- 1Department of Magnetic Resonance Imaging, The First Affiliated Hospital of Zhengzhou University, Zhengzhou, China
- 2Key Laboratory for Functional Magnetic Resonance Imaging and Molecular Imaging of Henan Province, Zhengzhou, China
- 3Engineering Technology Research Center for Detection and Application of Brain Function of Henan Province, Zhengzhou, China
- 4Engineering Research Center of Medical Imaging Intelligent Diagnosis and Treatment of Henan Province, Zhengzhou, China
- 5Key Laboratory of Magnetic Resonance and Brain Function of Henan Province, Zhengzhou, China
- 6Key Laboratory of Brain Function and Cognitive Magnetic Resonance Imaging of Zhengzhou, Zhengzhou, China
- 7Key Laboratory of Imaging Intelligence Research Medicine of Henan Province, Zhengzhou, China
Background: A large and growing body of neuroimaging research has concentrated on patients with attention-deficit/hyperactivity disorder (ADHD), but with inconsistent conclusions. This article was intended to investigate the common and certain neural alterations in the structure and function of the brain in patients with ADHD and further explore the differences in brain alterations between adults and children with ADHD.
Methods: We conducted an extensive literature search of whole-brain voxel-based morphometry (VBM) and functional magnetic resonance imaging (fMRI) studies associated with ADHD. Two separate meta-analyses with the seed-based d mapping software package for functional neural activation and gray matter volume (GMV) were carried out, followed by a joint analysis and a subgroup analysis.
Results: This analysis included 29 VBM studies and 36 fMRI studies. Structurally, VBM analysis showed that the largest GMV diminutions in patients with ADHD were in several frontal-parietal brain regions, the limbic system, and the corpus callosum. Functionally, fMRI analysis discovered significant hypoactivation in several frontal-temporal brain regions, the right postcentral gyrus, the left insula, and the corpus callosum.
Conclusion: This study showed that abnormal alterations in the structure and function of the left superior frontal gyrus and the corpus callosum may be the key brain regions involved in the pathogenesis of ADHD in patients and may be employed as an imaging metric for patients with ADHD pending future research. In addition, this meta-analysis discovered neuroanatomical or functional abnormalities in other brain regions in patients with ADHD as well as findings that can be utilized to guide future research.
Introduction
According to the Diagnostic and Statistical Manual of Mental Disorders, Fifth Edition (DSM-5) and International Classification of Diseases (ICD-10), attention-deficit/hyperactivity disorder (ADHD) is a widespread neurodevelopmental disorder affected by multiple factors, which are often manifested as inattention and/or impulsiveness hyperactivity (1). The epidemiological investigation showed that over 5% of children and adolescents in the world are impacted by this illness (2), and the morbidity is higher in boys, showing an increasing trend year by year. However, some symptoms of the children could continue into adulthood (3), which would disturb the varied aspects of their learning, family, social lives, and/or physical and psychological health (including a higher proportion of destructive behaviors, anxiety, depressive behaviors, substance use disorders, and so on) (4, 5).
At present, studies in various fields, such as genetics (6, 7), etiology (8), neuropsychology (9), and neurophysiology (10), attempt to explore the pathological mechanisms of ADHD. Similar to other psychiatric and developmental disorders, ADHD has genetic susceptibility (6, 7). Environmental factors (including psychosocial factors, prenatal and perinatal risk factors, nutritional factors, etc.) are also one of the risk factors for ADHD (11, 12). Defects in mood regulation are common in patients with ADHD, which is considered a key factor associated with it (10). Other studies showed that the lack of DA (dopamine) in functional brain areas, such as the cerebral cortex and the striatum, also leads to ADHD (13). In addition, more neuroimaging studies (14–16) focused on patients with ADHD and considered ADHD an important research topic. A large amount of evidence suggests that ADHD has abnormal brain structure and function, although the effect sizes are small.
Voxel-based morphometry is a scientific method used to measure and analyze magnetic resonance imaging (MRI) at the voxel level (17) and reflect anatomical differences via quantitatively calculating the differences in density or volume of the gray matter and white matter of each voxel in the MRI. However, most of the current research results are inconsistent. Some studies reported decreased gray matter volume (GMV) in the right and left anterior cingulate cortex (ACC) (18) and the right thalamus (19) in patients with ADHD compared to healthy controls (HCs), while some other studies reported decreased GMV in the frontal lobe, the hippocampus, the temporal cortex, and the occipital cortex using VBM (20). Sutcubasi Kaya et al.'s study (21) reported increased GMV in the precentral gyrus and the supplementary motor area in patients with ADHD. The differences from those of earlier studies are attributed to patients with ADHD having a relatively high average IQ in the current studies. McGrath et al. (22) analyzed the structural neuroimaging data between dyslexia and ADHD, conducted an anatomic likelihood estimate (ALE) meta-analysis on VBM studies of the two diseases, and identified an overlapping region of the right caudate nucleus when using a more relaxed statistical threshold. This suggests that the abnormality of caudate nucleus may be the basis of executive dysfunction.
Functional MRI (fMRI) describes the activation region of neural excitation by monitoring blood oxygen levels and using deoxyhemoglobin as an endogenous contrast agent, characterized by low time resolution and high spatial resolution (23). At present, the fMRI studies of ADHD involve different executive function processes, and different task paradigms can be used to evaluate the same executive function, but there are a lot of heterogeneous research results in these studies (24). Decreased activation in alert task-related brain areas in children with ADHD, including the right frontal-orbital, the superior frontal and bilateral temporoparietal regions, the cerebellum, the hippocampus, the striatum, and the thalamus, was reported in a study by Rubia et al. (25). A meta-analysis of fMRI studies (26) on children with ADHD, with low activation in executive function (frontal-parietal network) and attention (ventral attention network) and high activation in prefrontal and ventral attention networks, identified earlier abnormalities beyond abnormal prefrontal-striatal circuits. The recent meta-analysis (27) of whole-brain fMRI showed reduced activation of the bilateral anterior insula putamen and the globus pallidus in the left inferior frontal gyrus in patients with ADHD during cognitive conversion tasks.
Recently, the results of a combined VBM and fMRI analysis (15) showed that reduced GMV and fMRI underactivation overlapped in the right caudate nucleus during cognitive control in patients with ADHD compared to HCs. Similar studies (28) found that increased GMV and functional activation overlapped in the right fusiform gyrus, while decreased GMV and functional activation overlapped in the right superior temporal gyrus, the left inferior frontal gyrus, and the left postcentral gyrus in patients with ADHD compared to HCs. Samea et al. (29) pooled 96 studies in children and adolescents, and they observed the convergence dysfunction in the left pallidum/putamen for task-fMRI experiments (using neutral stimuli) and decreased activity in the left IFG among different sub-analyses. This study has profoundly elaborated the structural and functional alterations of the brain in patients with ADHD; however, the results remain inconsistent, possibly due to the diversity of sample characteristics, behavioral scales, imaging methods, and statistical analyses, suggesting the need for in-depth investigation to confirm, broaden, and/or amend previous findings.
Based on previous theories and empirical studies in ADHD, we hypothesized that both functional and structural alterations in frontostriatal and fronto-cingulate circuits occur in patients with ADHD relative to HCs. In addition, studies suggest that some symptoms of ADHD in children will gradually get better with age but will not completely disappear. Therefore, we expect that more brain areas in the children group will show changed GMV and abnormal activation compared to the adult group. It may also be associated with delayed maturation of certain brain regions. The objective of our study was to explore the common and specific neural alterations in the structure and function of the brain in ADHD and further explore the differences in brain alterations between adults and children with ADHD. Eventually, the significance of these research results and their prospective development paths are examined and discussed.
Methods
Literature search and selection
A comprehensive literature search of fMRI and VBM studies of ADHD from January 2010 to November 2021 was conducted primarily using PubMed, as well as additional searches in the Web of Knowledge and Science Direct databases, with a combination of the following keywords: “voxel-based morphometry” or “VBM” or “morphometry” or “gray matter” or “functional magnetic resonance imaging” or “fMRI” and “ADHD” or “Attention Deficit/Hyperactivity Disorder.” In addition, a manual search was conducted in reference lists of previous meta-analyses. Eligible original studies were identified through reference tracking and consultation to retrieve high-quality meta-analyses and review articles.
Studies were eligible if (1) they involved task-related fMRI or VBM study; (2) they compared patients with ADHD and HCs; (3) the whole-brain outcomes were presented in three-dimensional coordinates (x, y, z) for changes in standard stereotactic space (e.g., Talairach space or Montreal Neurological Institute space); (4) the diagnosis of patients with ADHD had to be based on DSM-IV-TR, or DSM-V, or ICD-10; and (5) they were peer-reviewed and published in English as an article. Studies were excluded if (1) the peak coordinates were not reported; (2) VBM was not used and only ROI analyses were used; (3) the patient data were duplicated or no eligible contrasts were found; and (4) neurological or psychiatric comorbidities (such as depression, anxiety, autism, learning disorder, and so on) were found in the patient group. In the case of incomplete or ambiguous information, we will contact the author for clarification.
All the authors evaluated the final inclusion of articles and reached a consensus on all of them before implementing the following steps.
Statistical analysis
The anisotropic effect size seed-based d mapping (AES-SDM) (version 5.15) meta-analytic software package (https://www.sdmproject.com/) was applied extensively in a recent meta-analysis. We plan to use it to analyze the GMV and functional neural activation changes between patients with ADHD and HCs, following related guidelines (MOOSE) for meta-analyses. In this study, we summarized the AES-SDM data processing procedure. First, the peak coordinates extracted from each dataset were manually imported into the software. Second, the maps of the lower and upper bounds of possible effect sizes were reconstructed for each VBM or fMRI study separately using an anisotropic non-normalized Gaussian kernel. Peak coordinates were converted to Montreal Neurological Institute (MNI) space. After that, this mean analysis was processed, which consists of calculating the mean of the voxel values in the different studies. This mean is weighted by the inverse of the variance and accounts for inter-study heterogeneity. In addition, the results of some studies were p-value or z-value, which needed to be converted to t-statistics online; full width at half maximum (FWHM) was set to 20 mm because this setting was optimal to balance sensitivity and specificity, and other parameters included voxel P = 0.005, peak height Z = 1, and cluster extent = 10 voxels (30).
Our research steps are as follows: First, analyses were conducted to examine regional GMV in the patient group relative to HCs using all available data, and fMRI meta-analyses were performed to examine the neural activation abnormalities using the above same method. Second, a conjunction analysis in patient groups relative to HCs was further carried out to examine common areas of structural and functional abnormalities. Then, we divided individuals into two different subtypes based on age group (see Table 2) for the next subgroup analysis, namely, adult and pediatric (including adolescents and children) subjects, using a random effects model under the same threshold as before. To examine the effects of age, gender, and IQ, meta-regression analyses were performed. Finally, to assess whether the results were replicable, a jackknife sensitivity analysis was performed, in which the same analysis was repeated in one study, excluding one data at a time. Moreover, Egger's test was used to examine the possible publication bias. A statistical threshold p-value of <0.005 was used for all meta-analyses (31, 32), and a decreased threshold p-value of <0.0005 and a cluster extent of 20 voxels were applied in the meta-regression to control for false positives (33).
Results
A total of 1,463 published papers (624 VBM studies and 839 fMRI studies) were screened, with an increase of 21 documents (six VBM studies and 15 fMRI studies) discovered via other sources. After repetitions were eliminated, 591 documents (239 VBM studies and 352 fMRI studies) were reviewed, and 144 full-text publications (61 VBM studies and 83 fMRI studies) were evaluated for eligibility. The final sample included 29 VBM research (1,211 patients with ADHD and 1,032 HCs) and 36 fMRI research (850 patients with ADHD and 813 HCs) studies (see Figure 1; Table 1 for more details).
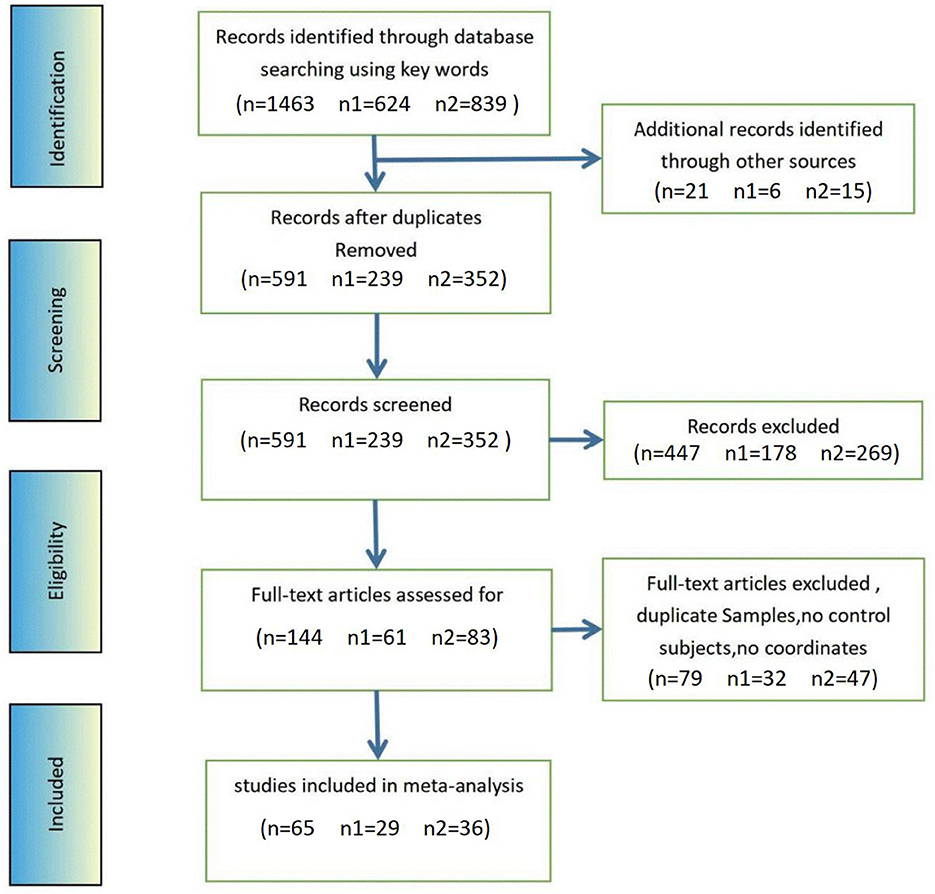
Figure 1. Methods and results of literature search for ADHD fMRI and VBM. n, total number of studies; n1, number of VBM study; n2, number of fMRI study.
The Wilcoxon W-tests in the whole-group VBM analysis indicated that patient groups did not differ in age (z = −0.325; P = 0.745), and the patient groups have a slightly lower IQ than the control groups (z = −2.653; P = 0.008). The chi-squared test revealed that both groups had a significantly higher proportion of boys/men (χ2 = 8.450; P = 0.004). In the whole-group fMRI meta-analysis, patient groups did not differ in age (z = −1.077; P = 0.282), and the patient groups have a slightly lower IQ than the control groups (z = −3.616; P < 0.001), but a higher percentage of patients with ADHD were boys/men (χ2 = 50.655; P < 0.001). Details of the regression analysis results of the subgroups are shown in Table 2. As a result, age, IQ, and sex were included as covariates in all between-group meta-analyses performed including only these studies which were age-, IQ-, and sex-matched (see Table 2 for more details).
Regional differences in GMV and fMRI by meta-analysis
ADHD VBM
Patients with ADHD showed significantly lower GMV in the bilateral anterior cingulate cortex/median cingulate/superior frontal gyrus/olfactory cortex, the left precentral/postcentral gyrus, the left inferior frontal gyrus opercular/orbital part, the left supramarginal gyrus, the left caudate nucleus, the left anterior thalamic projections, the right gyrus rectus, and the corpus callosum compared to HCs. In contrast, the results also showed an increase in the left striatum/lenticular nucleus, the right caudate nucleus, and the right anterior thalamic projections (see Table 3; Figure 2).
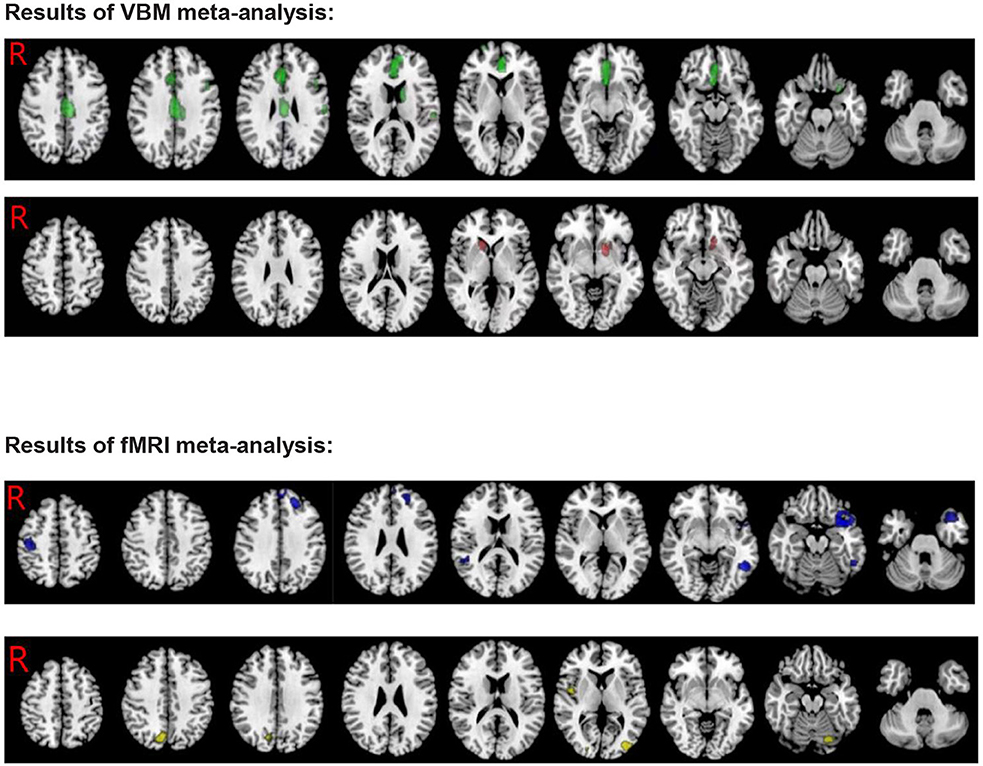
Figure 2. Results of VBM and fMRI meta-analysis for ADHD (Green, GMV decreased in ADHD; Red, GMV increased in ADHD; Blue, hypoactivation in ADHD; Yellow, overactivation in ADHD).
ADHD fMRI
Patients with ADHD showed overactivation in the middle occipital gyrus, the right insula, the right precuneus cortex, the left cerebellum hemispheric lobule, and the left fusiform gyrus compared to HCs. In contrast, the results also showed hypoactivation in the bilateral superior temporal gyrus, the left middle/inferior temporal gyrus, the left superior/middle/inferior frontal gyrus, the right precentral /postcentral gyrus, the left insula, and the corpus callosum (see Table 3; Figure 2).
Multimodal VBM and fMRI analyses
In patients with ADHD, there was an overlap between decreased GMV in the left inferior frontal gyrus and hypoactivation compared to HCs (MNI coordinates, −28, 16, −24; 17 voxels) (see Figure 3).
Subgroup analysis
ADHD VBM
Individuals were divided into two independent subgroups, namely, adult and pediatric groups (including adolescents and children). The findings of the adult group's (11 datasets) analysis were substantially in line with the pooled analysis, except for the additional significant GMV decrease in the right middle frontal gyrus. In addition, relative to the pooled analysis, no significant GMV decrease regions were found in the left supramarginal gyrus and the left inferior frontal gyrus. Similarly, the results also indicated increased GMV in the left striatum and the left lenticular nucleus. The results of the pediatric group's (19 datasets) analysis showed GMV decrease in additional brain regions—the bilateral middle frontal gyrus, the bilateral superior temporal gyrus, and the left insula. Similarly, relative to the pooled analysis, no significant GMV decrease regions were found in the left supramarginal gyrus, the left caudate nucleus, and the left anterior thalamic projections, the left precentral /postcentral gyrus, and the right gyrus rectus. In addition, the results showed increased GMV in the left striatum, the left middle occipital gyrus, the right caudate nucleus, and the right anterior thalamic projections (see Tables 4, 5; Figure 4).
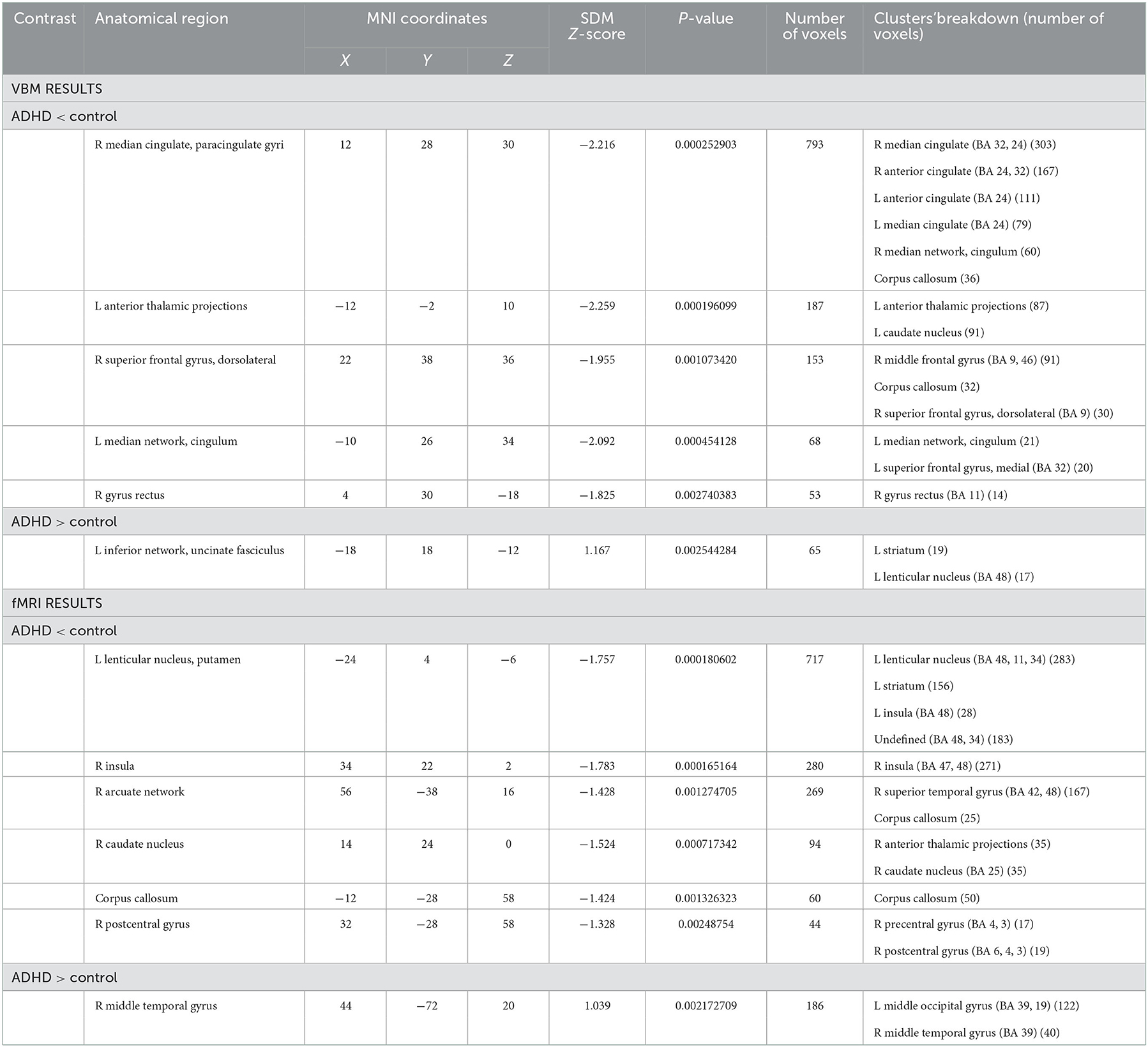
Table 4. Subgroup analysis results for voxel-based morphometry and fMRI studies in ADHD (adults group).
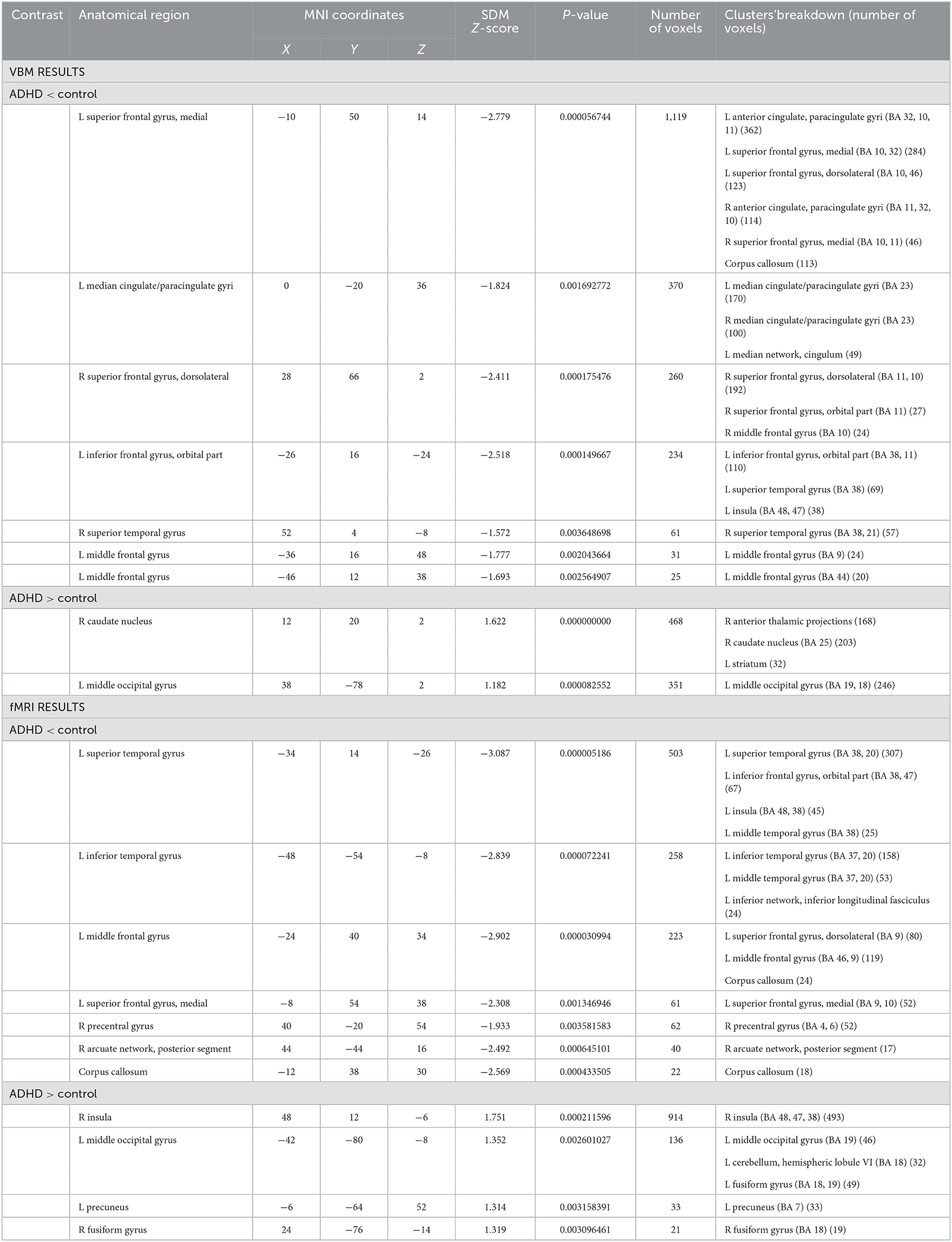
Table 5. Subgroup analysis results for voxel-based morphometry and fMRI studies in ADHD (pediatrics group).
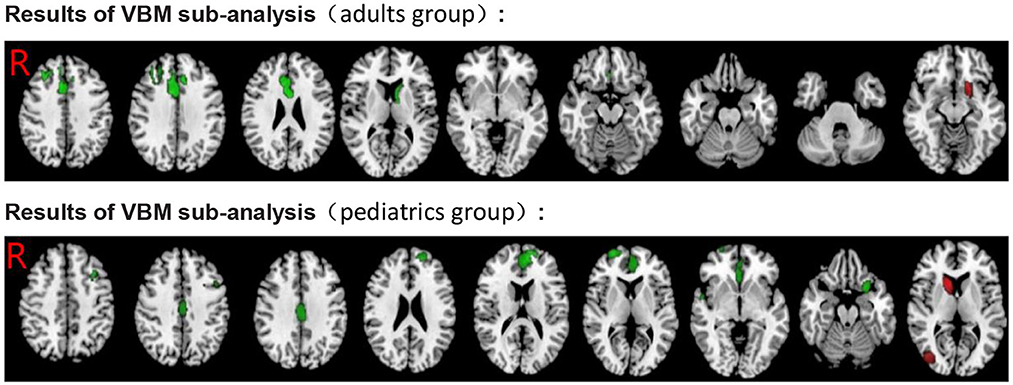
Figure 4. Results of subgroup analysis of VBM for ADHD. Green, GMV decreased in ADHD; Red, GMV increased in ADHD.
ADHD fMRI
Similarl to the previous analysis, we divided individuals into adults and pediatrics (including adolescents and children), two independent subgroups. The findings of the adult group (11 datasets) were partly consistent with the pooled analysis, and significant hypoactivation in the bilateral insula, the left striatum, the left lenticular nucleus, the right superior temporal gyrus, the right precentral/postcentral gyrus, the right caudate nucleus, the right anterior thalamic projections, and the corpus callosum was found. In addition, significant overactivation regions were found in the left middle occipital gyrus and the right middle temporal gyrus. Relative to the pooled analysis, in the pediatric group (22 datasets), no significant hypoactivation was found in the right superior temporal gyrus and the right postcentral gyrus. On the contrary, overactivation of the right fusiform gyrus was found. The rest was largely consistent with the pooled analysis (see Tables 4, 5; Figure 5).
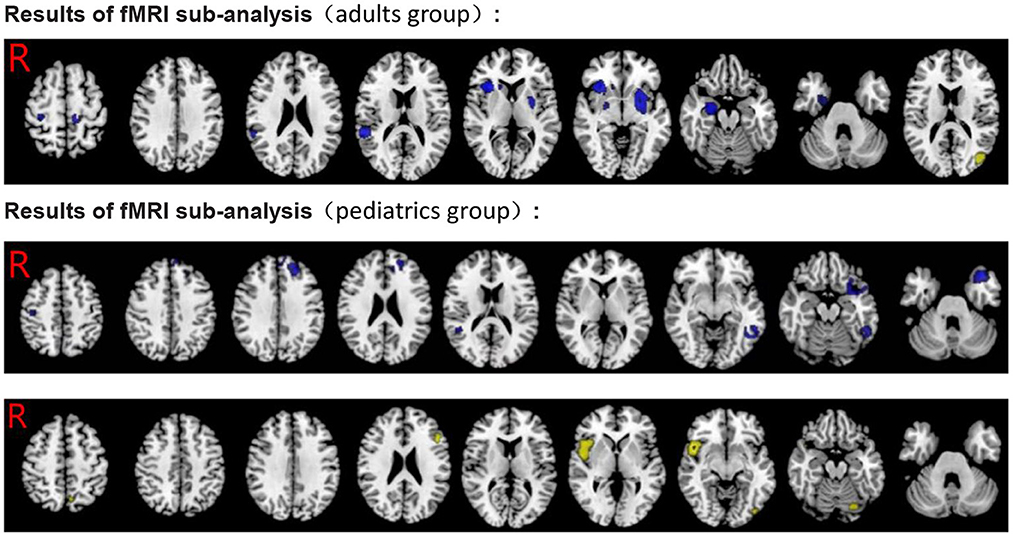
Figure 5. Results of subgroup analysis of fMRI for ADHD. Blue, hypoactivation in ADHD; Yellow, overactivation in ADHD.
Publication bias
Egger's tests were conducted to investigate the potential publication bias. The results of the Egger's tests were non-significant (P > 0.05 for all comparisons, Bonferroni corrected), indicating that there was no publication bias. Jackknife reliability analyses revealed that the findings were robust in terms of disorder differentiation.
Discussion
The purpose of our meta-analysis was to investigate the altered GMVs and functional happenings in patients with ADHD and further explore the differences in brain alterations between adults and children with ADHD. Our meta-analysis of structural studies showed that the largest GMV reductions in patients with ADHD relative to HCs were in several frontal parietal brain regions, the limbic system, and the corpus callosum. In addition, we also found that the GMV increased in some brain areas. In fMRI, our study discovered that patients with ADHD relative to HCs showed significant hypoactivation in several frontal-temporal brain regions, the right postcentral gyrus, the left insula, and the corpus callosum. Conversely, we observed overactivation in the middle occipital gyrus, the right insula, the right precuneus cortex, and the left cerebellum hemispheric lobule. Most of the brain region alterations we found were consistent with previous literature, suggesting that the results of the meta-analysis using the seed-based d mapping software package were credible. The results of the subgroup analysis further explored and supported the above results. Moreover, subgroup analysis found relatively more brain areas with reduced GMV and abnormal activation in children and adolescents relative to adults. This makes it clear that patients with ADHD may be associated with delayed maturation of brain development.
In recent years, ADHD has often been considered as related to brain changes in the frontal region. Earlier studies discovered reduced volume in the right superior frontal gyrus (96) and the bilateral dorsolateral prefrontal (97). Bralten et al.'s (37) VBM analysis mentioned that ADHD has a link with reduced GMV in the frontal and precentral regions (including the left precentral gyrus, the medial and left orbitofrontal cortices, the frontal pole, the paracingulate and cingulate cortices), concerning decision-making, executive function, and motor function areas. This is largely consistent with the results of our meta-analysis. In fMRI, we found hypoactivation in the left superior middle and the inferior frontal gyrus and the right anterior central gyrus. Task-based fMRI studies on patients with ADHD revealed inadequate activity in the frontal lobe and striatum (64, 98). Regarding previous fMRI studies, patients with ADHD had lower activation for inhibition in the supplementary motor area, the anterior cingulate cortex, and the dorsolateral prefrontal cortex compared to HCs (99, 100). It is of great importance for the orbitofrontal cortex to regulate emotion and impulsive behavior, and impairment in this area may be the cause of impulsivity in ADHD (101). Moreover, previous findings suggested that the prefrontal cortex and its connections may be closely related to ADHD symptoms including inattention, obliviousness, impulsivity, poor planning, and hyperaction in patients with ADHD (102). In addition, our multimodal results show a decrease in the volume of the left subfrontal gyrus gray matter and a decrease in activity. Thus, our findings support the frontal-striatal circuit theory of ADHD and confirm prior VBM and fMRI studies on ADHD. Future longitudinal studies should strive to evaluate the long-term consequences of ADHD treatments on prefrontal volume and function to confirm the existing results.
The present findings also involve the limbic system-associated brain area. Because of its importance in symptom severity and emotional regulation, the anterior cingulate cortex has long been a focus of ADHD study (103). Similarly, we also found that volume reductions in the bilateral anterior cingulate/paracingulate gyrus, the bilateral median cingulate, the bilateral olfactory cortex, and the left caudate nucleus. However, in the present pediatric group of subgroup analysis, we found no significant GMV decrease in the left caudate nucleus. In a study of 24 adolescent subjects with ADHD vs. HCs, the ADHD group had a lower mean (left and right) caudate and putamen volumes (104). The article of Montes et al. (35) suggested that patients with ADHD had a smaller GMV and a lower concentration of gray matter in the right caudate compared to HCs, both in the overall sample and within each sex. Moreover, a previous study (40) found reduced GMVs in the right orbitofrontal cortex, the left anterior cingulate cortex, and the left posterior midcingulate cortex in patients with ADHD. Amico et al. (18) found significantly smaller GMV in the right and left anterior cingulate cortices but no GMV difference in the PFC, the hippocampus, and the amygdala. Bonath et al. (36) discovered substantially lower GMV in participants with ADHD relative to their matched controls within the anterior cingulate cortex, within the bilateral hippocampus/amygdala, and in broad cerebellar areas. Further, reduced anterior cingulate cortex GMV was also observed to connect with grades of selective inattention. However, these alterations in the hippocampus, the amygdala, and the cerebellum did not appear in our study-based VBM, indicating that they are less consistent across samples compared to the changes in the limbic system. Conversely, we also found that the GMV increased in the left striatum/lenticular nucleus and the right caudate nucleus. Collectively, the limbic system should receive more attention in future research to explore the possible pathogenesis of ADHD.
In patients with ADHD, inattention was linked to reduced GMV not only in the frontal and anterior cingulate but also in the parietal regions. Although only a few ADHD functional MRI investigations have focused on the parietal cortex, it has been recognized as exhibiting changed function in ADHD. This can also be observed in our study, whose results showed that theparietal lobes (including the left postcentral gyrus and left supramarginal gyrus) are smaller in size in patients with ADHD. Simultaneously, we also found significant hypoactivation in the right postcentral gyrus. Previous research (105) found that patients with ADHD doing a visual oddball task showed considerably reduced parietal cortex activation, including the superior parietal gyrus and numerous regions of the inferior parietal lobe. Another study (106) indicated that, in participants with ADHD conducting a spatial working memory mental rotation assignment, the inferior parietal lobe activation was significantly lower, as well as parieto-occipital and caudate activation. Prior research (64) found that successful inhibition activated a cluster of the right angular gyrus, the intraparietal sulcus, and the inferior parietal gyrus in the ADHD group. These regions have been associated with action cancellation and action withholding (107). A previous meta-analysis (41) revealed that smaller GMV was found in the bilateral inferior parietal lobule in subjects with ADHD. However, Seidman et al. (20) found that adults with ADHD had significantly smaller GMVs in the inferior parietal cortex, relative to HCs. We speculated that this difference may be related to the maturational delay or sample diversity. The structural and functional abnormalities in the parietal lobes of those with ADHD further support the conclusion that the parietal cortex plays a role in the pathogenesis of ADHD in patients.
In addition, our analysis results suggest that the temporal lobe may play a crucial role in ADHD. The results of the meta-analysis of fMRI indicate significant hypoactivation in the bilateral superior temporal gyrus and left middle/inferior temporal gyrus. However, decreased GMV was found in the bilateral superior temporal gyrus of the pediatric group alone. Sowell et al. (97) found reduced GMV bilaterally in the lateral aspects of anterior and midtemporal cortices and increased GMV in the more posterior aspects of the temporal lobes bilaterally. The temporal lobe includes auditory language-related centers, while some children with ADHD have abnormal auditory language functions. Using VBM, the studies of Kobel et al. (44) detected isolated areas in right temporal regions where boys with ADHD displayed decreased GMV, demonstrating some type of impairment to temporal brain matter due to ADHD. This result was further supported by a prior investigation (108) where the authors discovered a link between damaged inhibitory control and decreases in medial temporal gray matter. Some studies (91, 109) found insufficient activation in the left superior temporal gyrus. Although these temporal lobe abnormalities in the brain are not completely consistent, we reasoned that the temporal lobe plays a key role in the development of ADHD. As a result, we came to an agreement that research on ADHD ought not to be limited to the frontostriatal deficit hypothesis but also expanded the range to less studied brain areas like the temporal lobe.
It is worth mentioning that we simultaneously found reduced corpus callosum GMV in patients with ADHD relative to HCs and showed hypoactivation in the corpus callosum in fMRI. Considering the anterior or genu region of the corpus callosum connects homologous areas of the frontal cortex (110), theories concerning ADHD being a frontal-striatal illness (111) have motivated some scholars to anticipate that the anterior or genu region of the corpus callosum would be influenced. Significantly smaller areas have been reported in the total corpus callosum area and splenium (112). The meta-analysis of Valera et al. (113) also showed that the splenium located in the posterior region of the corpus callosum is smaller in individuals with ADHD. In patients with ADHD, there is evidence that there are sex disparities in the morphology and white matter integrity of the corpus callosum. For example, female patients with ADHD distinctly had a smaller splenium than male patients with ADHD, according to a meta-analysis (114), while male children and adolescents with ADHD have a smaller anterior corpus callosum. Dramsdahl et al. (115) discovered lower fractional anisotropy values in the isthmus/splenium of the corpus callosum in the ADHD group, and subsequent investigation revealed that FA (fractional anisotropy) values in the posterior part of the corpus callosum were considerably lower in women than in men. These research results show that the role of the corpus callosum in ADHD should be further explored.
The meta-analysis of Jagger-Rickels et al. (41) found reduced left and right insula volume in the ADHD group. Furthermore, in a previous large meta-analysis (16) of sMRI and fMRI studies in children and adults with ADHD, the anterior insula was the only region with both structural and functional abnormalities. However, we found no statistically significant GMV change in the insula. However, overactivation was found in the right insula among patients with ADHD in our study, and this finding is consistent with the results of a previous study (80). In contrast, we also found hypoactivation in the left insula. The insula is also a critical neural structure in the emotional response during decision-making (116). Vetter et al. (92) demonstrated that male patients with ADHD who did not have oppositional defiant disorder/conduct disorder had hyperactivation of the anterior insula for negative stimuli, and hyperactivation of the anterior insula is the basis of this emotional lability.
In our study, increased activation was also found in the left middle occipital gyrus, right precuneus, and left cerebellar hemisphere among patients with ADHD compared to HCs. Occipital cortex, as the center of visual cortex, is responsible for the processing of visual information. To maintain attention, occipital cortex interacts with the dorsal attention network (117), inhibiting the attention caused by irrelevant stimuli (118), whereas the attention caused by hardly ignoring irrelevant stimuli is one of the main symptoms of ADHD. The precuneus is related to visual imagery, attention, and memory retrieval by engaging in the visual process and integrating relevant memory (119). A possible explanation is that high activation in the left middle occipital gyrus and the right precuneus is associated with the severity of symptoms of impulsive craving in patients with ADHD. Although many studies reported abnormalities of structure and function in the cerebellar in patients with ADHD, our study did not reveal its structural differences but found that the left cerebellar hemisphere showed high activation on the task-state fMRI. A recent study (120) suggests that inadequate neuromodulation of prefrontal-cerebellar circuits may underlie cognitive deficits in psychiatric disorders (including schizophrenia, bipolar disorder, and ADHD). In addition, studies (121) showed that both MPH and ATX can restore abnormal brain function in ADHD, mainly involving fronto-cingulo-parieto-cerebellum circuit. In conclusion, the brain changes of patients with ADHD may not be influenced by a single brain region but by multi-channel connections or there may be a certain brain region that is the most critical, which needs to be further studied by other methods such as brain structural network covariation and functional connectivity in the future.
The main results of the whole-group analysis were also found in the adult group and the pediatric group, which further supported the whole-group results. Alterations in the left superior frontal gyrus and the corpus callosum persisted in the pediatrics group and adult group, which we believe further illustrates the crux of this brain region. Subgroup analysis found relatively more brain areas with reduced GMV and abnormal activation in children and adolescents compared to adults. Longitudinal MRI studies (122, 123) have already shown that structural differences in the frontal, striatal, parietal, and cerebellar regions of children with ADHD could be caused by a delay in structural maturation. The research of Hoogman et al. (124) proposed a related model of ADHD brain maturation delay disorder and found a peak delay in subcortical volume maturation. The pediatric group result in our study found that both the left insula and the left superior temporal gyrus reduced GMV in the VBM meta-analysis and hypoactivation in the fMRI meta-analysis, and no such finding was observed in the adult group. The left insula and the left superior temporal gyrus brain area were changed in the pediatric group, but they did not change in the adult group, and we could consider that the alterations in these brain areas may result from delayed maturation. One study showed that many adult patients with ADHD have improved symptoms compared to childhood (125). In addition, evidence reveals that the symptoms of ADHD, as well as ADHD diagnosis, are linked to substantial alterations in the macroscopic and microscopic structures of the brain. Therefore, we speculate that abnormal structure and function of some brain regions in patients with ADHD may also be gradually normal with increasing age, and these brain regions are caused by delayed maturation disorder, which still needs further research to prove.
Limitation
Our study has several limitations that are inherent in all meta-analyses. In the first place, since it was based predominantly on the peak coordinates of published studies, instead of raw statistical brain maps, it may be underpowered to identify some results with small or moderate effect sizes. Second, for the many papers included in this meta-analysis, different statistical thresholds may have been applied. Additionally, the heterogeneity of the methodologies among VBM and fMRI studies could not be prevented, such as the differences in MRI machines, smoothing kernel size, statistical thresholding, and so on, which might have caused conflicting results. Moreover, fMRI studies are mostly based on tasks in different attention, inhibition, time, and motivation domain, and then, the results may not be precise enough. Future studies should minimize these differences and confounding variables to get a more convincing result.
Conclusion
ADHD has far-ranging structural and functional abnormalities in the brain. The VBM meta-analysis found reduced GMV in the left superior frontal gyrus and the corpus callosum in patients with ADHD compared to HCs. Studies in task-related fMRI found that patients with ADHD showed low activation in the abovementioned brain regions. We hold the opinion that abnormal alterations in the structure and function of the left superior frontal gyrus and the corpus callosum may be the key brain regions involved in the pathogenesis of ADHD in patients and may be employed as an imaging metric for patients with ADHD pending future research. This meta-analysis also identified neuroanatomical or functional abnormalities in other brain regions in ADHD. Besides, subgroup analysis further explored and supported the above results, and this information can be applied to guide future studies. However, there is a need for more research in this area because of the lack of clarity on the confounding effects. Our results, as solid as it appears, should be read with caution.
Data availability statement
The original contributions presented in the study are included in the article/supplementary material, further inquiries can be directed to the corresponding authors.
Author contributions
MY and XG designed the experiment. MY, XG, XN, and MZ performed the experiment. ZY, SH, JC, and YZ modified the experiment and drafted the manuscript. All authors contributed to the article and provided approval for the final version of the manuscript.
Conflict of interest
The authors declare that the research was conducted in the absence of any commercial or financial relationships that could be construed as a potential conflict of interest.
Publisher's note
All claims expressed in this article are solely those of the authors and do not necessarily represent those of their affiliated organizations, or those of the publisher, the editors and the reviewers. Any product that may be evaluated in this article, or claim that may be made by its manufacturer, is not guaranteed or endorsed by the publisher.
References
1. Battle DE. Diagnostic and statistical manual of mental disorders (DSM). Codas. (2013) 25:191–2. doi: 10.1590/s2317-17822013000200017
2. Polanczyk GV, Willcutt EG, Salum GA, Kieling C, Rohde LA. ADHD prevalence estimates across three decades: an updated systematic review and meta-regression analysis. Int J Epidemiol. (2014) 43:434–42. doi: 10.1093/ije/dyt261
3. Sibley MH, Swanson JM, Arnold LE, Hechtman LT, Owens EB, Stehli A, et al. Defining ADHD symptom persistence in adulthood: optimizing sensitivity and specificity. J Child Psychol Psychiatry. (2017) 58:655–62. doi: 10.1111/jcpp.12620
4. Biederman J, Faraone SV, Spencer T, Wilens T, Norman D, Lapey KA, et al. Patterns of psychiatric comorbidity, cognition, and psychosocial functioning in adults with attention deficit hyperactivity disorder. Am J Psychiatry. (1993) 150:1792–8. doi: 10.1176/ajp.150.12.1792
5. McGough JJ, Smalley SL, McCracken JT, Yang M, Del'Homme M, Lynn DE, et al. Psychiatric comorbidity in adult attention deficit hyperactivity disorder: findings from multiplex families. Am J Psychiatry. (2005) 162:1621–7. doi: 10.1176/appi.ajp.162.9.1621
6. Barker ED, Ing A, Biondo F, Jia T, Pingault J-B, Du Rietz E, et al. Do ADHD-impulsivity and BMI have shared polygenic and neural correlates? Mol Psychiatry. (2021) 26:1019–28. doi: 10.1038/s41380-019-0444-y
7. Pujol-Gualdo N, Sánchez-Mora C, Ramos-Quiroga JA, Ribasés M, Artigas MS. Integrating genomics and transcriptomics: towards deciphering ADHD. Eur Neuropsychopharmacol. (2021) 44:1–13. doi: 10.1016/j.euroneuro.2021.01.002
8. Fluegge K, Fluegge K. Environmental factors influencing the link between childhood ADHD and risk of adult coronary artery disease. Med Hypoth. (2018) 110:83–5. doi: 10.1016/j.mehy.2017.11.007
9. Bennett JK, O'Neill S, Rajendran K, Halperin JM. Do preschoolers' neuropsychological functioning and hyperactivity/inattention predict social functioning trajectories through childhood? J Pediatr Psychol. (2020) 45:793–802. doi: 10.1093/jpepsy/jsaa053
10. Christiansen H, Hirsch O, Albrecht B, Chavanon ML. Attention-deficit/hyperactivity disorder (ADHD) and emotion regulation over the life span. Curr Psychiatry Rep. (2019) 21:17. doi: 10.1007/s11920-019-1003-6
11. Deater-Deckard K. Parents’ and children’s ADHD in a family system. J Abnorm Child Psychol. (2017) 45:519–25. doi: 10.1007/s10802-017-0276-7
12. Peterson BS, Rauh VA, Bansal R, Hao X, Toth Z, Nati G, et al. Effects of prenatal exposure to air pollutants (polycyclic aromatic hydrocarbons) on the development of brain white matter, cognition, and behavior in later childhood. JAMA Psychiatry. (2015) 72:531–40. doi: 10.1001/jamapsychiatry.2015.57
13. Somkuwar SS, Jordan CJ, Kantak KM, Dwoskin LP. Adolescent atomoxetine treatment in a rodent model of ADHD: effects on cocaine self-administration and dopamine transporters in frontostriatal regions. Neuropsychopharmacology. (2013) 38:2588–97. doi: 10.1038/npp.2013.163
14. Hoogman M, Muetzel R, Guimaraes JP, Shumskaya E, Mennes M, Zwiers MP, et al. Brain imaging of the cortex in ADHD: a coordinated analysis of large-scale clinical and population-based samples. Am J Psychiatry. (2019) 176:531–42. doi: 10.1176/appi.ajp.2019.18091033
15. Lukito S, Norman L, Carlisi C, Radua J, Hart H, Simonoff E, et al. Comparative meta-analyses of brain structural and functional abnormalities during cognitive control in attention-deficit/hyperactivity disorder and autism spectrum disorder. Psychol Med. (2020) 50:894–919. doi: 10.1017/S0033291720000574
16. Norman LJ, Carlisi C, Lukito S, Hart H, Mataix-Cols D, Radua J, et al. Structural and functional brain abnormalities in attention-deficit/hyperactivity disorder and obsessive-compulsive disorder: a comparative meta-analysis. JAMA Psychiatry. (2016) 73:815–25. doi: 10.1001/jamapsychiatry.2016.0700
17. Ashburner J, Friston KJ. Voxel-based morphometry–the methods. Neuroimage. (2000) 11:805–21. doi: 10.1006/nimg.2000.0582
18. Amico F, Stauber J, Koutsouleris N, Frodl T. Anterior cingulate cortex gray matter abnormalities in adults with attention deficit hyperactivity disorder: a voxel-based morphometry study. Psychiatry Res. (2011) 191:31–5. doi: 10.1016/j.pscychresns.2010.08.011
19. Xia S, Li X, Kimball AE, Kelly MS, Lesser I, Branch C. Thalamic shape and connectivity abnormalities in children with attention-deficit/hyperactivity disorder. Psychiatry Res. (2012) 204:161–7. doi: 10.1016/j.pscychresns.2012.04.011
20. Seidman LJ, Biederman J, Liang L, Valera EM, Monuteaux MC, Brown A, et al. Gray matter alterations in adults with attention-deficit/hyperactivity disorder identified by voxel based morphometry. Biol Psychiatry. (2011) 69:857–66. doi: 10.1016/j.biopsych.2010.09.053
21. Kaya BS, Metin B, Tas ZC, Buyukaslan A, Soysal A, Hatiloglu D, et al. Gray matter increase in motor cortex in pediatric ADHD: a voxel-based morphometry study. J Atten Disord. (2018) 22:611–8. doi: 10.1177/1087054716659139
22. McGrath LM, Stoodley CJ. Are there shared neural correlates between dyslexia and ADHD? A meta-analysis of voxel-based morphometry studies. J Neurodev Disord. (2019) 11:31. doi: 10.1186/s11689-019-9287-8
23. Biswal B, Yetkin FZ, Haughton VM, Hyde JS. Functional connectivity in the motor cortex of resting human brain using echo-planar MRI. Magn Reson Med. (1995) 34:537–41. doi: 10.1002/mrm.1910340409
24. Morein-Zamir S, Dodds C, van Hartevelt TJ, Schwarzkopf W, Sahakian B, Müller U, et al. Hypoactivation in right inferior frontal cortex is specifically associated with motor response inhibition in adult ADHD. Hum Brain Mapp. (2014) 35:5141–52. doi: 10.1002/hbm.22539
25. Rubia K, Halari R, Cubillo A, Mohammad A-M, Brammer M, Taylor E. Methylphenidate normalises activation and functional connectivity deficits in attention and motivation networks in medication-naïve children with ADHD during a rewarded continuous performance task. Neuropharmacology. (2009) 57:640–52. doi: 10.1016/j.neuropharm.2009.08.013
26. Cortese S, Kelly C, Chabernaud C, Proal E, Di Martino A, Milham MP, et al. Toward systems neuroscience of ADHD: a meta-analysis of 55 fMRI studies. Am J Psychiatry. (2012) 169:1038–55. doi: 10.1176/appi.ajp.2012.11101521
27. Rubia K. Cognitive neuroscience of attention deficit hyperactivity disorder (ADHD) and its clinical translation. Front Hum Neurosci. (2018) 12:100. doi: 10.3389/fnhum.2018.00100
28. Gao X, Zhang M, Yang Z, Wen M, Huang H, Zheng R, et al. Structural and functional brain abnormalities in internet gaming disorder and attention-deficit/hyperactivity disorder: a comparative meta-analysis. Front Psychiatry. (2021) 12:679437. doi: 10.3389/fpsyt.2021.679437
29. Samea F, Soluki S, Nejati V, Zarei M, Cortese S, Eickhoff SB, et al. Brain alterations in children/adolescents with ADHD revisited: a neuroimaging meta-analysis of 96 structural and functional studies. Neurosci Biobehav Rev. (2019) 100:1–8. doi: 10.1016/j.neubiorev.2019.02.011
30. Yang Z, Zhang Y, Cheng J, Zheng R. Meta-analysis of brain gray matter changes in chronic smokers. Eur J Radiol. (2020) 132:109300. doi: 10.1016/j.ejrad.2020.109300
31. Radua J, Mataix-Cols D. Voxel-wise meta-analysis of grey matter changes in obsessive-compulsive disorder. Br J Psychiatry. (2009) 195:393–402. doi: 10.1192/bjp.bp.108.055046
32. Radua J, Mataix-Cols D, Phillips ML, El-Hage W, Kronhaus DM, Cardoner N, et al. A new meta-analytic method for neuroimaging studies that combines reported peak coordinates and statistical parametric maps. Eur Psychiatry. (2012) 27:605–11. doi: 10.1016/j.eurpsy.2011.04.001
33. Radua J, Borgwardt S, Crescini A, Mataix-Cols D, Meyer-Lindenberg A, McGuire PK, et al. Multimodal meta-analysis of structural and functional brain changes in first episode psychosis and the effects of antipsychotic medication. Neurosci Biobehav Rev. (2012) 36:2325–33. doi: 10.1016/j.neubiorev.2012.07.012
34. Ahrendts J, Rüsch N, Wilke M, Philipsen A, Eickhoff SB, Glauche V, et al. Visual cortex abnormalities in adults with ADHD: a structural MRI study. World J Biol Psychiatry. (2011) 12:260–70. doi: 10.3109/15622975.2010.518624
35. Montes LGA, Ricardo-Garcell J, Torre LBBD, Alcántara HP, García RBM, Fernández-Bouzas A, et al. Clinical correlations of grey matter reductions in the caudate nucleus of adults with attention deficit hyperactivity disorder. J Psychiatry Neurosci. (2010) 35:238–46. doi: 10.1503/jpn.090099
36. Bonath B, Tegelbeckers J, Wilke M, Flechtner H-H, Krauel K. Regional gray matter volume differences between adolescents with ADHD and typically developing controls: further evidence for anterior cingulate involvement. J Atten Disord. (2018) 22:627–38. doi: 10.1177/1087054715619682
37. Bralten J, Greven CU, Franke B, Mennes M, Zwiers MP, Rommelse NN, et al. Voxel-based morphometry analysis reveals frontal brain differences in participants with ADHD and their unaffected siblings. J Psychiatry Neurosci. (2016) 41:272–9. doi: 10.1503/jpn.140377
38. Tsai C-J, Lin H-Y, Tseng IW-Y, Gau SS-F. Brain voxel-based morphometry correlates of emotion dysregulation in attention-deficit hyperactivity disorder. Brain Imaging Behav. (2021) 15:1388–402. doi: 10.1007/s11682-020-00338-y
39. Gehricke J-G, Kruggel F, Thampipop T, Alejo SD, Tatos E, Fallon J, et al. The brain anatomy of attention-deficit/hyperactivity disorder in young adults - a magnetic resonance imaging study. PLoS ONE. (2017) 12:e0175433. doi: 10.1371/journal.pone.0175433
40. He N, Li F, Li Y, Guo L, Chen L, Huang X, et al. Neuroanatomical deficits correlate with executive dysfunction in boys with attention deficit hyperactivity disorder. Neurosci Lett. (2015) 600:45–9. doi: 10.1016/j.neulet.2015.05.062
41. Jagger-Rickels AC, Kibby MY, Constance JM. Global gray matter morphometry differences between children with reading disability, ADHD, and comorbid reading disability/ADHD. Brain Lang. (2018) 185:54–66. doi: 10.1016/j.bandl.2018.08.004
42. Kappel V, Lorenz RC, Streifling M, Renneberg B, Lehmkuhl U, Ströhle A, et al. Effect of brain structure and function on reward anticipation in children and adults with attention deficit hyperactivity disorder combined subtype. Soc Cogn Affect Neurosci. (2015) 10:945–51. doi: 10.1093/scan/nsu135
43. Klein M, Souza-Duran FL, Menezes AKPM, Alves TM, Busatto G, Louzã MR. Gray matter volume in elderly adults with ADHD: associations of symptoms and comorbidities with brain structures. J Atten Disord. (2021) 25:829–38. doi: 10.1177/1087054719855683
44. Kobel M, Bechtel N, Specht K, Klarhöfer M, Weber P, Scheffler K, et al. Structural and functional imaging approaches in attention deficit/hyperactivity disorder: does the temporal lobe play a key role? Psychiatry Res. (2010) 183:230–6. doi: 10.1016/j.pscychresns.2010.03.010
45. Kumar U, Arya A, Agarwal V. Neural alterations in ADHD children as indicated by voxel-based cortical thickness and morphometry analysis. Brain Dev. (2017) 39:403–10. doi: 10.1016/j.braindev.2016.12.002
46. Li X, Cao Q, Pu F, Li D, Fan Y, An L, et al. Abnormalities of structural covariance networks in drug-naive boys with attention deficit hyperactivity disorder. Psychiatry Res. (2015) 231:273–8. doi: 10.1016/j.pscychresns.2015.01.006
47. Lim L, Marquand A, Cubillo AA, Smith AB, Chantiluke K, Simmons A, et al. Disorder-specific predictive classification of adolescents with attention deficit hyperactivity disorder (ADHD) relative to autism using structural magnetic resonance imaging PLoS ONE. (2013) 8:e63660. doi: 10.1371/journal.pone.0063660
48. Stevens MC, Haney-Caron E. Comparison of brain volume abnormalities between ADHD and conduct disorder in adolescence. J Psychiatry Neurosci. (2012) 37:389–98. doi: 10.1503/jpn.110148
49. Moreno-Alcázar A, Ramos-Quiroga JA, Radua J, Salavert J, Palomar G, Bosch R, et al. Brain abnormalities in adults with attention deficit hyperactivity disorder revealed by voxel-based morphometry. Psychiatry Res Neuroimaging. (2016) 254:41–7. doi: 10.1016/j.pscychresns.2016.06.002
50. Ramesh M, Rai KS. Region-wise gray matter volume alterations in brain of adolescents with attention deficit hyperactive disorder: a voxel based morphometric analysis. Indian J Physiol Pharmacol. (2013) 57:270–9.
51. Roman-Urrestarazu A, Lindholm P, Moilanen I, Kiviniemi V, Miettunen J, Jääskeläinen E, et al. Brain structural deficits and working memory fMRI dysfunction in young adults who were diagnosed with ADHD in adolescence. Eur Child Adolesc Psychiatry. (2016) 25:529–38. doi: 10.1007/s00787-015-0755-8
52. Sasayama D, Hayashida A, Yamasue H, Harada Y, Kaneko T, Kasai K, et al. Neuroanatomical correlates of attention-deficit-hyperactivity disorder accounting for comorbid oppositional defiant disorder and conduct disorder. Psychiatry Clin Neurosci. (2010) 64:394–402. doi: 10.1111/j.1440-1819.2010.02102.x
53. Sethi A, Evelyn-Rahr E, Dowell N, Jain S, Voon V, Critchley HD, et al. Magnetization transfer imaging identifies basal ganglia abnormalities in adult ADHD that are invisible to conventional T1 weighted voxel-based morphometry. Neuroimage Clin. (2017) 15:8–14. doi: 10.1016/j.nicl.2017.03.012
54. Shimada K, Fujisawa TX, Takiguchi S, Naruse H, Kosaka H, Okazawa H, et al. Ethnic differences in COMT genetic effects on striatal grey matter alterations associated with childhood ADHD: a voxel-based morphometry study in a Japanese sample. World J Biol Psychiatry. (2017) 18:322–8. doi: 10.3109/15622975.2015.1102325
55. van Wingen GA, van den Brink W, Veltman DJ, Schmaal L, Dom G, Booij J, et al. Reduced striatal brain volumes in non-medicated adult ADHD patients with comorbid cocaine dependence. Drug Alcohol Depend. (2013) 131:198–203. doi: 10.1016/j.drugalcdep.2013.05.007
56. Vilgis V, Sun L, Chen J, Silk TJ, Vance A. Global and local grey matter reductions in boys with ADHD combined type and ADHD inattentive type. Psychiatry Res Neuroimaging. (2016) 254:119–26. doi: 10.1016/j.pscychresns.2016.06.008
57. Villemonteix T, De Brito SA, Slama H, Kavec M, Balériaux D, Metens T, et al. Structural correlates of COMT Val158Met polymorphism in childhood ADHD: a voxel-based morphometry study. World J Biol Psychiatry. (2015) 16:190–9. doi: 10.3109/15622975.2014.984629
58. Wang L-J, Li S-C, Kuo H-C, Chou W-J, Lee M-J, Chou M-C, et al. Gray matter volume and microRNA levels in patients with attention-deficit/hyperactivity disorder. Eur Arch Psychiatry Clin Neurosci. (2020) 270:1037–45. doi: 10.1007/s00406-019-01032-x
59. Zhao Y, Cui D, Lu W, Li H, Zhang H, Qiu J. Aberrant gray matter volumes and functional connectivity in adolescent patients with ADHD. J Magn Reson Imaging. (2020) 51:719–26. doi: 10.1002/jmri.26854
60. Sáenz AA, Septier M, Van Schuerbeek P, Baijot S, Deconinck N, Defresne P, et al. ADHD and ASD: distinct brain patterns of inhibition-related activation? Transl Psychiatry. (2020) 10:24. doi: 10.1038/s41398-020-0707-z
61. Bédard A-CV, Newcorn JH, Clerkin SM, Krone B, Fan J, Halperin JM, et al. Reduced prefrontal efficiency for visuospatial working memory in attention-deficit/hyperactivity disorder. J Am Acad Child Adolesc Psychiatry. (2014) 53:1020–30 e6. doi: 10.1016/j.jaac.2014.05.011
62. Chantiluke K, Barrett N, Giampietro V, Santosh P, Brammer M, Simmons A, et al. Inverse fluoxetine effects on inhibitory brain activation in non-comorbid boys with ADHD and with ASD. Psychopharmacology. (2015) 232:2071–82. doi: 10.1007/s00213-014-3837-2
63. Chen C-Y, Yen J-Y, Yen C-F, Chen C-S, Liu G-C, Liang C-Y, et al. Aberrant brain activation of error processing among adults with attention deficit and hyperactivity disorder. Kaohsiung J Med Sci. (2015) 31:179–87. doi: 10.1016/j.kjms.2015.01.001
64. Christakou A, Murphy CM, Chantiluke K, Cubillo AI, Smith AB, Giampietro V, et al. Disorder-specific functional abnormalities during sustained attention in youth with attention deficit hyperactivity disorder (ADHD) and with autism Mol Psychiatry. (2013) 18:236–44. doi: 10.1038/mp.2011.185
65. Cubillo A, Halari R, Giampietro V, Taylor E, Rubia K. Fronto-striatal underactivation during interference inhibition and attention allocation in grown up children with attention deficit/hyperactivity disorder and persistent symptoms. Psychiatry Res. (2011) 193:17–27. doi: 10.1016/j.pscychresns.2010.12.014
66. Cubillo A, Smith AB, Barrett N, Giampietro V, Brammer MJ, Simmons A, et al. Shared and drug-specific effects of atomoxetine and methylphenidate on inhibitory brain dysfunction in medication-naive ADHD boys. Cereb Cortex. (2014) 24:174–85. doi: 10.1093/cercor/bhs296
67. Cubillo A, Halari R, Ecker C, Giampietro V, Taylor E, Rubia K. Reduced activation and inter-regional functional connectivity of fronto-striatal networks in adults with childhood attention-deficit hyperactivity disorder (ADHD) and persisting symptoms during tasks of motor inhibition and cognitive switching. J Psychiatr Res. (2010) 44:629–39. doi: 10.1016/j.jpsychires.2009.11.016
68. Dibbets P, Evers EAT, Hurks PPM, Bakker K, Jolles J. Differential brain activation patterns in adult attention-deficit hyperactivity disorder (ADHD) associated with task switching. Neuropsychology. (2010) 24:413–23. doi: 10.1037/a0018997
69. Fan L-Y, Shang C-Y, Tseng W-YI, Gau SS-F, Chou T-L. Visual processing as a potential endophenotype in youths with attention-deficit/hyperactivity disorder: a sibling study design using the counting Stroop functional MRI. Hum Brain Mapp. (2018) 39:3827–35. doi: 10.1002/hbm.24214
70. Fan LY, Gau SS, Chou TL. Neural correlates of inhibitory control and visual processing in youths with attention deficit hyperactivity disorder: a counting Stroop functional MRI study. Psychol Med. (2014) 44:2661–71. doi: 10.1017/S0033291714000038
71. Janssen TWP, Heslenfeld DJ, van Mourik R, Logan GD, Oosterlaan J. Neural correlates of response inhibition in children with attention-deficit/hyperactivity disorder: a controlled version of the stop-signal task. Psychiatry Res. (2015) 233:278–84. doi: 10.1016/j.pscychresns.2015.07.007
72. Kooistra L, van der Meere JJ, Edwards JD, Kaplan BJ, Crawford S, Goodyear BG. Preliminary fMRI findings on the effects of event rate in adults with ADHD. J Neural Transm. (2010) 117:655–62. doi: 10.1007/s00702-010-0374-y
73. Ma J, Lei D, Jin X, Du X, Jiang F, Li F, et al. Compensatory brain activation in children with attention deficit/hyperactivity disorder during a simplified Go/No-go task. J Neural Transm. (2012) 119:613–9. doi: 10.1007/s00702-011-0744-0
74. Ma I, van Holstein M, Mies GW, Mennes M, Buitelaar J, Cools R, et al. Ventral striatal hyperconnectivity during rewarded interference control in adolescents with ADHD. Cortex. (2016) 82:225–36. doi: 10.1016/j.cortex.2016.05.021
75. Materna L, Wiesner CD, Shushakova A, Trieloff J, Weber N, Engell A, et al. Adult patients with ADHD differ from healthy controls in implicit, but not explicit, emotion regulation. J Psychiatry Neurosci. (2019) 44:340–9. doi: 10.1503/jpn.180139
76. Mehren A, Özyurt J, Thiel CM, Brandes M, Lam AP, Philipsen A. Effects of acute aerobic exercise on response inhibition in adult patients with ADHD. Sci Rep. (2019) 9:19884. doi: 10.1038/s41598-019-56332-y
77. Passarotti AM, Sweeney JA, Pavuluri MN. Neural correlates of response inhibition in pediatric bipolar disorder and attention deficit hyperactivity disorder. Psychiatry Res. (2010) 181:36–43. doi: 10.1016/j.pscychresns.2009.07.002
78. Dibbets P, Evers L, Hurks P, Marchetta N, Jolles J. Differences in feedback- and inhibition-related neural activity in adult ADHD. Brain Cogn. (2009) 70:73–83. doi: 10.1016/j.bandc.2009.01.001
79. Pretus C, Picado M, Ramos-Quiroga A, Carmona S, Richarte V, Fauquet J, et al. Presence of distractor improves time estimation performance in an adult ADHD sample. J Atten Disord. (2020) 24:1530–7. doi: 10.1177/1087054716648776
80. Rasmussen J, Casey BJ, van Erp TGM, Tamm L, Epstein JN, Buss C, et al. ADHD and cannabis use in young adults examined using fMRI of a Go/NoGo task. Brain Imaging Behav. (2016) 10:761–71. doi: 10.1007/s11682-015-9438-9
81. Rubia K, Halari R, Cubillo A, Smith AB, Mohammad A-M, Brammer M, et al. Methylphenidate normalizes fronto-striatal underactivation during interference inhibition in medication-naive boys with attention-deficit hyperactivity disorder. Neuropsychopharmacology. (2011) 36:1575–86. doi: 10.1038/npp.2011.30
82. Rubia K, Halari R, Mohammad A-M, Taylor E, Brammer M. Methylphenidate normalizes frontocingulate underactivation during error processing in attention-deficit/hyperactivity disorder. Biol Psychiatry. (2011) 70:255–62. doi: 10.1016/j.biopsych.2011.04.018
83. Schulz KP, Bédard A-CV, Fan J, Clerkin SM, Dima D, Newcorn JH, et al. Emotional bias of cognitive control in adults with childhood attention-deficit/hyperactivity disorder. Neuroimage Clin. (2014) 5:1–9. doi: 10.1016/j.nicl.2014.05.016
84. Sebastian A, Gerdes B, Feige B, Klöppel S, Lange T, Philipsen A, et al. Neural correlates of interference inhibition, action withholding and action cancelation in adult ADHD. Psychiatry Res. (2012) 202:132–41. doi: 10.1016/j.pscychresns.2012.02.010
85. Shang C-Y, Sheng C, Yang L-K, Chou T-L, Gau SS-F. Differential brain activations in adult attention-deficit/ hyperactivity disorder subtypes: a counting Stroop functional MRI study. Brain Imaging Behav. (2018) 12:882–90. doi: 10.1007/s11682-017-9749-0
86. Siniatchkin M, Glatthaar N, von Müller GG, Prehn-Kristensen A, Wolff S, Knöchel S, et al. Behavioural treatment increases activity in the cognitive neuronal networks in children with attention deficit/hyperactivity disorder. Brain Topogr. (2012) 25:332–44. doi: 10.1007/s10548-012-0221-6
87. Spinelli S, Joel S, Nelson TE, Vasa RA, Pekar JJ, Mostofsky SH. Different neural patterns are associated with trials preceding inhibitory errors in children with and without attention-deficit/hyperactivity disorder. J Am Acad Child Adolesc Psychiatry. (2011) 50:705–15. e3. doi: 10.1016/j.jaac.2011.03.014
88. Tamm L, Juranek J. Fluid reasoning deficits in children with ADHD: evidence from fMRI. Brain Res. (2012) 1465:48–56. doi: 10.1016/j.brainres.2012.05.021
89. Tegelbeckers J, Bunzeck N, Duzel E, Bonath B, Flechtner H-H, Krauel K. Altered salience processing in attention deficit hyperactivity disorder. Hum Brain Mapp. (2015) 36:2049–60. doi: 10.1002/hbm.22755
90. Thornton S, Bray S, Langevin LM, Dewey D. Functional brain correlates of motor response inhibition in children with developmental coordination disorder and attention deficit/hyperactivity disorder. Hum Mov Sci. (2018) 59:134–42. doi: 10.1016/j.humov.2018.03.018
91. van Rooij D, Hartman CA, Mennes M, Oosterlaan J, Franke B, Rommelse N, et al. Altered neural connectivity during response inhibition in adolescents with attention-deficit/hyperactivity disorder and their unaffected siblings. Neuroimage Clin. (2015) 7:325–35. doi: 10.1016/j.nicl.2015.01.004
92. Vetter NC, Buse J, Backhausen LL, Rubia K, Smolka MN, Roessner V. Anterior insula hyperactivation in ADHD when faced with distracting negative stimuli. Hum Brain Mapp. (2018) 39:2972–86. doi: 10.1002/hbm.24053
93. Wang S, Yang Y, Xing W, Chen J, Liu C, Luo X. Altered neural circuits related to sustained attention and executive control in children with ADHD: an event-related fMRI study. Clin Neurophysiol. (2013) 124:2181–90. doi: 10.1016/j.clinph.2013.05.008
94. Yang D-Y, Chi MH, Chu C-L, Lin C-Y, Hsu S-E, Chen KC, et al. Orbitofrontal dysfunction during the reward process in adults with ADHD: an fMRI study. Clin Neurophysiol. (2019) 130:627–33. doi: 10.1016/j.clinph.2019.01.022
95. Zamorano F, Billeke P, Kausel L, Larrain J, Stecher X, Hurtado JM, et al. Lateral prefrontal activity as a compensatory strategy for deficits of cortical processing in attention deficit hyperactivity disorder. Sci Rep. (2017) 7:7181. doi: 10.1038/s41598-017-07681-z
96. Overmeyer S, Bullmore ET, Suckling J, Simmons A, Williams SC, Santosh PJ, et al. Distributed grey and white matter deficits in hyperkinetic disorder: MRI evidence for anatomical abnormality in an attentional network. Psychol Med. (2001) 31:1425–35. doi: 10.1017/S0033291701004706
97. Sowell ER, Thompson PM, Welcome SE, Henkenius AL, Toga AW, Peterson BS. Cortical abnormalities in children and adolescents with attention-deficit hyperactivity disorder. Lancet. (2003) 362:1699–707. doi: 10.1016/S0140-6736(03)14842-8
98. Zametkin AJ, Nordahl TE, Gross M, King AC, Semple WE, Rumsey J, et al. Cerebral glucose metabolism in adults with hyperactivity of childhood onset. N Engl J Med. (1990) 323:1361–6. doi: 10.1056/NEJM199011153232001
99. De La Fuente A, Xia S, Branch C, Li X. A review of attention-deficit/hyperactivity disorder from the perspective of brain networks. Front Hum Neurosci. (2013) 7:192. doi: 10.3389/fnhum.2013.00192
100. Hart H, Radua J, Nakao T, Mataix-Cols D, Rubia K. Meta-analysis of functional magnetic resonance imaging studies of inhibition and attention in attention-deficit/hyperactivity disorder: exploring task-specific, stimulant medication, and age effects. JAMA Psychiatry. (2013) 70:185–98. doi: 10.1001/jamapsychiatry.2013.277
101. Biederman J, Faraone SV. Attention-deficit hyperactivity disorder. Lancet. (2005) 366:237–48. doi: 10.1016/S0140-6736(05)66915-2
102. Arnsten AF. Fundamentals of attention-deficit/hyperactivity disorder: circuits and pathways. J Clin Psychiatry. (2006) 67(Suppl. 8):7–12.
103. Castellanos FX, Proal E. Large-scale brain systems in ADHD: beyond the prefrontal-striatal model. Trends Cogn Sci. (2012) 16:17–26. doi: 10.1016/j.tics.2011.11.007
104. Lopez-Larson M, Michael ES, Terry JE, Breeze JL, Hodge SM, Tang L, et al. Subcortical differences among youths with attention-deficit/hyperactivity disorder compared to those with bipolar disorder with and without attention-deficit/hyperactivity disorder. J Child Adolesc Psychopharmacol. (2009) 19:31–9. doi: 10.1089/cap.2008.041
105. Tamm L, Menon V, Reiss AL. Parietal attentional system aberrations during target detection in adolescents with attention deficit hyperactivity disorder: event-related fMRI evidence. Am J Psychiatry. (2006) 163:1033–43. doi: 10.1176/ajp.2006.163.6.1033
106. Vance A, Silk TJ, Casey M, Rinehart NJ, Bradshaw JL, Bellgrove MA, et al. Right parietal dysfunction in children with attention deficit hyperactivity disorder, combined type: a functional MRI study. Mol Psychiatry. (2007) 12:826–32, 793. doi: 10.1038/sj.mp.4001999
107. Zhang R, Geng X, Lee TMC. Large-scale functional neural network correlates of response inhibition: an fMRI meta-analysis. Brain Struct Funct. (2017) 222:3973–90. doi: 10.1007/s00429-017-1443-x
108. McAlonan GM, Cheung V, Chua SE, Oosterlaan J, Hung SF, Tang CP, et al. Age-related grey matter volume correlates of response inhibition and shifting in attention-deficit hyperactivity disorder. Br J Psychiatry. (2009) 194:123–9. doi: 10.1192/bjp.bp.108.051359
109. Carmona S, Hoekzema E, Ramos-Quiroga JA, Richarte V, Canals C, Bosch R, et al. Response inhibition and reward anticipation in medication-naive adults with attention-deficit/hyperactivity disorder: a within-subject case-control neuroimaging study. Hum Brain Mapp. (2012) 33:2350–61. doi: 10.1002/hbm.21368
110. Giedd JN, Castellanos FX, Casey BJ, Kozuch P, King AC, Hamburger SD, et al. Quantitative morphology of the corpus callosum in attention deficit hyperactivity disorder. Am J Psychiatry. (1994) 151:665–9. doi: 10.1176/ajp.151.5.665
111. Castellanos FX, Giedd JN, Marsh WL, Hamburger SD, Vaituzis AC, Dickstein DP, et al. Quantitative brain magnetic resonance imaging in attention-deficit hyperactivity disorder. Arch Gen Psychiatry. (1996) 53:607–16. doi: 10.1001/archpsyc.1996.01830070053009
112. Hill DE, Yeo RA, Campbell RA, Hart B, Vigil J, Brooks W. Magnetic resonance imaging correlates of attention-deficit/hyperactivity disorder in children. Neuropsychology. (2003) 17:496–506. doi: 10.1037/0894-4105.17.3.496
113. Valera EM, Faraone SV, Murray KE, Seidman LJ, et al. Meta-analysis of structural imaging findings in attention-deficit/hyperactivity disorder. Biol Psychiatry. (2007) 61:1361–9. doi: 10.1016/j.biopsych.2006.06.011
114. Hutchinson AD, Mathias JL, Banich MT. Corpus callosum morphology in children and adolescents with attention deficit hyperactivity disorder: a meta-analytic review. Neuropsychology. (2008) 22:341–9. doi: 10.1037/0894-4105.22.3.341
115. Dramsdahl M, Westerhausen R, Haavik J, Hugdahl K, Plessen KJ. Adults with attention-deficit/hyperactivity disorder - a diffusion-tensor imaging study of the corpus callosum. Psychiatry Res. (2012) 201:168–73. doi: 10.1016/j.pscychresns.2011.08.005
116. Naqvi NH, Bechara A. The insula and drug addiction: an interoceptive view of pleasure, urges, and decision-making. Brain Struct Funct. (2010) 214:435–50. doi: 10.1007/s00429-010-0268-7
117. Capotosto P, Babiloni C, Romani GL, Corbetta M. Frontoparietal cortex controls spatial attention through modulation of anticipatory alpha rhythms. J Neurosci. (2009) 29:5863–72. doi: 10.1523/JNEUROSCI.0539-09.2009
118. Shulman GL, Astafiev SV, Franke D, Pope DLW, Snyder AZ, McAvoy MP, et al. Interaction of stimulus-driven reorienting and expectation in ventral and dorsal frontoparietal and basal ganglia-cortical networks. J Neurosci. (2009) 29:4392–407. doi: 10.1523/JNEUROSCI.5609-08.2009
119. Cavanna AE, Trimble MR. The precuneus: a review of its functional anatomy and behavioural correlates. Brain. (2006) 129(Pt 3):564–83. doi: 10.1093/brain/awl004
120. Cao H. Prefrontal-cerebellar dynamics during post-success and post-error cognitive controls in major psychiatric disorders. Psychol Med. (2022). doi: 10.1017/S0033291722001829. [Epub ahead of print].
121. Fu Z, Yuan J, Pei X, Zhang K, Xu C, Hu N, et al. Shared and unique effects of long-term administration of methylphenidate and atomoxetine on degree centrality in medication-naïve children with attention-deficit/hyperactive disorder. Int J Neuropsychopharmacol. (2022) 25:709–19. doi: 10.1093/ijnp/pyac028
122. Castellanos FX, Lee PP, Sharp W, Jeffries NO, Greenstein DK, Clasen LS, et al. Developmental trajectories of brain volume abnormalities in children and adolescents with attention-deficit/hyperactivity disorder. JAMA. (2002) 288:1740–8. doi: 10.1001/jama.288.14.1740
123. Shaw P, Rabin C. New insights into attention-deficit/hyperactivity disorder using structural neuroimaging. Curr Psychiatry Rep. (2009) 11:393–8. doi: 10.1007/s11920-009-0059-0
124. Hoogman M, Bralten J, Hibar DP, Mennes M, Zwiers MP, Schweren LS, et al. Subcortical brain volume differences in participants with attention deficit hyperactivity disorder in children and adults: a cross-sectional mega-analysis. Lancet Psychiatry. (2017) 4:310–9. doi: 10.1016/S2215-0366(17)30049-4
125. Bramham J, Murphy DG, Xenitidis K, Asherson P, Hopkin G, Young S. Adults with attention deficit hyperactivity disorder: an investigation of age-related differences in behavioural symptoms, neuropsychological function and co-morbidity. Psychol Med. (2012) 42:2225–34. doi: 10.1017/S0033291712000219
Keywords: meta-analysis, ADHD, voxel-based morphometry, functional MRI, neuroimaging
Citation: Yu M, Gao X, Niu X, Zhang M, Yang Z, Han S, Cheng J and Zhang Y (2023) Meta-analysis of structural and functional alterations of brain in patients with attention-deficit/hyperactivity disorder. Front. Psychiatry 13:1070142. doi: 10.3389/fpsyt.2022.1070142
Received: 14 October 2022; Accepted: 05 December 2022;
Published: 06 January 2023.
Edited by:
Alessandra Carta, University of Sassari, ItalyReviewed by:
Silke Lux, University Hospital Bonn, GermanyMeihao Wang, First Affiliated Hospital of Wenzhou Medical University, China
Copyright © 2023 Yu, Gao, Niu, Zhang, Yang, Han, Cheng and Zhang. This is an open-access article distributed under the terms of the Creative Commons Attribution License (CC BY). The use, distribution or reproduction in other forums is permitted, provided the original author(s) and the copyright owner(s) are credited and that the original publication in this journal is cited, in accordance with accepted academic practice. No use, distribution or reproduction is permitted which does not comply with these terms.
*Correspondence: Yong Zhang, enp1emhhbmd5b25nMjAxM0AxNjMuY29t; Jingliang Cheng,
Y2pyLmNoamxAdmlwLjE2My5jb20=; Shaoqiang Han,
U2hhb3FpYW5nSGFuQDE2My5jb20=