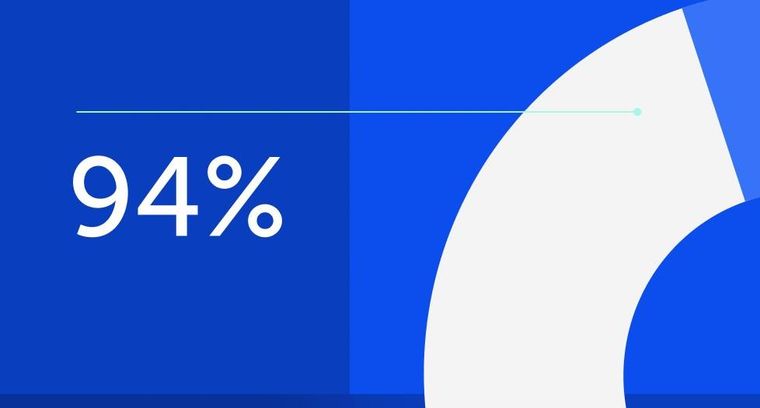
94% of researchers rate our articles as excellent or good
Learn more about the work of our research integrity team to safeguard the quality of each article we publish.
Find out more
SYSTEMATIC REVIEW article
Front. Psychiatry, 14 February 2022
Sec. Schizophrenia
Volume 13 - 2022 | https://doi.org/10.3389/fpsyt.2022.793938
This article is part of the Research TopicSubstance Use and the Psychosis SpectrumView all 11 articles
Background: Substance use disorders (SUDs) are a common yet poorly studied comorbidity in individuals with psychotic disorders. The co-occurrence of the two complicates recovery and interferes with pharmacological and behavioral treatment response and adherence. Recently, researchers have been exploring both invasive and non-invasive neuromodulation techniques as potential treatment methods for SUDs. We review the evidence that neuromodulation may reduce substance craving and consumption in individuals with schizophrenia.
Methods: A comprehensive literature search of PubMed, MEDLINE, and PsycINFO databases was conducted (N = 1,432). Of these, we identified seven studies examining the effects of repetitive transcranial magnetic stimulation (rTMS) and two studies using transcranial direct current stimulation (tDCS) on drug consumption and craving in schizophrenia or schizoaffective disorders.
Results: Despite the limited number of studies in this area, the evidence suggests that rTMS to the dorsolateral prefrontal cortex (DLPFC) may reduce cannabis and tobacco use in patients with schizophrenia and schizoaffective disorder. Findings with tDCS, however, were inconclusive.
Discussion: Our systematic review suggests that rTMS applied to DLPFC is a safe and promising therapeutic technique for the management of comorbid schizophrenia and SUDs, with the majority of the evidence in tobacco use disorder. However, there was substantial heterogeneity in study methods, underscoring the need to optimize stimulation parameters (e.g., frequency, duration, and target regions). Larger clinical trials are needed to establish the efficacy of rTMS in reducing drug consumption and craving in psychotic patients, ideally in comparison to existing pharmacological and behavioral interventions.
Schizophrenia (SCZ) is a serious mental illness affecting nearly 20 million people worldwide (1). SCZ is characterized by positive (i.e., paranoia and hallucinations), negative (i.e., amotivation and anhedonia), disorganized (i.e., thought disorder and disorganized behaviors), and cognitive (i.e., deficits in attention and sensory processing) symptoms (2). The course and prognosis of SCZ is often complicated by co-occurring substance use disorders (SUD), evidenced by a global prevalence of ~42% for any SUD, including illicit drugs (27.5%), cannabis (26.2%), and alcohol [24.3%; (3)]. Such high levels of comorbidity are potentially due to, inter alia, shared genetic and environmental factors increasing SUD vulnerability [for review see (4, 5)] or to alleviate cognitive and psychotic symptoms (6). Use of psychoactive substances can interfere with antipsychotic medication (7), are associated with reduced adherence to SCZ interventions (8), and can lead to symptom exacerbation (9). There are mixed findings with respect to antipsychotics for treating SUDs in SCZ and preliminary support for the use of naltrexone in reducing alcohol use in SCZ (10). Behavioral interventions have also shown some success in reducing substance use, mostly during the intervention period (10). However, research remains relatively limited as individuals with co-occurring serious mental illnesses are often excluded from SUD clinical trials. Moreover, these methods are difficult to implement in SCZ patients; psychotic symptoms and cognitive deficits may reduce patients' ability to engage meaningfully in SUD behavioral interventions (11), while certain pharmacological addiction interventions may worsen positive symptoms of psychosis (12–14), urging investigation into novel and effective interventions for SCZ patients.
Invasive and non-invasive neurostimulation techniques are emerging innovative treatments that have been investigated in the context of treatment-resistant illnesses (15) in individuals who struggle with adherence. As such, they are a promising modality for treating SUDs in SCZ as they can directly target putative brain regions, such as the prefrontal cortex and nucleus accumbens, that are associated with SCZ and SUD pathophysiology (4) with less effort than is required for medication compliance. Moreover, they are safe, time-effective, and patient-friendly, offering a neuroscience-based treatment that may be superior to conventional medications and behavioral therapies. Such techniques include non-invasive repetitive transcranial magnetic stimulation (rTMS), transcranial direct current stimulation (tDCS), or invasive deep brain stimulation (DBS). The purpose of this review is to systematically review the evidence that neurostimulation techniques reduce substance craving and consumption in individuals with SCZ.
rTMS is a non-invasive stimulation method that involves positioning an electromagnetic Figure-of-8 or H-coil on the scalp to produce a time-varying magnetic field (16, 17). This current can be localized to specific brain regions to modulate neurotransmission (18). Options include low- or high-frequency rTMS, which tend to produce inhibitory or excitatory effects, respectively (17). A variation of rTMS is intermittent or continuous theta burst stimulation, which generally produces similar results, usually with shorter session duration (19, 20). rTMS is well tolerated, with some people reporting headache, tingling, or lightheadedness (20–22) and rarely cognitive deficits or seizures [0.071%; (23, 24)], with H-coil carrying a slightly higher risk than Figure-of-8.
tDCS is another non-invasive stimulation method that involves a low-intensity, steady-state direct current that is delivered to a localized brain region via two or more electrodes on the scalp (18, 25). There are variations between protocols regarding the size and number of electrodes, duration of stimulation, and current strength that modify the dispersion of the current to the brain. tDCS electrodes can either increase (if anodal) or decrease (if cathodal) the likelihood of neuronal firing by modulating the resting membrane potential (25). Furthermore, prolonged stimulation may modify synaptic plasticity via long-term potentiation or depression (17, 25). tDCS is relatively low risk, with some patients reporting sleepiness, minor discomfort, or mild burning or pain in the neck or scalp (26).
DBS is an invasive technique, where microelectrodes are embedded in the brain, thus allowing for sustained modulation of neuronal firing to regulate neurotransmission in specific brain regions (18). Embedded electrodes are coupled with a pulse generator to facilitate continuous stimulation (18). Although DBS offers a more localized and deeper signal that can modulate oscillatory activity, it involves a surgical procedure and thus carries risks including infection or hemorrhage (27).
The evidence to date suggests that stimulation of regions in the mesocorticolimbic system may modulate dysregulated neurotransmitter release, thus reducing craving and consumption of addictive substances (16, 28). The most consistent positive results occur when multiple sessions of high-frequency (>10 Hz) stimulation is applied to the dorsolateral prefrontal cortex (DLPFC), as this enhances its inhibitory actions on the mesolimbic DA circuits (16, 18, 28). Indeed, preliminary studies using small sample sizes have found that after 10- 20 sessions, both Figure-of-8 and H-coil rTMS are effective at reducing alcohol cravings (29–32) and consumption (33). Furthermore, figure-of-8 coil rTMS has been effective in reducing cigarette craving and consumption (34–37), cocaine craving (38, 39) and producing greater abstinence rates (40, 41) when applied to the DLPFC. tDCS has also shown promising results in reducing craving and consumption of alcohol, opioids, cannabis, cocaine, and methamphetamines [for review see (18)], and tobacco (42). Additionally, several case series investigating DBS targeting the nucleus accumbens suggest it may also be effective in reducing alcohol craving and intake as well as cocaine use [for review see (17)].
However, there are some studies that are inconsistent with the above findings (43–45). Such discrepancies are likely to due to inconsistencies in stimulation parameters, such as number of pulses, duration of stimulation, stimulation frequency, and number of sessions. These parameters influence whether stimulation is excitatory, the magnitude of the electric field delivered, neuronal activation, and tolerability (23). As such, investigation into the effectiveness of neurostimulation in treating SUDs is paramount, as is standardization of stimulation parameters and extension of this research to individuals with comorbid SCZ and SUDs.
Given the high prevalence of SUDs (3) coupled with the lack of effective treatments for addiction in SCZ patients, novel, low-effort, and quick to administer treatments are needed. The neuropathological correlates of SCZ, including dysregulated dopaminergic, serotonergic, and cholinergic systems result in characteristic psychopathological symptoms, along with deficits in reward processing and cognitive function (46). Dysregulated responses to rewarding stimuli are thought to underlie the increased reinforcement of substances in SCZ relative to non-psychiatric controls [for review see (5)]. Moreover, individuals with SCZ may use substances as a way to cope with negative symptoms (e.g., restricted affect) and/or attenuate cognitive deficits (9, 47). In light of the promising effects of neurostimulation on regulation of neurotransmitters (28), improvements on negative symptoms in SCZ (48) that may contribute to use, improvements on depressive symptoms (49), cognitive functioning, and reductions in cravings and consumption (17, 18) in non-psychiatric SUDs, individuals with SCZ stand to benefit from investigation into the utility of neurostimulation.
Accordingly, we review the available evidence on neurostimulation techniques as a treatment modality for SUDs in SCZ. In an effort to be comprehensive, all psychotic-spectrum disorders were included in the search, however, only studies assessing SCZ and schizoaffective disorder (SCA) were found.
A comprehensive literature search was conducted by two of the authors (SJ and MS) using PsycINFO, PubMed, and MEDLINE following PRISMA guidelines. Search terms included: neuromodulation, neurostimulation, stimulation, (repetitive) transcranial magnetic stimulation, transcranial direct current stimulation, theta burst stimulation, deep brain stimulation, vagus nerve stimulation, and psychosis, schizophrenia, schizoaffective disorder, schizotypal, delusional, schizophreniform, psychotic, bipolar psychosis, depressive psychosis, and substance use disorder, substances, addiction, drugs, cocaine, crack, cannabis, tobacco, nicotine, alcohol, methamphetamines, amphetamines, opioids. We included only randomized sham-controlled trials (RCT), open-label studies, or case studies whose population had a psychosis-spectrum disorder and whose primary or secondary outcomes were an end point measure of substance consumption or craving. Exclusion criteria included substance-induced psychosis, non-validated measures of substance use, and reviews or meta-analyses.
Risk of bias was evaluated using the Cochrane Collaboration Tool (50), which assesses studies on the following criteria: random sequence generation, allocation concealment, blinding of participants and research personnel, blinding of outcome measures, incomplete outcome data, and selective reporting of results.
As depicted in Figure 1, after identifying 1,438 unique studies, 47 studies were assessed for full eligibility, leaving eight published papers and one unpublished manuscript (rTMS = 7, n = 204; tDCS = 2, n = 49). Seven of the included studies were RCTs whereas the other two employed an open-label design. All but one of the studies examined the effects of neurostimulation on cigarette craving or consumption in individuals with SCZ or SCA. The remaining study investigated cannabis craving and consumption along with cigarette consumption in SCZ. The main characteristics of the included studies are described in Table 1 below.
Figure 1. PRISM-A diagram depicting study inclusion process (51).
Table 1 shows the results of the risk of bias assessments. Overall, the seven RCTs were of high methodological quality with all but one scoring a 6, while the two open-label studies indicated a high risk of bias.
As seen in Table 2, two studies (55, 60) investigated the effects of rTMS on cigarette cravings, measured by the Tiffany Questionnaire for Smoking Urges (TQSU). While both studies administered rTMS at 20 Hz to the DLPFC bilaterally, the short-term (6 sessions; 3 days) study found no reduction in cigarette cravings or withdrawal (55) at the end of stimulation, whereas the longer intervention (20 sessions; 28 days) found a significant reduction in desire and intention to smoke cigarettes in the active group relative to the sham group (60). However, only the acute trial involved contingent abstinence, potentially resulting in increased cravings for participants, making direct comparison difficult. One study investigated the effect of rTMS on the bilateral DLPFC (20 Hz, 20 sessions) on cannabis cravings and withdrawal (56). While not statistically significant, the active group reported greater (50%) reductions in cravings than the sham group, particularly in terms of expectations of positive outcomes (e.g., feeling more social) after using cannabis.
Five of the identified studies investigated the effects of rTMS on cigarette or cannabis consumption. Four of these studies applied 10 Hz (15-20 sessions) to the left DLPFC and assessed changes in cigarette consumption from baseline to the 21st day of stimulation (57) or after a 21 day follow-up (53, 54, 58). In three of the four studies, rTMS significantly reduced the number of cigarettes smoked relative to the control group; one study did not find a significant reduction (54). However, Kamp et al. found that individuals in the active group who smoked a higher number of cigarettes reported a greater reduction in consumption (54). One study investigating the effects of bilateral DLPFC (20 Hz, 20 sessions) found trending reductions in self-reported and biologically verified cannabis use in the active group that were greater than the sham group, as well as a statistically significant and strong reductions in cigarette use (56).
Table 3 depicts the results of the tDCS studies. One study investigated the effect of tDCS on cigarette cravings. Smith et al. (59) applied 2 mA through a cathode to the contralateral supraorbital ridge and through an anode to the left DLPFC (20 min; five sessions) and found no reduction in urge to smoke or dependence, as measured by the Questionnaire on Smoking Urges.
Two studies investigated the effects of tDCS on cigarette consumption. Brunelin et al. (52) applied 2 mA through a cathode to the left temporo-parietal junction and through an anode to the left prefrontal region for 20 min (10 sessions) and found no effect on cigarette consumption. Moreover, cigarette consumption was associated with a reduction in the clinical efficacy of tDCS on auditory hallucinations. However, there was no sham group in this study. Similarly, when applying 2 mA for 20 min (five sessions), through a cathode to the contralateral supraorbital ridge and through an anode to the left DLPFC, Smith et al. (59) found no reductions in self-reported or biologically verified cigarette abstinence.
In three out of the four studies that examined cognitive outcomes, tDCS and rTMS were both effective in improving performance on some measures, including the discrimination index on the Hopkins Verbal Learning Test [HVLT; assesses immediate and delayed recall; (55)], hit reaction time and variability on the Continuous Performance Test [CPT; (56)], and the composite score of the MATRICS Consensus Cognitive Battery (assesses a range of cognitive functioning in SCZ) as well as working memory and attention subscales (59). However, there were a few cognitive tasks on which rTMS had no effect depicted in Table 2. With respect to clinical outcomes, two studies found reductions in total scores of the Positive and Negative Symptom Scale (56, 57) and one found improvements on the Montgomery-Asberg Depression Rating Scale (MADRS) as a result of rTMS. Moreover, one study found improvements on auditory hallucinations after tDCS (52). However, three studies found no effects of rTMS (n = 2) on the MADRS or PANSS (53, 58) or tDCS (n = 1) on PANSS scores or hallucinations (59).
rTMS and tDCS procedures were well-tolerated in the included studies. Some participants reported mild to moderate application site pain, neck pain, headache, or dizziness. All resolved naturally (52, 53, 56, 59, 60). No participants dropped out of the study due to pain from the study device. There were no reports of treatment emergent memory or other cognitive deficits, or seizures.
Our review of the extant literature suggests that rTMS applied to the left or bilateral DLPFC may be effective in reducing craving for and consumption of tobacco and cannabis in individuals with SCZ or SCA. However, evidence did not support the efficacy of tDCS in reducing cigarette craving or consumption, possibly due to the limited number of stimulation sessions employed (5–10) relative to the rTMS studies where 15 or more sessions were performed. While the results of studies in this review provide support for continuing investigation of rTMS as an addiction treatment, there remains a need for more robust clinical trials as well as standardization of stimulation parameters.
Based on calculated effect sizes (Tables 2, 3) the evidence suggests that 10 Hz of rTMS directed at the left DLPFC for at least 20 sessions is effective in reducing cigarettes smoked per day. Moreover, 20 Hz for at least 20 sessions directed at the bilateral DLPFC is effective in reducing cravings for cigarettes, cigarettes smoked per day, and- albeit on the basis of a single study might be effective in reducing cannabis use. Interestingly, high-frequency rTMS (10 Hz or more) applied to the DLPFC for a greater number of sessions is also supported by data from neurostimulation studies in non-psychiatric SUD samples (18, 28). While difficult to compare across diagnoses, the lack of efficacy of tDCS on tobacco craving and consumption does not align with literature in non-psychiatric individuals with SUDs, which did show significant effects after 1-5 sessions (18) with a similar intensity (2 mA) and duration (20 min). It is possible that neurobiological underpinnings of SCZ are not concordant with tDCS stimulation targets or that more sessions are needed to see similar effects. Further investigation is needed for conclusive guidance.
Although the effectiveness of rTMS in reducing tobacco cravings in people with SCZ was variable across reviewed studies, the null findings in Kozak et al. (55) might be explained by the short number of treatment sessions or by the effects of contingent abstinence. While measurement of cravings is clinically useful and may point to mechanisms through which neurostimulation works (e.g., regulation of reward pathways), they represent subjective ratings of an introspective phenomenon (61) and therefore are subject to bias.
Unverified self-reported changes in consumption were present in five of the reviewed studies. Although this is more informative regarding effectiveness, biologically-verified measures of consumption represent a more objective measure of changes in substance use and should be employed in future investigations. Moreover, immediately before and after stimulation, fMRI and EEG measures of addiction-related circuitries would be helpful in assessing changes produced through stimulation (62).
Cognitive outcomes were reported in four of the reviewed studies. Improvements were found in three, which may be explained through direct effects of stimulation on targeted brain regions (e.g., DLPFC) mediating cognitive performance or indirectly through reduced substance use. Of note, previous studies have found support for nicotine-induced improvements in SCZ cognitive impairments, specifically in attention, visuospatial working memory, and verbal learning and memory; it has been proposed that these factors may contribute to increased tobacco addiction vulnerability in people with SCZ (47, 63–66). It is also possible that alleviation of clinical symptoms as a direct result of the neuromodulation or an indirect result of reduced substance use may have contributed to improvements in cognitive functioning due to reduced cognitive load or enhancement of cognitive resources. While conclusions are limited due to the preliminary nature of the evidence, future research should investigate whether neuromodulation interventions in prodromal SCZ aimed at improving cognitive deficits are effective in reducing the likelihood of future tobacco or cannabis use disorder.
Evidence of alleviation of depression or positive and negative SCZ symptoms was mixed. However, given that neuromodulation has also been used to ameliorate positive and negative symptoms (67, 68) and meta-analyses have shown rTMS to be effective in the treatment of both major depression and schizophrenia (69). Thus, future studies should continue to investigate the possibility of neuromodulation as an integrated treatment, as well as potential pathways to efficacy via reductions in negative symptoms, while controlling for symptom changes that are associated with reductions in substance use.
While this review shows preliminary support for the use of neuromodulation in individuals with SCZ and SCA, there remains a gap in evidence supporting its use in other psychotic disorders (e.g., bipolar disorder with psychotic features, first-episode psychosis). To that end, there remains a question of who the appropriate candidate for brain stimulation is; would individuals with acute substance-related exogenous psychosis (70) or first-episode psychosis (71) benefit from neuromodulation or should it be reserved for individuals experiencing more chronic and resistant psychosis? Additionally, case-studies (72–74) of rTMS in individuals with mood disorders have reported the occurrence of neuromdulation-induced mania as an adverse event, which is particularly relevant to treating SUDs in individuals with bipolar or depressive disorders with psychotic features. It is emphasized that caution should be exercised and that further empirical research should be conducted to establish definitive guidelines for clinicians.
There are a number of limitations to the current review. Primarily, is that despite the high prevalence of cannabis, stimulant, alcohol, and polysubstance use in SCZ and other psychotic-spectrum disorders (75–77), gaps remain in elucidating the effectiveness of neurostimulation for these substances, with only one study investigating cannabis in this population to date (56). Future studies of neuromodulation in SCZ should examine these substances for a more comprehensive understanding of its utility in treating SUDs. Furthermore, despite similar patient samples and outcome measures across studies, the stimulation parameters and targeted brain regions were highly heterogenous. In addition, self-reported substance use is subject to recall bias (78). Future studies should aim to biologically verify reductions in self-reported substance use. Moreover, antipsychotic medications that antagonize D2 dopamine receptors are known to reduce the effectiveness of tDCS on psychopathological symptoms (79), and this was not factored into the included studies. Participants in Smith et al. (59) and Brunelin et al. (52) were on clozapine during stimulation treatment, potentially impacting results. There were no studies of DBS in psychosis-spectrum populations, however, the feasibility of recruiting such patients for invasive brain stimulation procedures may prove challenging. Finally, the majority of the studies were conducted with predominantly male samples. While this may in part be due to sex differences in the diagnosis of SCZ (80) it limits generalizability of these results to females with SCZ.
rTMS is a promising and well-tolerated option in the treatment of tobacco, and possibly, cannabis use disorders in SCZ and SCA. However, there is a need to optimize stimulation parameters (e.g., frequency, duration, and stimulation target regions), as has been noted in previous reviews (18). In addition, while this review suggests 5-10 sessions of tDCS may not be effective for reducing tobacco use in SCZ, future research should investigate whether more sessions may have efficacy. Larger sham-controlled clinical trials with longer follow-ups and more accurate substance use measures are needed to establish the efficacy of neuromodulation in reducing drug consumption, ideally in comparison to existing pharmacological and behavioral interventions. Moreover, future research should investigate the effects of rTMS on consumption of alcohol and other drugs (e.g., cannabis, cocaine, methamphetamine, opioids) in SCZ and other psychosis-spectrum illness.
The original contributions presented in the study are included in the article/supplementary material, further inquiries can be directed to the corresponding author/s.
SJ wrote the manuscript. SJ, MS, and NA-S conducted the systematic review. VS, GP, MS, DL, DC, and TG edited and revised the manuscript. TG, DC, SJ, and MS conceptualized the review. All authors contributed to the article and approved the submitted version.
This work was funded in part by NIH grant R21-DA-043949 to TG.
The authors declare that the research was conducted in the absence of any commercial or financial relationships that could be construed as a potential conflict of interest.
All claims expressed in this article are solely those of the authors and do not necessarily represent those of their affiliated organizations, or those of the publisher, the editors and the reviewers. Any product that may be evaluated in this article, or claim that may be made by its manufacturer, is not guaranteed or endorsed by the publisher.
1. James SL, Abate D, Abate KH, Abay SM, Abbafati C, Abbasi N, et al. Global, regional, and national incidence, prevalence, and years lived with disability for 354 diseases and injuries for 195 countries and territories, 1990–2017: a systematic analysis for the Global Burden of Disease Study 2017. Lancet. (2018) 392:1789–858. doi: 10.1016/S0140-6736(18)32279-7
2. Liddle PF. The Multidimensional Phenotype of Schizophrenia. Schizophrenia in a Molecular Age. Review of Psychiatry Series. Arlington, VA: American Psychiatric Association (1999). p. 1–28.
3. Hunt GE, Large MM, Cleary M, Lai HMX, Saunders JB. Prevalence of comorbid substance use in schizophrenia spectrum disorders in community and clinical settings, 1990–2017: systematic review and meta-analysis. Drug Alcohol Depend. (2018) 191:234–58. doi: 10.1016/j.drugalcdep.2018.07.011
4. Chambers RA, Krystal JH, Self DW. A neurobiological basis for substance abuse comorbidity in schizophrenia. Biol Psychiatry. (2001) 50:71–83. doi: 10.1016/S0006-3223(01)01134-9
5. Lucatch AM, Lowe DJE, Clark RC, Kozak K, George TP. Neurobiological determinants of tobacco smoking in schizophrenia. Front Psychiatry. (2018) 9:672. doi: 10.3389/fpsyt.2018.00672
6. Spencer C, Castle D, Michie PT. Motivations that maintain substance use among individuals with psychotic disorders. Schizophr Bull. (2002) 28:233–47. doi: 10.1093/oxfordjournals.schbul.a006934
7. Andersen FD, Simonsen U, Andersen CU. Quetiapine and other antipsychotics combined with opioids in legal autopsy cases: a random finding or cause of fatal outcome? Basic Clin Pharmacol Toxicol. (2021) 128:66–79. doi: 10.1111/bcpt.13480
8. Hunt GE, Bergen J, Bashir M. Medication compliance and comorbid substance abuse in schizophrenia: impact on community survival 4 years after a relapse. Schizophr Res. (2002) 54:253–64. doi: 10.1016/S0920-9964(01)00261-4
9. Talamo A, Centorrino F, Tondo L, Dimitri A, Hennen J, Baldessarini RJ. Comorbid substance-use in schizophrenia: Relation to positive and negative symptoms. Schizophr Res. (2006) 86:251–5. doi: 10.1016/j.schres.2006.04.004
10. Bennett ME, Bennett ME, Bradshaw KR, Catalano LT. Treatment of substance use disorders in schizophrenia. Am J Drug Alcohol Abuse. (2017) 43:377–90. doi: 10.1080/00952990.2016.1200592
11. Hunt GE, Siegfried N, Morley K, Brooke-Sumner C, Cleary M. Psychosocial interventions for people with both severe mental illness and substance misuse. Cochr Database Syst Rev. (2019) 12:Cd001088. doi: 10.1002/14651858.CD001088.pub4
12. George TP. Neurobiological links between nicotine addiction and schizophrenia. J Dual Diagn. (2007) 3:27–42. doi: 10.1300/J374v03n03_04
13. Green AI, Noordsy DL, Brunette MF, O'Keefe C. Substance abuse and schizophrenia: pharmacotherapeutic intervention. J Substance Abuse Treatment. (2008) 34:61–71. doi: 10.1016/j.jsat.2007.01.008
14. Kozak K, Barr MS, George TP. Traits and biomarkers for addiction risk in schizophrenia. Curr Addict Rep. (2017) 4:14–24. doi: 10.1007/s40429-017-0130-6
15. Bewernick B, Schlaepfer TE. Update on neuromodulation for treatment-resistant depression. F1000Res. (2015) 4:F1000 Faculty Rev-389. doi: 10.12688/f1000research.6633.1
16. Lefaucheur J-P, André-Obadia N, Antal A, Ayache SS, Baeken C, Benninger DH, et al. Evidence-based guidelines on the therapeutic use of repetitive transcranial magnetic stimulation (rTMS) Clin Neurophysiol. (2014) 125:2150–206. doi: 10.1016/j.clinph.2014.05.021
17. Luigjes J, Segrave R, de Joode N, Figee M, Denys D. Efficacy of invasive and non-invasive brain modulation interventions for addiction. Neuropsychol Rev. (2019) 29:116–38. doi: 10.1007/s11065-018-9393-5
18. Coles AS, Kozak K, George TP. A review of brain stimulation methods to treat substance use disorders. Am J Addict. (2018) 27:71–91. doi: 10.1111/ajad.12674
19. Huang Y-Z, Edwards MJ, Rounis E, Bhatia KP, Rothwell JC. Theta burst stimulation of the human motor cortex. Neuron. (2005) 45:201–6. doi: 10.1016/j.neuron.2004.12.033
20. Nursey J, Sbisa A, Knight H, Ralph N, Cowlishaw S, Forbes D, et al. Exploring theta burst stimulation for post-traumatic stress disorder in Australian veterans—a pilot study. Milit Med. (2020) 185:e1770–8. doi: 10.1093/milmed/usaa149
21. Su H, Zhong N, Gan H, Wang J, Han H, Chen T, et al. High frequency repetitive transcranial magnetic stimulation of the left dorsolateral prefrontal cortex for methamphetamine use disorders: a randomised clinical trial. Drug Alcohol Depend. (2017) 175:84–91. doi: 10.1016/j.drugalcdep.2017.01.037
22. Lerner AJ, Wassermann EM, Tamir DI. Seizures from transcranial magnetic stimulation 2012–2016: results of a survey of active laboratories and clinics. Clin Neurophysiol. (2019) 130:1409–16. doi: 10.1016/j.clinph.2019.03.016
23. Ekhtiari H, Tavakoli H, Addolorato G, Baeken C. Transcranial electrical and magnetic stimulation (tES and TMS) for addiction medicine: a consensus paper on the present state of the science and the road ahead. Neurosci Biobehav Rev. (2019) 104:118–40. doi: 10.1016/j.neubiorev.2019.06.007
24. Taylor JJ, Newberger NG, Stern AP, Phillips A, Feifel D, Betensky RA, et al. Seizure risk with repetitive TMS: survey results from over a half-million treatment sessions. Brain Stimulation. (2021) 14:965–73. doi: 10.1016/j.brs.2021.05.012
25. Woods AJ, Antal A, Bikson M, Boggio PS, Brunoni AR, Celnik P, et al. A technical guide to tDCS, and related non-invasive brain stimulation tools. Clin Neurophysiol. (2016) 127:1031–48. doi: 10.1016/j.clinph.2015.11.012
26. Chhabra H, Bose A, Shivakumar V, Agarwal SM, Sreeraj VS, Shenoy S, et al. Tolerance of transcranial direct current stimulation in psychiatric disorders: an analysis of 2000+ sessions. Psychiatry Res. (2020) 284:112744. doi: 10.1016/j.psychres.2020.112744
27. Gault JM, Davis R, Cascella NG, Saks ER, Corripio-Collado I, Anderson WS, et al. Approaches to neuromodulation for schizophrenia. J Neurol Neurosurg Psychiatry. (2018) 89:777–87. doi: 10.1136/jnnp-2017-316946
28. Moretti J, Poh EZ, Rodger J. rTMS-induced changes in glutamatergic and dopaminergic systems: relevance to cocaine and methamphetamine use disorders. Front Neurosci. (2020) 14:137. doi: 10.3389/fnins.2020.00137
29. Herremans SC, Van Schuerbeek P, De Raedt R, Matthys F, Buyl R, De Mey J, et al. The impact of accelerated right prefrontal high-frequency repetitive transcranial magnetic stimulation (rTMS) on cue-reactivity: an fMRI study on craving in recently detoxified alcohol-dependent patients. PLoS ONE. (2015) 10:e0136182. doi: 10.1371/journal.pone.0136182
30. Mishra BR, Nizamie SH, Das B, Praharaj SK. Efficacy of repetitive transcranial magnetic stimulation in alcohol dependence: a sham-controlled study. Addiction. (2010) 105:49–55. doi: 10.1111/j.1360-0443.2009.02777.x
31. Rapinesi C, Kotzalidis GD, Serata D, Del Casale A, Bersani FS, Solfanelli A, et al. Efficacy of add-on deep transcranial magnetic stimulation in comorbid alcohol dependence and dysthymic disorder: three case reports. Prim Care Companion CNS Disord. (2013) 15:PCC.12m01438. doi: 10.4088/PCC.12m01438
32. Rapinesi C, Curto M, Kotzalidis GD, Del Casale A, Serata D, Ferri VR, et al. Antidepressant effectiveness of deep Transcranial Magnetic Stimulation (dTMS) in patients with Major Depressive Disorder (MDD) with or without Alcohol Use Disorders (AUDs): a 6-month, open label, follow-up study. J Affect Disord. (2015) 174:57–63. doi: 10.1016/j.jad.2014.11.015
33. Ceccanti M, Inghilleri M, Attilia ML, Raccah R, Fiore M, Zangen A, et al. Deep TMS on alcoholics: effects on cortisolemia and dopamine pathway modulation. A pilot study. Can J Physiol Pharmacol. (2015) 93:283–90. doi: 10.1139/cjpp-2014-0188
34. Amiaz R, Levy D, Vainiger D, Grunhaus L, Zangen A. Repeated high-frequency transcranial magnetic stimulation over the dorsolateral prefrontal cortex reduces cigarette craving and consumption. Addiction. (2009) 104:653–60. doi: 10.1111/j.1360-0443.2008.02448.x
35. Eichhammer P, Johann M, Kharraz A, Binder H, Pittrow D, Wodarz N, et al. High-frequency repetitive transcranial magnetic stimulation decreases cigarette smoking. J Clin Psychiatry. (2003) 64:951–3. doi: 10.4088/JCP.v64n0815
36. Pripfl J, Tomova L, Riecansky I, Lamm C. Transcranial magnetic stimulation of the left dorsolateral prefrontal cortex decreases cue-induced nicotine craving and EEG delta power. Brain Stimulation. (2014) 7:226–33. doi: 10.1016/j.brs.2013.11.003
37. Sheffer CE, Bickel WK, Brandon TH, Franck CT, Deen D, Panissidi L, et al. Preventing relapse to smoking with transcranial magnetic stimulation: feasibility and potential efficacy. Drug Alcohol Depend. (2018) 182:8–18. doi: 10.1016/j.drugalcdep.2017.09.037
38. Camprodon JA, Martínez-Raga J, Alonso-Alonso M, Shih M-C, Pascual-Leone A. One session of high frequency repetitive transcranial magnetic stimulation (rTMS) to the right prefrontal cortex transiently reduces cocaine craving. Drug Alcohol Depend. (2007) 86:91–4. doi: 10.1016/j.drugalcdep.2006.06.002
39. Politi E, Fauci E, Santoro A, Smeraldi E. Daily sessions of transcranial magnetic stimulation to the left prefrontal cortex gradually reduce cocaine craving. Am J Addictions. (2008) 17:345–6. doi: 10.1080/10550490802139283
40. Terraneo A, Leggio L, Saladini M, Ermani M, Bonci A, Gallimberti L. Transcranial magnetic stimulation of dorsolateral prefrontal cortex reduces cocaine use: a pilot study. Eur Neuropsychopharmacol. (2016) 26:37–44. doi: 10.1016/j.euroneuro.2015.11.011
41. Steele VR, Maxwell AM, Ross TJ, Stein EA, Salmeron BJ. Accelerated intermittent theta-burst stimulation as a treatment for cocaine use disorder: a proof-of-concept study. Front Neurosci. (2019) 13:1147. doi: 10.3389/fnins.2019.01147
42. Fischell AS, Ross TJ, Deng ZD, Salmeron BJ, Stein EA. Transcranial direct current stimulation applied to the dorsolateral and ventromedial prefrontal cortices in smokers modifies cognitive circuits implicated in the nicotine withdrawal syndrome. Biol Psychiatry Cogn Neurosci Neuroimaging. (2020) 5:448–60. doi: 10.1016/j.bpsc.2019.12.020
43. Del Felice A, Bellamoli E, Formaggio E, Manganotti P, Masiero S, Cuoghi G, et al. Neurophysiological, psychological and behavioural correlates of rTMS treatment in alcohol dependence. Drug Alcohol Depend. (2016) 158:147–53. doi: 10.1016/j.drugalcdep.2015.11.018
44. Herremans SC, Baeken C, Vanderbruggen N, Vanderhasselt MA, Zeeuws D, Santermans L, et al. No influence of one right-sided prefrontal HF-rTMS session on alcohol craving in recently detoxified alcohol-dependent patients: Results of a naturalistic study. Drug Alcohol Depend. (2012) 120:209–13. doi: 10.1016/j.drugalcdep.2011.07.021
45. Herremans SC, Vanderhasselt MA, De Raedt R, Baeken C. Reduced intra-individual reaction time variability during a Go–NoGo task in detoxified alcohol-dependent patients after one right-sided dorsolateral prefrontal HF-rTMS session. Alcohol Alcohol. (2013) 48:552–7. doi: 10.1093/alcalc/agt054
46. Harrison PJ. Neuropathology of schizophrenia. Psychiatry. (2008) 7:421–4. doi: 10.1016/j.mppsy.2008.07.013
47. Liu H, Luo Q, Du W, Li X, Zhang Z, Yu R, et al. Cigarette smoking and schizophrenia independently and reversibly altered intrinsic brain activity. Brain Imaging Behav. (2018) 12:1457–65. doi: 10.1007/s11682-017-9806-8
48. Pettorruso M, Spagnolo PA, Leggio L, Janiri L, Di Giannantonio M, Gallimberti L, et al. Repetitive transcranial magnetic stimulation of the left dorsolateral prefrontal cortex may improve symptoms of anhedonia in individuals with cocaine use disorder: a pilot study. Brain Stimul. (2018) 11:1195–7. doi: 10.1016/j.brs.2018.06.001
49. George MS, Taylor JJ, Short EB. The expanding evidence base for rTMS treatment of depression. Curr Opin Psychiatry. (2013) 26:13–8. doi: 10.1097/YCO.0b013e32835ab46d
50. Sterne JA, Savović J, Page MJ, Elbers RG, Blencowe NS, Boutron I, et al. RoB 2: a revised tool for assessing risk of bias in randomised trials. BMJ. (2019) 366:l4898. doi: 10.1136/bmj.l4898
51. Moher D, Liberati A, Tetzlaff J, Altman DG, The PRISMA Group (2009). Preferred Reporting items for Systematic Reviews and Meta-Analyses: the PRISMA Statement. PLoS Med. 6:e1000097. doi: 10.1371/journal.pmed1000097
52. Brunelin J. Nicotine smoking prevents the effects of frontotemporal transcranial direct current stimulation (tDCS) in hallucinating patients with schizophrenia. Brain Stimulation. (2015) 8:1225–7. doi: 10.1016/j.brs.2015.08.002
53. Huang W, Shen F, Jiangtao Z, Baoping X. Effect of repetitive transcranial magnetic stimulation on cigarette smoking in patients with schizophrenia. Shanghai Arch Psychiatry. (2016) 28:309–17. doi: 10.11919/j.issn.1002-0829.216044
54. Kamp D, Engelke C, Wobrock T, Kunze B. Letter to the editor: influence of rTMS on smoking in patients with schizophrenia. Schizophr Res. (2018) 192:481–4. doi: 10.1016/j.schres.2017.05.036
55. Kozak K, Sharif-Razi M, Morozova M, Gaudette EV, Barr MS, Daskalakis ZJ, et al. Effects of short-term, high-frequency repetitive transcranial magnetic stimulation to bilateral dorsolateral prefrontal cortex on smoking behavior and cognition in patients with schizophrenia and non-psychiatric controls. Schizophr Res. (2018) 197:441–3. doi: 10.1016/j.schres.2018.02.015
56. Kozak-Bidzinski K, Lowe DJE, Sanches M, Boileau I, Kiang M, Blumberger DM, et al. A preliminary randomized, sham-controlled trial of high-frequency repetitive transcranial magnetic stimulation (rTMS) to treat cannabis use and cognitive dysfunction in schizophrenia. NPJ Schizophr. (2022). [Epub ahead of print].
57. Prikryl RPKH, Kašpárek T, Vrzalová M, Cešková E, Sochorová A. The effect of repetitive transcranial magnetic stimulation on nicotinism in schizophrenia: an open study. Czech Slovak Psychiatry. (2013) 109:5.
58. Prikryl R, Ustohal L, Kucerova HP, Kasparek T, Jarkovsky J, Hublova V, et al. Repetitive transcranial magnetic stimulation reduces cigarette consumption in schizophrenia patients. Prog Neuropsychopharmacol Biol Psychiatry. (2014) 49:30–5. doi: 10.1016/j.pnpbp.2013.10.019
59. Smith RC, Boules S, Mattiuz S, Youssef M, Tobe RH, Sershen H, et al. Effects of transcranial direct current stimulation (tDCS) on cognition, symptoms, and smoking in schizophrenia: a randomized controlled study. Schizophr Res. (2015) 168:260–6. doi: 10.1016/j.schres.2015.06.011
60. Wing VC, Bacher I, Wu BS, Daskalakis ZJ, George TP. High frequency repetitive transcranial magnetic stimulation reduces tobacco craving in schizophrenia. Schizophr Res. (2012) 139:264–6. doi: 10.1016/j.schres.2012.03.006
61. Sayette MA, Shiffman S, Tiffany ST, Niaura RS, Martin CS, Shadel WG. The measurement of drug craving. Addiction. (2000) 95:S189–210. doi: 10.1046/j.1360-0443.95.8s2.8.x
62. Steele VR, Steele VR, Maxwell AM. Treating cocaine and opioid use disorder with transcranial magnetic stimulation: a path forward. Pharmacol Biochem Behav. (2021) 209:173240. doi: 10.1016/j.pbb.2021.173240
63. George TP, Vessicchio JC, Termine A, Sahady DM, Head CA, Pepper WT, et al. Effects of smoking abstinence on visuospatial working memory function in schizophrenia. Neuropsychopharmacology. (2002) 26:75–85. doi: 10.1016/S0893-133X(01)00296-2
64. Koukouli F, Rooy M, Tziotis D, Sailor KA, O'Neill HC, Levenga J, et al. Nicotine reverses hypofrontality in animal models of addiction and schizophrenia. Nat Med. (2017) 23:347–54. doi: 10.1038/nm.4274
65. Kozak K, Lowe DJE, George TP. Effects of tobacco smoking status on verbal learning and memory in patients with schizophrenia and non-psychiatric controls. Am J Addict. (2019) 28:503–11. doi: 10.1111/ajad.12903
66. Wing VC, Bacher I, Sacco KA, George TP. Neuropsychological performance in patients with schizophrenia and controls as a function of cigarette smoking status. Psychiatry Res. (2011) 188:320–6. doi: 10.1016/j.psychres.2011.05.037
67. Freitas C, Fregni F, Pascual-Leone A. Meta-analysis of the effects of repetitive transcranial magnetic stimulation (rTMS) on negative and positive symptoms in schizophrenia. Schizophr Res. (2009) 108:11–24. doi: 10.1016/j.schres.2008.11.027
68. Limongi R, Mackinley M, Dempster K, Khan AR, Gati JS, Palaniyappan L. Frontal–striatal connectivity and positive symptoms of schizophrenia: implications for the mechanistic basis of prefrontal rTMS. Eur Arch Psychiatry Clin Neurosci. (2021) 271:3–15. doi: 10.1007/s00406-020-01163-6
69. Hovington CL, McGirr A, Lepage M, Berlim MT. Repetitive transcranial magnetic stimulation (rTMS) for treating major depression and schizophrenia: a systematic review of recent meta-analyses. Ann Med. (2013) 45:308–21. doi: 10.3109/07853890.2013.783993
70. Martinotti G, De Risio L, Vannini C, Schifano F, Pettorruso M, Di Giannantonio M. Substance-related exogenous psychosis: a postmodern syndrome. CNS Spectrums. (2021) 26:84–91. doi: 10.1017/S1092852920001479
71. Ricci V, Ceci F, Di Carlo F, Lalli A, Ciavoni L, Mosca A, et al. Cannabis use disorder and dissociation: a report from a prospective first-episode psychosis study. Drug Alcohol Depend. (2021) 229:109118. doi: 10.1016/j.drugalcdep.2021.109118
72. Sakkas P, Mihalopoulou P, Mourtzouhou P, Psarros C, Masdrakis V, Politis A, et al. Induction of mania by rTMS: report of two cases. Eur Psychiatry. (2003) 18:196–8. doi: 10.1016/S0924-9338(03)00048-8
73. Kaster TS, Knyahnytska Y, Noda Y, Downar J, Daskalakis ZJ, Blumberger DM. Treatment-emergent mania with psychosis in bipolar depression with left intermittent theta-burst rTMS. Brain Stimulation. (2020) 13:705–6. doi: 10.1016/j.brs.2020.02.018
74. Knox ED, Bota RG. Transcranial magnetic stimulation-associated mania with psychosis: a case report. Ment Health Clin. (2021) 11:373–5. doi: 10.9740/mhc.2021.11.373
75. Archibald L. Alcohol use disorder and schizophrenia and schizoaffective? disorders. Alcohol Res Curr Rev. (2019) 40:1–9. doi: 10.35946/arcr.v40.1.06
76. Ringen PA, Melle I, Birkenæs AB, Engh JA, Færden A, Jónsdóttir H, et al. Illicit drug use in patients with psychotic disorders compared with that in the general population: a cross-sectional study. Act Psychiatr Scand. (2008) 117:133–8. doi: 10.1111/j.1600-0447.2007.01135.x
77. Sara GE, Burgess PM, Malhi GS, Whiteford HA, Hall WC. Stimulant and other substance use disorders in schizophrenia: Prevalence, correlates and impacts in a population sample. Australian N Z J Psychiatry. (2014) 48:1036–47. doi: 10.1177/0004867414533838
78. Althubaiti A. Information bias in health research: definition, pitfalls, and adjustment methods. J Multidisciplin Healthcare. (2016) 2016:211. doi: 10.2147/JMDH.S104807
79. Agarwal SM, Bose A, Shivakumar V, Narayanaswamy JC, Chhabra H, Kalmady SV, et al. Impact of antipsychotic medication on transcranial direct current stimulation (tDCS) effects in schizophrenia patients. Psychiatry Res. (2016) 235:97–103. doi: 10.1016/j.psychres.2015.11.042
Keywords: substance use disorder, nicotine, cannabis, psychosis, schizophrenia, neuromodulation, rTMS, tDCS
Citation: Johnstone S, Sorkhou M, Al-Saghir N, Lowe DJE, Steele VR, Pearlson GD, Castle DJ and George TP (2022) Neuromodulation to Treat Substance Use Disorders in People With Schizophrenia and Other Psychoses: A Systematic Review. Front. Psychiatry 13:793938. doi: 10.3389/fpsyt.2022.793938
Received: 12 October 2021; Accepted: 14 January 2022;
Published: 14 February 2022.
Edited by:
Sinan Guloksuz, Maastricht University Medical Centre, NetherlandsReviewed by:
Giovanni Martinotti, University of Studies G. d'Annunzio Chieti and Pescara, ItalyCopyright © 2022 Johnstone, Sorkhou, Al-Saghir, Lowe, Steele, Pearlson, Castle and George. This is an open-access article distributed under the terms of the Creative Commons Attribution License (CC BY). The use, distribution or reproduction in other forums is permitted, provided the original author(s) and the copyright owner(s) are credited and that the original publication in this journal is cited, in accordance with accepted academic practice. No use, distribution or reproduction is permitted which does not comply with these terms.
*Correspondence: Tony P. George, dG9ueS5nZW9yZ2VAY2FtaC5jYQ==
Disclaimer: All claims expressed in this article are solely those of the authors and do not necessarily represent those of their affiliated organizations, or those of the publisher, the editors and the reviewers. Any product that may be evaluated in this article or claim that may be made by its manufacturer is not guaranteed or endorsed by the publisher.
Research integrity at Frontiers
Learn more about the work of our research integrity team to safeguard the quality of each article we publish.