- 1Peking University Huilongguan Clinical Medical School, Beijing Huilongguan Hospital, Beijing, China
- 2Department of Psychiatry, Yale University School of Medicine, New Haven, CT, United States
- 3Department of Neuroscience, Yale University School of Medicine, New Haven, CT, United States
Objective: Our study aimed to investigate the associations between the serum level of kynurenine pathway (KP) metabolites and P50 auditory gating in non-smoking patients with first-episode schizophrenia (FES).
Materials and methods: In this study, 82 non-smoking patients with FES and 73 healthy controls (HC). P50 auditory gating was measured using a fully functional digital 64-channel EEG system, and the components included S1 amplitude, S2 amplitude, gating ratio (S2/S1), and amplitude difference (S1–S2). Serum levels of kynurenine and kynurenine acid were assessed using a combination of liquid chromatography with tandem mass spectrometry. Psychopathology was assessed by the Positive and Negative Syndrome Scale (PANSS).
Results: The serum kynurenine (251.46 ± 65.93 ng/ml vs. 320.65 ± 65.89 ng/ml, t = –6.38, p < 0.001), and kynurenine acid levels (5.19 ± 2.22 ng/ml vs. 13.26 ± 4.23 ng/ml, t = –14.73, p < 0.001), S1 amplitude [2.88 (1.79, 3.78) μV vs. 3.08 (2.46, 4.56) μV, Z = –2.17, p = 0.030] and S1–S2 [1.60 (0.63, 2.49) μV vs. 1.92 (1.12, 2.93) μV, Z = –2.23, p = 0.026] in patients with FES were significantly lower than those in HC. The serum kynurenine and kynurenine acid levels were negatively associated with S1–S2 (r = –0.32, p = 0.004 and r = –0.42, p < 0.001; respectively) and positively correlated with S2/S1 ratio (r = 0.34, p = 0.002 and r = 0.35, p = 0.002; respectively) in patients.
Conclusion: Our findings suggested that neuroactive metabolites of the KP might play an important role in sensory gating deficit in first episode patients with schizophrenia. Furthermore, metabolites of the KP may be a new target for the treatment of cognitive impairments in schizophrenia.
Introduction
Cognitive impairment is one of the core symptoms of schizophrenia that mostly leads to social disabilities (1, 2) and determines the prognosis of the disease (3). As an essential component of human cognitive function, sensory gating refers to the neurological process of filtering out redundant or unnecessary sensory input during information processing (4), its dysfunction has been frequently observed in patients with schizophrenia (5). Individuals with schizophrenia show sensory gating deficits, which may be associated with impaired sustained attention (6), slowed processing speed (7), and poor working memory performance (7, 8).
Some studies suggested that sensory gating deficits might be associated with schizophrenia symptom severity (5). However, a systematic review reported that cognitive impairment was presented before the onset of first-episode schizophrenia (FES) and persisted even if psychotic symptoms have been normalized (9). Furthermore, previous studies have shown that sensory gating deficit exacerbated schizophrenia symptoms (10, 11). Sensory gating deficit, usually reflected by P50 suppression, is a sensitive biological marker for identifying schizophrenia (12, 13). However, these arguments have mainly been based on studies conducted in antipsychotic medication patients. Although there is some evidence supporting sensory gating deficits in individuals with schizophrenia, further studies are needed to assess the deficits according to different stages of the disease, symptom severity, treatment, and other possible confounders, such as smoking.
Growing evidence indicated that dysregulation of kynurenine pathway (KP) metabolites might play an important role in the pathophysiology of schizophrenia (14). Kynurenine (KYN) is a tryptophan metabolite that can cross the blood-brain barrier (BBB). Kynurenic acid (KYNA), a product of the KYN pathway of tryptophan metabolism, may cause glutamate hypofunction through sustained N-methyl D-aspartate (NMDA) receptor antagonism, which may cause psychotic symptoms and cognitive deficits in people with schizophrenia (15, 16). Interestingly, due to its antagonistic action on NMDA receptors, effective antioxidant properties and hydroxyl radical scavenging capacity, a highly elevated level of KYNA has also been found to have neuroprotection potential in experimental animals (17, 18). In contrast to the replicable findings that cerebrospinal fluid (CSF) levels of KYN and KYNA were significantly higher in patients with schizophrenia than in healthy controls (HC) (19), either increase (20) or decrease (21) in their serum and plasma levels has been found in patients with schizophrenia compared with that in controls. In addition, Shovestul et al. (22) found no differences in serum KYN and KYNA levels between patients with schizophrenia and controls.
As a glutamate receptor antagonist, KYNA is reported to inhibit α7-nicotinic acetylcholine receptors (α7nAChRs) and may also act on targets downstream of α7nAChRs function (23). Recent studies have suggested that deficits in auditory gating are associated with α7nAChRs dysfunction (24). Preclinical research has revealed a link between elevated brain KYNA levels and disruption of auditory gating in adult rodents (25, 26). However, correlations between auditory gating deficits and serum KYN and KYNA levels in people with schizophrenia remain unclear.
The aims of this study included, (1) to compare P50 auditory gating and serum KYN and KYNA levels between non-smoking patients with FES and HC; (2) to explore the association between P50 auditory gating and serum KYN and KYNA levels in patients with FES and HC, respectively. Our findings might provide evidence for understanding of neurobiological mechanisms and the treatment of schizophrenia.
Materials and methods
Participants
Eighty-two non-smoking patients with FES were enrolled from Beijing Huilongguan Hospital. All patients met the following inclusion criteria: Diagnostic and Statistical Manual of Mental Disorders fourth edition (DSM-IV), criteria for schizophrenia (< 3 years of illness duration); between 18 and 45 years; treated with antipsychotic medication for less than 2 weeks; and no major physical illness. Seventy-three HC were recruited through advertising from the local community and screened by a trained psychiatrist, excluding those with major psychiatric disorders, personality disorders, substance abuse, major medical conditions, and a history of traumatic brain injury. All participants had normal hearing acuity (subjective hearing threshold of 40 dB) and were non-smokers, given that hearing acuity and smoking may affect auditory sensory gating and the kynurenine pathway, respectively (27). All participants were Han Chinese. This study was approved by the Research and Ethics Committee of Beijing Huilongguan Hospital. All eligible participants signed consent forms and were informed of their right to withdraw consent at any time.
Measures
P50 testing
The electroencephalography signals were recorded using a fully functional digital 64-channel electroencephalography system (Brain Products, Germany). We assessed sensory gating by recording P50 waves of auditory evoked responses in the conditioned reflex test paradigm. The test was conducted in a shielded sound-insulating room. The participants sat down and were asked to relax their entire body but remained awake and focused while awaiting stimulation.
Sensory gating is usually evaluated using neurophysiological approaches in the auditory double-click paradigm (28). The double-click paradigm involves two auditory stimuli, 500 ms apart, to measure the amplitude response. Data from the apex are reported because this is the best site to distinguish patients with schizophrenia from healthy participants when using this electrode array (29).
P50 inhibition was recorded in the conditional test mode. According to the international electroencephalography 10/20 system, the recording electrode is placed in the central midline, the reference electrode is placed in the right ear, the forehead is grounded, and the impedance between the electrodes is set at < 5 K. The analysis time was 600 ms, non-target stimulation frequency was 1,000 Hz, and rule intensity was 80 dB. The target stimulation frequency was 4,000 Hz, probability was 20%, and intensity was 90 dB. It appears randomly and is mixed with non-target stimulation.
The regulatory P50 wave (S1) was the most positive peak between 40 and 90 ms after regulatory stimulation. The test P50 wave (S2) was determined as the maximum peak after test stimulation, which was closest to the regulated P50 wave during the incubation period. In the present study, P50 auditory gating components included the S1 amplitude, S2 amplitude, amplitude difference (S1–S2), and gating ratio (S2/S1) (5). S1 is an individual’s response to normal auditory stimuli, whereas S2 refers to the ability to suppress non-target stimuli in the presence of S1. The relative decrease in the P50 waveform from the first click (S1) to the second (S2) is typically used as an indicator of P50 auditory gating and quantified as the S1–S2 amplitude difference or the S2/S1 ratio. A lower ratio or higher P50 difference indicated stronger sensory gating (30).
Serum kynurenine and kynurenic acid levels
Blood samples were collected between 7 a.m. and 8 a.m. after overnight fasting, centrifuged at 3,000 rpm at 4°C for 10 min, and then the serum was separated and stored at –80°C. Serum KYN and KYNA levels were measured using high performance liquid chromatography and tandem mass spectrometry, and then quantified using standard protocols. Concentrations were calculated from the peak areas. The identity of the participants was coded and saved by the investigator until all biochemical analyses were completed.
Positive and Negative Syndrome Scale
Psychotic symptoms were assessed using the Positive and Negative Syndrome Scale (PANSS) (31). The PANSS is a 30-item rating assessment used to evaluate the severity of schizophrenia symptoms and contains three subscales: positive, negative, and general psychopathology. The raters were trained and showed good interrater reliability (intraclass correlation coefficient ≥ 0.75).
Statistical analysis
Comparisons of serum levels of KYN and KYNA between patients with FES and HC were conducted using t-test and analysis of covariance (ANCOVA). Age and sex were controlled as possible confounding variables in the ANCOVA. Since the data of P50 parameters (S1 amplitude, S2 amplitude, S1–S2, and S2/S1 ratio) were highly skewed, the Mann–Whitney U-test was performed to compare differences between patients and HC. The χ2 test was performed to examine differences in categorical variables between the two groups.
Spearman’s correlations were used to test the associations between P50 parameters, serum KYN and KYNA levels, and the PANSS score in the patient group. We used spearman’s correlations to analyze the relationships between P50 parameters and serum KYN and KYNA levels in HC. Pearson’s correlations were used to analyze the associations between serum KYN and KYNA levels and PANSS scores. Two-tailed tests were performed for the analyses, with the significance level set at 0.05.
Results
Baseline clinical and demographic participant characteristics
Table 1 summarizes the demographic and clinical characteristics of all participants. There were no significant differences in age, sex, or educational level between the patient and control groups (p > 0.05). After inclusion in the study, 76 patients (92%) commenced treatment with antipsychotics (2 with first-generation and 74 with second-generation antipsychotics).
Comparisons of P50 parameters (S1, S2, S1–S2, and S2/S1) and serum kynurenine and kynurenic acid levels between patient and control groups
The S1 amplitude and amplitude difference (S1–S2) were significantly lower in the patient group than in the control group (Z = –2.17, p = 0.030 and Z = –2.23, p = 0.026; respectively), while there was no difference in S2 amplitude and S2/S1 ratio between the two groups. Compared with the control group, patients had lower serum levels of KYN (t = –6.38, p < 0.001) and KYNA (t = –14.73, p < 0.001). These significant differences were still observed in ANCOVA analyses after controlling for age and sex (Table 2).
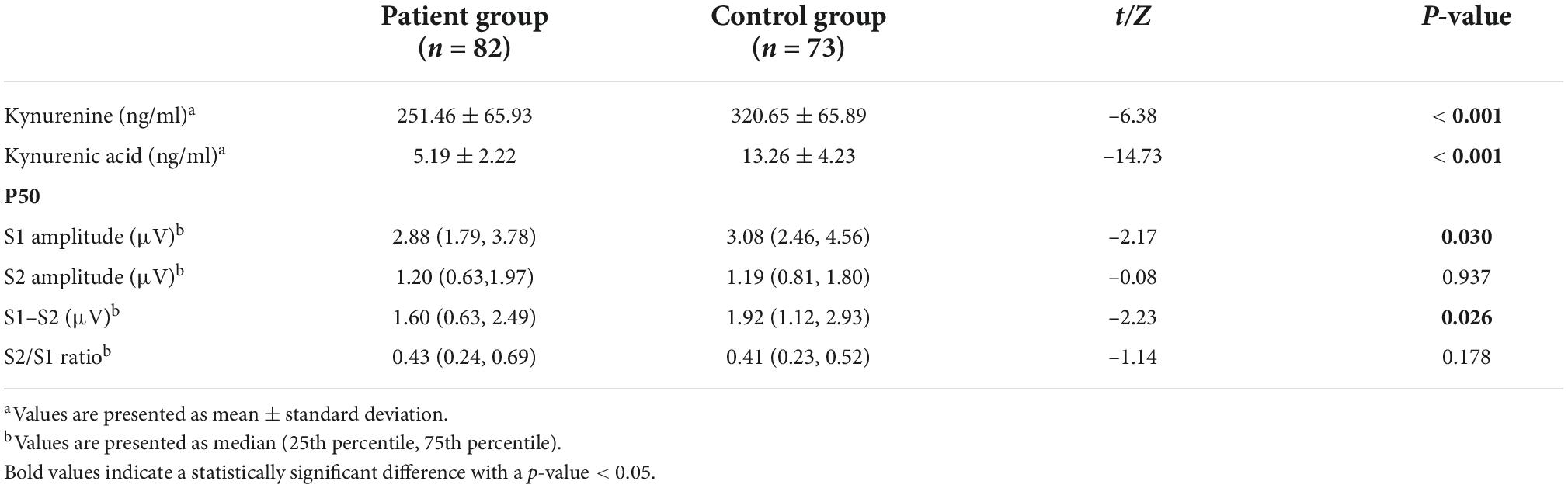
Table 2. Comparisons of P50 parameters and serum levels of KYN and KYNA between patients and healthy controls.
Correlations between the P50 parameters (S1, S2, S1–S2, and S2/S1), serum kynurenine and kynurenic acid levels, and psychotic symptoms
In the patient group, the serum KYN levels was positively associated with S2 amplitude (r = 0.24, p = 0.038; Table 3) and S2/S1 ratio (r = 0.34, p = 0.002; Figure 1), but negatively associated with amplitude difference (S1–S2) (r = –0.32, p = 0.004; Figure 2). There was no significant association between S1 amplitude and serum KYN levels (Table 3).
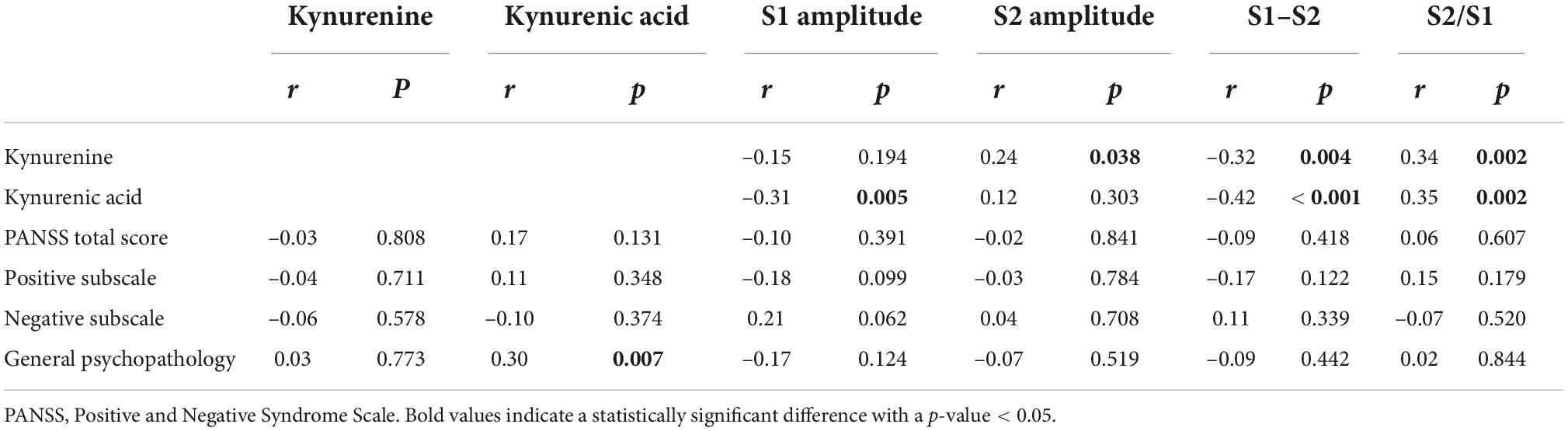
Table 3. Correlations between P50 parameters, the serum levels of KYN and KYNA as well as the severity of symptoms in the patient group.
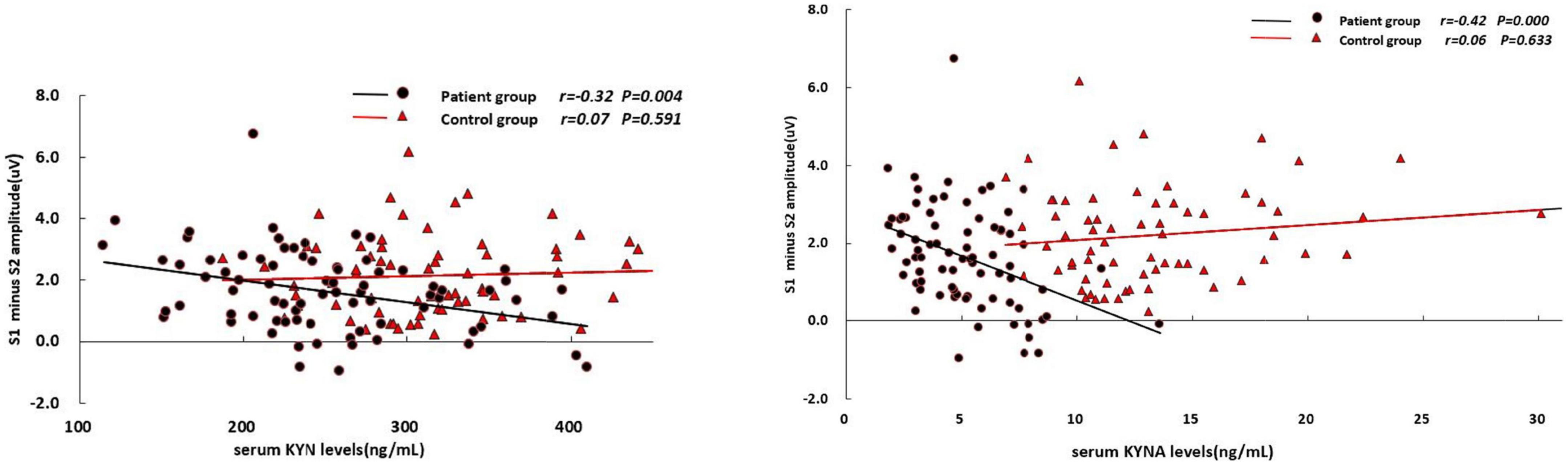
Figure 2. Correlations between S1–S2 amplitude difference and the serum levels of KYN and KYNA, respectively.
The serum KYNA level was positively associated with S2/S1 (r = 0.35, p = 0.002; Figure 1), but negatively associated with amplitude S1 (r = –0.31, p = 0.005; Table 3) and amplitude difference (S1–S2) (r = –0.42, p < 0.001; Figure 2). There was no significant correlation between serum KYNA level and amplitude S2 (Table 3).
Neither the P50 parameters nor serum KYN level was significantly associated with positive, negative, or general pathological symptoms. The serum KYNA level was significantly correlated with general pathological symptoms but not with positive or negative symptoms. P50 parameters and serum KYN and KYNA levels were not associated with antipsychotic status.
In the control group, none of the P50 parameters were significantly associated with serum levels of KYN or KYNA.
Discussion
This study was designed to examine presence of the deficits in sensory gating and its association with kynurenine pathway metabolites among non-smoking patients with FES. Moreover, this study investigated the relationships between sensory gating deficits and serum KYN and KYNA. In the present study, people with FES showed lower levels of amplitude S1 and amplitude difference (S1–S2) than HC, whereas no differences were found in S2 or S2/S1 ratio between the two groups. Serum levels of KYN and KYNA were lower in the patient group than in the control group. Furthermore, the higher the serum levels of KYN and KYNA, the greater the impairment in sensory gating would be in patients with FES.
Our findings are consistent with those of previous studies on impaired P50 auditory gating in patients with FES (32). Specifically, we found that patients with FES had abnormal responses to the first stimuli and failure suppression of the S2 response (S1–S2), whereas no impairments were observed in the S2 and S2/S1 ratios. These findings are supported by previous research on sensory gating deficits in Chinese patients with FES (5). Compared with HC, patients showed lower S1 amplitude, which may be explained by less sensitivity to novel sound stimuli and, therefore, decreased reactivity. P50 sensory gating reflects a predominantly pre-attentional filtering mechanism that protects higher-order cognitive functions (33). Some researchers have proposed that the S1 amplitude largely determines the differences in sensory gating between patients and controls (34). A lower P50 amplitude difference (S1–S2) that has been suggested to be the most reliable index of sensory gating (35), represents a weaker suppression of an evoked response. The impaired mechanisms of sensory gating can result in overflow of irrelevant sensory input and may be associated with thought disorders and cognitive fragmentation (32). Our findings confirm that sensory gating is impaired in the early stages of schizophrenia (36). Previous studies have frequently reported an elevated P50 gating ratio (S2/S1) in patients with prodromal and FES (37). However, the reliability of the suppression ratio can be determined by the correlation between S1 and S2 (38). Although some studies (39) have demonstrated that more severe negative symptoms are related to more sensory gating deficits in schizophrenia, we failed to find any associations between P50 parameters and psychiatric symptoms.
Elevated levels of KYN and KYNA in the CSF and blood have often been reported in patients with schizophrenia, with or without antipsychotic treatment (20). Conversely, the present study found that the serum KYN and KYNA levels were significantly lower in patients than in HC. Our findings are in accordance with those of studies on individuals with first-episode and antipsychotic-naïve schizophrenia (40). Similarly, Szymona et al. (41) found reduced serum KYNA levels in patients with schizophrenia during acute relapse and after a 4-week treatment with neuroleptics. Antipsychotics have been found to possibly reduce peripheral KYN and KYNA in medication-naïve or medication-free patients with schizophrenia (42). In the present study, more than 90% of the patients were taking antipsychotic medication after they entered the study, which may have affected the results.
Previous studies (43) have found that inhibition of KYNA synthesis is associated with increased extracellular dopamine (DA) levels. The decreased KYNA level may lead to activation of α7nAChRs, which can trigger an increase in glutamate and DA release. On the other hand, elevated DA concentration may reduce KYNA production by interacting with astrocytic DA receptors (43, 44). Therefore, decreased levels of KYN and KYNA might represent a possible feedback mechanism following increased DA neurotransmission in schizophrenia (45). A meta-analysis (20) suggested elevated KYNA levels in patients with schizophrenia when measured in the central nervous system rather than in the periphery. Additionally, factors such as patients’ age, sex and antipsychotic treatment may affect the expression of KYN and KYNA in serum. In the present study, patients were in their late 20 s and had a mean illness duration of 6 months. The mean PANSS total score (76.07 ± 11.93) indicated that they were moderately to markedly ill (46). A recent meta-analysis (47) showed that the peripheral KYNA level was significantly decreased in patients with schizophrenia spectrum disorders, particularly those with acute and severe symptoms. Our results revealed that a higher serum level of KYNA was associated with more severe general psychopathology; however, no correlation was found between the serum levels of KYN and KYNA and age, sex, or other PANSS dimensions.
The associations between serum levels of KYN and KYNA and P50 parameters existed only in the patient group. In agreement with previous preclinical and clinical studies (48–50), our study showed that among non-smoking patients with FES, higher levels of KYN and KYNA were associated with more impairments in auditory gating, namely decreased amplitude differences and a higher P50 auditory gating ratio. KYNA is widely considered neuroprotective because of its capacity to block neuronal excitation (48); however, abnormally increased KYNA levels may play a role in impaired attention and vigilance among people with schizophrenia (49, 50). For example, a fourfold increase in brain KYNA levels was associated with reduced prepulse inhibition in rats, an effect mimicking clinical sensory gating impairment (26). Previous research has postulated that KYNA is a non-competitive antagonist of α7nAChRs (51). Furthermore, combined cholinergic treatment with galantamine, a positive allosteric modulator of α7nAChRs, increased and prolonged nicotinic receptor activity, alleviating P50 auditory gating impairments. These findings suggest that sensory gating deficits may be associated with α7nAChRs dysfunction (24). Hence, the association between serum levels of KYN and KYNA and P50 impairments might be mediated by α7nAChRs dysfunction. As a significant molecular and neuropharmacological target, α7nAChRs have been found to improve learning, memory, and attentional mechanisms. Moreover, they are essential modulators of sensory gating (52). Our findings suggest that treatment regulating the NMDAergic system and targeting α7nAChRs could potentially improve auditory sensory gating and cognitive function in people with schizophrenia (53).
This study design has several limitations. First, the cross-sectional design limited the inference of causality. Prospective and longitudinal studies should be conducted to clarify the causal relationship between kynurenine pathway metabolites and P50 sensory gating. Second, most patients began using antipsychotic treatment after entering this study, which may have affected kynurenine pathway metabolites and sensory gating function. However, antipsychotic status was not associated with KYN and KYNA levels or with P50 parameters in this study. Third, many unmeasured potential variables, such as distress intolerance (54) and childhood trauma (22), may influence serum levels of KYN and KYNA or sensory gating. Fourth, given our small sample size and non-normally distributed data, we were not able to conduct analyses of the functional relationship between sensory gating and serum levels of KYN and KYNA.
Conclusion
To the best of our knowledge, the present study is the first to examine serum kynurenine metabolites and P50 sensory gating, as well as their association in non-smoking patients with FES. Compared to HC, patients with FES have sensory gating deficits showing lower S1 amplitudes, amplitude differences, and serum levels of KYN and KYNA. Higher serum KYN and KYNA levels were associated with more severe P50 gating deficits. Treatments targeting the kynurenine pathway, α7 nicotinic and NMDA receptors may improve the regulation of sensory gating performance in patients with FES.
Data availability statement
The data that support the findings of this study are available at: https://www.jianguoyun.com/p/DV5YmLEQt66GCxi7xN8EIAA, further requests can be directed to yltan21@126.com.
Ethics statement
The studies involving human participants were reviewed and approved by the Research and Ethics Committee of Beijing Huilongguan Hospital. The patients/participants provided their written informed consent to participate in this study.
Author contributions
YT: concept and design, integrity of the data, accuracy of data analysis, full access to all data in the study, administrative, technical, and material support. QY and YZ: drafting of the manuscript. XL, ST, C-SL, and YT: critically revise manuscripts of important intellectual content. QY, YZ, FF, and YT: statistical analysis. YZ, SC, and YT: obtain the funding. All authors contributed to the acquisition, analysis, and interpretation of data.
Funding
This study was funded by the National Natural Science Foundation of China (82171507 and 82001415) and Beijing Municipal Administration of Hospitals incubating Program (PX2019072).
Acknowledgments
We are greatly indebted to all study participants.
Conflict of interest
The author declares that the research was conducted in the absence of any commercial or financial relationships that could be construed as a potential conflict of interest.
The reviewer WY declared a shared parent affiliation with several of the authors YT, QY, YZ, KY, YN, FF, SC, ST, ZW, JT, and FY to the handling editor at the time of the review.
Publisher’s note
All claims expressed in this article are solely those of the authors and do not necessarily represent those of their affiliated organizations, or those of the publisher, the editors and the reviewers. Any product that may be evaluated in this article, or claim that may be made by its manufacturer, is not guaranteed or endorsed by the publisher.
Abbreviations
KP, kynurenine pathway; KYN, kynurenine; KYNA, kynurenic acid; FES, first-episode schizophrenia; NMDA, N-methylD-aspartate; CSF, cerebrospinal fluid; α 7nAChRs, α 7-nicotinic acetylcholine receptors; PANSS, Positive and Negative Syndrome Scale; ANCOVA, analysis of covariance.
References
1. Harvey PD, Green MF, Keefe RS, Velligan DI. Cognitive functioning in schizophrenia: a consensus statement on its role in the definition and evaluation of effective treatments for the illness. J Clin Psychiatry. (2004) 65:361–72. doi: 10.4088/JCP.v65n0312
2. Marder SR, Cannon TD. Schizophrenia. N Engl J Med. (2019) 381:1753–61. doi: 10.1056/NEJMra1808803
3. Kaneda Y, Jayathilak K, Meltzer HY. Determinants of work outcome in schizophrenia and schizoaffective disorder: role of cognitive function. Psychiatry Res. (2009) 169:178–9. doi: 10.1016/j.psychres.2008.08.003
4. Boutros NN, Zouridakis G, Overall J. Replication and extension of P50 findings in schizophrenia. Clin Electroencephalogr. (1991) 22:40–5. doi: 10.1177/155005949102200109
5. Xia L, Wang D, Wei G, Wang J, Zhou H, Xu H, et al. P50 inhibition defects with psychopathology and cognitive impairment in patients with first-episode drug naïve schizophrenia. Prog Neuropsychopharmacol Biol Psychiatry. (2021) 107:110246. doi: 10.1016/j.pnpbp.2021.110246
6. Toyomaki A, Hashimoto N, Kako Y, Tomimatsu Y, Koyama T, Kusumi I. Different P50 sensory gating measures reflect different cognitive dysfunctions in schizophrenia. Schizophr Res Cogn. (2015) 2:166–9. doi: 10.1016/j.scog.2015.07.002
7. Hamilton HK, Williams TJ, Ventura J, Jasperse LJ, Owens EM, Miller GA, et al. Clinical and cognitive significance of auditory sensory processing deficits in schizophrenia. Am J Psychiatry. (2018) 175:275–83. doi: 10.1176/appi.ajp.2017.16111203
8. Smith AK, Edgar JC, Huang M, Lu BY, Thoma RJ, Hanlon FM, et al. Cognitive abilities and 50- and 100-msec paired-click processes in schizophrenia. Am J Psychiatry. (2010) 167:1264–75. doi: 10.1176/appi.ajp.2010.09071059
9. Bhat PS, Raj J, Chatterjee K, Srivastava K. Cognitive dysfunction in first-episode schizophrenia and its correlation with negative symptoms and insight. Ind Psychiatry J. (2021) 30:310–5. doi: 10.4103/ipj.ipj_107_20
10. Johannesen JK, O’Donnell BF, Shekhar A, McGrew JH, Hetrick WP. Diagnostic specificity of neurophysiological endophenotypes in schizophrenia and bipolar disorder. Schizophr Bull. (2013) 39:1219–29. doi: 10.1093/schbul/sbs093
11. San-Martin R, Castro LA, Menezes PR, Fraga FJ, Simões PW, Salum C. Meta-analysis of sensorimotor gating deficits in patients with schizophrenia evaluated by prepulse inhibition test. Schizophr Bull. (2020) 46:1482–97. doi: 10.1093/schbul/sbaa059
12. Adler LE, Olincy A, Cawthra EM, McRae KA, Harris JG, Nagamoto HT, et al. Varied effects of atypical neuroleptics on P50 auditory gating in schizophrenia patients. Am J Psychiatry. (2004) 161:1822–8. doi: 10.1176/ajp.161.10.1822
13. Mao Q, Tan YL, Luo XG, Tian L, Wang ZR, Tan SP, et al. Association of catechol-O-methyltransferase Val(108/158) Met genetic polymorphism with schizophrenia, P50 sensory gating, and negative symptoms in a Chinese population. Psychiatry Res. (2016) 242:271–6. doi: 10.1016/j.psychres.2016.04.029
14. Chen Y, Guillemin GJ. Kynurenine pathway metabolites in humans: disease and healthy States. Int J Tryptophan Res. (2009) 2:1–19. doi: 10.4137/IJTR.S2097
15. Chess AC, Simoni MK, Alling TE, Bucci DJ. Elevations of endogenous kynurenic acid produce spatial working memory deficits. Schizophr Bull. (2007) 33:797–804. doi: 10.1093/schbul/sbl033
16. Wonodi I, Stine OC, Sathyasaikumar KV, Roberts RC, Mitchell BD, Hong LE, et al. Downregulated kynurenine 3-monooxygenase gene expression and enzyme activity in schizophrenia and genetic association with schizophrenia endophenotypes. Arch Gen Psychiatry. (2011) 68:665–74. doi: 10.1001/archgenpsychiatry.2011.71
17. Gigler G, Szénási G, Simó A, Lévay G, Hársing LG Jr., Sas K, et al. Neuroprotective effect of L-kynurenine sulfate administered before focal cerebral ischemia in mice and global cerebral ischemia in gerbils. Eur J Pharmacol. (2007) 564:116–22. doi: 10.1016/j.ejphar.2007.02.029
18. Bratek-Gerej E, Ziembowicz A, Godlewski J, Salinska E. The mechanism of the neuroprotective effect of kynurenic acid in the experimental model of neonatal hypoxia-ischemia: the link to oxidative stress. Antioxidants. (2021) 10:1775. doi: 10.3390/antiox10111775
19. Almulla AF, Vasupanrajit A, Tunvirachaisakul C, Al-Hakeim HK, Solmi M, Verkerk R, et al. The tryptophan catabolite or kynurenine pathway in schizophrenia: meta-analysis reveals dissociations between central, serum, and plasma compartments. Mol Psychiatry. (2022). [Epub ahead of print]. doi: 10.1038/s41380-022-01552-4
20. Plitman E, Iwata Y, Caravaggio F, Nakajima S, Chung JK, Gerretsen P, et al. Kynurenic acid in schizophrenia: a systematic review and meta-analysis. Schizophr Bull. (2017) 43:764–77. doi: 10.1093/schbul/sbw221
21. Wurfel BE, Drevets WC, Bliss SA, McMillin JR, Suzuki H, Ford BN, et al. Serum kynurenic acid is reduced in affective psychosis. Transl Psychiatry. (2017) 7:e1115. doi: 10.1038/tp.2017.88
22. Shovestul BJ, Glassman M, Rowland LM, McMahon RP, Liu F, Kelly DL. Pilot study examining the relationship of childhood trauma, perceived stress, and medication use to serum kynurenic acid and kynurenine levels in schizophrenia. Schizophr Res. (2017) 185:200–1. doi: 10.1016/j.schres.2016.12.026
23. Albuquerque EX, Schwarcz R. Kynurenic acid as an antagonist of α7 nicotinic acetylcholine receptors in the brain: facts and challenges. Biochem Pharmacol. (2013) 85:1027–32. doi: 10.1016/j.bcp.2012.12.014
24. Choueiry J, Blais CM, Shah D, Smith D, Fisher D, Illivitsky V, et al. Combining CDP-choline and galantamine: effects of a selective α7 nicotinic acetylcholine receptor agonist strategy on P50 sensory gating of speech sounds in healthy volunteers. J Psychopharmacol. (2019) 33:688–99. doi: 10.1177/0269881119836217
25. Shepard PD, Joy B, Clerkin L, Schwarcz R. Micromolar brain levels of kynurenic acid are associated with a disruption of auditory sensory gating in the rat. Neuropsychopharmacology. (2003) 28:1454–62. doi: 10.1038/sj.npp.1300188
26. Erhardt S, Schwieler L, Emanuelsson C, Geyer M. Endogenous kynurenic acid disrupts prepulse inhibition. Biol Psychiatry. (2004) 56:255–60. doi: 10.1016/j.biopsych.2004.06.006
27. Mathai AJ, Kanwar J, Okusaga O, Fuchs D, Lowry CA, Peng X, et al. Blood levels of monoamine precursors and smoking in patients with Schizophrenia. Front Public Health. (2016) 4:182. doi: 10.3389/fpubh.2016.00182
28. Smucny J, Olincy A, Eichman LC, Lyons E, Tregellas JR. Early sensory processing deficits predict sensitivity to distraction in schizophrenia. Schizophr Res. (2013) 147:196–200. doi: 10.1016/j.schres.2013.03.025
29. Clementz BA, Geyer MA, Braff DL. Multiple site evaluation of P50 suppression among schizophrenia and normal comparison subjects. Schizophr Res. (1998) 30:71–80. doi: 10.1016/S0920-9964(97)00122-9
30. Freedman R, Olsen-Dufour AM, Olincy A. P50 inhibitory sensory gating in schizophrenia: analysis of recent studies. Schizophr Res. (2020) 218:93–8. doi: 10.1016/j.schres.2020.02.003
31. Kay SR, Fiszbein A, Opler LA. The positive and negative syndrome scale (PANSS) for schizophrenia. Schizophr Bull. (1987) 13:261–76. doi: 10.1093/schbul/13.2.261
32. Atagun MI, Drukker M, Hall MH, Altun IK, Tatli SZ, Guloksuz S, et al. Meta-analysis of auditory P50 sensory gating in schizophrenia and bipolar disorder. Psychiatry Res Neuroimaging. (2020) 300:111078. doi: 10.1016/j.pscychresns.2020.111078
33. Wan L, Friedman BH, Boutros NN, Crawford HJ. P50 sensory gating and attentional performance. Int J Psychophysiol. (2008) 67:91–100. doi: 10.1016/j.ijpsycho.2007.10.008
34. Clementz BA, Blumenfeld LD. Multichannel electroencephalographic assessment of auditory evoked response suppression in schizophrenia. Exp Brain Res. (2001) 139:377–90. doi: 10.1007/s002210100744
35. Owens EM, Bachman P, Glahn DC, Bearden CE. Electrophysiological Endophenotypes for Schizophrenia. Harv Rev Psychiatry. (2016) 24:129–47. doi: 10.1097/HRP.0000000000000110
36. Morales-Muñoz I, Jurado-Barba R, Fernández-Guinea S, Rodríguez-Jiménez R, Jiménez-Arriero MÁ, Criado JR, et al. Sensory gating deficits in first-episode psychosis: evidence from neurophysiology, psychophysiology, and neuropsychology. J Nerv Ment Dis. (2016) 204:877–84. doi: 10.1097/NMD.0000000000000572
37. Brockhaus-Dumke A, Schultze-Lutter F, Mueller R, Tendolkar I, Bechdolf A, Pukrop R, et al. Sensory gating in schizophrenia: P50 and N100 gating in antipsychotic-free subjects at risk, first-episode, and chronic patients. Biol Psychiatry. (2008) 64:376–84. doi: 10.1016/j.biopsych.2008.02.006
38. Alferness C, Bayly PV, Krassowska W, Daubert JP, Smith WM, Ideker RE. Strength-interval curves in canine myocardium at very short cycle lengths. Pacing Clin Electrophysiol. (1994) 17:876–81. doi: 10.1111/j.1540-8159.1994.tb01428.x
39. Louchart-de la Chapelle S, Levillain D, Ménard JF, Van der Elst A, Allio G, Haouzir S, et al. P50 inhibitory gating deficit is correlated with the negative symptomatology of schizophrenia. Psychiatry Res. (2005) 136:27–34. doi: 10.1016/j.psychres.2003.04.001
40. Leppik L, Kriisa K, Koido K, Kajalaid K, Haring L, Vasar E, et al. Profiling of amino acids and their derivatives biogenic amines before and after antipsychotic treatment in first-episode psychosis. Front Psychiatry. (2018) 9:155. doi: 10.3389/fpsyt.2018.00155
41. Szymona K, Zdzisińska B, Karakuła-Juchnowicz H, Kocki T, Kandefer-Szerszeń M, Flis M, et al. Correlations of Kynurenic Acid, 3-Hydroxykynurenine, sIL-2R, IFN-α, and IL-4 with Clinical Symptoms During Acute Relapse of Schizophrenia. Neurotox Res. (2017) 32:17–26. doi: 10.1007/s12640-017-9714-0
42. Condray R, Dougherty GG Jr., Keshavan MS, Reddy RD, Haas GL, Montrose DM, et al. 3-Hydroxykynurenine and clinical symptoms in first-episode neuroleptic-naive patients with schizophrenia. Int J Neuropsychopharmacol. (2011) 14:756–67. doi: 10.1017/S1461145710001689
43. Wu HQ, Rassoulpour A, Schwarcz R. Kynurenic acid leads, dopamine follows: a new case of volume transmission in the brain. J Neural Transm. (2007) 114:33–41. doi: 10.1007/s00702-006-0562-y
44. Rassoulpour A, Wu HQ, Ferre S, Schwarcz R. Nanomolar concentrations of kynurenic acid reduce extracellular dopamine levels in the striatum. J Neurochem. (2005) 93:762–5. doi: 10.1111/j.1471-4159.2005.03134.x
45. Nilsson-Todd LK, Nordin C, Jönsson EG, Skogh E, Erhardt S. Cerebrospinal fluid kynurenic acid in male patients with schizophrenia – correlation with monoamine metabolites. Acta Neuropsychiatr. (2007) 19:45–52. doi: 10.1111/j.1601-5215.2006.00170.x
46. Leucht S, Kane JM, Kissling W, Hamann J, Etschel E, Engel RR. What does the PANSS mean. Schizophr Res. (2005) 79:231–8. doi: 10.1016/j.schres.2005.04.008
47. Morrens M, De Picker L, Kampen JK, Coppens V. Blood-based kynurenine pathway alterations in schizophrenia spectrum disorders: a meta-analysis. Schizophr Res. (2020) 223:43–52.
48. Foster AC, Vezzani A, French ED, Schwarcz R. Kynurenic acid blocks neurotoxicity and seizures induced in rats by the related brain metabolite quinolinic acid. Neurosci Lett. (1984) 48:273–8. doi: 10.1016/0304-3940(84)90050-8
49. Muller N, Schwarz M. Schizophrenia as an inflammation-mediated dysbalance of glutamatergic neurotransmission. Neurotox Res. (2006) 10:131–48. doi: 10.1007/BF03033242
50. Huang X, Ding W, Wu F, Zhou S, Deng S, Ning Y. Increased plasma kynurenic acid levels are associated with impaired attention/vigilance and social cognition in patients with schizophrenia. Neuropsychiatr Dis Treat. (2020) 16:263–71. doi: 10.2147/NDT.S239763
51. Dobelis P, Staley KJ, Cooper DC. Lack of modulation of nicotinic acetylcholine alpha-7 receptor currents by kynurenic acid in adult hippocampal interneurons. PLoS One. (2012) 7:e41108. doi: 10.1371/journal.pone.0041108
52. Jones LA, Hills PJ, Dick KM, Jones SP, Bright P. Cognitive mechanisms associated with auditory sensory gating. Brain Cogn. (2016) 102:33–45. doi: 10.1016/j.bandc.2015.12.005
53. Koola MM. Alpha7 nicotinic-N-methyl-D-aspartate hypothesis in the treatment of schizophrenia and beyond. Hum Psychopharmacol. (2021) 36:1–16.
Keywords: first-episode schizophrenia, kynurenine pathway, kynurenine, kynurenine acid, sensory gating, P50
Citation: Yang Q, Zhang Y, Yang K, Niu Y, Fan F, Chen S, Luo X, Tan S, Wang Z, Tong J, Yang F, Li C-SR and Tan Y (2022) Associations of the serum kynurenine pathway metabolites with P50 auditory gating in non-smoking patients with first-episode schizophrenia. Front. Psychiatry 13:1036421. doi: 10.3389/fpsyt.2022.1036421
Received: 04 September 2022; Accepted: 05 October 2022;
Published: 20 October 2022.
Edited by:
Wenbin Guo, Second Xiangya Hospital, Central South University, ChinaReviewed by:
Weihua Yue, Peking University Sixth Hospital, ChinaRonghuan Jiang, First Medical Center of PLA General Hospital, China
Copyright © 2022 Yang, Zhang, Yang, Niu, Fan, Chen, Luo, Tan, Wang, Tong, Yang, Li and Tan. This is an open-access article distributed under the terms of the Creative Commons Attribution License (CC BY). The use, distribution or reproduction in other forums is permitted, provided the original author(s) and the copyright owner(s) are credited and that the original publication in this journal is cited, in accordance with accepted academic practice. No use, distribution or reproduction is permitted which does not comply with these terms.
*Correspondence: Yunlong Tan, yltan21@126.com
†These authors have contributed equally to this work