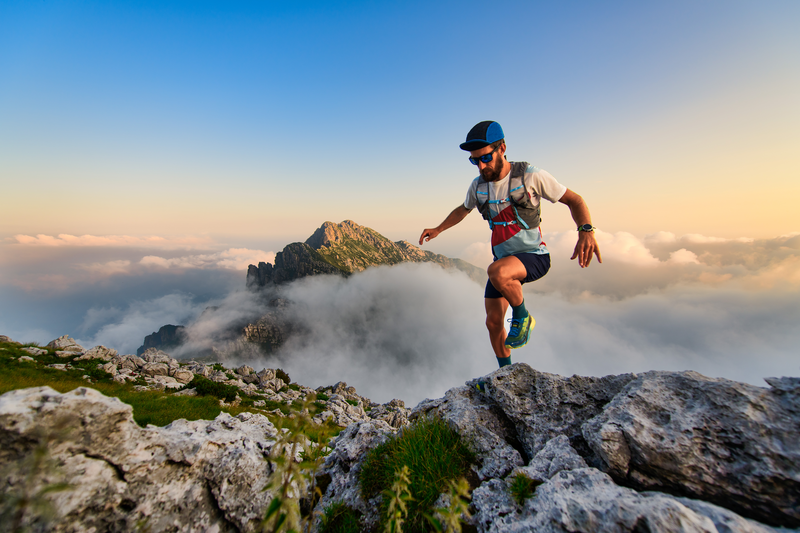
94% of researchers rate our articles as excellent or good
Learn more about the work of our research integrity team to safeguard the quality of each article we publish.
Find out more
SYSTEMATIC REVIEW article
Front. Psychiatry , 22 December 2022
Sec. Schizophrenia
Volume 13 - 2022 | https://doi.org/10.3389/fpsyt.2022.1083480
This article is part of the Research Topic Cerebellar Structure and Function in Psychotic Disorders: From Mechanisms to Clinics View all 7 articles
Background: In schizophrenia, the structural changes in the cerebellum are associated with patients’ cognition and motor deficits. However, the findings are inconsistent owing to the heterogeneity in sample size, magnetic resonance imaging (MRI) scanners, and other factors among them. In this study, we conducted a meta-analysis to characterize the anatomical changes in cerebellar subfields in patients with schizophrenia.
Methods: Systematic research was conducted to identify studies that compare the gray matter volume (GMV) differences in the cerebellum between patients with schizophrenia and healthy controls with a voxel-based morphometry (VBM) method. A coordinate-based meta-analysis was adopted based on seed-based d mapping (SDM) software. An exploratory meta-regression analysis was conducted to associate clinical and demographic features with cerebellar changes.
Results: Of note, 25 studies comprising 996 patients with schizophrenia and 1,109 healthy controls were included in the present meta-analysis. In patients with schizophrenia, decreased GMVs were demonstrated in the left Crus II, right lobule VI, and right lobule VIII, while no increased GMV was identified. In the meta-regression analysis, the mean age and illness duration were negatively associated with the GMV in the left Crus II in patients with schizophrenia.
Conclusion: The most significant structural changes in the cerebellum are mainly located in the posterior cerebellar hemisphere in patients with schizophrenia. The decreased GMVs of these regions might partly explain the cognitive deficits and motor symptoms in patients with schizophrenia.
Schizophrenia has been widely considered a psychiatric disorder characterized by cognitive deficits (1–6) and motor dysfunctions (7, 8), notably in verbal memory, working memory, processing speed, and motor control (9). A wide range of brain structural and functional alterations (10) have been found in magnetic resonance imaging (MRI) studies of schizophrenia, for instance, the progressive losses of cerebral cortical volume and thickness in the frontal, temporal, parietal, and cingulate cortices and the thalamus (11); decreased regional homogeneity (ReHo) in the cingulate cortex, occipital gyrus and cuneus; and altered functional connectivity of the salience, central executive and default-mode networks (12–15). Although the cerebral function and structure are of great importance in the pathophysiological progression of schizophrenia, accumulating evidence indicates that the cerebellum also plays a vital role in emotion, cognition, motor, and executive functions in patients with schizophrenia (16–18). Andreasen et al. (19, 20) first proposed the role of the cerebellum in “cognitive dysmetria” and raised the concept of the “cerebello-thalamo-cortical circuit” in schizophrenia. This circuit establishes the functional pathway of information transfer between the cerebral cortex and cerebellum. Its hyperconnectivity was identified as a potential biomarker for genetic risk, diagnosis, and disorder progression in schizophrenia (21–24). In addition, a previous study using a large adolescent cohort indicated that cerebellar morphology was correlated with both general cognitive function and general psychopathology and that the cerebellum might be a critical structure in the development of grievous mental psychosis (25). Regarding the abnormalities of cerebellar subregions in patients with schizophrenia, decreased gray matter volumes (GMV) were reported in the Crus I/II (26, 27) and lobule III, IV (28), V (29, 30), VI (27, 31), and VIIb/VIIIa (32). Some studies reported no significant cerebellar structural changes when comparing patients with schizophrenia to healthy controls (33, 34). In general, the altered cerebellar subregions were inconsistent in the structural MRI studies of schizophrenia.
Various reasons may account for the heterogeneity among abnormal cerebellar structures in patients with schizophrenia, including disorder heterogeneity, sample size, demographic characteristics, the administration of antipsychotic drugs, scanning parameters, and processing methods. The heterogeneity might be explained by the fact that previous studies mainly focused on the cerebral structures instead of structural deficits in the cerebellum.
Previous studies demonstrated structural alterations of the cerebellum in schizophrenia. A mega-analysis of 983 patients with schizophrenia spectrum disorders indicated that the losses of cerebellar GMVs in the patients were mainly located in regions concerning higher-level cognitive functions (35). A previous meta-analysis of first-episode schizophrenia involving both adolescents and adults suggested that the decreased GMVs were mainly located in Crus II and lobule IV, right lobule V, and right lobule VII (36).
To further illustrate the remarkable regional changes in the cerebellum in patients with schizophrenia, a meta-analysis was conducted that mainly focused on cerebellar changes in participants aged ≥ 18 years and only patients diagnosed with schizophrenia in terms of the Diagnostic and Statistical Manual of Mental Disorders (DSM). An exploratory meta-regression was performed to determine the potential relationship between abnormal cerebellar structures and clinical variables.
This meta-analysis adhered to the Preferred Reporting Items for Systematic Reviews and Meta-Analyses (PRISMA) statement. Related literature was searched in the Embase, PubMed, and Web of Science databases from 1 August 1985 to 1 August 2022. The keywords were “schizophrenia” and “cerebellum” and “magnetic resonance imaging” on the condition of “All Fields”. We manually searched the reference lists of the selected articles and related reviews. We included studies meeting the following criteria: (1) peer-reviewed articles published in English; (2) studies comparing cerebellar GMV changes between patients with schizophrenia and healthy controls using voxel-based analytical methods; and (3) studies demonstrating cerebellar GMV alterations in the Montreal Neurological Institute (MNI) or Talairach coordinates. Studies were excluded if (1) they were commentaries, editorials, case reports, or letters; (2) they included patients with a diagnosis other than schizophrenia, such as schizoaffective disorder, bipolar affective disorders, organic mental disorders, substance-related disorders, or early onset schizophrenia (both childhood and adolescent schizophrenia) in the patients’ group; (3) they did not use MRI to show gray matter differences in the cerebellum; or (4) they carried out image processing using only region of interest (ROI) or manual approaches. Two investigators conducted the literature search independently, and the results were compared. When confronted with controversies, an agreement was reached between the investigators during the inclusion of studies for this meta-analysis.
We recorded demographic information and clinical data, including sample size, sex, mean age, age of onset, duration of illness, years of education, and Positive and Negative Syndrome Scale (PANSS) scores. Basic methodological materials (statistical threshold and correction) and scanning parameters [slice thickness, field strength, and full width at half maximum (FWHM)] were well documented using Microsoft Excel. In addition, the peak coordinates of the main results and effect sizes were recorded for SDM calculations.
To assess the quality of each study, a modified 10-point checklist was obtained from earlier studies in line with Newcastle Ottawa Scale (37, 38). The checklist contained three categories: five items for participant inclusion and exclusion, three items for imaging scanning parameters and analytical methods, and two items for results and conclusions. The scores were separated into three levels: 7–9 was regarded as good, 4–6 was fair, and 0–3 was poor. Each item was scored as 0, 0.5, or 1 point if the criteria were unfulfilled, partially met, or fully met, respectively, and any study scoring > 5.0 points was included in the meta-analysis. The details of the checklist are presented in Supplementary Table 1. However, this checklist was only used to evaluate the quality of the studies included in this meta-analysis rather than to judge the work or authors.
An anisotropic effect-size version of seed-based d mapping (AES-SDM) software (version 5.15)1 was adopted in this meta-analysis to detect consistent GMV abnormalities in patients with schizophrenia when compared with healthy controls. AES-SDM uses effect sizes and permits the combination of reported peak coordinates with statistical parametric maps, providing elaborate and convincing meta-analyses (39, 40). According to the AES-SDM tutorial, statistical maps and effect size maps of the coordinates of each study were recreated (“gray matter” numbers of randomization = 1, anisotropy = 1, isotropic full width at half maximum FWHM = 20 mm, mask = “gray matter”). Moreover, individual research maps were entered into the meta-analysis. Jackknife sensitivity, heterogeneity, and publication bias analyses were performed to assess the sensitivity and heterogeneity of the results. The analytical parameters obtained from previous studies (41–43) are listed as follows: voxel threshold p = 0.005, peak height threshold z = 1.00, and cluster size threshold = 10 voxels.
Subgroup analyses were tested according to studies reported with corrected results, and studies used a 3.0-T MRI scanning machine. Based on a linear model, meta-regression analysis was performed to detect the association between GMV abnormalities and clinical data (age, age of onset, sex, illness duration, and PANSS subscale scores). The analytical parameters were as follows: threshold of p = 0.0005, peak height threshold z = 1.00, and cluster size threshold = 10 voxels (37, 43). Further details of the jackknife, heterogeneity, publication bias analyses, and meta-regression are described in the Supplementary material.
The flowchart of the literature search is presented in Figure 1. The demographic information, clinical data, and scanning materials of all included GMV studies are summarized in Supplementary Table 2. A total of 25 VBM studies (6, 27, 30, 32, 44–64) were distinguished based on our search protocol. Two articles (52, 53) published by the same author were both included because the cohorts did not overlap. All patients were diagnosed with schizophrenia in line with the DSM criteria, excluding patients with any other schizoaffective disorder, bipolar affective disorders, organic mental disorders, or other mental disorders. In total, 996 patients with schizophrenia (men, 572; mean age, 29.63 years; mean illness duration, 6.19 years; mean PANSS total score, 103.70) and 1,109 matched healthy controls (649 men, mean age 29.90 years) were analyzed. Only five studies (45, 48, 50, 55, 59) were focused on drug-naïve patients. The threshold of 15 studies (27, 30, 44, 46, 48–50, 56–61, 63, 64) was corrected for multiple comparisons, and 14 studies (27, 30, 32, 45–48, 50, 52, 53, 59, 60, 63, 64) used the PANSS for psychotic symptom assessment. The field strength of partial studies was 3.0-T MRI (9/25 datasets), and the thickness was 1 mm (14/25 datasets). The average quality score of the 25 studies was 8.04 (range 7–9.5), which implies that the quality of the included studies was at a high level.
Notably, 17 datasets revealed decreased GMVs involving the bilateral cerebellum, especially in the left Crus I/II and right lobule VI/VIIb in patients with schizophrenia. Six datasets suggested increased GMVs in the bilateral cerebellum, involving the anterior part of the bilateral cerebellum, bilateral cerebellum III, and Vermis IV and V.
Integrating all 25 studies in this meta-analysis, patients with schizophrenia showed decreased GMVs in the left Crus II (z = −1.991, p = 0.000165164), right lobule VI (z = −1.484, p = 0.001656592), and right lobule VIII (z = −1.409, p = 0.002353311; Table 1 and Figure 2) when compared with healthy controls. No increased cerebellar GMV was identified.
Table 1. Gray matter volume changes between patients with schizophrenia and healthy controls (25 studies).
Figure 2. Regional cerebellar GMV changes in patients with schizophrenia compared with healthy controls in our meta-analysis. The blue color represented lower gray matter volume in left Crus II, right lobule VI, and right lobule VIII compared with healthy controls in our meta-analysis. The corresponding cerebellar regions were pointed out at the peak coordinate level.
In the subgroup meta-analysis, studies that reported corrected results (15 studies) and studies that used a 3.0-T scanning machine (9 studies) were in high accordance with the integrated results (Supplementary Table 3).
In the jackknife analysis, decreased GMV in the left Crus II was in accordance with all combinations of the 25 datasets. Moreover, decreased GMVs in the right lobule VI and right lobule VIII remained statistically significant in 22/25 datasets (Supplementary Table 4). This finding indicates that the significant cerebellar gray volume differences showed good robustness and consistency in this meta-analysis. No significant statistical heterogeneity was identified in the meaningful cerebellar GMV alterations between studies. The Egger test of funnel plot asymmetry did not show statistical significance in the analysis of publication bias. The forest plots are shown in Supplementary Figure 1.
In the linear regression analysis, mean age (r = −0.461, p = 0.020) and illness duration (r = −0.496, p = 0.019) were negatively associated with GMV in the left Crus II in patients with schizophrenia (Figure 3). No association was found between statistically significant GMV alterations and age of onset, PANSS total scores, or subscale scores.
Figure 3. The results of the meta-regression analysis. (A) The mean age was negatively associated with GMV in left Crus II in patients with schizophrenia (r = –0.461, p = 0.020). (B) The related significant cluster of the left crus II in this meta-regression analysis of mean age. (C) The illness duration was negatively associated with GMV in left Crus II in patients with schizophrenia (r = –0.461, p = 0.020). (D) The related significant cluster of the left Crus II in this meta-regression analysis of illness duration. In panels (A,C), the effect sizes to create the plot were extracted from the peak of the maximum slope difference, and each study was represented as a dot (meta-regression signed differential mapping slope). In panels (B,D), the decreased GMV in the left Crus II was shown in blue color.
This study, which included 996 patients with schizophrenia and 1,109 healthy controls, mainly investigated structural changes in the cerebellum and identified GMV decreases in the left Crus II, right lobule VI, and right lobule VIII in patients with schizophrenia. Similarly, these findings showed good repeatability in both subgroup meta-analysis and jackknife sensitivity analysis. The cerebellar subregional GMV alterations discovered in our meta-analysis might be one of the schizophrenic neuroanatomical bases, especially in the left Crus II. Moreover, we also found that mean age and illness duration were negatively associated with the GMV in the left Crus II, which might suggest that schizophrenia is a progressive disorder.
Consistent with our findings in this meta-analysis, multiple former studies identified decreased GMVs mainly located in the left Crus II, right lobule VI, and right lobule VIII (35, 36, 65–67). In a meta-analysis of 283 volumetric brain studies, decreased cerebellar volume was identified in medicated patients with schizophrenia (68). Moberget et al. (35) found regional decreased GMVs in the bilateral Crus I, left Crus II, right lobule VIII, and right lobule IX in a large voxel-wise level mega-analysis and clarified that the cerebellum was a critical point of brain connectivity in patients with schizophrenia spectrum disorders. A worldwide multicenter study (66), including 182 patients with schizophrenia and 198 healthy controls, suggested that GMV losses mainly occurred in lobule VIIb and Crus II. The volume changes in the cerebellum may be the most vigorous and stable brain imaging findings in patients with schizophrenia.
Purkinje cells (PCs), a central component of the cerebellum, are correlated with cerebellar function and development. In addition, PCs provide signals in balance, motor coordination, and cognition learning (69–71). A former animal experiment stated that the losses of PCs may lead to motion abnormalities and schizophrenia-like behaviors (72). In addition, the number and size of PCs are related to extensive cognitive impairments and psychopathological symptoms in schizophrenia patients (73). Decreased Purkinje neuron linear density was detected in the cerebellum, especially in the vermis, and presented as cerebellar volume decreases in MRI (72, 74, 75). Thus, a reduction in cerebellar GMV, shown on brain neuroimaging, presumably results in clinical symptoms in patients with schizophrenia, which might be explained by the abnormal number and size of PCs.
Nevertheless, the findings of decreased cerebellar GMV in the left Crus II in patients with schizophrenia are contrary to those of previous studies. For instance, a former study by Morimoto et al. (33) suggested that no differences were found in either white matter volumes or GMVs of the bilateral Crus I/II between patients with schizophrenia and healthy controls. The inconsistency of results might be explained by the differences in the study design, the heterogeneous conditions of schizophrenia, and methodological differences.
The Crus II and lobule VI/VIII occupy a major part of the posterior cerebellar hemisphere (76). These altered cerebellar GMV regions were considered to connect and function together with the cerebrum for high-level cognitive operations, such as sensorimotor control, language, verb generation, working memory, spatial processing, and emotion processing (67, 77–83). More specifically, the Crus II was regarded as a critical hub in a recent functional connectome study of healthy volunteers. The Crus II connected with multiple resting-state networks in the cerebrum, such as the default-mode, cingulo-parietal, frontoparietal, ventral attention, and language networks (84). We suggested that the GMV decreases in these cerebellar subregions might cause the interruptions of cerebrocerebellar communications in schizophrenia (85, 86). For patients with schizophrenia, decreased connectivity between the Crus II and ventral attention, salience, and default-mode networks, as well as increased connectivity with the somatomotor network, were shown in a cerebrocerebellar functional connectivity study (86, 87). An updated review also identified that lobule VI was related to the default-mode network and the executive control network; furthermore, lobule VIII was linked with the sensorimotor network (88). Regions of anatomical abnormalities were extensively involved in functional connectivity between the cerebrum and cerebellum. A non-invasive transcranial magnetic stimulation targeting the Crus I/II was adopted in humans, and it strengthened the point of view that the cerebellum plays a key role in cerebral functional connectivity within networks, especially in the default-mode network (89). Moreover, the GMVs of the bilateral cerebellum I/II were associated with the severity of symptoms in both individuals with ultrahigh-risk and patients with first-episode schizophrenia (33). In summary, the Crus II and lobule VI/VIII widely participated in the cerebrocerebellar functional connectivity and were involved in high-level functions in patients with schizophrenia. We hypothesized that abnormal volume changes in these regions might be potential factors leading to cognitive dysfunction and motor symptoms in patients with schizophrenia.
In addition, our study also found that mean age and illness duration were negatively associated with GMV in the left Crus II in patients with schizophrenia. This finding indicated a further reduction of GMV in the cerebellum with increased age and a prolonged illness course. In accordance with the previous opinion, schizophrenia is a progressive disorder (6, 90–93). However, antipsychotic medication might contribute to changes in cerebellar GMV (94). The progressive loss of GMV might be a confounding consequence of antipsychotic medication, age, and illness duration. Thus, this finding should be interpreted with caution.
There are some limitations to our meta-analysis. First, all the included studies were VBM studies conducted mainly from the perspective of the whole brain, and the details of subregional cerebellar information were hard to obtain, except for the specific peak coordinates. Technically, more precise segmentation approaches have been applied to cerebellar subfields (66). However, diverse novel methods (95) have only been applied in limited studies, which do not have enough quantity to conduct a meta-analysis. Second, we only concentrated on the significant cerebellar changes that have been reported, and we omitted the results with no significance in the VBM studies. At the same time, no publication bias was identified in our study. Third, clinical and methodological heterogeneity among different studies could contribute to the evaluation of GMV. To minimize the confounding factors, the subgroup meta-analysis was performed based on studies concerning the 3.0-T MRI and studies with corrected results. The results of the subgroup analysis were in line with the present research. Fourth, most of the patients with schizophrenia were medicated or had a long illness duration in the included studies. A meta-regression analysis was carried out to specify the association between illness duration and significant cerebellar GMV changes, which implicated that illness duration was negatively associated with decreased GMV in the left Crus II.
The current meta-analysis of VBM studies provides consolidated evidence that structural changes in the cerebellum are consistently located in the left Crus II, right lobule VI, and right lobule VIII in patients with schizophrenia. The decreased GMVs of these regions might associate with the interruptions of cerebrocerebellar communications in patients with schizophrenia and might partly explain cognitive deficits and motor symptoms in patients with schizophrenia.
The original contributions presented in this study are included in the article/Supplementary material, further inquiries can be directed to the corresponding authors.
WZ and SL contributed to the design of the study and the supervision of all the work of this review. XL and NL contributed to the literature search and drafted the manuscript. CY guided the meta-analysis process. All authors made critical revisions to the manuscript for important intellectual content and gave final approval of the version to be submitted.
This study was supported by the National Key R&D Program of China (Project nos. 2022YFC2009901 and 2022YFC2009900), the National Natural Science Foundation of China (Project nos. 82120108014, 82071908, and 81621003), CAMS Innovation Fund for Medical Sciences (CIFMS) (Project no. 2021-I2M-C&T-A-022), Sichuan Science and Technology Program (Project no. 2021JDTD0002), and SL acknowledges the support from Humboldt Foundation Friedrich Wilhelm Bessel Research Award and Chang Jiang Scholars (Program no. T2019069).
WZ consults with VeraSci.
The remaining authors declare that the research was conducted in the absence of any commercial or financial relationships that could be construed as a potential conflict of interest.
All claims expressed in this article are solely those of the authors and do not necessarily represent those of their affiliated organizations, or those of the publisher, the editors and the reviewers. Any product that may be evaluated in this article, or claim that may be made by its manufacturer, is not guaranteed or endorsed by the publisher.
The Supplementary Material for this article can be found online at: https://www.frontiersin.org/articles/10.3389/fpsyt.2022.1083480/full#supplementary-material
1. Kurtz M, Gopal S, John S, Thara R. Cognition, social cognition and functional disability in early-stage schizophrenia: a study from southern India. Psychiatry Res. (2018) 265:231–7. doi: 10.1016/j.psychres.2018.03.091
2. Dickinson D, Zaidman S, Giangrande E, Eisenberg D, Gregory M, Berman K. Distinct polygenic score profiles in schizophrenia subgroups with different trajectories of cognitive development. Am J Psychiatry. (2020) 177:298–307. doi: 10.1176/appi.ajp.2019.19050527
3. Toulopoulou T, Zhang X, Cherny S, Dickinson D, Berman K, Straub R, et al. Polygenic risk score increases schizophrenia liability through cognition-relevant pathways. Brain. (2019) 142:471–85. doi: 10.1093/brain/awy279
4. Seitz-Holland J, Wojcik J, Cetin-Karayumak S, Lyall A, Pasternak O, Rathi Y, et al. Cognitive deficits, clinical variables, and white matter microstructure in schizophrenia: a multisite harmonization study. Mol Psychiatry. (2022) 2022:1731. doi: 10.1038/s41380-022-01731-3
5. Cannon T. How schizophrenia develops: cognitive and brain mechanisms underlying onset of psychosis. Trends Cogn Sci. (2015) 19:744–56. doi: 10.1016/j.tics.2015.09.009
6. Tanskanen P, Ridler K, Murray G, Haapea M, Veijola J, Jääskeläinen E, et al. Morphometric brain abnormalities in schizophrenia in a population-based sample: relationship to duration of illness. Schizophr Bull. (2010) 36:766–77. doi: 10.1093/schbul/sbn141
7. Dickson H, Laurens K, Cullen A, Hodgins S. Meta-analyses of cognitive and motor function in youth aged 16 years and younger who subsequently develop schizophrenia. Psychol Med. (2012) 42:743–55. doi: 10.1017/s0033291711001693
8. Forsyth J, Lewis D. Mapping the consequences of impaired synaptic plasticity in schizophrenia through development: an integrative model for diverse clinical features. Trends Cogn Sci. (2017) 21:760–78. doi: 10.1016/j.tics.2017.06.006
9. Geisler D, Walton E, Naylor M, Roessner V, Lim K, Charles Schulz S, et al. Brain structure and function correlates of cognitive subtypes in schizophrenia. Psychiatry Res. (2015) 234:74–83. doi: 10.1016/j.pscychresns.2015.08.008
10. Sui J, Qi S, van Erp T, Bustillo J, Jiang R, Lin D, et al. Multimodal neuromarkers in schizophrenia via cognition-guided mri fusion. Nat Commun. (2018) 9:3028. doi: 10.1038/s41467-018-05432-w
11. Alemán-Gómez Y, Najdenovska E, Roine T, Fartaria MJ, Canales-Rodríguez EJ, Rovó Z, et al. Partial-volume modeling reveals reduced gray matter in specific thalamic nuclei early in the time course of psychosis and chronic schizophrenia. Hum Brain Mapp. (2020) 41:4041–61. doi: 10.1002/hbm.25108
12. Huang Y, Wang W, Hei G, Yang Y, Long Y, Wang X, et al. Altered regional homogeneity and cognitive impairments in first-episode schizophrenia: a resting-state fmri study. Asian J Psychiatr. (2022) 71:103055. doi: 10.1016/j.ajp.2022.103055
13. Yang M, Gao S, Zhang X. Cognitive deficits and white matter abnormalities in never-treated first-episode schizophrenia. Transl Psychiatry. (2020) 10:368. doi: 10.1038/s41398-020-01049-0
14. Fujiwara H, Yassin W, Murai T. Neuroimaging studies of social cognition in schizophrenia. Psychiatry Clin Neurosci. (2015) 69:259–67. doi: 10.1111/pcn.12258
15. Yasuda Y, Okada N, Nemoto K, Fukunaga M, Yamamori H, Ohi K, et al. Brain morphological and functional features in cognitive subgroups of schizophrenia. Psychiatry Clin Neurosci. (2020) 74:191–203. doi: 10.1111/pcn.12963
16. Kong L, Herold C, Cheung E, Chan R, Schröder J. Neurological soft signs and brain network abnormalities in schizophrenia. Schizophr Bull. (2020) 46:562–71. doi: 10.1093/schbul/sbz118
17. Gao J, Tang X, Wang C, Yu M, Sha W, Wang X, et al. Aberrant cerebellar neural activity and cerebro-cerebellar functional connectivity involving executive dysfunction in schizophrenia with primary negative symptoms. Brain Imaging Behav. (2020) 14:869–80. doi: 10.1007/s11682-018-0032-9
18. Moberget T, Ivry R. Prediction, psychosis, and the cerebellum. Biol Psychiatry Cogn Neurosci Neuro. (2019) 4:820–31. doi: 10.1016/j.bpsc.2019.06.001
19. Andreasen N, O’Leary D, Cizadlo T, Arndt S, Rezai K, Ponto L, et al. Schizophrenia and cognitive dysmetria: a positron-emission tomography study of dysfunctional prefrontal-thalamic-cerebellar circuitry. Proc Natl Acad Sci USA. (1996) 93:9985–90. doi: 10.1073/pnas.93.18.9985
20. Andreasen N, Paradiso S, O’Leary D. “Cognitive dysmetria” as an integrative theory of schizophrenia: a dysfunction in cortical-subcortical-cerebellar circuitry? Schizophr Bull. (1998) 24:203–18. doi: 10.1093/oxfordjournals.schbul.a033321
21. Bernard J, Orr J, Mittal V. Cerebello-thalamo-cortical networks predict positive symptom progression in individuals at ultra-high risk for psychosis. Neuroimage Clin. (2017) 14:622–8. doi: 10.1016/j.nicl.2017.03.001
22. Cao H, Ingvar M, Hultman C, Cannon T. Evidence for cerebello-thalamo-cortical hyperconnectivity as a heritable trait for schizophrenia. Transl Psychiatry. (2019) 9:192. doi: 10.1038/s41398-019-0531-5
23. Cao H, Wei X, Hu N, Zhang W, Xiao Y, Zeng J, et al. Cerebello-thalamo-cortical hyperconnectivity classifies patients and predicts long-term treatment outcome in first-episode schizophrenia. Schizophr Bull. (2022) 48:505–13. doi: 10.1093/schbul/sbab112
24. Cao H, Chén O, Chung Y, Forsyth J, McEwen S, Gee D, et al. Cerebello-thalamo-cortical hyperconnectivity as a state-independent functional neural signature for psychosis prediction and characterization. Nat Commun. (2018) 9:3836. doi: 10.1038/s41467-018-06350-7
25. Moberget T, Alnæs D, Kaufmann T, Doan N, Córdova-Palomera A, Norbom L, et al. Cerebellar gray matter volume is associated with cognitive function and psychopathology in adolescence. Biol Psychiatry. (2019) 86:65–75. doi: 10.1016/j.biopsych.2019.01.019
26. Kühn S, Romanowski A, Schubert F, Gallinat J. Reduction of cerebellar grey matter in crus i and ii in schizophrenia. Brain Struct Funct. (2012) 217: 523–9. doi: 10.1007/s00429-011-0365-2
27. He H, Luo C, Luo Y, Duan M, Yi Q, Biswal B, et al. Reduction in gray matter of cerebellum in schizophrenia and its influence on static and dynamic connectivity. Hum Brain Mapp. (2019) 40:517–28. doi: 10.1002/hbm.24391
28. Rasser P, Schall U, Peck G, Cohen M, Johnston P, Khoo K, et al. Cerebellar grey matter deficits in first-episode schizophrenia mapped using cortical pattern matching. NeuroImage. (2010) 53:1175–80.
29. Gupta C, Calhoun V, Rachakonda S, Chen J, Patel V, Liu J, et al. Patterns of gray matter abnormalities in schizophrenia based on an international mega-analysis. Schizophr Bull. (2015) 41:1133–42. doi: 10.1093/schbul/sbu177
30. Sheng J, Zhu Y, Lu Z, Liu N, Huang N, Zhang Z, et al. Altered volume and lateralization of language-related regions in first-episode schizophrenia. Schizophr Res. (2013) 148:168–74. doi: 10.1016/j.schres.2013.05.021
31. Leung M, Cheung C, Yu K, Yip B, Sham P, Li Q, et al. Gray matter in first-episode schizophrenia before and after antipsychotic drug treatment. anatomical likelihood estimation meta-analyses with sample size weighting. Schizophr Bull. (2011) 37:199–211. doi: 10.1093/schbul/sbp099
32. Cierpka M, Wolf N, Kubera K, Schmitgen M, Vasic N, Frasch K, et al. Cerebellar contributions to persistent auditory verbal hallucinations in patients with schizophrenia. Cerebellum. (2017) 16:964–72.
33. Morimoto C, Uematsu A, Nakatani H, Takano Y, Iwashiro N, Abe O, et al. Volumetric differences in gray and white matter of cerebellar crus i/ii across the different clinical stages of schizophrenia. Psychiatry Clin Neurosci. (2021) 75:256–64. doi: 10.1111/pcn.13277
34. Moussa-Tooks A, Rogers B, Huang A, Sheffield J, Heckers S, Woodward N. Cerebellar structure and cognitive ability in psychosis. Biol Psychiatry. (2022) 2022:13. doi: 10.1016/j.biopsych.2022.03.013
35. Moberget T, Doan N, Alnaes D, Kaufmann T, Cordova-Palomera A, Lagerberg T, et al. Cerebellar volume and cerebellocerebral structural covariance in schizophrenia: a multisite mega-analysis of 983 patients and 1349 healthy controls. Mol Psychiatry. (2018) 23:1512–20. doi: 10.1038/mp.2017.106
36. Ding Y, Ou Y, Pan P, Shan X, Chen J, Liu F, et al. Cerebellar structural and functional abnormalities in first-episode and drug-naive patients with schizophrenia: a meta-analysis. Psychiatry Res Neuro. (2019) 283:24–33. doi: 10.1016/j.pscychresns.2018.11.009
37. Li Q, Zhao Y, Chen Z, Long J, Dai J, Huang X, et al. Meta-analysis of cortical thickness abnormalities in medication-free patients with major depressive disorder. Neuropsychopharmacology. (2020) 45:703–12. doi: 10.1038/s41386-019-0563-9
38. Gao X, Zhang W, Yao L, Xiao Y, Liu L, Liu J, et al. Association between structural and functional brain alterations in drug-free patients with schizophrenia: a multimodal meta-analysis. J Psychiatry Neurosci. (2017) 43:1–12. doi: 10.1503/jpn.160219
39. Radua J, Mataix-Cols D. Meta-analytic methods for neuroimaging data explained. Biol Mood Anxiety Disord. (2012) 2:6. doi: 10.1186/2045-5380-2-6
40. Müller V, Cieslik E, Laird A, Fox P, Radua J, Mataix-Cols D, et al. Ten simple rules for neuroimaging meta-analysis. Neurosci Biobehav Rev. (2018) 84:151–61. doi: 10.1016/j.neubiorev.2017.11.012
41. Wei X, Luo C, Li Q, Hu N, Xiao Y, Liu N, et al. White matter abnormalities in patients with parkinson’s disease: a meta-analysis of diffusion tensor imaging using tract-based spatial statistics. Front Aging Neurosci. (2020) 12:610962. doi: 10.3389/fnagi.2020.610962
42. Yang C, Li L, Hu X, Luo Q, Kuang W, Lui S, et al. Psychoradiologic abnormalities of white matter in patients with bipolar disorder: diffusion tensor imaging studies using tract-based spatial statistics. J Psychiatry Neurosci. (2019) 44:32–44. doi: 10.1503/jpn.170221
43. Chen L, Hu X, Ouyang L, He N, Liao Y, Liu Q, et al. A systematic review and meta-analysis of tract-based spatial statistics studies regarding attention-deficit/hyperactivity disorder. Neurosci Biobehav Rev. (2016) 68:838–47. doi: 10.1016/j.neubiorev.2016.07.022
44. Delvecchio G, Lorandi A, Perlini C, Barillari M, Ruggeri M, Altamura A, et al. Brain anatomy of symptom stratification in schizophrenia: a voxel-based morphometry study. Nordic J Psychiatry. (2017) 71:348–54.
45. Filippi M, Canu E, Gasparotti R, Agosta F, Valsecchi P, Lodoli G, et al. Patterns of brain structural changes in first-contact, antipsychotic drug-naive patients with schizophrenia. AJNR Am J Neuroradiol. (2014) 35:30–7. doi: 10.3174/ajnr.A3583
46. Ha T, Youn T, Ha K, Rho K, Lee J, Kim I, et al. Gray matter abnormalities in paranoid schizophrenia and their clinical correlations. Psychiatry Res Neuro. (2004) 132:251–60.
47. Huang P, Xi Y, Lu Z, Chen Y, Li X, Li W, et al. Decreased bilateral thalamic gray matter volume in first-episode schizophrenia with prominent hallucinatory symptoms: a volumetric mri study. Sci Rep. (2015) 5:14505. doi: 10.1038/srep14505
48. Jayakumar P, Venkatasubramanian G, Gangadhar B, Janakiramaiah N, Keshavan M. Optimized voxel-based morphometry of gray matter volume in first-episode, antipsychotic-naive schizophrenia. Prog Neuro Psychopharmacol Biol Psychiatry. (2005) 29:587–91.
49. Kuehn S, Romanowski A, Schubert F, Gallinat J. Reduction of cerebellar grey matter in crus I and II in schizophrenia. Brain Struct Funct. (2012) 217: 523–9.
50. Lei W, Deng W, Li M, He Z, Han Y, Huang C, et al. Gray matter volume alterations in first-episode drug-naïve patients with deficit and nondeficit schizophrenia. Psychiatry Res. (2015) 234:219–26. doi: 10.1016/j.pscychresns.2015.09.015
51. McDonald C, Bullmore E, Sham P, Chitnis X, Suckling J, MacCabe J, et al. Regional volume deviations of brain structure in schizophrenia and psychotic bipolar disorder: computational morphometry study. Br J Psychiatry. (2005) 186:369–77. doi: 10.1192/bjp.186.5.369
52. Molina V, Galindo G, Cortes B, De Herrera A, Ledo A, Sanz J, et al. Different gray matter patterns in chronic schizophrenia and chronic bipolar disorder patients identified using voxel-based morphometry. Eur Arch Psychiatry Clin Neurosci. (2011) 261:313–22. doi: 10.1007/s00406-010-0183-1
53. Molina V, Martín C, Ballesteros A, de Herrera A, Hernández-Tamames J. Optimized voxel brain morphometry: association between brain volumes and the response to atypical antipsychotics. Eur Arch Psychiatry Clin Neurosci. (2011) 261:407–16. doi: 10.1007/s00406-010-0182-2
54. Nenadic I, Sauer H, Smesny S, Gaser C. Aging effects on regional brain structural changes in schizophrenia. Schizophr Bull. (2012) 38: 838–44.
55. Salgado-Pineda P, Baeza I, Pérez-Gómez M, Vendrell P, Junqué C, Bargalló N, et al. Sustained attention impairment correlates to gray matter decreases in first episode neuroleptic-naive schizophrenic patients. Neuroimage. (2003) 19:365–75. doi: 10.1016/s1053-811900094-6
56. Segarra N, Bernardo M, Valdes M, Caldu X, Falcón C, Rami L, et al. Cerebellar deficits in schizophrenia are associated with executive dysfunction. Neuroreport. (2008) 19:1513–7. doi: 10.1097/WNR.0b013e3283108bd8
57. Spalthoff R, Gaser C, Nenadic I. Altered gyrification in schizophrenia and its relation to other morphometric markers. Schizophr Res. (2018) 202:195–202. doi: 10.1016/j.schres.2018.07.014
58. Suzuki M, Nohara S, Hagino H, Kurokawa K, Yotsutsuji T, Kawasaki Y, et al. Regional changes in brain gray and white matter in patients with schizophrenia demonstrated with voxel-based analysis of Mri. Schizophr Res. (2002) 55:41–54.
59. Venkatasubramanian G. Neuroanatomical correlates of psychopathology in antipsychotic-naive schizophrenia. Indian J Psychiatry. (2010) 52:28–36. doi: 10.4103/0019-5545.58892
60. Watson D, Anderson J, Bai F, Barrett S, McGinnity T, Mulholland C, et al. A voxel based morphometry study investigating brain structural changes in first episode psychosis. Behav Brain Res. (2012) 227:91–9.
61. Whitford T, Farrow T, Gomes L, Brennan J, Harris A, Williams L. Grey matter deficits and symptom profile in first episode schizophrenia. Psychiatry Res. (2005) 139:229–38. doi: 10.1016/j.pscychresns.2005.05.010
62. Wilke M, Kaufmann C, Grabner A, Putz B, Wetter T, Auer D. Gray matter-changes and correlates of disease severity in schizophrenia: a statistical parametric mapping study. NeuroImage. (2001) 13:814–24. doi: 10.1006/nimg.2001.0751
63. Yang Y, Li X, Cui Y, Liu K, Qu H, Lu Y, et al. Reduced gray matter volume in orbitofrontal cortex across schizophrenia, major depressive disorder, and bipolar disorder: a comparative imaging study. Front Neurosci. (2022) 16:919272. doi: 10.3389/fnins.2022.919272
64. Zhang X, Yao J, Lv Y, Zhao X, Li Y, Sui Y, et al. An association study on the cognitive function and the cerebral grey matter volume of patients with first-episode schizophrenia. Shanghai Arch Psychiatry. (2018) 30:154–67. doi: 10.11919/j.issn.1002-0829.217138
65. Joyal C, Pennanen C, Tiihonen E, Laakso M, Tiihonen J, Aronen H. Mri volumetry of the vermis and the cerebellar hemispheres in men with schizophrenia. Psychiatry Res. (2004) 131:115–24. doi: 10.1016/j.pscychresns.2003.09.003
66. Laidi C, Hajek T, Spaniel F, Kolenic M, d’Albis M, Sarrazin S, et al. Cerebellar parcellation in schizophrenia and bipolar disorder. Acta Psychiatr Scand. (2019) 140:468–76. doi: 10.1111/acps.13087
67. Stoodley C, Schmahmann J. Functional topography in the human cerebellum: a meta-analysis of neuroimaging studies. Neuroimage. (2009) 44:489–501. doi: 10.1016/j.neuroimage.2008.08.039
68. Haijma S, Van Haren N, Cahn W, Koolschijn P, Hulshoff Pol H, Kahn R. Brain volumes in schizophrenia: a meta-analysis in over 18 000 subjects. Schizophr Bull. (2013) 39:1129–38. doi: 10.1093/schbul/sbs118
69. Zhu J, Wang H, Chen Y, Yan L, Han Y, Liu L, et al. The joubert syndrome gene arl13b is critical for early cerebellar development in zebrafish. Neurosci Bull. (2020) 36:1023–34. doi: 10.1007/s12264-020-00554-y
70. Wang M, Ye R, Barron E, Baumeister P, Mao C, Luo S, et al. Essential role of the unfolded protein response regulator grp78/bip in protection from neuronal apoptosis. Cell Death Differ. (2010) 17:488–98. doi: 10.1038/cdd.2009.144
71. Sillitoe R, Joyner A. Morphology, molecular codes, and circuitry produce the three-dimensional complexity of the cerebellum. Ann Rev Cell Dev Biol. (2007) 23:549–77. doi: 10.1146/annurev.cellbio.23.090506.123237
72. Maloku E, Covelo I, Hanbauer I, Guidotti A, Kadriu B, Hu Q, et al. Lower number of cerebellar purkinje neurons in psychosis is associated with reduced reelin expression. Proc Natl Acad Sci USA. (2010) 107:4407–11. doi: 10.1073/pnas.0914483107
73. Tran K, Smutzer G, Doty R, Arnold S. Reduced purkinje cell size in the cerebellar vermis of elderly patients with schizophrenia. Am J Psychiatry. (1998) 155:1288–90. doi: 10.1176/ajp.155.9.1288
74. Andersen B, Pakkenberg B. Stereological quantitation in cerebella from people with schizophrenia. Br J Psychiatry. (2003) 182:354–61. doi: 10.1192/bjp.182.4.354
75. MacKenzie-Graham A, Tiwari-Woodruff S, Sharma G, Aguilar C, Vo K, Strickland L, et al. Purkinje cell loss in experimental autoimmune encephalomyelitis. Neuroimage. (2009) 48:637–51. doi: 10.1016/j.neuroimage.2009.06.073
76. D’Mello A, Stoodley C. Cerebro-cerebellar circuits in autism spectrum disorder. Front Neurosci. (2015) 9:408. doi: 10.3389/fnins.2015.00408
77. Van Overwalle F, Ma Q, Heleven E. The posterior crus II cerebellum is specialized for social mentalizing and emotional self-experiences: a meta-analysis. Soc Cogn Affect Neurosci. (2020) 15:905–28. doi: 10.1093/scan/nsaa124
78. Van Overwalle F, Manto M, Cattaneo Z, Clausi S, Ferrari C, Gabrieli J, et al. Consensus paper: cerebellum and social cognition. Cerebellum. (2020) 19:833–68. doi: 10.1007/s12311-020-01155-1
79. Nakatani H, Nakamura Y, Okanoya K. Respective involvement of the right cerebellar crus i and ii in syntactic and semantic processing for comprehension of language. Cerebellum. (2022) 2022:1451. doi: 10.1007/s12311-022-01451-y
80. Stoodley C, Valera E, Schmahmann J. An fmri study of intra-individual functional topography in the human cerebellum. Behav Neurol. (2010) 23:65–79. doi: 10.3233/ben-2010-0268
81. Olivito G, Lupo M, Iacobacci C, Clausi S, Romano S, Masciullo M, et al. Structural cerebellar correlates of cognitive functions in spinocerebellar ataxia type 2. J Neurol. (2018) 265:597–606. doi: 10.1007/s00415-018-8738-6
82. Pierce J, Thomasson M, Voruz P, Selosse G, Péron J. Explicit and implicit emotion processing in the cerebellum: a meta-analysis and systematic review. Cerebellum. (2022) 2022:1459. doi: 10.1007/s12311-022-01459-4
83. Guell X, Schmahmann J. Cerebellar functional anatomy: a didactic summary based on human fmri evidence. Cerebellum. (2020) 19:1–5. doi: 10.1007/s12311-019-01083-9
84. Kawabata K, Bagarinao E, Watanabe H, Maesawa S, Mori D, Hara K, et al. Functional connector hubs in the cerebellum. Neuroimage. (2022) 257:119263. doi: 10.1016/j.neuroimage.2022.119263
85. Anticevic A, Haut K, Murray J, Repovs G, Yang G, Diehl C, et al. Association of thalamic dysconnectivity and conversion to psychosis in youth and young adults at elevated clinical risk. JAMA Psychiatry. (2015) 72:882–91. doi: 10.1001/jamapsychiatry.2015.0566
86. Kim D, Moussa-Tooks A, Bolbecker A, Apthorp D, Newman S, O’Donnell B, et al. Cerebellar-cortical dysconnectivity in resting-state associated with sensorimotor tasks in schizophrenia. Hum Brain Mapp. (2020) 41:3119–32. doi: 10.1002/hbm.25002
87. Shinn A, Baker J, Lewandowski K, Öngür D, Cohen B. Aberrant cerebellar connectivity in motor and association networks in schizophrenia. Front Hum Neurosci. (2015) 9:134. doi: 10.3389/fnhum.2015.00134
88. Picard H, Amado I, Mouchet-Mages S, Olie J, Krebs M. The role of the cerebellum in schizophrenia: an update of clinical, cognitive, and functional evidences. Schizophr Bul. (2008) 34:155–72. doi: 10.1093/schbul/sbm049
89. Halko M, Farzan F, Eldaief M, Schmahmann J, Pascual-Leone A. Intermittent theta-burst stimulation of the lateral cerebellum increases functional connectivity of the default network. J Neurosci. (2014) 34:12049–56. doi: 10.1523/jneurosci.1776-14.2014
90. Gallardo-Ruiz R, Crespo-Facorro B, Setién-Suero E, Tordesillas-Gutierrez D. Long-term grey matter changes in first episode psychosis: a systematic review. Psychiatry Investig. (2019) 16:336–45. doi: 10.30773/pi.2019.02.10.1
91. Zhang W, Deng W, Yao L, Xiao Y, Li F, Liu J, et al. Brain structural abnormalities in a group of never-medicated patients with long-term schizophrenia. Am J Psychiatry. (2015) 172:995–1003. doi: 10.1176/appi.ajp.2015.14091108
92. Jiang Y, Luo C, Li X, Duan M, He H, Chen X, et al. Progressive reduction in gray matter in patients with schizophrenia assessed with mr imaging by using causal network analysis. Radiology. (2018) 287:633–42. doi: 10.1148/radiol.2017171832
93. Tordesillas-Gutierrez D, Koutsouleris N, Roiz-Santiañez R, Meisenzahl E, Ayesa-Arriola R, Marco de Lucas E, et al. Grey matter volume differences in non-affective psychosis and the effects of age of onset on grey matter volumes: a voxelwise study. Schizophr Res. (2015) 164:74–82. doi: 10.1016/j.schres.2015.01.032
94. Yue Y, Kong L, Wang J, Li C, Tan L, Su H, et al. Regional abnormality of grey matter in schizophrenia: effect from the illness or treatment? PLoS One. (2016) 11:e0147204. doi: 10.1371/journal.pone.0147204
Keywords: cerebellum, schizophrenia, magnetic resonance imaging, cognition, gray matter volume
Citation: Li X, Liu N, Yang C, Zhang W and Lui S (2022) Cerebellar gray matter volume changes in patients with schizophrenia: A voxel-based meta-analysis. Front. Psychiatry 13:1083480. doi: 10.3389/fpsyt.2022.1083480
Received: 29 October 2022; Accepted: 28 November 2022;
Published: 22 December 2022.
Edited by:
Hengyi Cao, Feinstein Institute for Medical Research, United StatesReviewed by:
Sheeba Arnold, Carle Foundation Hospital, United StatesCopyright © 2022 Li, Liu, Yang, Zhang and Lui. This is an open-access article distributed under the terms of the Creative Commons Attribution License (CC BY). The use, distribution or reproduction in other forums is permitted, provided the original author(s) and the copyright owner(s) are credited and that the original publication in this journal is cited, in accordance with accepted academic practice. No use, distribution or reproduction is permitted which does not comply with these terms.
*Correspondence: Wenjing Zhang, ✉ d2p6aGFuZzE5QHNjdS5lZHUuY24=; Su Lui, ✉ bHVzdXdjdW1zQHRvbS5jb20=
†These authors have contributed equally to this work
Disclaimer: All claims expressed in this article are solely those of the authors and do not necessarily represent those of their affiliated organizations, or those of the publisher, the editors and the reviewers. Any product that may be evaluated in this article or claim that may be made by its manufacturer is not guaranteed or endorsed by the publisher.
Research integrity at Frontiers
Learn more about the work of our research integrity team to safeguard the quality of each article we publish.