- Department of Psychiatry, Jikei University School of Medicine, Tokyo, Japan
Despite the high prevalence and clinical importance of comorbid psychosis in epilepsy, its neurobiological mechanisms remain understudied. This narrative mini-review aims to provide an overview of recent updates in in vivo neuroimaging studies on psychosis in epilepsy, including structural and diffusion magnetic resonance imaging (MRI) and functional and molecular imaging, and to discuss future directions in this field. While the conventional morphological analysis of structural MRI has provided relatively inconsistent results, advanced methods, including brain network analysis, hippocampal subregion volumetry, and machine learning models, have recently provided novel findings. Diffusion MRI, for example, has revealed a reduction in white matter integrity mainly in the frontal and temporal lobes, as well as a disruption of brain white matter networks. Functional neuroimaging, such as perfusion single-photon emission computed tomography (SPECT) or fluorodeoxyglucose positron emission tomography (FDG-PET), often identifies hyperactivity in various brain regions. The current limitations of these more recent studies may include small and sometimes heterogeneous samples, insufficient control groups, the effects of psychoactive drugs, and the lack of longitudinal analysis. Further investigations are required to establish novel treatments and identify clinical diagnostic or disease-monitoring biomarkers in psychosis in epilepsy.
Introduction
It is known that patients with epilepsy suffer from psychiatric comorbidities with a 35% life-time prevalence (1). This occurrence is more frequent than in the general population (1), and can greatly affect quality of life of patients and their caregivers (2, 3). In addition, psychiatric symptoms sometimes occur prior to the onset of seizures and correlate with seizure outcomes (4); currently, a bidirectional relationship between epilepsy and psychiatric comorbidities is assumed. Both psychiatric disorders and epilepsy occur in the brain, and it is therefore possible that epilepsy-related changes in the brain may be associated with the development and manifestation of psychiatric symptoms. Although past studies have focused on endocrinology, neurotransmitters, brain structures, and immunology (4), the neurobiological mechanisms of psychiatric comorbidity in epilepsy remain to be elucidated.
Psychosis is a serious psychiatric condition characterized by hallucinations, delusions, and bizarre or disorganized behaviors (5). The odds ratio for psychosis in people with epilepsy is 7.8 times higher than in the general population (6), and historically, psychosis has received attention in terms of forced normalization or alternative psychosis, i.e., psychosis associated with a reduction in epileptiform discharges or seizures (5, 7). Temporal lobe epilepsy (TLE) has a greater prevalence (up to 20%) of psychosis, particularly postictal psychosis (8), while the remaining >80% of patients do not develop psychosis. Considering these epidemiological studies, it is reasonable to conclude that some neural mechanisms in epilepsy and seizure, especially the limbic circuit in TLE, may potentially be involved in the development of psychosis. Such bidirectional neurobiological mechanisms should also be supported by the evidence of beneficial effect of electroconvulsive therapy (ECT) on psychotic disorders (9). However, the neural basis of psychosis in epilepsy remains unknown and requires more investigation, particularly regarding what mechanisms underlie psychosis in epilepsy and whether they are different from those in the general population (e.g., schizophrenia).
Neuroimaging is a powerful tool that can investigate human brains non-invasively, and it has frequently been applied to investigations on various neuropsychiatric disorders (10). In fact, several past neuroimaging studies have reported various and varied findings in psychosis in epilepsy, with diverse and sometimes inconsistent results (11). More recently, advanced neuroimaging methods, such as brain network analysis, machine learning, and hippocampal subfields, have been applied and have provided novel findings.
The aim of this narrative review is to provide an overview of recent updates in in vivo neuroimaging studies on psychosis in epilepsy, including structural and diffusion magnetic resonance imaging (MRI), and functional and molecular imaging, and to discuss future directions in this field. In addition to neuroscientific progress, a better understanding of the neurobiological aspects of psychosis in epilepsy may potentially lead to novel treatments as well as to the identification of clinical diagnostic or disease-monitoring biomarkers. A search of literature was conducted in PubMed database on October 1, 2022 using “psychosis,” “epilepsy,” “MRI,” “PET,” and/or “SPECT” as key words, and relevant studies were manually selected, although no rigorous systematic selection criteria was adopted for this narrative review.
Structural magnetic resonance imaging of psychosis in epilepsy
Earlier studies on brain morphological changes in psychosis in epilepsy focused primarily on mesial temporal lobe structures, such as the hippocampus and amygdala, using manual tracing methods. The hippocampal and amygdala findings of psychosis in epilepsy in these studies were diverse, including bilateral volume loss of the hippocampus and amygdala (12), no difference in total hippocampal volumes (13), left hippocampal volume reduction (14), widespread gray matter volume reduction (15), and bilateral amygdala enlargement (16).
Along with the development of automated whole brain MRI analysis, such as voxel-based morphometry (17) or surface-based morphometry (18), trends in structural neuroimaging studies on psychosis in epilepsy have also changed. Since 2004, several automated brain morphometric studies have reported a variety of results in psychosis in epilepsy, such as bilaterally widespread gray and white matter reduction (19), increased and decreased cortical thickness (20), cortical thinning in the inferior frontal gyrus (21), gray matter reduction in the left parietal lobe (22), and no significant effect of psychosis on brain morphological changes (23–25) (Figure 1A).
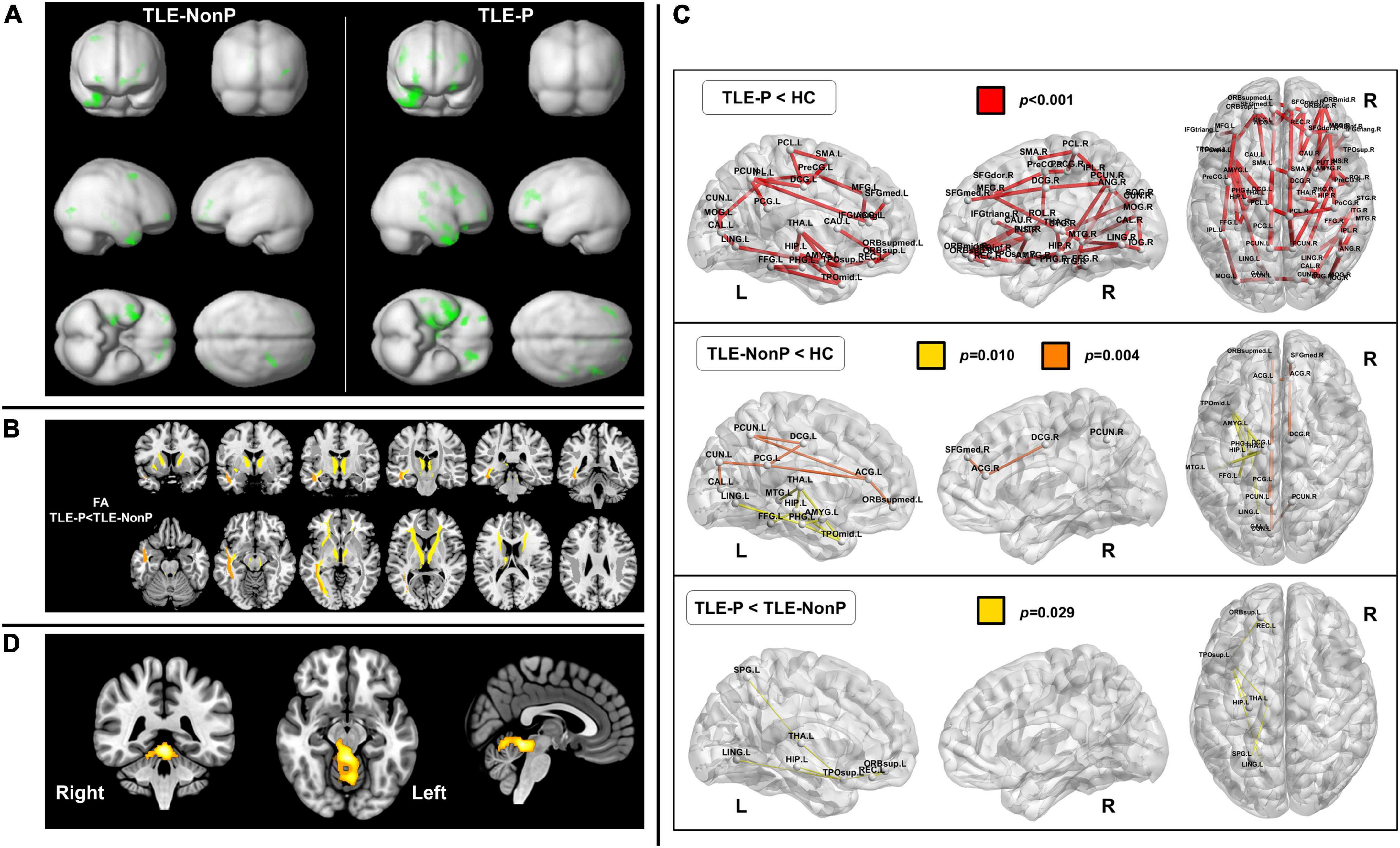
Figure 1. Neuroimaging findings in psychosis in epilepsy. (A) Gray matter reduction in temporal lobe epilepsy (TLE) with and without psychosis (TLE-P and TLE-NonP) compared with healthy controls, derived from structural magnetic resonance imaging (MRI). Both TLE-P and TLE-NonP showed significant volume reduction in the ipsilateral temporal and other brain areas, but there was no significant direct difference between them [Cited from Sone et al. (25)]. (B) Significant reduction in fractional anisotropy (FA) within the white matter tracts in TLE-P, derived from diffusion MRI [Cited from Sone et al. (40)]. (C) Disrupted brain white matter networks in TLE-P and TLE-NonP, derived from diffusion MRI [Cited from Sone et al. (40)]. (D) Hypermetabolic areas in TLE-P compared to TLE-NonP, derived from fluorodeoxyglucose positron emission tomography (FDG-PET) [Cited from Sone et al. (48)].
While automated brain morphometry is suitable for whole-brain analysis, and is fully reproducible and more efficient in terms of time and effort, manual tracing is less error-prone and more accurate when rigorously applied. However, in the literature to date, neither automated nor manual methodologies have succeeded in providing consistent results, and thus simple brain morphology may be insufficient to reveal the neural mechanisms of psychosis in epilepsy.
Recently, several advanced analytical methods for neuroimaging have emerged and are expected to provide further evidence on epilepsy. These include brain network analysis (26), machine learning (27), and hippocampal subfield analysis (28). Epilepsy-related alterations are usually distributed across multiple brain regions beyond a single focus, e.g., the hippocampus in TLE, and thus epilepsy is currently considered a network-level disorder (26). As this concept of network neuroscience could be applied to psychiatric disorders (29), psychosis in epilepsy may also benefit from brain network analysis. In a previous study using graph theoretical analysis based on gray matter anatomical covariance network (25), TLE with psychosis showed a significantly higher characteristic path length and transitivity, and lower global efficiency, as well as reduced resilience to attacks, compared to TLE without psychosis. In addition, a recent study identified increased cortical thickness in psychosis in epilepsy, primarily in the cognitive control network and default mode network areas (30).
The hippocampal formation consists of major subfields, i.e., the cornu ammonis, as well as other subregions such as the dentate gyrus and subiculum. These hippocampal subregions have different functions and neural pathways (31). Functional organization along the long axis of the hippocampus is also attracting attention (32). Thus, hippocampal subfield and/or subregion analysis is expected to provide further knowledge about epilepsy, particularly TLE, beyond what can be learned by analyzing the hippocampus as a single region. In psychosis in epilepsy, bilateral posterior hippocampal atrophy has been identified, while the head and body of the hippocampi were not affected (33). According to another recent study, hippocampal subfield volumes in psychosis in epilepsy were not significantly different from those in epilepsy without psychosis, while the hippocampal fissure was significantly enlarged in psychosis in epilepsy (34).
Machine learning analysis has the advantage over conventional methods of accurate, automated, and fast pattern learning and is expected to lead to optimal algorithms for clinical neuropsychiatry and epilepsy (27). One of the emerging trends in machine learning for neuropsychiatry is the brain-age prediction framework, which estimates the age of each individual’s brain image using a machine learning regression model (35, 36). Given the close relationship between aging and neuropsychiatric disorders, brain-age prediction may contribute as a clinically useful, individualized biomarker. In TLE with interictal psychosis, brain age has been found to be higher than chronological age by 10.6 years, and the gap was significantly higher than that in TLE without psychosis (+5.3 years) (37). It is possible that accelerated aging and a brain that appears older may be suggested as potential biomechanisms of psychosis in epilepsy. Thus, several advanced methodologies have been applied to psychosis in epilepsy, and further work may expand our knowledge.
Diffusion magnetic resonance imaging of psychosis in epilepsy
Diffusion tensor imaging (DTI) is sensitive to water diffusion features, and diffusion anisotropy can be used as a marker for brain white matter tract integrity (38). In TLE with interictal psychosis, one study identified significantly lower fractional anisotropy in the bilateral frontal and temporal white matter regions, compared with TLE without psychosis, using manual region-of-interest (ROI) delineation (39). More recently, another study applied whole-brain tract-based analysis of DTI and reported a more widespread reduction in white matter integrity in TLE with interictal psychosis, and in particular, the anterior thalamic radiation, inferior fronto-occipital fasciculus, and inferior longitudinal fasciculus were damaged compared with TLE without psychosis (40) (Figure 1B), which seems consistent with the previous study (39). In addition, white matter structural network analysis revealed significantly reduced global and local efficiency as well as network abnormalities involving the left limbic and prefrontal areas in TLE with interictal psychosis (40) (Figure 1C). While DTI studies on psychosis in epilepsy remain scarce, their results are expected to be more consistent than those of structural MRI studies.
Functional neuroimaging of psychosis in epilepsy
Most functional neuroimaging studies on psychosis in epilepsy have utilized single-photon emission computed tomography (SPECT) to measure cerebral blood flow. Interictally, the reported findings range from hypoperfusion in the left superior temporal gyrus (41) to no significant differences (42). During the psychotic period, hyperperfusion findings have been reported in the lateral temporal lobe (43), fronto-temporal lobes (44, 45), and right temporal lobe (46). 18F-fluorodeoxyglucose positron emission tomography (FDG-PET) is also widely used in clinical practice in cases of epilepsy (47), but FDG-PET studies on psychosis in epilepsy are more limited compared with perfusion SPECT studies. A recent study found brain glucose hypermetabolism in the upper cerebellum, superior cerebellar peduncle, and midbrain (48) (Figure 1D), which might be associated with cerebellar involvement in cognition and emotion (49, 50). Thus, most functional neuroimaging studies have suggested brain hyperactivity in psychosis in epilepsy, while the reported abnormal areas are diverse. Apart from nuclear imaging, reduced N-acetyl aspartate (NAA) within the hippocampi has been reported in psychosis in epilepsy (12). On the other hand, the lack of functional MRI studies may be notable, given the abundant applications to psychiatric disorders (51). While FDG-PET and perfusion SPECT are widely used in clinical practice for epilepsy (52), the clinical use of functional MRI is still limited, which might be a cause of the discrepancy. At any rate, considering the advantage for non-invasive evaluation of dynamic brain activity, future studies on functional MRI findings in psychosis of epilepsy should be desirable.
Future directions
Compared with the substantial neuroimaging evidence regarding pure psychiatric disorders and common types of epilepsies, psychosis in epilepsy is distinctly understudied. Additionally, the cohorts in these studies are small (up to N = 30) and sometimes heterogeneous in terms of type of epilepsy and type of psychosis, e.g., mixed cohorts with postictal and interictal psychoses. Furthermore, most studies do not include a control cohort of psychosis without epilepsy, e.g., schizophrenia. To reveal the neurobiology of psychosis in epilepsy, it is desirable to compare psychosis in epilepsy with epilepsy without psychosis, psychosis without epilepsy, and healthy subjects. Moreover, most patients in these studies take antipsychotic medications, and thus the effects of drugs must be taken into consideration. Further longitudinal investigation on drug-naïve cases from the early stage of psychosis may address such problems and could lead to more useful imaging biomarkers for prediction and disease-monitoring. Finally, further evidence may be provided by other advanced imaging techniques that have not yet been used to study psychosis in epilepsy, such as functional MRI, multi-shell diffusion MRI, or specific PET tracers.
Conclusion
There have been efforts to reveal the neurobiological mechanisms of psychosis in epilepsy using structural and functional neuroimaging. Though results from structural MRI are relatively inconsistent, advanced imaging techniques are now being applied and provide further knowledge on this condition. Psychosis in epilepsy remains understudied, however, and additional research is required in order to address the current issues.
Author contributions
The author confirms being the sole contributor of this work and has approved it for publication.
Funding
This study was supported by grants from the Japan Society for the Promotion of Science (KAKENHI; No. JP21K15720), the Japan Epilepsy Research Foundation (JERF TENKAN 22007), and The Uehara Memorial Foundation (all to DS).
Conflict of interest
The author declares that the research was conducted in the absence of any commercial or financial relationships that could be construed as a potential conflict of interest.
Publisher’s note
All claims expressed in this article are solely those of the authors and do not necessarily represent those of their affiliated organizations, or those of the publisher, the editors and the reviewers. Any product that may be evaluated in this article, or claim that may be made by its manufacturer, is not guaranteed or endorsed by the publisher.
References
1. Tellez-Zenteno JF, Patten SB, Jette N, Williams J, Wiebe S. Psychiatric comorbidity in epilepsy: a population-based analysis. Epilepsia. (2007) 48:2336–44. doi: 10.1111/j.1528-1167.2007.01222.x
2. Gilliam F, Hecimovic H, Sheline Y. Psychiatric comorbidity, health, and function in epilepsy. Epilepsy Behav. (2003) 4(Suppl 4):S26–30.
3. Vinti V, Dell’Isola GB, Tascini G, Mencaroni E, Cara GD, Striano P, et al. Temporal lobe epilepsy and psychiatric comorbidity. Front Neurol. (2021) 12:775781. doi: 10.3389/fneur.2021.775781
4. Kanner AM. Can neurobiological pathogenic mechanisms of depression facilitate the development of seizure disorders? Lancet Neurol. (2012) 11:1093–102. doi: 10.1016/S1474-4422(12)70201-6
5. Nadkarni S, Arnedo V, Devinsky O. Psychosis in epilepsy patients. Epilepsia. (2007) 48(Suppl 9):17–9. doi: 10.1111/j.1528-1167.2007.01394.x
6. Clancy MJ, Clarke MC, Connor DJ, Cannon M, Cotter DR. The prevalence of psychosis in epilepsy; a systematic review and meta-analysis. BMC Psychiatry. (2014) 14:75. doi: 10.1186/1471-244X-14-75
7. Kanner AM, Rivas-Grajales AM. Psychosis of epilepsy: a multifaceted neuropsychiatric disorder. CNS Spectr. (2016) 21:247–57. doi: 10.1017/S1092852916000250
8. Gaitatzis A, Trimble MR, Sander JW. The psychiatric comorbidity of epilepsy. Acta Neurol Scand. (2004) 110:207–20. doi: 10.1111/j.1600-0404.2004.00324.x
9. Tor PC, Tan XW, Martin D, Loo C. Comparative outcomes in electroconvulsive therapy (Ect): a naturalistic comparison between outcomes in psychosis, mania, depression, psychotic depression and catatonia. Eur Neuropsychopharmacol. (2021) 51:43–54. doi: 10.1016/j.euroneuro.2021.04.023
10. Linden D, Thome J. Modern neuroimaging in psychiatry: towards the integration of functional and molecular information. World J Biol Psychiatry. (2011) 12(Suppl 1):6–10. doi: 10.3109/15622975.2011.598713
11. Allebone J, Kanaan R, Wilson SJ. Systematic review of structural and functional brain alterations in psychosis of epilepsy. J Neurol Neurosurg Psychiatry. (2018) 89:611–7. doi: 10.1136/jnnp-2017-317102
12. Maier M, Mellers J, Toone B, Trimble M, Ron MA. Schizophrenia, temporal lobe epilepsy and psychosis: an in vivo magnetic resonance spectroscopy and imaging study of the hippocampus/amygdala complex. Psychol Med. (2000) 30:571–81. doi: 10.1017/s0033291799001993
13. Briellmann RS, Kalnins RM, Hopwood MJ, Ward C, Berkovic SF, Jackson GD. Tle patients with postictal psychosis: mesial dysplasia and anterior hippocampal preservation. Neurology. (2000) 55:1027–30. doi: 10.1212/wnl.55.7.1027
14. Marchetti RL, Azevedo D Jr., de Campos Bottino CM, Kurcgant D, de Fatima Horvath Marques A, Marie SK, et al. Volumetric evidence of a left laterality effect in epileptic psychosis. Epilepsy Behav. (2003) 4:234–40. doi: 10.1016/s1525-5050(03)00056-8
15. Marsh L, Sullivan EV, Morrell M, Lim KO, Pfefferbaum A. Structural brain abnormalities in patients with schizophrenia, epilepsy, and epilepsy with chronic interictal psychosis. Psychiatry Res. (2001) 108:1–15. doi: 10.1016/s0925-4927(01)00115-9
16. Tebartz Van Elst L, Baeumer D, Lemieux L, Woermann FG, Koepp M, Krishnamoorthy S, et al. Amygdala pathology in psychosis of epilepsy: a magnetic resonance imaging study in patients with temporal lobe epilepsy. Brain. (2002) 125(Pt 1):140–9.
17. Ashburner J, Friston KJ. Voxel-based morphometry–the methods. NeuroImage. (2000) 11(6 Pt 1):805–21. doi: 10.1006/nimg.2000.0582
19. Sundram F, Cannon M, Doherty CP, Barker GJ, Fitzsimons M, Delanty N, et al. Neuroanatomical correlates of psychosis in temporal lobe epilepsy: voxel-based morphometry study. Br J Psychiatry. (2010) 197:482–92. doi: 10.1192/bjp.bp.110.080218
20. DuBois JM, Devinsky O, Carlson C, Kuzniecky R, Quinn BT, Alper K, et al. Abnormalities of cortical thickness in postictal psychosis. Epilepsy Behav. (2011) 21:132–6. doi: 10.1016/j.yebeh.2011.03.024
21. Gutierrez-Galve L, Flugel D, Thompson PJ, Koepp MJ, Symms MR, Ron MA, et al. Cortical abnormalities and their cognitive correlates in patients with temporal lobe epilepsy and interictal psychosis. Epilepsia. (2012) 53:1077–87. doi: 10.1111/j.1528-1167.2012.03504.x
22. Hirakawa N, Kuga H, Hirano Y, Sato J, Oribe N, Nakamura I, et al. Neuroanatomical substrate of chronic psychosis in epilepsy: an MRI study. Brain Imaging Behav. (2020) 14:1382–7. doi: 10.1007/s11682-019-00044-4
23. Rusch N, Tebartz van Elst L, Baeumer D, Ebert D, Trimble MR. Absence of cortical gray matter abnormalities in psychosis of epilepsy: a voxel-based Mri study in patients with temporal lobe epilepsy. J Neuropsychiatry Clin Neurosci. (2004) 16:148–55. doi: 10.1176/jnp.16.2.148
24. Flugel D, Cercignani M, Symms MR, Koepp MJ, Foong JA. Magnetization transfer imaging study in patients with temporal lobe epilepsy and interictal psychosis. Biol Psychiatry. (2006) 59:560–7. doi: 10.1016/j.biopsych.2005.07.023
25. Sone D, Matsuda H, Ota M, Maikusa N, Kimura Y, Sumida K, et al. Graph theoretical analysis of structural neuroimaging in temporal lobe epilepsy with and without psychosis. PLoS One. (2016) 11:e0158728. doi: 10.1371/journal.pone.0158728
26. Bernhardt BC, Bonilha L, Gross DW. Network analysis for a network disorder: the emerging role of graph theory in the study of epilepsy. Epilepsy Behav. (2015) 50:162–70. doi: 10.1016/j.yebeh.2015.06.005
27. Sone D, Beheshti I. Clinical application of machine learning models for brain imaging in epilepsy: a review. Front Neurosci. (2021) 15:684825. doi: 10.3389/fnins.2021.684825
28. Sone D, Sato N, Maikusa N, Ota M, Sumida K, Yokoyama K, et al. Automated subfield volumetric analysis of hippocampus in temporal lobe epilepsy using high-resolution T2-weighed Mr imaging. NeuroImage Clin. (2016) 12:57–64. doi: 10.1016/j.nicl.2016.06.008
29. Bassett DS, Xia CH, Satterthwaite TD. Understanding the emergence of neuropsychiatric disorders with network neuroscience. Biol Psychiatry Cogn Neurosci Neuroimaging. (2018) 3:742–53. doi: 10.1016/j.bpsc.2018.03.015
30. Allebone J, Wilson SJ, Bradlow RCJ, Maller J, O’Brien T, Mullen SA, et al. Increased cortical thickness in nodes of the cognitive control and default mode networks in psychosis of epilepsy. Seizure. (2022) 101:244–52. doi: 10.1016/j.seizure.2022.09.006
31. Schultz C, Engelhardt M. Anatomy of the hippocampal formation. Front Neurol Neurosci. (2014) 34:6–17. doi: 10.1159/000360925
32. Strange BA, Witter MP, Lein ES, Moser EI. Functional organization of the hippocampal longitudinal axis. Nat Rev Neurosci. (2014) 15:655–69. doi: 10.1038/nrn3785
33. Allebone J, Kanaan R, Maller J, O’Brien T, Mullen SA, Cook M, et al. Bilateral volume reduction in posterior hippocampus in psychosis of epilepsy. J Neurol Neurosurg Psychiatry. (2019) 90:688–94. doi: 10.1136/jnnp-2018-319396
34. Allebone J, Kanaan RA, Maller JJ, O’Brien T, Mullen S, Cook M, et al. Enlarged hippocampal fissure in psychosis of epilepsy. Epilepsy Behav. (2020) 111:107290. doi: 10.1016/j.yebeh.2020.107290
35. Franke K, Gaser C. Ten years of brainage as a neuroimaging biomarker of brain aging: what insights have we gained? Front Neurol. (2019) 10:789. doi: 10.3389/fneur.2019.00789
36. Sone D, Beheshti I. Neuroimaging-based brain age estimation: a promising personalized biomarker in neuropsychiatry. J Pers Med. (2022) 12:1850. doi: 10.3390/jpm12111850
37. Sone D, Beheshti I, Maikusa N, Ota M, Kimura Y, Sato N, et al. Neuroimaging-based brain-age prediction in diverse forms of epilepsy: a signature of psychosis and beyond. Mol Psychiatry. (2021) 26:825–34. doi: 10.1038/s41380-019-0446-9
38. Smith SM, Jenkinson M, Johansen-Berg H, Rueckert D, Nichols TE, Mackay CE, et al. Tract-based spatial statistics: voxelwise analysis of multi-subject diffusion data. NeuroImage. (2006) 31:1487–505. doi: 10.1016/j.neuroimage.2006.02.024
39. Flugel D, Cercignani M, Symms MR, O’Toole A, Thompson PJ, Koepp MJ, et al. Diffusion tensor imaging findings and their correlation with neuropsychological deficits in patients with temporal lobe epilepsy and interictal psychosis. Epilepsia. (2006) 47:941–4. doi: 10.1111/j.1528-1167.2006.00527.x
40. Sone D, Sato N, Shigemoto Y, Kimura Y, Maikusa N, Ota M, et al. Disrupted white matter integrity and structural brain networks in temporal lobe epilepsy with and without interictal psychosis. Front Neurol. (2020) 11:556569. doi: 10.3389/fneur.2020.556569
41. Mellers JD, Adachi N, Takei N, Cluckie A, Toone BK, Lishman WA. Spet study of verbal fluency in schizophrenia and epilepsy. Br J Psychiatry. (1998) 173:69–74. doi: 10.1192/bjp.173.1.69
42. Guarnieri R, Wichert-Ana L, Hallak JE, Velasco TR, Walz R, Kato M, et al. Interictal spect in patients with mesial temporal lobe epilepsy and psychosis: a case-control study. Psychiatry Res. (2005) 138:75–84. doi: 10.1016/j.pscychresns.2004.10.003
43. Fong GC, Ho WY, Tsoi TH, Fong KY, Ho SL. Lateral temporal hyperperfusion in postictal psychosis assessed by 99mtc-Hmpao Spect. Neuroimage. (2002) 17:1634–7. doi: 10.1006/nimg.2002.1292
44. Leutmezer F, Podreka I, Asenbaum S, Pietrzyk U, Lucht H, Back C, et al. Postictal psychosis in temporal lobe epilepsy. Epilepsia. (2003) 44:582–90. doi: 10.1046/j.1528-1157.2003.32802.x
45. Nishida T, Kudo T, Inoue Y, Nakamura F, Yoshimura M, Matsuda K, et al. Postictal mania versus postictal psychosis: differences in clinical features, epileptogenic zone, and brain functional changes during postictal period. Epilepsia. (2006) 47:2104–14. doi: 10.1111/j.1528-1167.2006.00893.x
46. Oshima T, Motooka H, Kanemoto K. Spect findings during postictal psychoses: predominance of relative increase of perfusion in right temporal lobe. Epilepsia. (2011) 52:1192–4. doi: 10.1111/j.1528-1167.2010.02962.x
47. Shigemoto Y, Sone D, Kimura Y, Sato N, Matsuda H. Nuclear imaging in epilepsy: principles and progress. Epilepsy Seiz. (2020) 12:40–8. doi: 10.3805/eands.12.40
48. Sone D, Sato N, Shigemoto Y, Kimura Y, Matsuda H. Upper cerebellar glucose hypermetabolism in patients with temporal lobe epilepsy and interictal psychosis. Epilepsia Open. (2022) [Online ahead of print]. doi: 10.1002/epi4.12645
49. Baumann O, Mattingley JB. Functional topography of primary emotion processing in the human cerebellum. Neuroimage. (2012) 61:805–11. doi: 10.1016/j.neuroimage.2012.03.044
50. Schmahmann JD. The cerebellum and cognition. Neurosci Lett. (2019) 688:62–75. doi: 10.1016/j.neulet.2018.07.005
51. Nour MM, Liu Y, Dolan RJ. Functional neuroimaging in psychiatry and the case for failing better. Neuron. (2022) 110:2524–44. doi: 10.1016/j.neuron.2022.07.005
Keywords: psychosis, epilepsy, comorbidity, functional neuroimaging, structural neuroimaging
Citation: Sone D (2022) Neurobiological mechanisms of psychosis in epilepsy: Findings from neuroimaging studies. Front. Psychiatry 13:1079295. doi: 10.3389/fpsyt.2022.1079295
Received: 25 October 2022; Accepted: 08 November 2022;
Published: 23 November 2022.
Edited by:
Luciana D’Alessio, Consejo Nacional de Investigaciones Científicas y Técnicas (CONICET), ArgentinaReviewed by:
Jeroen Antonius Van Waarde, Rijnstate Hospital, NetherlandsCopyright © 2022 Sone. This is an open-access article distributed under the terms of the Creative Commons Attribution License (CC BY). The use, distribution or reproduction in other forums is permitted, provided the original author(s) and the copyright owner(s) are credited and that the original publication in this journal is cited, in accordance with accepted academic practice. No use, distribution or reproduction is permitted which does not comply with these terms.
*Correspondence: Daichi Sone, d-sone@jikei.ac.jp