- Department of Neurosurgery, The Second Affiliated Hospital, Zhejiang University School of Medicine, Hangzhou, China
Background: Deep brain stimulation (DBS) has been proposed as a last-resort treatment for major depressive disorder (MDD) and has shown potential antidepressant effects in multiple clinical trials. However, the clinical effects of DBS for MDD are inconsistent and suboptimal, with 30–70% responder rates. The currently used DBS targets for MDD are not individualized, which may account for suboptimal effect.
Objective: We aim to review and summarize currently used DBS targets for MDD and relevant diffusion tensor imaging (DTI) studies.
Methods: A literature search of the currently used DBS targets for MDD, including clinical trials, case reports and anatomy, was performed. We also performed a literature search on DTI studies in MDD.
Results: A total of 95 studies are eligible for our review, including 51 DBS studies, and 44 DTI studies. There are 7 brain structures targeted for MDD DBS, and 9 white matter tracts with microstructural abnormalities reported in MDD. These DBS targets modulate different brain regions implicated in distinguished dysfunctional brain circuits, consistent with DTI findings in MDD.
Conclusions: In this review, we propose a taxonomy of DBS targets for MDD. These results imply that clinical characteristics and white matter tracts abnormalities may serve as valuable supplements in future personalized DBS for MDD.
Introduction
Major depressive disorder (MDD) is a type of mood disorder characterized by significant and persistent depressed mood with various degrees of cognitive and behavioral changes. According to the World Health Organization, MDD will be ranked first in disease burden worldwide by 2030 (1). The effectiveness of antidepressant medications is limited, and up to 35% of MDD cases remain recurrent and resistant to medications (2). Deep brain stimulation (DBS), as a promising neuromodulation therapy, has shown potential antidepressant effects in otherwise-refractory MDD.
DBS exerts electric impulse to modulate neuronal activity and dysfunctional brain circuits, and serves as a therapy for various neurological disorders, including movement disorders, epilepsy, pain, and psychiatric disorders. DBS was first tested as a potential treatment for MDD in a clinical setting in 2005 (3). Since then, open-label clinical trials are performed via different targets with response rates varying from 36 to 60% at 1-year follow-up (4). Recently, two double-blind, randomized, sham-controlled trials failed to show statistically significant improvement in terms of antidepressant efficacy of DBS for MDD in stimulation group vs. sham group (5, 6). As a result, DBS remains an investigational treatment for MDD. There are many contributing factors, one of which is the diversity of brain circuits modulated by DBS between individuals from a group of heterogeneous MDD patients. Recent studies indicate that white matter tracts (WMT) play a crucial role in DBS for MDD (7), and DBS alleviates depressive symptoms by modulating neural network involved via fiber connections (8). However, currently used DBS targets for MDD are not based on personalized circuit targeting, which may lead to inaccurate modulation and suboptimal effects (9).
Diffusion weight imaging (DWI) is a non-invasive technique sensitive to water movement to quantify the tissue diffusion rate within imaging voxel. DTI is a specific type of modeling of the DWI using at least six diffusion measurements and directions. It can be used for studying WMT microintegrity by properties, such as fractional anisotropy (FA), mean diffusivity (MD), apparent diffusion coefficient (ADC), and axial and radial diffusivity in disease and healthy control. Regions of interest (ROIs), voxel-based analysis and tract-based spatial statistics (TBSS) are typically performed for group statistical analysis after obtaining parametric maps (10). Overall, DTI is a promising tool for studying in vivo WMT microstructure abnormality and neural circuit dysfunction, which are implicated in MDD. This conceptualize new insights into different models of dysfunctional brain circuits in MDD patients, including default mode, salience, negative affect, positive affect, attention, and cognitive control, while suited treatment is proposed for each model (11). DTI can also be used for tractography based on the primary eigenvector of diffusion to obtain three-dimensional representations of WMT. Tractography based on DTI unravels the relationship between DBS targets and associated WMT, which may elucidate the action mechanism of DBS for each target, and provide a practical utility for personalized targeting. There are two kinds of tractography methods: probabilistic and deterministic. Deterministic tractography reconstruct one fiber from each seed based on maximum vector, while probabilistic approaches take into account the uncertainty of the estimation and provides probability maps for each seed. Connectomic DBS is gradually receiving more and more endorsement for its ability to achieve network-level targeting. Here, we speculate that different DBS targets for MDD modulate different dysfunctional neural circuits, and conduct a systematic review of existing DBS targets for MDD to present a taxonomy of modulated WMT and dysfunctional brain circuits.
Methods
This systematic review was conducted following the 2020 PRISMA guideline (12). To summarize the DBS clinical trials for MDD, articles and review papers were searched from January 2005 to June 2021 using PubMed. Combinations of 3 medical subject heading terms (i.e., “depressive disorder,” “major, treatment-resistant,” and “depression”) and 3 keywords, including “deep brain stimulation,” “DBS,” and “electrical stimulation”, were used as search criteria. The reference lists of relevant articles were also screened. The articles include case reports, open-label trials and randomized controlled trials, using DBS treatment for MDD. A total of 51 clinical studies of DBS for MDD were matched. Then, the latter set of keywords was replaced by the terms “diffusion tensor imaging,” “DTI,” or “white matter tracts” in the search process for DTI-relevant literature from January 2010 to June 2021. The articles using DTI to study abnormal WMT in MDD were included, and a total of 44 MDD DTI studies were matched. The search was limited to articles published in English. Three co-authors (QY, XXG, and ZLZ) searched and assessed studies independently to ensure accuracy and completeness, and to reduce selection bias. Different terminologies are used in different DTI studies to describe similar WMT (e.g., genu of the cingulate cortex vs. forceps minor), which remains a source of information bias despite the co-authors' best effort to eliminate it.
Results
Review of the DBS Targets and Associated WMT
DBS is used as a treatment for MDD since 2005 (3). Here we summarize currently used DBS targets for MDD as well as its associated WMT through anatomic and connectomic studies. An overview of DBS targets for MDD is listed in Supplementary Table 1.
Subcallosal Cingulate Gyrus (SCG)
In 2005, Mayberg et al. first proposed the SCG as an effective target for treating MDD, with four of six patients experiencing remission at 6 months (3). A series of open-label trials followed, with moderately satisfactory effects, showing expected responder rates between 55 and 75% (13–15). Several case reports also demonstrated the effectiveness of SCG-DBS (16–21). In a preliminary, double-blind, randomized, sham-controlled, crossover trial, 4 out of 5 patients were remitted after 6 months of stimulation, and none of them experienced relapse (22). The therapeutic efficacy varies with the length of stimulation time. An immediate antidepressive consequence of SCG-DBS was reported in an open-label trial (23). In the studies by Kennedy et al. and Holtzheimer et al., considerable responsiveness and remission rates were observed even 3–6 years after DBS implantation (14). Moreover, an open-label study reported that 28 participants experienced a robust and sustained antidepressant response to SCG-DBS in a 2–8 years observation (24). On the other hand, Merkl et al. reported that merely 33% of participants showed an antidepressive response within 24–36 weeks, and there was no significant difference of effectiveness between the active and sham groups (25). In addition, a randomized, double-blind, sham-controlled trial failed to demonstrate significant antidepressant efficacy of SCG-DBS during double-blind period (6). Similarly, another double-blind study of 8 MDD patients showed no significant difference in responder rates in SCG-DBS vs. sham (26). Altogether, these data suggest that SCG-DBS could be efficacious for the management of MDD, but how to improve the responder rate effectively remains a challenge for clinicians.
The SCG is located at the center of a rich network of fiber connections including the cingulum bundle (CB), forceps minor (FM), and uncinate fasciculus (UF), and projects to the orbitofrontal cortex (OFC), anterior cingulate cortex (ACC), thalamus, ventral striatum, hippocampus, amygdala, and temporal lobe (27). Howell et al. suggested that CB and FM were the most likely targeted WMT for SCG-DBS (28). Studies suggested that medial frontal cortex via FM and UF, cingulate cortex via CB, and subcortical nuclei were the critical activation volumes for responders (7), and antidepressant responses were only demonstrated in the patient who had strong connectivity of the stimulation areas to the medial prefrontal cortex (mPFC) (29). Riva-Posse et al. and Choi et al. used post-operative and intraoperative tractography mapping of the “depression switch” of SCG-DBS and demonstrated that responders showed distinct activation pathways from the ventromedial prefrontal cortex (vmPFC) via the FM and UF and the rostral and dorsal cingulate cortex via the CB (7, 30). For this purpose, Riva-Posse et al. used tractography-based surgical targeting for CB, UF, and FM, and reported 9 of 11 patients responding to the treatment at 2 years (31). Lujan et al. used a tractography-activation model tool and found that the most therapeutic electrode contacts primarily projected to the brain regions associated with the vmPFC, nucleus accumbens (NAcc), and CB, and any small differences in the electrode site may produce substantial differences in the activated pathways (32).
Medial Forebrain Bundle (MFB)
Schlaepfer et al. first reported the clinical antidepressant effect of MFB-DBS (33). Fenoy et al. assessed the efficacy of MFB-DBS and reported two out of three patients continued to have more than 80% decrease in Montgomery Asberg Depression Rating Scale (MADRS) scores at 26 weeks. Fenoy et al. further reported that four out of five patients had a 70% decrease in MADRS scores relative to baseline at 52 weeks in a longitudinal study (34). An open-label trial of DBS of the MFB in MDD suggested that 6 out of 8 participants responded at 1 year, including 4 patients who achieved total remission (35). Furthermore, the antidepressant efficacy remained stable for up to 4 years, which suggested acute and sustained antidepressant efficacy (36). Recently, Coenen et al. provided long-term data for a small phase I, randomized controlled clinical trial. They reported that all patients reached the response criterion, 63% responded within a week, and 50% were classified as remitters after 12 months of stimulation (37). No evidence of side effects has yet been reported, but a case study reported that a patient suffered from blurred vision after 10 months (38).
The MFB incorporates mesolimbic pathways that originate from the ventral tegmental area (VTA) and projects to the NAcc and the prefrontal cortex (PFC). It is a central component of the mesolimbic–mesocortical dopamine reward system (39, 40). MFB-DBS could activate the mesocorticolimbic system by increasing neuronal activity through the modulation of dopaminergic and glutamatergic neurotransmission (41). MFB serves both as a specific stimulation target and the center of the reward pathway simultaneously. Nonetheless, the complex midbrain area contains tightly intertwined myelinated fibers. There is an ongoing debate about which WMT actually contributes to anti-depressive mechanism. The VTA dopaminergic axons do not travel within internal capsule, while superolateral MFB (slMFB), a branch undercuts the thalamus, moving laterally toward the internal capsule in its ventral portion, and then goes profoundly into Nacc and PFC, may contribute to the antidepressant effect (42–44).
Nucleus Accumbens
The effectiveness of NAcc-DBS has been reported in several trials (45, 46). An improvement in the Hamilton Depression Rating Scale (HDRS) was observed in 3 out of 4 patients in an open-label trial, whose moods improved simultaneously (45). In addition, an open-label trial with 10 patients reported 50% responder rates of HDRS scores 1 year after the implantation of NAcc-DBS (47). Long-term trial reported a sustained antidepressant effect of up to 4 years, with 5 out of 11 patients reaching the response criterion (46). Meanwhile, the neuropsychological safety of NAcc-DBS for MDD was demonstrated in a 12-month follow-up study (48).
Anatomically, the NAcc is divided into the core and the shell, which receive motor and limbic system information, respectively (49). In general, efferents of the NAcc project to the cingulate gyrus, ventral pallidum, and thalamus (50). The afferents to the NAcc are glutamatergic from the PFC, hippocampus, and amygdala, which excites neurons of the NAcc to establish roles in the neurocircuitry of pleasure and reward (51). The NAcc and SCG have close relationships with direct fiber connections, and the underlying tracts are the CB, FM, and a part of the UF (52). The strength of the connections between the NAcc stimulation sites and the medial and lateral PFC significantly predicted clinical improvement in obsessive-compulsive disorder (OCD) based on diffusion magnetic resonance imaging (53), but similar studies have not been reported in MDD yet. As the clinical effect and mechanism of NAcc-DBS remain a matter of debate, pilot studies are needed to prove effective stimulation target localization and possible brain networks in NAcc-DBS. In anatomical studies, the fiber pathway passing adjacent to or connecting to the NAcc has eight tracts, including the CB, UF, and FM, forming a capsule around the sides of the Nacc (52). Another study combining anatomical structure and tractography finds NAcc-DBS involves modulation of the anterior thalamic radiation (ATR), inferior fronto-occipital fasciculus (IFOF), and inferior longitudinal fasciculus (ILF) (54). MFB pass through the NAcc, and then the fibers extend toward the OFC and PFC (40).
Ventral Capsule/Ventral Striatum (VS/VS)
The VC/VS is crucial in the cortico-striatal-pallidal neural circuits and is vital in reward and motivation (55). VC/VS-DBS was first employed in patients with OCD. During these studies, it was found that the subjects' comorbid depressive symptoms also significantly improved (56, 57). These results led to an initial open-label trial of VC/VS-DBS for MDD, and the trials showed favorable response rates (response rates with the HDRS were 40% at 6 months and 53.3% at last follow-up) (58, 59). Meanwhile, a case report described a single responder after VC/VS-DBS who showed cessation of smoking, which indicated that VC/VS-DBS might compensate for reward deficits and lead to reduced smoking (60). These positive results led to randomized controlled trials. However, the results were contradictory. Dougherty et al. suggested VC/VS-DBS is not an efficacious therapy for MDD, as the response rates at 12, 18, and 24 months during the continuation phase were 20%, 26.7%, and 23.3%, respectively (5). Additionally, the application of VC/VS-DBS was also referred to as ventral-ALIC-DBS (a brain structure that is slightly anterior and ventral to the VC/VS) in some studies (61–63). Bergfeld et al. showed that ventral-ALIC-DBS resulted in a significant decrease in depressive symptoms in 10 out of 25 patients and was well-tolerated (63).
Different targets may modulate the same neural network that is responsible for clinical improvement. Studies by Li et al. and van der Vlis et al. showed that a subpart of the ALIC, which connects areas of the prefrontal cortex with the subthalamic nucleus and medial nucleus of the thalamus, is associated with an effective response in VC/VS stimulation for refractory OCD (64, 65). Previous studies using DTI showed that the ventral ALIC contains two fiber bundles: the ATR and the slMFB, which are implicated in reward and punishment functions (42). slMFB emerged as a target for the treatment of MDD and provided dopaminergic input from the brainstem. Another study argued that clinical behavioral improvements with either ventral-ALIC- or NAcc-DBS result from activation of the slMFB (66). Our previous tractography study showed that ALIC-DBS also activated IFOF and FM, which projected to the prefrontal cortex, ventral striatum, and occipital lobe (54).
Bed Nucleus of the Stria Terminalis (BNST)
To date, only few clinical reports of BNST-DBS for MDD are available. In a case report, a patient with severe MDD combined with anorexia nervosa received a DBS implant in the MFB but treatment was discontinued due to the side effects of blurred vision after 2 years. BNST-DBS was then employed, which resulted in profound and persistent improvement (38). In another case study, the authors had a longitudinal neuropsychological assessment performed for an MDD patient following 12 months of BNST-DBS, and significant clinical improvements in mood and anxiety were indicated post-stimulation (67). A pilot open-label clinical study was conducted in five patients, which presented sustained remission of depressive symptoms in two participants, substantial antidepressant effects in two patients, but had minimal therapeutic effects in one patient (68). Neumann et al. recorded local field potential activity in 7 MDD patients who received DBS electrode implants in the BNST and proposed that α-activity in the limbic system may be a biomarker of symptom severity in MDD (69). BNST is a potential target for MDD, but further exploration is warranted in larger, well-designed clinical trials.
The BNST, located in the immediate vicinity of the VC/VS and NAcc regions, is part of the limbic system (70) and has projections to many structures associated with reward, stress, and anxiety processing (71). An in-depth dissection of the structural connectivity of the BNST is of utter importance to decipher its role in depression. Avery et al. showed structural and functional connections with BNST convergence in the NAcc, thalamus, hippocampus, pallidum, caudate, and putamen via stria teminalis (72). Moreover, the BNST is connected to several brainstem structures via the MFB and the periventricular system. Kruger et al. used probabilistic fiber tracking methods to examine the connectivity mode of the human BNST in vivo, and there were three distinct pathways: the stria terminalis as a posterior pathway to the lateral amygdala, a ventral pathway toward the hypothalamus, and the medial amygdala via the ansa peduncularis (73). These findings suggested that BNST-DBS may produce modulatory effects on the cortico-subcortical and slMFB reward circuit.
Inferior Thalamic Peduncle (ITP)
Several case reports have described the outcomes of ITP-DBS in two patients with MDD with favorable outcomes (74–76). More recently, in a double-blind crossover study, the effects of DBS at the ITP and ALIC-BNST targets were compared in patients suffering from MDD. Although both ITP and ALIC-BNST stimulation may alleviate depressive symptoms, only 1 patient out of 7 preferred ITP over ALIC-BNST stimulation (77).
The ITP is a structure of WM fibers that transmits bidirectional information from the midline and intralaminar thalamic nuclei of the non-specific thalamic system to the OFC (75). The disruption of the thalamo-orbitofrontal system ameliorates depressive symptoms, and the ITP together with the nucleus reticularis thalami plays a vital role in the pathophysiology of MDD (78). The ITP-DBS activate fibers engaged in ATR, MFB, and IFOF, which are consistent with the above anatomical structure involved in the thalamo-orbitofrontal circuit.
Lateral Habenula (LH)
Sartorius et al. firstly reported a significant remission of depressive symptoms after 4 months of LH-DBS in a patient with MDD, and an obvious rekindling of depressive symptoms occurred after erroneous suspension of the stimulation (79). More precisely, they put electrodes into the main limbic afferent WMT of the LHb (stria medullaris) for two participants in the same trial. Both of them had improvements of more than 50% on a depressive symptom scale (80). Recently, a case report revealed that a patient with DBS of bilateral LH achieved significant clinical improvement at 12 weeks follow-up (81). They demonstrated the feasibility LH-DBS for MDD, but larger clinical trials are necessary to confirm its efficacy.
The LH is a compact nucleus that appears as a triangular ridge stretching into the third ventricle on the dorsomedial surface of the caudal thalamus (82). It receives the afferent pathway of the limbic system via the stria medullaris from the amygdala, and provides an efferent pathway to the BNST and dorsal raphe nucleus. Then, these fibers project to several target brain regions, such as the hippocampus, hypothalamus, amygdala, and cerebral cortex, ultimately playing an important role in emotion regulation.
The halt of a pivotal clinical trial of DBS for MDD marks the urgent need of understanding the action mechanism of DBS for MDD (6). Recent studies reveal that WMT connectivity plays an essential role in the clinical effect of DBS for MDD. Riva-Posse et al. identify four fiber pathways associated with antidepressant efficacy in SCG-DBS (7). Similar findings are reported by Lujan et al. (32). In addition, Coenen et al. propose that targeting superolateral branch of MFB is critical for effective MFB-DBS (83). These findings of association between WMT connectivity and clinical effect form a hypothesis that DBS for MDD exert antidepressant efficacy via WMT modulation, especially modulation of the abnormal WMT in MDD. In the following review, we summarize abnormal WMT in MDD based on DTI studies to reveal relevant WMT in DBS for MDD.
Review of Abnormal WMT Associated With MDD
DTI is a variant of diffusion-weighted imaging, which is capable to assess microstructural changes in the brain using water molecule degree of anisotropy and structural orientation in each voxel. It uncovers different fiber connectivity integrity between diseased and healthy subjects by quantitative power. Voxel-based analysis and tract-based spatial statistics can be applied to extract summary measures from brain regions of interest (84). In this part, we summarize the abnormal WMT in MDD discovered in previous DTI studies (Supplementary Table 2).
Cingulum Bundle
CB runs through the cingulate gyrus superior to the corpus callosum, connecting the medial frontal, parietal, occipital, and temporal lobes, and the cingulate cortex. One previous study reported increased FA in the left posterior part of the CB (85). The majority of articles reported reduced FA values in MDD patients. These fibers are in the left CB (86, 87), anterior CB (85, 88) and bilateral CB (89–91). Another two studies by Zhang et al. and Carballedo et al. found that the CB had no alteration in FA and its subregions (92, 93). In summary, most studies have shown that FA is reduced in the CB in patients with MDD.
Medial Forebrain Bundle
MFB is an important pathway for connecting the limbic forebrain, midbrain, and cerebellum, and plays a key role in the reward circuit (42). The main tract splits into two distinct directions through the VTA (42). The inferomedial MFB traces the wall of the third ventricle anteriorly until reaching the lateral hypothalamus, and the slMFB branches into the NAcc, and then project out to the OFC and PFC (39, 42). Brache et al. reported reduced FA in the right VTA-lOFC (lateral OFC) and VTA-dlPFC (dorsal lateral prefrontal cortex) connections in melancholic patients (94). In addition, a battery of DTI studies reported reduced FA in depressed patients in the left (95, 96), right (97), and bilateral ALIC (98–100). Similarly, decreased FA values were reported in relevant frontal brain regions (100–103). These studies may reflect white matter microstructure alterations of the slMFB. However, one tractography study showed that the microstructure of the MFB in remitted depressed participants did not differ from participants without history of depression (104). Identification of MFB can be technically challenging due to extensive overlap of different fibers. For instance, the ATR is located closely and overlaps partially with the slMFB (42). Thus, further tractography studies should explore the differential role of ATR and slMFB in depression. Innovative fiber tracking techniques may provide more accurate fiber identification results in regions with crossing fibers (105).
Uncinate Fasciculus
UF is a significant bundle that connects the anterior temporal lobe, including the hippocampus and amygdala, with the medial and lateral frontal cortex. Several studies have suggested that the FA value in the UF is reduced in patients with depression, including the left (106), right (107), and bilateral UF (87, 89, 91, 108, 109). However, increase in FA has also been reported (110). Further evidence for microstructural changes in the UF may arise from alterations in the temporal and frontal brain areas, potentially incorporating the UF. Reduced FA values in the frontal regions have been reported in the left middle frontal gyrus (101), right frontal lobe (102, 111), and bilateral frontal regions (99, 100, 103, 112). Furthermore, reduced FA in the left middle frontal gyrus and the right inferior frontal gyrus was found in unipolar depressed patients compared with healthy controls (113). Likewise, reduced FA values were found in the temporal lobe, such as the right parahippocampal gyrus (95), bilateral temporal white matter (103), left limbic lobe uncus (101) and bilateral temporal lobe (108).
Forceps Minor
FM courses along the anteromedial surface of the NAcc toward the frontal poles to connect to the prefrontal and medial orbitofrontal brain regions, and the NAcc from side to side (52). Given that the vital function of the PFC and the NAcc are implicated in reward-processing incentives, it is not surprising that the broken integrity of the FM may lead to the development of MDD. Specifically, FA alteration in corpus callosum was found to be the most common results in a meta-analysis (86, 114). Yang et al. found that first-episode medication-naive MDD patients exhibited reduced FA in the left FM compared with healthy controls, and the mean FA values were significantly correlated with anhedonia (86). Meanwhile, Seok et al. and Murphy et al. reported that FA reductions in the bilateral FM, which connected the medial sides of the PFC, were known to be involved in depressive symptoms (88, 91). Another study was employed to demonstrate the abnormalities of the genu of the corpus callosum, which showed that reduced FA may contribute to the pathogenesis of treatment-responsive MDD (97). A similar finding was reported in a study by Lu et al., which showed diminished integrity within the genu of the corpus callosum and the FM in young healthy subjects with high-trait anxiety (115).
Anterior Thalamic Radiation
The ATR connects the prefrontal lobe (mainly in the dlPFC) and the thalamus through the ALIC. It is difficult to identify the two fibers in the ALIC, MFB, and ATR, but they have different functional roles in the pathogenetic mechanism of MDD (42). Previous studies have found reduced FA in the left ATR, and the abnormal diffusion portion was primarily located in the dlPFC area (107). Walther et al. delineated that FA values in MDD in the left ATR are decreased (116). Bessette et al. and Lai and Wu reported that white matter integrity in patients with MDD have lower FA values in the right ATR (99, 117). Moreover, researches showed that in participants with anxiety-related disorders, the integrity of the bilateral ATR is diminished (115, 118). Owing to the difficulty in distinguishing the ATR and MFB among the ALIC section, some studies suggested that ALIC changes may also contain areas of the ATR (95).
Inferior Fronto-Occipital Fasciculus
The IFOF, which plays a pivotal role in frontal-subcortical circuits, projects from the occipital lobe, striatum, and thalamus into the frontal cortex. A meta-analysis of DTI studies in patients with MDD showed reduced FA in the IFOF traversing the right fusiform gyrus of the temporal lobe and the ILF (119). Several DTI studies demonstrated reduced FA in the IFOF in MDD patients (8, 87, 89, 99, 120–122). Recently, Sugimoto et al. found that the FA values of the bilateral IFOF and the genu of the corpus callosum in MDD patients were significantly decreased and inversely correlated with IL-1β levels (microstructural changes in the IFOF and the genu of the corpus callosum are associated with high IL-1β levels in the early stage of MDD) (8). Compared with controls, Wang et al. found decreased FA in right IFOF in late-onset depression. The results from this study also showed that WMT structural connectomic changes correlated with cognitive deficits (123).
Inferior Longitudinal Fasciculus
The ILF connects the occipital and anterior temporal lobes and projects to the lateral and medial anterior temporal regions. The investigation of the ILF suggested that it may be involved in facial recognition, visual perception, reading, and visual memory (124). While the function of the ILF is poorly understood, several studies found reduced FA in the ILF in MDD patients (119, 125). Liang et al. reported reduced FA in the bilateral ILF and part of the left ILF in two MDD subtypes (subgroup 1, deficits in sustained attention and delayed memory; subgroup 2, dysfunction in delayed memory) (125). Zheng et al. also reported reduced FA in the left ILF in MDD (126).
Corticospinal Tract
The CST runs through the internal capsule, which projects into the basal ganglia and separates the thalamus from the putamen, globus pallidus, and caudate nucleus, and is associated with MFB. The CST is involved in the processing and coordination of sensorimotor information that may be associated with motor retardation and slower reaction times in MDD (106). Previous studies reported reduced FA in the CST in adolescents and children with MDD (89, 99). However, the functional significance is yet to be revealed. Vilgis et al. found reduced FA in the left CST, and a significant positive association with anxious-depressed symptoms by exploratory post-hoc analysis (106). Recently, Liang et al. reported widespread FA reduction in the superior portion of the bilateral CST in patients with MDD who suffered more deficits in sustained attention and delayed visual memory (125). Reduced FA in the bilateral CST was also reported in drug-naive patients with MDD (8).
Superior Longitudinal Fasciculus
The SLF connects the PFC, temporal lobe, occipital lobe, parietal lobe and limbic system, and plays an important role in emotion regulation and cognitive function (87, 90, 107). Several DTI studies reported decreased FA in the SLF in MDD compared to controls (88, 90, 91, 111, 117, 122, 123, 127, 128). Previous studies showed that increased depression severity was negatively correlated with decreased integrity in the SLF (129, 130). Liang et al. revealed widespread disruption in the bilateral SLF specifically in the subgroup of deficit in attention and memory (125). Deng et al. reported increased FA in the left SLF of the frontal lobe in MDD patients compared to healthy controls (107).
Each DBS Target Corresponds to Dysfunctional Brain Regions and Circuits Associated With Different MDD Subtypes
The abnormal WMT in MDD causes dysfunctional connections in multiple brain regions, including medial PFC, dlPFC, ACC, striatum, thalamus, anterior temporal lobe, parietal lobe, occipital lobe, and brainstem. Figure 1 shows a schematic diagram of abnormal WMT and corresponding brain regions in MDD. The mPFC and striatum are two hub dysfunctional brain regions in MDD, which correspond to SCG, VC/VS, BNST, and NAcc target locations. SCG and VC/VS are the most commonly used DBS targets for MDD in previous studies. Thalamus and brainstem are also implicated in MDD, which correspond to MFB, ITP, and LH target locations. Several brain regions, including occipital lobes, parietal lobes, dlPFC, ACC, and anterior temporal lobes are not directly targeted by DBS, but can be modulated by connected WMT.
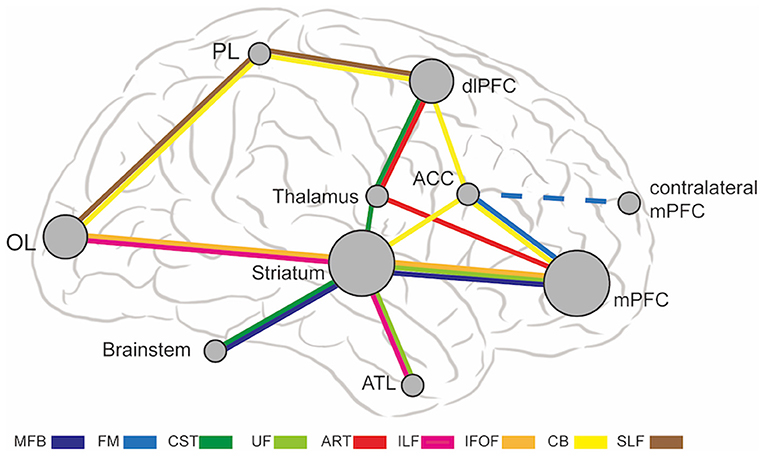
Figure 1. The abnormal WMT and associated brain regions in MDD. The brain region node size represents the number of passing WMT. ACC, anterior cingulate cortex; ATL, anterior temporal lobe; ATR, anterior thalamic radiation; CB, cingulum bundle; CST, corticospinal tract; dlPFC, dorsal lateral prefrontal cortex; FM, forceps minor; IFOF, inferior fronto-occipital fasciculus; ILF, inferior longitudinal fasciculus; MFB, medial forebrain bundle; mPFC, medial prefrontal cortex; OL, occipital lobes; PL, parietal lobes; SLF, superior longitudinal fasciculus; UF, uncinate fasciculus.
The abnormal WMT found in DTI studies is inconsistent, which may due to mixed symptomatology and biotypes, which are likely to overlap in individuals. Different dysfunctional brain circuits underlie different biotypes (11). A previous study has proposed six dysfunctional brain circuits in MDD, namely default mode, salience, negative affect, positive affect, attention, and cognitive control (11). Figure 2 is an illustrative summary of these dysfunctional brain circuits and their associations with WMT modulated by different DBS targets in MDD. FM and CB are implicated in the default mode circuit; UF is implicated in the salience circuit; CB, UF, and FM are implicated in the negative affect circuit; CB, UF, FM, MFB, and IFOF are implicated in the positive affect circuit; CB, SLF, and IFOF are implicated in the attention circuit; CB and SLF are implicated in the cognitive control circuit. We further present a taxonomy of DBS targets based on their associated WMT and modulated dysfunctional brain circuits: SCG, NAcc, and VC/VS modulate default mode, salience, and negative affect circuits simultaneously; SCG, Nacc, VC/VS, BNST, ITP, and LH modulate attention circuit; All of the targets modulate positive affect circuit, and only SCG target modulates cognitive control circuit. In summary, SCG are implicated in all of the dysfunctional brain circuits modulation, Nacc and VC/VS are implicated in modulating most of the dysfunctional brain circuits except cognitive control circuit, and other targets are mainly implicated in modulation of positive affect and attention circuits. We hypothesize that DTI-based personalized targeting strategy will render more favorable clinical outcome for each subtype of MDD. For example, abnormality is reported in CB, IFOF, and SLF in MDD with cognitive deficit, and SCG, based on DTI studies, may be the optimal target of DBS for this subtype (123). Abnormality in CB, UF, SLF, IFL, IFOF is reported in MDD with attention deficit, and multiple DBS targets are implicated in these networks, including SCG, NAcc, VC/VS, BNST, ITP, and LH (125). However, the majority of DTI studies reported mixed results from different subtypes of MDD, and we are not yet at a stage where we can pinpoint a DBS target based on individual clinical manifestations. Further DTI studies underlying different subtypes of MDD is warrant for optimizing DBS targets.
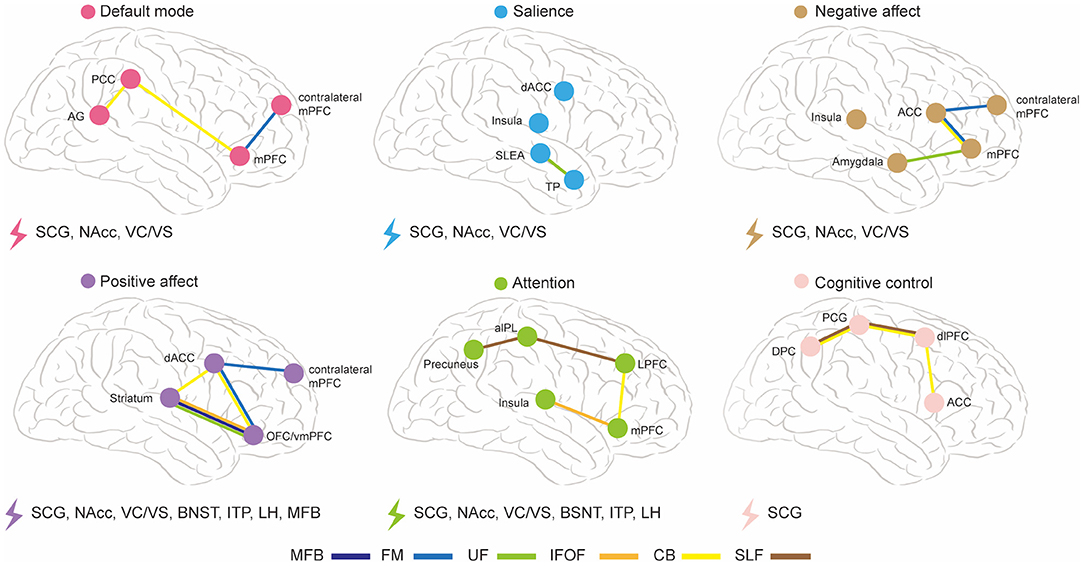
Figure 2. The abnormal WMT in MDD and their implications in different DBS targets and dysfunctional brain circuits. ACC, anterior cingulate cortex; AG, angular gyrus; aIPL, anterior inferior parietal lobule; BNST, bed nucleus of the stria terminalis; CB, cingulum bundle; dACC, dorsal anterior cingulate cortex; dlPFC, dorsal lateral prefrontal cortex; DPC, dorsal parietal cortex; FM, forceps minor; IFOF, inferior fronto-occipital fasciculus; ITP, inferior thalamic peduncle; LH, lateral habenula; LPFC, lateral prefrontal cortex; MFB, medial forebrain bundle; mPFC, medial prefrontal cortex; NAcc, nucleus accumbens; OFC, orbitofrontal cortex; PCC, posterior cingulate cortex; PCG, precentral gyrus; SCG, subcallosal cingulate gyrus; SLF, superior longitudinal fasciculus; TP, temporal pole; UF, uncinate fasciculus; VC/VS, ventral capsule-ventral striatum; vmPFC, ventromedial prefrontal cortex.
Conclusions and Perspectives
In this review, we focus on seven currently used DBS targets for MDD. Most of these clinical trials show that DBS has potential efficacy in the treatment of MDD, though the outcomes are inconsistent. Well-documented and well-designed double-blind RCTs are necessary to provide more powerful evidence of the efficacy and safety of DBS for MDD. Trials comparing various combinations of stimulation parameters and symptoms improvement to identify optimal stimulation parameters are also required (131). In addition, lack of personalized targeting is another important factor that should be taken into account for suboptimal outcomes. A recent study reported the use of intracranial electrophysiology and focal electrical stimulation to identify personalized treatment location (9). In this review, we propose a taxonomy of DBS targets for MDD that may help clinicians to choose the personalized DBS target by non-invasive methods, such as functional magnetic resonance imaging, diffusion tensor imaging, and positron-emission tomography. Among these techniques, DTI is important for understanding the network mechanism and development of MDD, which reveals widespread structural connectivity dysfunctions in MDD. Recently, a study of WMT abnormalities in different subgroups of MDD patients suggested a novel pathway to understanding the heterogeneity of MDD, and may shed light on optimization of subtype-specific treatment approaches (125). Longitudinal study, such as evaluating the time-dependent changes of WMT structures in MDD before and after DBS, may lead to a better understanding of the action mechanisms of DBS. Finally, the identification of individual clinical characteristics and specific WMT abnormalities could serve as a biomarker of therapeutic response in future DBS for MDD studies.
Author Contributions
QY, XG, and ZZhu performed the literature review and drafted the manuscript. ZZhe, CF, and HJ collected the data and revised the manuscript. JZha, JZhu, and HW supervised all aspects of the study and revised the manuscript. All authors reviewed the manuscript and approved the final version.
Funding
This work was supported by the National Natural Science Foundation of China (General Program 82171519), Academy of Science and Technology of Zhejiang University Program (K20210252), and ZJU-XITOU Brain-Machine Intelligence Research Center.
Conflict of Interest
The authors declare that the research was conducted in the absence of any commercial or financial relationships that could be construed as a potential conflict of interest.
Publisher's Note
All claims expressed in this article are solely those of the authors and do not necessarily represent those of their affiliated organizations, or those of the publisher, the editors and the reviewers. Any product that may be evaluated in this article, or claim that may be made by its manufacturer, is not guaranteed or endorsed by the publisher.
Supplementary Material
The Supplementary Material for this article can be found online at: https://www.frontiersin.org/articles/10.3389/fpsyt.2022.806916/full#supplementary-material
References
2. Carreno FR, Frazer A. Vagal nerve stimulation for treatment-resistant depression. Neurotherapeutics. (2017) 14:716–27. doi: 10.1007/s13311-017-0537-8
3. Mayberg HS, Lozano AM, Voon V, McNeely HE, Seminowicz D, Hamani C, et al. Deep brain stimulation for treatment-resistant depression. Neuron. (2005) 45:651–60. doi: 10.1016/j.neuron.2005.02.014
4. Zhou C, Zhang H, Qin Y, Tian T, Xu B, Chen J, et al. A systematic review and meta-analysis of deep brain stimulation in treatment-resistant depression. Prog Neuropsychopharmacol Biol Psychiatry. (2018) 82:224–32. doi: 10.1016/j.pnpbp.2017.11.012
5. Dougherty DD, Rezai AR, Carpenter LL, Howland RH, Bhati MT, O'Reardon JP, et al. A randomized sham-controlled trial of deep brain stimulation of the ventral capsule/ventral striatum for chronic treatment-resistant depression. Biol Psychiatry. (2015) 78:240–8. doi: 10.1016/j.biopsych.2014.11.023
6. Holtzheimer PE, Husain MM, Lisanby SH, Taylor SF, Whitworth LA, McClintock S, et al. Subcallosal cingulate deep brain stimulation for treatment-resistant depression: a multisite, randomised, sham-controlled trial. Lancet Psychiatry. (2017) 4:839–49. doi: 10.1016/S2215-0366(17)30371-1
7. Riva-Posse P, Choi KS, Holtzheimer PE, McIntyre CC, Gross RE, Chaturvedi A, et al. Defining critical white matter pathways mediating successful subcallosal cingulate deep brain stimulation for treatment-resistant depression. Biol Psychiatry. (2014) 76:963–9. doi: 10.1016/j.biopsych.2014.03.029
8. Sugimoto K, Kakeda S, Watanabe K, Katsuki A, Ueda I, Igata N, et al. Relationship between white matter integrity and serum inflammatory cytokine levels in drug-naive patients with major depressive disorder: diffusion tensor imaging study using tract-based spatial statistics. Transl Psychiatry. (2018) 8:141. doi: 10.1038/s41398-018-0174-y
9. Scangos KW, Khambhati AN, Daly PM, Makhoul GS, Sugrue LP, Zamanian H, et al. Closed-loop neuromodulation in an individual with treatment-resistant depression. Nat Med. (2021) 27:1696–700. doi: 10.1038/s41591-021-01480-w
10. Soares JM, Marques P, Alves V, Sousa N. A Hitchhiker's guide to diffusion tensor imaging. Front Neurosci. (2013) 7:31. doi: 10.3389/fnins.2013.00031
11. Williams LM. Precision psychiatry: a neural circuit taxonomy for depression and anxiety. Lancet Psychiatry. (2016) 3:472–80. doi: 10.1016/s2215-0366(15)00579-9
12. Page MJ, McKenzie JE, Bossuyt PM, Boutron I, Hoffmann TC, Mulrow CD, et al. The prisma 2020 statement: an updated guideline for reporting systematic reviews. BMJ. (2021) 372:n71. doi: 10.1136/bmj.n71
13. Hamani C, Mayberg H, Snyder B, Giacobbe P, Kennedy S, Lozano AM. Deep brain stimulation of the subcallosal cingulate gyrus for depression: anatomical location of active contacts in clinical responders and a suggested guideline for targeting. J Neurosurg. (2009) 111:1209–15. doi: 10.3171/2008.10.JNS08763
14. Kennedy SH, Giacobbe P, Rizvi SJ, Placenza FM, Nishikawa Y, Mayberg HS, et al. Deep brain stimulation for treatment-resistant depression: follow-up after 3 to 6 years. Am J Psychiatry. (2011) 168:502–10. doi: 10.1176/appi.ajp.2010.10081187
15. Puigdemont D, Perez-Egea R, Portella MJ, Molet J, de Diego-Adelino J, Gironell A, et al. Deep brain stimulation of the subcallosal cingulate gyrus: further evidence in treatment-resistant major depression. Int J Neuropsychopharmacol. (2012) 15:121–33. doi: 10.1017/S1461145711001088
16. Funayama M, Kato M, Mimura M. Disappearance of treatment-resistant depression after damage to the orbitofrontal cortex and subgenual cingulate area: a case study. BMC Neurol. (2016) 16:198. doi: 10.1186/s12883-016-0717-x
17. Guinjoan SM, Mayberg HS, Costanzo EY, Fahrer RD, Tenca E, Antico J, et al. Asymmetrical contribution of brain structures to treatment-resistant depression as illustrated by effects of right subgenual cingulum stimulation. J Neuropsychiatry Clin Neurosci. (2010) 22:265–77. doi: 10.1176/jnp.2010.22.3.265
18. Puigdemont D, Portella MJ, Perez-Egea R, de Diego-Adelino J, Gironell A, Molet J, et al. Depressive relapse after initial response to subcallosal cingulate gyrus-deep brain stimulation in a patient with a treatment-resistant depression: electroconvulsive therapy as a feasible strategy. Biol Psychiatry. (2009) 66:e11–2. doi: 10.1016/j.biopsych.2009.03.018
19. Torres CV, Ezquiaga E, Navas M, de Sola RG. Deep brain stimulation of the subcallosal cingulate for medication-resistant type i bipolar depression: case report. Bipolar Disord. (2013) 15:719–21. doi: 10.1111/bdi.12102
20. Richieri R, Borius PY, Lagrange G, Faget-Agius C, Guedj E, Mc Gonigal A, et al. Unmasking partial seizure after deep brain stimulation for treatment-resistant depression: a case report. Brain Stimul. (2016) 9:636–8. doi: 10.1016/j.brs.2016.05.001
21. Holtzheimer PE III, Mayberg HS. Deep brain stimulation for treatment-resistant depression. Am J Psychiatry. (2010) 167:1437–44. doi: 10.1176/appi.ajp.2010.10010141
22. Puigdemont D, Portella M, Perez-Egea R, Molet J, Gironell A, de Diego-Adelino J, et al. A randomized double-blind crossover trial of deep brain stimulation of the subcallosal cingulate gyrus in patients with treatment-resistant depression: a pilot study of relapse prevention. J Psychiatry Neurosci. (2015) 40:224–31. doi: 10.1503/jpn.130295
23. Lozano AM, Giacobbe P, Hamani C, Rizvi SJ, Kennedy SH, Kolivakis TT, et al. A multicenter pilot study of subcallosal cingulate area deep brain stimulation for treatment-resistant depression. J Neurosurg. (2012) 116:315–22. doi: 10.3171/2011.10.JNS102122
24. Crowell AL, Riva-Posse P, Holtzheimer PE, Garlow SJ, Kelley ME, Gross RE, et al. Long-term outcomes of subcallosal cingulate deep brain stimulation for treatment-resistant depression. Am J Psychiatry. (2019) 176:949–56. doi: 10.1176/appi.ajp.2019.18121427
25. Merkl A, Schneider GH, Schonecker T, Aust S, Kuhl KP, Kupsch A, et al. Antidepressant effects after short-term and chronic stimulation of the subgenual cingulate gyrus in treatment-resistant depression. Exp Neurol. (2013) 249:160–8. doi: 10.1016/j.expneurol.2013.08.017
26. Merkl A, Aust S, Schneider GH, Visser-Vandewalle V, Horn A, Kuhn AA, et al. Deep brain stimulation of the subcallosal cingulate gyrus in patients with treatment-resistant depression: a double-blinded randomized controlled study and long-term follow-up in eight patients. J Affect Disord. (2018) 227:521–9. doi: 10.1016/j.jad.2017.11.024
27. Kisely S, Li A, Warren N, Siskind D. A systematic review and meta-analysis of deep brain stimulation for depression. Depress Anxiety. (2018) 35:468–80. doi: 10.1002/da.22746
28. Howell B, Choi KS, Gunalan K, Rajendra J, Mayberg HS, McIntyre CC. Quantifying the axonal pathways directly stimulated in therapeutic subcallosal cingulate deep brain stimulation. Hum Brain Mapp. (2019) 40:889–903. doi: 10.1002/hbm.24419
29. Accolla EA, Aust S, Merkl A, Schneider GH, Kuhn AA, Bajbouj M, et al. Deep brain stimulation of the posterior gyrus rectus region for treatment resistant depression. J Affect Disord. (2016) 194:33–7. doi: 10.1016/j.jad.2016.01.022
30. Choi KS, Riva-Posse P, Gross RE, Mayberg HS. Mapping the “depression switch” during intraoperative testing of subcallosal cingulate deep brain stimulation. JAMA Neurol. (2015) 72:1252–60. doi: 10.1001/jamaneurol.2015.2564
31. Riva-Posse P, Choi KS, Holtzheimer PE, Crowell AL, Garlow SJ, Rajendra JK, et al. A connectomic approach for subcallosal cingulate deep brain stimulation surgery: prospective targeting in treatment-resistant depression. Mol Psychiatry. (2018) 23:843–9. doi: 10.1038/mp.2017.59
32. Lujan JL, Chaturvedi A, Choi KS, Holtzheimer PE, Gross RE, Mayberg HS, et al. Tractography-activation models applied to subcallosal cingulate deep brain stimulation. Brain Stimul. (2013) 6:737–9. doi: 10.1016/j.brs.2013.03.008
33. Schlaepfer TE, Bewernick BH, Kayser S, Madler B, Coenen VA. Rapid effects of deep brain stimulation for treatment-resistant major depression. Biol Psychiatry. (2013) 73:1204–12. doi: 10.1016/j.biopsych.2013.01.034
34. Fenoy AJ, Schulz P, Selvaraj S, Burrows C, Spiker D, Cao B, et al. Deep brain stimulation of the medial forebrain bundle: distinctive responses in resistant depression. J Affect Disord. (2016) 203:143–51. doi: 10.1016/j.jad.2016.05.064
35. Fenoy AJ, Schulz PE, Selvaraj S, Burrows CL, Zunta-Soares G, Durkin K, et al. A longitudinal study on deep brain stimulation of the medial forebrain bundle for treatment-resistant depression. Transl Psychiatry. (2018) 8:111. doi: 10.1038/s41398-018-0160-4
36. Bewernick BH, Kayser S, Gippert SM, Switala C, Coenen VA, Schlaepfer TE. Deep brain stimulation to the medial forebrain bundle for depression- long-term outcomes and a novel data analysis strategy. Brain Stimul. (2017) 10:664–71. doi: 10.1016/j.brs.2017.01.581
37. Coenen VA, Bewernick BH, Kayser S, Kilian H, Bostrom J, Greschus S, et al. Superolateral medial forebrain bundle deep brain stimulation in major depression: a gateway trial. Neuropsychopharmacology. (2019) 44:1224–32. doi: 10.1038/s41386-019-0369-9
38. Blomstedt P, Naesstrom M, Bodlund O. Deep brain stimulation in the bed nucleus of the stria terminalis and medial forebrain bundle in a patient with major depressive disorder and anorexia nervosa. Clin Case Rep. (2017) 5:679–84. doi: 10.1002/ccr3.856
39. Coenen VA, Honey CR, Hurwitz T, Rahman AA, McMaster J, Burgel U, et al. Medial forebrain bundle stimulation as a pathophysiological mechanism for hypomania in subthalamic nucleus deep brain stimulation for Parkinson's disease. Neurosurgery. (2009) 64:1106–14; discussion 14–5. doi: 10.1227/01.NEU.0000345631.54446.06
40. Galvez JF, Keser Z, Mwangi B, Ghouse AA, Fenoy AJ, Schulz PE, et al. The medial forebrain bundle as a deep brain stimulation target for treatment resistant depression: a review of published data. Prog Neuropsychopharmacol Biol Psychiatry. (2015) 58:59–70. doi: 10.1016/j.pnpbp.2014.12.003
41. Dandekar MP, Fenoy AJ, Carvalho AF, Soares JC, Quevedo J. Deep brain stimulation for treatment-resistant depression: an integrative review of preclinical and clinical findings and translational implications. Mol Psychiatry. (2018) 23:1094–112. doi: 10.1038/mp.2018.2
42. Coenen VA, Panksepp J, Hurwitz TA, Urbach H, Madler B. Human medial forebrain bundle (Mfb) and anterior thalamic radiation (Atr): imaging of two major subcortical pathways and the dynamic balance of opposite affects in understanding depression. J Neuropsychiatry Clin Neurosci. (2012) 24:223–36. doi: 10.1176/appi.neuropsych.11080180
43. Haber SN, Yendiki A, Jbabdi S. Four deep brain stimulation targets for obsessive-compulsive disorder: are they different? Biol Psychiatry. (2021) 90:667–77. doi: 10.1016/j.biopsych.2020.06.031
44. Coenen VA, Dobrossy MD, Teo SJ, Wessolleck J, Sajonz BEA, Reinacher PC, et al. Diverging prefrontal cortex fiber connection routes to the subthalamic nucleus and the mesencephalic ventral tegmentum investigated with long range (Normative) and short range (ex-vivo high resolution) 7t Dti. Brain Struct Funct. (2022) 227:23–47. doi: 10.1007/s00429-021-02373-x
45. Millet B, Jaafari N, Polosan M, Baup N, Giordana B, Haegelen C, et al. Limbic versus cognitive target for deep brain stimulation in treatment-resistant depression: accumbens more promising than caudate. Eur Neuropsychopharmacol. (2014) 24:1229–39. doi: 10.1016/j.euroneuro.2014.05.006
46. Bewernick BH, Kayser S, Sturm V, Schlaepfer TE. Long-term effects of nucleus accumbens deep brain stimulation in treatment-resistant depression: evidence for sustained efficacy. Neuropsychopharmacology. (2012) 37:1975–85. doi: 10.1038/npp.2012.44
47. Bewernick BH, Hurlemann R, Matusch A, Kayser S, Grubert C, Hadrysiewicz B, et al. Nucleus accumbens deep brain stimulation decreases ratings of depression and anxiety in treatment-resistant depression. Biol Psychiatry. (2010) 67:110–6. doi: 10.1016/j.biopsych.2009.09.013
48. Grubert C, Hurlemann R, Bewernick BH, Kayser S, Hadrysiewicz B, Axmacher N, et al. Neuropsychological safety of nucleus accumbens deep brain stimulation for major depression: effects of 12-month stimulation. World J Biol Psychiatry. (2011) 12:516–27. doi: 10.3109/15622975.2011.583940
49. Park YS, Sammartino F, Young NA, Corrigan J, Krishna V, Rezai AR. Anatomic review of the ventral capsule/ventral striatum and the nucleus accumbens to guide target selection for deep brain stimulation for obsessive-compulsive disorder. World Neurosurg. (2019) 126:1–10. doi: 10.1016/j.wneu.2019.01.254
50. Halpern CH, Wolf JA, Bale TL, Stunkard AJ, Danish SF, Grossman M, et al. Deep brain stimulation in the treatment of obesity. J Neurosurg. (2008) 109:625–34. doi: 10.3171/JNS/2008/109/10/0625
51. Kalivas PW. The glutamate homeostasis hypothesis of addiction. Nat Rev Neurosci. (2009) 10:561–72. doi: 10.1038/nrn2515
52. Baydin S, Yagmurlu K, Tanriover N, Gungor A, Rhoton AL Jr. Microsurgical and fiber tract anatomy of the nucleus accumbens operative. Neurosurgery. (2016) 12:269–88. doi: 10.1227/NEU.0000000000001133
53. Baldermann JC, Melzer C, Zapf A, Kohl S, Timmermann L, Tittgemeyer M, et al. Connectivity profile predictive of effective deep brain stimulation in obsessive-compulsive disorder. Biol Psychiatry. (2019) 85:735–43. doi: 10.1016/j.biopsych.2018.12.019
54. Zhu Z, Hubbard E, Guo X, Barbosa DAN, Popal AM, Cai C, et al. A connectomic analysis of deep brain stimulation for treatment-resistant depression. Brain Stimul. (2021) 14:1226–33. doi: 10.1016/j.brs.2021.08.010
55. Haber SN, Kim KS, Mailly P, Calzavara R. Reward-related cortical inputs define a large striatal region in primates that interface with associative cortical connections, providing a substrate for incentive-based learning. J Neurosci. (2006) 26:8368–76. doi: 10.1523/JNEUROSCI.0271-06.2006
56. Greenberg BD, Gabriels LA, Malone DA Jr, Rezai AR, Friehs GM, Okun MS, et al. Deep brain stimulation of the ventral internal capsule/ventral striatum for obsessive-compulsive disorder: worldwide experience. Mol Psychiatry. (2010) 15:64–79. doi: 10.1038/mp.2008.55
57. Ooms P, Mantione M, Figee M, Schuurman PR, van den Munckhof P, Denys D. Deep brain stimulation for obsessive-compulsive disorders: long-term analysis of quality of life. J Neurol Neurosurg Psychiatry. (2014) 85:153–8. doi: 10.1136/jnnp-2012-302550
58. Malone DA Jr. Use of deep brain stimulation in treatment-resistant depression cleve. Clin J Med. (2010) 77(Suppl. 3):S77–80. doi: 10.3949/ccjm.77.s3.14
59. Malone DA Jr, Dougherty DD, Rezai AR, Carpenter LL, Friehs GM, Eskandar EN, et al. Deep brain stimulation of the ventral capsule/ventral striatum for treatment-resistant depression. Biol Psychiatry. (2009) 65:267–75. doi: 10.1016/j.biopsych.2008.08.029
60. Strong DR, Haber SN, Tyrka AR, Bernier JA, Rassmussen SA, Greenberg BD. Reversible increase in smoking after withdrawal of ventral capsule/ventral striatum deep brain stimulation in a depressed smoker. J Addict Med. (2012) 6:94–5. doi: 10.1097/ADM.0b013e318240acf5
61. Bergfeld IO, Mantione M, Hoogendoorn MLC, Ruhe HG, Horst F, Notten P, et al. Impact of deep brain stimulation of the ventral anterior limb of the internal capsule on cognition in depression. Psychol Med. (2017) 47:1647–58. doi: 10.1017/S0033291717000113
62. Bergfeld IO, Mantione M, Hoogendoorn MLC, Horst F, Notten P, Schuurman PR, et al. Episodic memory following deep brain stimulation of the ventral anterior limb of the internal capsule and electroconvulsive therapy. Brain Stimul. (2017) 10:959–66. doi: 10.1016/j.brs.2017.07.006
63. Bergfeld IO, Mantione M, Hoogendoorn ML, Ruhe HG, Notten P, van Laarhoven J, et al. Deep brain stimulation of the ventral anterior limb of the internal capsule for treatment-resistant depression: a randomized clinical trial. JAMA Psychiatry. (2016) 73:456–64. doi: 10.1001/jamapsychiatry.2016.0152
64. Li N, Baldermann JC, Kibleur A, Treu S, Akram H, Elias GJB, et al. A unified connectomic target for deep brain stimulation in obsessive-compulsive disorder. Nat Commun. (2020) 11:3364. doi: 10.1038/s41467-020-16734-3
65. van der Vlis T, Ackermans L, Mulders AEP, Vrij CA, Schruers K, Temel Y, et al. Ventral capsule/ventral striatum stimulation in obsessive-compulsive disorder: toward a unified connectomic target for deep brain stimulation? Neuromodulation. (2021) 24:316–23. doi: 10.1111/ner.13339
66. Coenen VA, Schlaepfer TE, Maedler B, Panksepp J. Cross-species affective functions of the medial forebrain bundle-implications for the treatment of affective pain and depression in humans. Neurosci Biobehav Rev. (2011) 35:1971–81. doi: 10.1016/j.neubiorev.2010.12.009
67. Cassimjee N, van Coller R, Slabbert P, Fletcher L, Vaidyanathan J. Longitudinal neuropsychological outcomes in treatment-resistant depression following bed nucleus of the stria terminalis-area deep brain stimulation: a case review. Neurocase. (2018) 24:231–7. doi: 10.1080/13554794.2018.1549680
68. Fitzgerald PB, Segrave R, Richardson KE, Knox LA, Herring S, Daskalakis ZJ, et al. A pilot study of bed nucleus of the stria terminalis deep brain stimulation in treatment-resistant depression. Brain Stimul. (2018) 11:921–8. doi: 10.1016/j.brs.2018.04.013
69. Neumann WJ, Huebl J, Brucke C, Gabriels L, Bajbouj M, Merkl A, et al. Different patterns of local field potentials from limbic Dbs targets in patients with major depressive and obsessive compulsive disorder. Mol Psychiatry. (2014) 19:1186–92. doi: 10.1038/mp.2014.2
70. Luyten L, Hendrickx S, Raymaekers S, Gabriels L, Nuttin B. Electrical stimulation in the bed nucleus of the stria terminalis alleviates severe obsessive-compulsive disorder. Mol Psychiatry. (2016) 21:1272–80. doi: 10.1038/mp.2015.124
71. Crestani CC, Alves FH, Gomes FV, Resstel LB, Correa FM, Herman JP. Mechanisms in the bed nucleus of the stria terminalis involved in control of autonomic and neuroendocrine functions: a review. Curr Neuropharmacol. (2013) 11:141–59. doi: 10.2174/1570159X11311020002
72. Avery SN, Clauss JA, Winder DG, Woodward N, Heckers S, Blackford JU. Bnst neurocircuitry in humans. Neuroimage. (2014) 91:311–23. doi: 10.1016/j.neuroimage.2014.01.017
73. Kruger O, Shiozawa T, Kreifelts B, Scheffler K, Ethofer T. Three distinct fiber pathways of the bed nucleus of the stria terminalis to the amygdala and prefrontal cortex. Cortex. (2015) 66:60–8. doi: 10.1016/j.cortex.2015.02.007
74. Jimenez F, Nicolini H, Lozano AM, Piedimonte F, Salin R, Velasco F. Electrical stimulation of the inferior thalamic peduncle in the treatment of major depression and obsessive compulsive disorders. World Neurosurg. (2013) 80:S30.e17–25. doi: 10.1016/j.wneu.2012.07.010
75. Jimenez F, Velasco F, Salin-Pascual R, Hernandez JA, Velasco M, Criales JL, et al. A patient with a resistant major depression disorder treated with deep brain stimulation in the inferior thalamic peduncle. Neurosurgery. (2005) 57:585–93; discussion 93. doi: 10.1227/01.NEU.0000170434.44335.19
76. Jimenez F, Velasco F, Salin-Pascual R, Velasco M, Nicolini H, Velasco AL, et al. Neuromodulation of the inferior thalamic peduncle for major depression and obsessive compulsive disorder. Acta Neurochir Suppl. (2007) 97:393–8. doi: 10.1007/978-3-211-33081-4_44
77. Raymaekers S, Luyten L, Bervoets C, Gabriels L, Nuttin B. Deep brain stimulation for treatment-resistant major depressive disorder: a comparison of two targets and long-term follow-up. Transl Psychiatry. (2017) 7:e1251. doi: 10.1038/tp.2017.66
78. Velasco F, Velasco M, Jimenez F, Velasco AL, Salin-Pascual R. Neurobiological background for performing surgical intervention in the inferior thalamic peduncle for treatment of major depression disorders. Neurosurgery. (2005) 57:439–48; discussion 48. doi: 10.1227/01.NEU.0000172172.51818.51
79. Sartorius A, Kiening KL, Kirsch P, von Gall CC, Haberkorn U, Unterberg AW, et al. Remission of major depression under deep brain stimulation of the lateral habenula in a therapy-refractory patient. Biol Psychiatry. (2010) 67:e9–e11. doi: 10.1016/j.biopsych.2009.08.027
80. Kiening K, Sartorius A. A new translational target for deep brain stimulation to treat depression. EMBO Mol Med. (2013) 5:1151–3. doi: 10.1002/emmm.201302947
81. Wang Z, Cai X, Qiu R, Yao C, Tian Y, Gong C, et al. Case report: lateral habenula deep brain stimulation for treatment-resistant depression. Front Psychiatry. (2020) 11:616501. doi: 10.3389/fpsyt.2020.616501
82. Kochanski RB, Dawe R, Eddelman DB, Kocak M, Sani S. Identification of the stria medullaris thalami using diffusion tensor imaging. Neuroimage Clin. (2016) 12:852–7. doi: 10.1016/j.nicl.2016.10.018
83. Coenen VA, Schlaepfer TE, Bewernick B, Kilian H, Kaller CP, Urbach H, et al. Frontal white matter architecture predicts efficacy of deep brain stimulation in major depression. Transl Psychiatry. (2019) 9:197. doi: 10.1038/s41398-019-0540-4
84. Bach M, Laun FB, Leemans A, Tax CM, Biessels GJ, Stieltjes B, et al. Methodological considerations on tract-based spatial statistics (Tbss). Neuroimage. (2014) 100:358–69. doi: 10.1016/j.neuroimage.2014.06.021
85. Henderson SE, Johnson AR, Vallejo AI, Katz L, Wong E, Gabbay V, et al. Preliminary study of white matter in adolescent depression: relationships with illness severity, anhedonia, and irritability. Front Psychiatry. (2013) 4:152. doi: 10.3389/fpsyt.2013.00152
86. Yang XH, Wang Y, Wang DF, Tian K, Cheung EFC, Xie GR, et al. White matter microstructural abnormalities and their association with anticipatory anhedonia in depression. Psychiatry Res Neuroimag. (2017) 264:29–34. doi: 10.1016/j.pscychresns.2017.04.005
87. Cullen KR, Klimes-Dougan B, Muetzel R, Mueller BA, Camchong J, Houri A, et al. Altered white matter microstructure in adolescents with major depression: a preliminary study. J Am Acad Child Adolesc Psychiatry. (2010) 49:173–83.e1. doi: 10.1016/j.jaac.2009.11.005
88. Seok JH, Choi S, Lim HK, Lee SH, Kim I, Ham BJ. Effect of the comt Val158met polymorphism on white matter connectivity in patients with major depressive disorder. Neurosci Lett. (2013) 545:35–9. doi: 10.1016/j.neulet.2013.04.012
89. LeWinn KZ, Connolly CG, Wu J, Drahos M, Hoeft F, Ho TC, et al. White matter correlates of adolescent depression: structural evidence for frontolimbic disconnectivity. J Am Acad Child Adolesc Psychiatry. (2014) 53:899–909.e1–7. doi: 10.1016/j.jaac.2014.04.021
90. de Diego-Adelino J, Pires P, Gomez-Anson B, Serra-Blasco M, Vives-Gilabert Y, Puigdemont D, et al. Microstructural white-matter abnormalities associated with treatment resistance, severity and duration of illness in major depression. Psychol Med. (2014) 44:1171–82. doi: 10.1017/S003329171300158X
91. Murphy ML, Carballedo A, Fagan AJ, Morris D, Fahey C, Meaney J, et al. Neurotrophic tyrosine kinase polymorphism impacts white matter connections in patients with major depressive disorder. Biol Psychiatry. (2012) 72:663–70. doi: 10.1016/j.biopsych.2012.04.015
92. Zhang A, Leow A, Ajilore O, Lamar M, Yang S, Joseph J, et al. Quantitative tract-specific measures of uncinate and cingulum in major depression using diffusion tensor imaging. Neuropsychopharmacology. (2012) 37:959–67. doi: 10.1038/npp.2011.279
93. Carballedo A, Amico F, Ugwu I, Fagan AJ, Fahey C, Morris D, et al. Reduced fractional anisotropy in the uncinate fasciculus in patients with major depression carrying the met-allele of the Val66met brain-derived neurotrophic factor genotype. Am J Med Genet Part B Neuropsychiat Genet. (2012) 159b:537–48. doi: 10.1002/ajmg.b.32060
94. Bracht T, Horn H, Strik W, Federspiel A, Schnell S, Hofle O, et al. White matter microstructure alterations of the medial forebrain bundle in melancholic depression. J Affect Disord. (2014) 155:186–93. doi: 10.1016/j.jad.2013.10.048
95. Zhu X, Wang X, Xiao J, Zhong M, Liao J, Yao S. Altered white matter integrity in first-episode, treatment-naive young adults with major depressive disorder: a tract-based spatial statistics study. Brain Res. (2011) 1369:223–9. doi: 10.1016/j.brainres.2010.10.104
96. Zou K, Huang X, Li T, Gong Q, Li Z, Ou-yang L, et al. Alterations of white matter integrity in adults with major depressive disorder: a magnetic resonance imaging study. J Psychiatry Neurosci. (2008) 33:525–30.
97. Guo WB, Liu F, Chen JD, Xu XJ, Wu RR, Ma CQ, et al. Altered white matter integrity of forebrain in treatment-resistant depression: a diffusion tensor imaging study with tract-based spatial statistics. Prog Neuropsychopharmacol Biol Psychiatry. (2012) 38:201–6. doi: 10.1016/j.pnpbp.2012.03.012
98. Jia Z, Wang Y, Huang X, Kuang W, Wu Q, Lui S, et al. Impaired frontothalamic circuitry in suicidal patients with depression revealed by diffusion tensor imaging at 30 T. J Psychiatry Neurosci. (2014) 39:170–7. doi: 10.1503/jpn.130023
99. Bessette KL, Nave AM, Caprihan A, Stevens MC. White matter abnormalities in adolescents with major depressive disorder. Brain Imaging Behav. (2014) 8:531–41. doi: 10.1007/s11682-013-9274-8
100. Tha KK, Terae S, Nakagawa S, Inoue T, Kitagawa N, Kako Y, et al. Impaired integrity of the brain parenchyma in non-geriatric patients with major depressive disorder revealed by diffusion tensor imaging. Psychiatry Res. (2013) 212:208–15. doi: 10.1016/j.pscychresns.2012.07.004
101. Peng HJ, Zheng HR, Ning YP, Zhang Y, Shan BC, Zhang L, et al. Abnormalities of cortical-limbic-cerebellar white matter networks may contribute to treatment-resistant depression: a diffusion tensor imaging study. BMC Psychiatry. (2013) 13:72. doi: 10.1186/1471-244X-13-72
102. Tymofiyeva O, Connolly CG, Ho TC, Sacchet MD, Henje Blom E, LeWinn KZ, et al. Dti-based connectome analysis of adolescents with major depressive disorder reveals hypoconnectivity of the right caudate. J Affect Disord. (2017) 207:18–25. doi: 10.1016/j.jad.2016.09.013
103. Korgaonkar MS, Grieve SM, Koslow SH, Gabrieli JD, Gordon E, Williams LM. Loss of white matter integrity in major depressive disorder: evidence using tract-based spatial statistical analysis of diffusion tensor imaging. Hum Brain Mapp. (2011) 32:2161–71. doi: 10.1002/hbm.21178
104. Bracht T, Doidge AN, Keedwell PA, Jones DK. Hedonic tone is associated with left supero-lateral medial forebrain bundle microstructure. Psychol Med. (2015) 45:865–74. doi: 10.1017/S0033291714001949
105. Jeurissen B, Leemans A, Tournier JD, Jones DK, Sijbers J. Investigating the prevalence of complex fiber configurations in white matter tissue with diffusion magnetic resonance imaging. Hum Brain Mapp. (2013) 34:2747–66. doi: 10.1002/hbm.22099
106. Vilgis V, Vance A, Cunnington R, Silk TJ. White matter microstructure in boys with persistent depressive disorder. J Affect Disord. (2017) 221:11–6. doi: 10.1016/j.jad.2017.06.020
107. Deng F, Wang Y, Huang H, Niu M, Zhong S, Zhao L, et al. Abnormal segments of right uncinate fasciculus and left anterior thalamic radiation in major and bipolar depression. Prog Neuropsychopharmacol Biol Psychiatry. (2018) 81:340–9. doi: 10.1016/j.pnpbp.2017.09.006
108. Ouyang X, Tao HJ, Liu HH, Deng QJ, Sun ZH, Xu L, et al. White matter integrity deficit in treatment-naive adult patients with major depressive disorder. East Asian Arch Psychiatry. (2011) 21:5–9.
109. de Kwaasteniet B, Ruhe E, Caan M, Rive M, Olabarriaga S, Groefsema M, et al. Relation between structural and functional connectivity in major depressive disorder. Biol Psychiatry. (2013) 74:40–7. doi: 10.1016/j.biopsych.2012.12.024
110. Aghajani M, Veer IM, van Lang ND, Meens PH, van den Bulk BG, Rombouts SA, et al. Altered white-matter architecture in treatment-naive adolescents with clinical depression. Psychol Med. (2014) 44:2287–98. doi: 10.1017/S0033291713003000
111. Wu F, Tang Y, Xu K, Kong L, Sun W, Wang F, et al. Whiter matter abnormalities in medication-naive subjects with a single short-duration episode of major depressive disorder. Psychiatry Res. (2011) 191:80–3. doi: 10.1016/j.pscychresns.2010.09.002
112. Blood AJ, Iosifescu DV, Makris N, Perlis RH, Kennedy DN, Dougherty DD, et al. Microstructural abnormalities in subcortical reward circuitry of subjects with major depressive disorder. PLoS ONE. (2010) 5:e13945. doi: 10.1371/journal.pone.0013945
113. Repple J, Meinert S, Grotegerd D, Kugel H, Redlich R, Dohm K, et al. A voxel-based diffusion tensor imaging study in unipolar and bipolar depression. Bipolar Disord. (2017) 19:23–31. doi: 10.1111/bdi.12465
114. Wise T, Radua J, Nortje G, Cleare AJ, Young AH, Arnone D. Voxel-based meta-analytical evidence of structural disconnectivity in major depression and bipolar disorder. Biol Psychiatry. (2016) 79:293–302. doi: 10.1016/j.biopsych.2015.03.004
115. Liu X, Watanabe K, Kakeda S, Yoshimura R, Abe O, Ide S, et al. Relationship between white matter integrity and serum cortisol levels in drug-naive patients with major depressive disorder: diffusion tensor imaging study using tract-based spatial statistics. Br J Psychiatry. (2016) 208:585–90. doi: 10.1192/bjp.bp.114.155689
116. Walther S, Hugli S, Hofle O, Federspiel A, Horn H, Bracht T, et al. Frontal white matter integrity is related to psychomotor retardation in major depression. Neurobiol Dis. (2012) 47:13–9. doi: 10.1016/j.nbd.2012.03.019
117. Lai CH, Wu YT. Alterations in white matter micro-integrity of the superior longitudinal fasciculus and anterior thalamic radiation of young adult patients with depression. Psychol Med. (2014) 44:2825–32. doi: 10.1017/S0033291714000440
118. Lu M, Yang C, Chu T, Wu S. Cerebral white matter changes in young healthy individuals with high trait anxiety: a tract-based spatial statistics study. Front Neurol. (2018) 9:704. doi: 10.3389/fneur.2018.00704
119. Liao Y, Huang X, Wu Q, Yang C, Kuang W, Du M, et al. Is depression a disconnection syndrome? Meta-analysis of diffusion tensor imaging studies in patients with Mdd. J Psychiatry Neurosci. (2013) 38:49–56. doi: 10.1503/jpn.110180
120. Jiang W, Gong G, Wu F, Kong L, Chen K, Cui W, et al. The papez circuit in first-episode, treatment-naive adults with major depressive disorder: combined atlas-based tract-specific quantification analysis and voxel-based analysis. PLoS ONE. (2015) 10:e0126673. doi: 10.1371/journal.pone.0126673
121. Liu Y, Duan Y, He Y, Yu C, Wang J, Huang J, et al. Whole brain white matter changes revealed by multiple diffusion metrics in multiple sclerosis: a Tbss study. Eur J Radiol. (2012) 81:2826–32. doi: 10.1016/j.ejrad.2011.11.022
122. Huang H, Fan X, Williamson DE, Rao U. White matter changes in healthy adolescents at familial risk for unipolar depression: a diffusion tensor imaging study. Neuropsychopharmacology. (2011) 36:684–91. doi: 10.1038/npp.2010.199
123. Wang Z, Yuan Y, You J, Zhang Z. Disrupted structural brain connectome underlying the cognitive deficits in remitted late-onset depression. Brain Imaging Behav. (2020) 14:1600–11. doi: 10.1007/s11682-019-00091-x
124. Fox CJ, Iaria G, Barton JJ. Disconnection in prosopagnosia and face processing. Cortex. (2008) 44:996–1009. doi: 10.1016/j.cortex.2008.04.003
125. Liang S, Wang Q, Kong X, Deng W, Yang X, Li X, et al. White matter abnormalities in major depression biotypes identified by diffusion tensor imaging. Neurosci Bull. (2019) 35:867–76. doi: 10.1007/s12264-019-00381-w
126. Zheng K, Wang H, Li J, Yan B, Liu J, Xi Y, et al. Structural networks analysis for depression combined with graph theory and the properties of fiber tracts via diffusion tensor imaging. Neurosci Lett. (2019) 694:34–40. doi: 10.1016/j.neulet.2018.11.025
127. Srivastava S, Bhatia MS, Bhargava SK, Kumari R, Chandra S. A diffusion tensor imaging study using a voxel-based analysis, region-of-interest method to analyze white matter abnormalities in first-episode, treatment-naive major depressive disorder. J Neuropsychiatry Clin Neurosci. (2016) 28:131–7. doi: 10.1176/appi.neuropsych.15050120
128. Chen VC, Shen CY, Liang SH, Li ZH, Tyan YS, Liao YT, et al. Assessment of abnormal brain structures and networks in major depressive disorder using morphometric and connectome analyses. J Affect Disord. (2016) 205:103–11. doi: 10.1016/j.jad.2016.06.066
129. Cole J, Chaddock CA, Farmer AE, Aitchison KJ, Simmons A, McGuffin P, et al. White matter abnormalities and illness severity in major depressive disorder. Br J Psychiatry. (2012) 201:33–9. doi: 10.1192/bjp.bp.111.100594
130. Dalby RB, Frandsen J, Chakravarty MM, Ahdidan J, Sorensen L, Rosenberg R, et al. Depression severity is correlated to the integrity of white matter fiber tracts in late-onset major depression. Psychiatry Res. (2010) 184:38–48. doi: 10.1016/j.pscychresns.2010.06.008
Keywords: deep brain stimulation, diffusion tensor imaging, major depressive disorder, white matter tracks, review
Citation: Yu Q, Guo X, Zhu Z, Feng C, Jiang H, Zheng Z, Zhang J, Zhu J and Wu H (2022) White Matter Tracts Associated With Deep Brain Stimulation Targets in Major Depressive Disorder: A Systematic Review. Front. Psychiatry 13:806916. doi: 10.3389/fpsyt.2022.806916
Received: 01 November 2021; Accepted: 21 March 2022;
Published: 28 April 2022.
Edited by:
William Omar Contreras Lopez, Clínica FOSCAL, ColombiaReviewed by:
Romain Duprat, Ghent University, BelgiumKathrin Koch, Technical University of Munich, Germany
Copyright © 2022 Yu, Guo, Zhu, Feng, Jiang, Zheng, Zhang, Zhu and Wu. This is an open-access article distributed under the terms of the Creative Commons Attribution License (CC BY). The use, distribution or reproduction in other forums is permitted, provided the original author(s) and the copyright owner(s) are credited and that the original publication in this journal is cited, in accordance with accepted academic practice. No use, distribution or reproduction is permitted which does not comply with these terms.
*Correspondence: Hemmings Wu, aGVtbWluZ3NAemp1LmVkdS5jbg==; Junming Zhu, ZHIuemh1anVubWluZ0B6anUuZWR1LmNu
†These authors have contributed equally to this work