gross JJ. Posttraumatic stress, difficulties in emotion regulation, and coping-oriented marijuana use. Cogn Behav Ther. (2011) 40:34–44. doi: 10.1080/16506073.2010.525253
69. Golden CJ. Sex differences in performance on the stroop color and word test. Perceptual Motor Skills. (1974) 39:1067–70. doi: 10.2466/pms.1974.39.3.1067
70. Sarmány I. Different performance in Stroop’s interference test from the aspect of personality and sex. Studia Psychologica. (1977) 19:60–7.
71. Sjoberg EA, Cole GG. Sex differences on the go/no-go test of inhibition. Arch Sex Behav. (2018) 47:537–42. doi: 10.1007/s10508-017-1010-9
72. Killgore WD, Oki M, Yurgelun-Todd DA. Sex-specific developmental changes in amygdala responses to affective faces. Neuroreport. (2001) 12:427–33. doi: 10.1097/00001756-200102120-00047
73. Killgore WD, Yurgelun-Todd DA. Sex differences in amygdala activation during the perception of facial affect. Neuroreport. (2001) 12:2543–7. doi: 10.1097/00001756-200108080-00050
74. Killgore WD, Yurgelun-Todd DA. Sex-related developmental differences in the lateralized activation of the prefrontal cortex and amygdala during perception of facial affect. Percept Mot Skills. (2004) 99:371–91. doi: 10.2466/pms.99.2.371-391
75. Whittle S, Yucel M, Yap MB, Allen NB. Sex differences in the neural correlates of emotion: evidence from neuroimaging. Biol Psychol. (2011) 87:319–33. doi: 10.1016/j.biopsycho.2011.05.003
76. Hall GB, Witelson SF, Szechtman H, Nahmias C. Sex differences in functional activation patterns revealed by increased emotion processing demands. Neuroreport. (2004) 15:219–23. doi: 10.1097/00001756-200402090-00001
77. Kempton MJ, Haldane M, Jogia J, Christodoulou T, Powell J, Collier D, et al. The effects of gender and COMT Val158Met polymorphism on fearful facial affect recognition: a fMRI study. Int J Neuropsychopharmacol. (2009) 12:371–81. doi: 10.1017/S1461145708009395
78. Dreyfuss M, Caudle K, Drysdale AT, Johnston NE, Cohen AO, Somerville LH, et al. Teens impulsively react rather than retreat from threat. Dev Neurosci. (2014) 36:220–7. doi: 10.1159/000357755
79. Viveros MP, Llorente R, Suarez J, Llorente-Berzal A, Lopez-Gallardo M, De Fonseca FR. The endocannabinoid system in critical neurodevelopmental periods: sex differences and neuropsychiatric implications. J Psychopharmacol. (2012) 26:164–76. doi: 10.1177/0269881111408956
80. Rodriguez de Fonseca F, Martin Calderon JL, Mechoulam R, Navarro M. Repeated stimulation of D1 dopamine receptors enhances (-)-11-hydroxy-delta 8-tetrahydrocannabinol-dimethyl-heptyl-induced catalepsy in male rats. Neuroreport. (1994) 5:761–5. doi: 10.1097/00001756-199403000-00006
81. Gonzalez S, Bisogno T, Wenger T, Manzanares J, Milone A, Berrendero F, et al. Sex steroid influence on cannabinoid CB(1) receptor mRNA and endocannabinoid levels in the anterior pituitary gland. Biochem Biophys Res Commun. (2000) 270:260–6. doi: 10.1006/bbrc.2000.2406
82. Rubino T, Vigano D, Realini N, Guidali C, Braida D, Capurro V, et al. Chronic delta 9-tetrahydrocannabinol during adolescence provokes sex-dependent changes in the emotional profile in adult rats: behavioral and biochemical correlates. Neuropsychopharmacology. (2008) 33:2760–71. doi: 10.1038/sj.npp.1301664
83. Crane NA, Schuster RM, Fusar-Poli P, Gonzalez R. Effects of cannabis on neurocognitive functioning: recent advances, neurodevelopmental influences, and sex differences. Neuropsychol Rev. (2013) 23:117–37. doi: 10.1007/s11065-012-9222-1
84. Medina KL, Mcqueeny T, Nagel BJ, Hanson KL, Yang TT, Tapert SF. Prefrontal cortex morphometry in abstinent adolescent marijuana users: subtle gender effects. Addict Biol. (2009) 14:457–68. doi: 10.1111/j.1369-1600.2009.00166.x
85. McQueeny T, Padula CB, Price J, Medina KL, Logan P, Tapert SF. Gender effects on amygdala morphometry in adolescent marijuana users. Behav Brain Res. (2011) 224:128–34. doi: 10.1016/j.bbr.2011.05.031
86. Sullivan RM, Wallace AL, Wade NE, Swartz AM, Lisdahl KM. Cannabis use and brain volume in adolescent and young adult cannabis users: effects moderated by sex and aerobic fitness. J Int Neuropsychol Soc. (2021) 27:607–20. doi: 10.1017/S135561772100062X
87. Feldstein Ewing SW, Chung T, Caouette JD, Ketcherside A, Hudson KA, Filbey FM. Orbitofrontal cortex connectivity as a mechanism of adolescent behavior change. Neuroimage. (2017) 151:14–23. doi: 10.1016/j.neuroimage.2016.12.076
88. Kelly C, Castellanos FX, Tomaselli O, Lisdahl K, Tamm L, Jernigan T, et al. Distinct effects of childhood ADHD and cannabis use on brain functional architecture in young adults. Neuroimage Clin. (2017) 13:188–200. doi: 10.1016/j.nicl.2016.09.012
89. Stewart DG, Brown SA. Withdrawal and dependency symptoms among adolescent alcohol and drug abusers. Addiction. (1995) 90:627–35. doi: 10.1046/j.1360-0443.1995.9056274.x
90. Brown SA, Myers MG, Lippke L, Tapert SF, Stewart DG, Vik PW. Psychometric evaluation of the customary drinking and drug use record (CDDR): a measure of adolescent alcohol and drug involvement. J Stud Alcohol. (1998) 59:427–38. doi: 10.15288/jsa.1998.59.427
91. Sheehan DV, Lecrubier Y, Sheehan KH, Amorim P, Janavs J, Weiller E, et al. The Mini-International Neuropsychiatric Interview (M.I.N.I.): the development and validation of a structured diagnostic psychiatric interview for DSM-IV and ICD-10. J Clin Psychiatry. (1998) 59(Suppl. 20):22–33; quiz 34–57.
92. Sobell LC, Sobell MB. Timeline follow-back. In: Litten RZ, Allen JP editors. Measuring Alcohol Consumption. Totowa, NJ: Humana Press (1992). p. 41–72. doi: 10.1007/978-1-4612-0357-5_3
93. Cohen S, Kamarck T, Mermelstein R. A global measure of perceived stress. J Health Soc Behav. (1983) 24:385–96. doi: 10.2307/2136404
94. Hewitt PL, Flett GL, Mosher SW. The perceived stress scale: factor structure and relation to depression symptoms in a psychiatric sample. J Psychopathol Behav Assessment. (1992) 14:247–57. doi: 10.1007/bf00962631
95. Hare TA, Tottenham N, Davidson MC, Glover GH, Casey BJ. Contributions of amygdala and striatal activity in emotion regulation. Biol Psychiatry. (2005) 57:624–32. doi: 10.1016/j.biopsych.2004.12.038
96. Somerville LH, Hare T, Casey BJ. Frontostriatal maturation predicts cognitive control failure to appetitive cues in adolescents. J Cogn Neurosci. (2011) 23:2123–34. doi: 10.1162/jocn.2010.21572
97. Tottenham N, Tanaka JW, Leon AC, Mccarry T, Nurse M, Hare TA, et al. The NimStim set of facial expressions: judgments from untrained research participants. Psychiatry Res. (2009) 168:242–9. doi: 10.1016/j.psychres.2008.05.006
98. Cox RW. AFNI: software for analysis and visualization of functional magnetic resonance neuroimages. Comput Biomed Res. (1996) 29:162–73. doi: 10.1006/cbmr.1996.0014
99. Desikan RS, Segonne F, Fischl B, Quinn BT, Dickerson BC, Blacker D, et al. An automated labeling system for subdividing the human cerebral cortex on MRI scans into gyral based regions of interest. Neuroimage. (2006) 31:968–80. doi: 10.1016/j.neuroimage.2006.01.021
100. Votruba KL, Langenecker SA. Factor structure, construct validity, and age- and education-based normative data for the Parametric Go/No-Go Test. J Clin Exp Neuropsychol. (2013) 35:132–46. doi: 10.1080/13803395.2012.758239
101. Cox RW, Chen G, Glen DR, Reynolds RC, Taylor PA. FMRI clustering in AFNI: false-positive rates redux. Brain Connect. (2017) 7:152–71. doi: 10.1089/brain.2016.0475
102. Hill SY, Sharma V, Jones BL. Lifetime use of cannabis from longitudinal assessments, cannabinoid receptor (CNR1) variation, and reduced volume of the right anterior cingulate. Psychiatry Res Neuroimaging. (2016) 255:24–34. doi: 10.1016/j.pscychresns.2016.05.009
103. Li CS, Milivojevic V, Constable RT, Sinha R. Recent cannabis abuse decreased stress-induced BOLD signals in the frontal and cingulate cortices of cocaine dependent individuals. Psychiatry Res. (2005) 140:271–80. doi: 10.1016/j.pscychresns.2005.09.002
104. Wetherill RR, Childress AR, Jagannathan K, Bender J, Young KA, Suh JJ, et al. Neural responses to subliminally presented cannabis and other emotionally evocative cues in cannabis-dependent individuals. Psychopharmacology (Berl). (2014) 231:1397–407. doi: 10.1007/s00213-013-3342-z
105. Aloi J, Blair KS, Crum KI, Meffert H, White SF, Tyler PM, et al. Adolescents show differential dysfunctions related to Alcohol and Cannabis use disorder severity in emotion and executive attention neuro-circuitries. Neuroimage Clin. (2018) 19:782–92. doi: 10.1016/j.nicl.2018.06.005
106. Nigg JT, Wong MM, Martel MM, Jester JM, Puttler LI, Glass JM, et al. Poor response inhibition as a predictor of problem drinking and illicit drug use in adolescents at risk for alcoholism and other substance use disorders. J Am Acad Child Adolesc Psychiatry. (2006) 45:468–75. doi: 10.1097/01.chi.0000199028.76452.a9
107. McNamee RL, Dunfee KL, Luna B, Clark DB, Eddy WF, Tarter RE. Brain activation, response inhibition, and increased risk for substance use disorder. Alcohol Clin Exp Res. (2008) 32:405–13. doi: 10.1111/j.1530-0277.2007.00604.x
108. Segalowitz SJ, Dywan J. Individual differences and developmental change in the ERN response: implications for models of ACC function. Psychol Res. (2009) 73:857–70. doi: 10.1007/s00426-008-0193-z
109. Pfeifer JH, Peake SJ. Self-development: integrating cognitive, socioemotional, and neuroimaging perspectives. Dev Cogn Neurosci. (2012) 2:55–69. doi: 10.1016/j.dcn.2011.07.012
110. Tamnes CK, Walhovd KB, Torstveit M, Sells VT, Fjell AM. Performance monitoring in children and adolescents: a review of developmental changes in the error-related negativity and brain maturation. Dev Cogn Neurosci. (2013) 6:1–13. doi: 10.1016/j.dcn.2013.05.001
111. Lichenstein SD, Verstynen T, Forbes EE. Adolescent brain development and depression: a case for the importance of connectivity of the anterior cingulate cortex. Neurosci Biobehav Rev. (2016) 70:271–87. doi: 10.1016/j.neubiorev.2016.07.024
112. Hirvonen J, Goodwin RS, Li CT, Terry GE, Zoghbi SS, Morse C, et al. Reversible and regionally selective downregulation of brain cannabinoid CB1 receptors in chronic daily cannabis smokers. Mol Psychiatry. (2012) 17:642–9. doi: 10.1038/mp.2011.82
113. Micale V, Drago F. Endocannabinoid system, stress and HPA axis. Eur J Pharmacol. (2018) 834:230–9. doi: 10.1016/j.ejphar.2018.07.039
114. Clauss JA, Avery SN, Vanderklok RM, Rogers BP, Cowan RL, Benningfield MM, et al. Neurocircuitry underlying risk and resilience to social anxiety disorder. Depress Anxiety. (2014) 31:822–33. doi: 10.1002/da.22265
115. Swartz JR, Phan KL, Angstadt M, Klumpp H, Fitzgerald KD, Monk CS. Altered activation of the rostral anterior cingulate cortex in the context of emotional face distractors in children and adolescents with anxiety disorders. Depress Anxiety. (2014) 31:870–9. doi: 10.1002/da.22289
116. Ho TC, Sacchet MD, Connolly CG, Margulies DS, Tymofiyeva O, Paulus MP, et al. Inflexible functional connectivity of the dorsal anterior cingulate cortex in adolescent major depressive disorder. Neuropsychopharmacology. (2017) 42:2434–45. doi: 10.1038/npp.2017.103
117. Cuttler C, Mischley LK, Sexton M. Sex differences in cannabis use and effects: a cross-sectional survey of cannabis users. Cannabis Cannabinoid Res. (2016) 1:166–75. doi: 10.1089/can.2016.0010
118. Xing G, Carlton J, Zhang L, Jiang X, Fullerton C, Li H, et al. Cannabinoid receptor expression and phosphorylation are differentially regulated between male and female cerebellum and brain stem after repeated stress: implication for PTSD and drug abuse. Neurosci Lett. (2011) 502:5–9. doi: 10.1016/j.neulet.2011.05.013
119. Desmond JE, Chen SH, Derosa E, Pryor MR, Pfefferbaum A, Sullivan EV. Increased frontocerebellar activation in alcoholics during verbal working memory: an fMRI study. Neuroimage. (2003) 19:1510–20. doi: 10.1016/s1053-8119(03)00102-2
120. Hester R, Garavan H. Executive dysfunction in cocaine addiction: evidence for discordant frontal, cingulate, and cerebellar activity. J Neurosci. (2004) 24:11017–22. doi: 10.1523/JNEUROSCI.3321-04.2004
121. Bolla KI, Eldreth DA, Matochik JA, Cadet JL. Neural substrates of faulty decision-making in abstinent marijuana users. Neuroimage. (2005) 26:480–92. doi: 10.1016/j.neuroimage.2005.02.012
122. Behan B, Connolly CG, Datwani S, Doucet M, Ivanovic J, Morioka R, et al. Response inhibition and elevated parietal-cerebellar correlations in chronic adolescent cannabis users. Neuropharmacology. (2014) 84:131–7. doi: 10.1016/j.neuropharm.2013.05.027
123. Woo CW, Krishnan A, Wager TD. Cluster-extent based thresholding in fMRI analyses: pitfalls and recommendations. Neuroimage. (2014) 91:412–9. doi: 10.1016/j.neuroimage.2013.12.058
124. Mahmood OM, Goldenberg D, Thayer R, Migliorini R, Simmons AN, Tapert SF. Adolescents’ fMRI activation to a response inhibition task predicts future substance use. Addict Behav. (2013) 38:1435–41. doi: 10.1016/j.addbeh.2012.07.012
Keywords: cannabis, neuroimaging, adolescents, affective processing, inhibitory control
Citation: Sullivan RM, Maple KE, Wallace AL, Thomas AM and Lisdahl KM (2022) Examining Inhibitory Affective Processing Within the Rostral Anterior Cingulate Cortex Among Abstinent Cannabis-Using Adolescents and Young Adults. Front. Psychiatry 13:851118. doi: 10.3389/fpsyt.2022.851118
Received: 09 January 2022; Accepted: 28 February 2022;
Published: 28 March 2022.
Edited by:
Lucy J. Troup, University of the West of Scotland, United KingdomReviewed by:
Grace Blest-Hopley, King’s College London, United KingdomLiangsuo Ma, Virginia Commonwealth University, United States
Copyright © 2022 Sullivan, Maple, Wallace, Thomas and Lisdahl. This is an open-access article distributed under the terms of the Creative Commons Attribution License (CC BY). The use, distribution or reproduction in other forums is permitted, provided the original author(s) and the copyright owner(s) are credited and that the original publication in this journal is cited, in accordance with accepted academic practice. No use, distribution or reproduction is permitted which does not comply with these terms.
*Correspondence: Krista M. Lisdahl, a3Jpc3RhLm1lZGluYUBnbWFpbC5jb20=