- 1Department of Psychiatry and Psychology, Mayo Clinic Arizona, Phoenix, AZ, United States
- 2Department of Neuroscience, Mayo Clinic Graduate School of Biomedical Sciences, Collaborative Research Building (CRB), Scottsdale, AZ, United States
- 3Department of Medical Physiology, Faculty of Medicine, Mansoura University, Mansoura, Egypt
- 4Department of Cardiothoracic Surgery, Mayo Clinic Arizona, Phoenix, AZ, United States
- 5Alix School of Medicine at Mayo Clinic, Phoenix, AZ, United States
- 6Department of Critical Care, Mayo Clinic Arizona, Phoenix, AZ, United States
- 7Department of Neurology, Mayo Clinic Arizona, Phoenix, AZ, United States
We examined the effects of psychiatric comorbidity, sex, and ICU admission on serum ferritin concentration in 628 elderly patients (79.7 ± 8.5 years) with positive SARS-CoV-2 PCR test. Hospitalization was required in 96% of patients and 17% required ICU admission. Patients with COVID-19 and psychiatric comorbidities (n = 212) compared to patients without psychiatric comorbidities (n = 416) had significantly lower ferritin concentration (570.4 ± 900.1 vs. 744.1 ± 965, P = 0.029), a greater incidence of delirium (22.6 vs. 14.4%, P = 0.013) and higher mortality (35.3 vs. 27.6%, P = 0.015). Furthermore, we found significant effects for sex (P = 0.002) and ICU admission (P = 0.007). Among patients without comorbid psychiatric conditions, males had significantly higher ferritin compared to females (1,098.3 ± 78.4 vs. 651.5 ± 94.4, P < 0.001). ICU patients without comorbid psychiatric conditions had significantly higher serum ferritin compared to ICU patients with comorbid psychiatric conditions: (1,126.6 ± 110.7 vs. 668.6 ± 156.5, P < 0.001). Our results suggest that the presence of comorbid psychiatric conditions in elderly patients with COVID-19 is associated with higher rates of delirium and mortality and lower ferritin levels during severe illness. Whether high serum ferritin is protective during severe infection requires further investigation.
Introduction
Illness severity and mortality rates are high among geriatric patients with coronavirus disease 2019 (COVID-19) caused by severe acute respiratory syndrome coronavirus 2 (SARS-CoV-2) (1–3). Several factors contribute to this age-related vulnerability such as frailty and medical comorbidity (4–6). One of the medical defense mechanisms that may be impaired in elderly patients is the ability to augment the synthesis of ferritin under conditions of increased cellular oxidative stress, as seen during SARS-CoV-2 infection (7).
Ferritin is a protein synthesized by hepatocytes, macrophages, oligodendrocytes, and other cells to store excess cellular iron and to sequester iron rapidly in response to oxidative stress, to prevent the formation of reactive oxygen species (8–10). During infection, increased ferritin production represents an important host defense mechanism that deprives pathogens of iron necessary for replication and limits the production of free radicals (11).
Serum ferritin is a non-specific acute phase protein (12) that is elevated in many different conditions including inflammation (11, 13–17), malignancy (16, 18, 19), and certain autoimmune diseases (20–22). In COVID-19 patients, higher serum ferritin levels are reported in hospitalized (23, 24), critically ill patients (25–27) with more severe illness (23, 28–31), longer length of hospital stay (32) and in non-survivors (1, 25, 33–39). In addition, one study screened adult COVID-19 survivors (n = 109) for depression and anxiety using Beck Depression and Beck Anxiety Inventories (BDI, BAI) (40, 41) on the 15th post-hospitalization day, and reported significant positive correlation between BAI scores and ferritin levels in patients with anxiety (42).
In fact, patients with psychiatric conditions, regardless of COVID-19 infection status, have abnormalities in serum ferritin. High serum ferritin levels were reported in patients with major depression (n = 15) compared to patients without major depression (n = 92) (43), in patients with post-stroke depression compared to stroke patients without depression (44), in depressed patients with melancholia compared to those with simple major depression and normal controls (45), and in first episode (n = 23) and antidepressant-naïve depressed patients (n = 15) compared to healthy controls (n = 63) (46).
Other studies have reported opposite findings: decreased serum ferritin levels associated with depression. In a large-scale study from Germany, patients with major depression (n = 619) had lower ferritin levels compared to patients without major depression (n = 3,562) (log-transformed mean, 4.08 vs. 4.2; P = 0.003). Among males with cardiovascular disorders (congestive heart failure or hypertension), those with comorbid depression had lower levels of ferritin compared to patients without comorbid depression (47). In another study, depressed patients (n = 67) had lower serum ferritin compared to healthy controls (n = 125) (48). Moreover, low ferritin was reported in women who developed postpartum depression (n = 65) with a strong association between ferritin concentration and postpartum depressive symptom severity (49). Furthermore, ferritin levels were significantly lower in schizophrenic patients with (n = 30) and without (n = 30) akathisia compared to the control group (n = 30) (50) and also in bipolar manic patients compared to bipolar euthymic patients and to healthy controls. Still other studies found no significant differences in serum ferritin concentrations between patients with schizophrenia, bipolar disorder, anxiety, depression compared with controls (40, 41, 51).
These inconsistent results could reflect different responses of ferritin production under different illness states and at different ages since serum ferritin (47, 52) and brain iron and ferritin content increase with aging (53–57), rendering elderly patients with psychiatric disorders vulnerable to oxidative damage (58), ferroptosis (59) and mortality (60) during severe COVID-19.
In this study we aimed to examine whether geriatric COVID-19 patients with comorbid psychiatric conditions demonstrate increased ferritin production as illness severity increases necessitating ICU admission.
Materials and methods
Patients and data collection
This study was approved by the Institutional Review Board of the Mayo Clinic and COVID-19 Research Task Force (ID: 21-010940). It included electronic medical records (EMR) review of 927 geriatric patients (≥65 years old) with a confirmed positive SARS-CoV-2 by reverse-transcriptase polymerase chain reaction (RT-PCR) test (nasopharyngeal/oropharyngeal swab specimen), who received medical care at Mayo Clinic Health System from March 1, 2020, through October 1, 2021, and completed a serum ferritin concentration test. For patients completing more than one serum ferritin laboratory test, we selected the first ferritin level at time of admission for consistency and also based on recent reports suggesting the initial ferritin level, rather than change over hospital admission, predicts severity of illness, ICU admission, and need for mechanical ventilation (61). Patient data included demographics, psychiatric and medical comorbidities, and COVID-19 associated hospital course, ICU admission, and mortality.
Statistical analysis
Continuous variables were summarized using means (SD) and categorical variables using frequencies and percentages. Student’s t-test was used to compare the means of continuous variables and Fisher exact test was used to compare frequencies of categorical variables between the two study groups (with and without psychiatric comorbidities). Factorial ANOVAs were performed to examine the effects of sex, ICU admission, delirium, mortality, and psychiatric comorbidities on serum ferritin concentration covarying for age. Linear regression analysis was utilized to examine the association between serum ferritin and the duration between SARS-CoV-2 positive test and mortality dates in males and females. Analyses were performed with PRISM GraphPad 9 (San Diego, CA) and SPSS V27 software (Armonk, NY: IBM Corp). Results were considered significant at P < 0.05.
Results
Sample identification
Electronic medical records of Mayo Clinic Health System patients with COVID-19 diagnosis during the interval from March 1, 2020, through October 1, 2021, were examined to identify geriatric patients (≥65 years) with SARS-CoV-2 positive RT-PRC test and serum ferritin values (n = 927). We included patients with serum ferritin results up to 14 days before, and up to 30 days after, SARS-CoV-2 positive test (n = 628).
Patient demographics
The study cohort was categorized by the presence (n = 212) or absence (n = 416) of psychiatric comorbidities. The mean age did not differ between the two groups (79.6 ± 8.6 vs. 79.8 ± 8.4 years), but there were significant sex and race differences. The “no psychiatric comorbidity” group had more males (61.8 vs. 40.1%, P < 0.0001), and slightly fewer Caucasians (94.7 vs. 99.1%, P = 0.006). Among the no psychiatric comorbidity group (62.5 vs. 48.1, P = 0.0006), more reported being married, whereas 33% (vs. 23.1%, P = 0.009) of the psychiatric comorbidity group were widowed. Obesity (BMI 30.1–40 kg/m2) was reported in 33% of the psychiatric comorbidity group compared to 24% in the no psychiatric comorbidity group (P = 0.017) (Table 1).
Psychiatric and medical comorbidities
Patients in the psychiatric comorbidity group had clinical diagnoses of depression (70.3%), anxiety (42.5%), substance use (9.4%), or schizophrenia/schizoaffective disorders (2.8%). Hypothyroidism and other thyroid disorders were significantly more prevalent in the psychiatric comorbidity group (29.2 vs. 17.8%, P = 0.001). Similarly, the percentage of patients with chronic pain and dementia were significantly higher in the psychiatric comorbidity group (20.8 and 11.8% vs. 7.2 and 6.5%, respectively). No other significant differences were found in medical comorbidities between the two groups (Table 2).
Serum ferritin concentration, hospitalization, ICU admission, delirium, and mortality
Normal serum ferritin in healthy geriatric individuals from Copenhagen (age range 60–93) ranged between 16 and 240 μg/L (62). COVID-19 patients with psychiatric comorbidities had significantly lower ferritin concentration compared to patients without psychiatric comorbidities (570.4 ± 900.1 vs. 744.1 ± 965, P = 0.029). However, since the psychiatric comorbidity group had significantly more female patients and there is known sex difference in serum ferritin concentration (63), we compared male and female patients separately within four different ferritin quartiles (<100 μg/L, 100–500 μg/L, 501–1,000 μg/L, and >1,000 μg/L) and found no significant differences (Table 3).
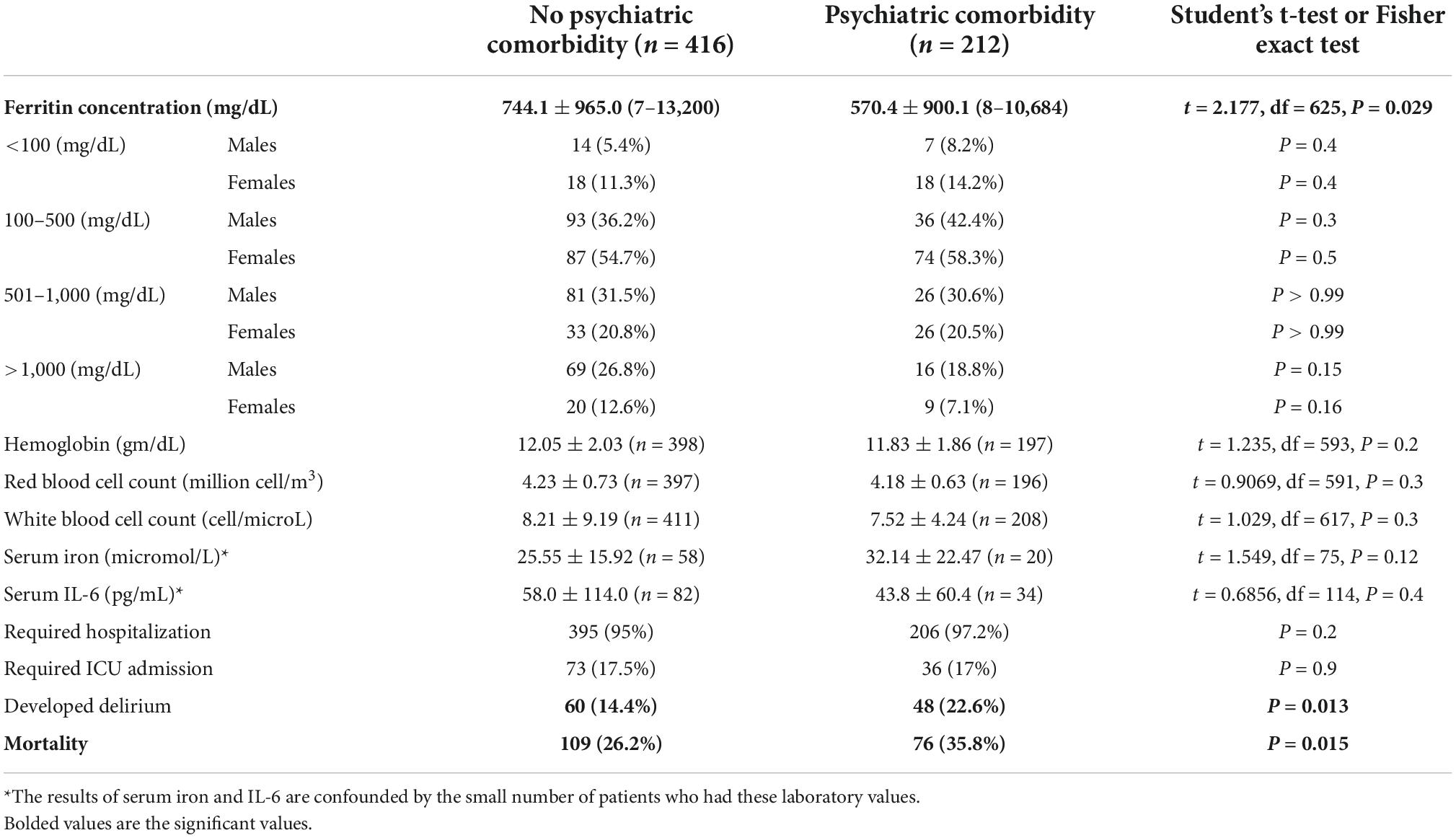
Table 3. Ferritin concentration, hemoglobin, red and white cell counts, serum iron, interleukin 6 (IL-6) concentrations and hospitalization, ICU admission, delirium, and mortality.
Almost all patients (97.2% with psychiatric comorbidity and 95% without) required hospitalization, and approximately 17% in each group required ICU admission. Patients with psychiatric comorbidities had a significantly higher rate of delirium (22.6 vs. 14.4%, P = 0.013) and a higher mortality rate (35.3 vs. 27.6%, P = 0.015). The interval between SARS-CoV-2 positive test and death was significantly longer in the psychiatric comorbidity group 64.9 ± 93.3 vs. 39.3 ± 54.2 days, P = 0.22 (Table 3).
The effects of sex, ICU admission, and psychiatric comorbidities on serum ferritin
Factorial ANOVA using age as a covariate showed significant effects for sex [F(1, 601) = 9.840, P = 0.002], ICU admission [F(1, 601) = 7.344. P = 0.007], and the presence of comorbid psychiatric conditions [F(1, 601) = 5.205, P = 0.02] on serum ferritin concentration. In addition, we found significant interactions between ICU admission and the presence of comorbid psychiatric conditions [F(1, 601) = 4.204, P = 0.041].
Pairwise comparisons reveal significantly higher serum ferritin in patients who were male [compared to females: 920.6 ± 76.9 vs. 588.1 ± 72.8], admitted to the ICU [compared to no ICU admission: 897.6 ± 95.8 vs. 611.0 ± 44.7], and without comorbid psychiatric conditions [compared to patients with comorbid psychiatric conditions: 874.9 ± 61.2 vs. 633.7 ± 86.1]. Marked differences between male and female patients in serum ferritin were observed only in those without comorbid psychiatric conditions [male vs. female: 1,098.3 ± 78.4 vs. 651.5 ± 94.4, P < 0.001]. No sex difference was observed in serum ferritin among patients with comorbid psychiatric conditions [male vs. female: 742.5 ± 132.0 vs. 524.6 ± 110.8, P = 0.2].
In addition, robust increase in serum ferritin in ICU admitted patients was only observed in those without comorbid psychiatric conditions [ICU admitted patients without comorbid psychiatric conditions vs. with comorbid psychiatric conditions: 1,126.6 ± 110.7 vs. 668.6 ± 156.5, P < 0.001]. Among patients who did not require ICU admission, we did not find any significant difference in serum ferritin between those with and without comorbid psychiatric conditions [598.8 ± 72.1 vs. 623.2 ± 52.8, P = 0.6] (Figure 1).
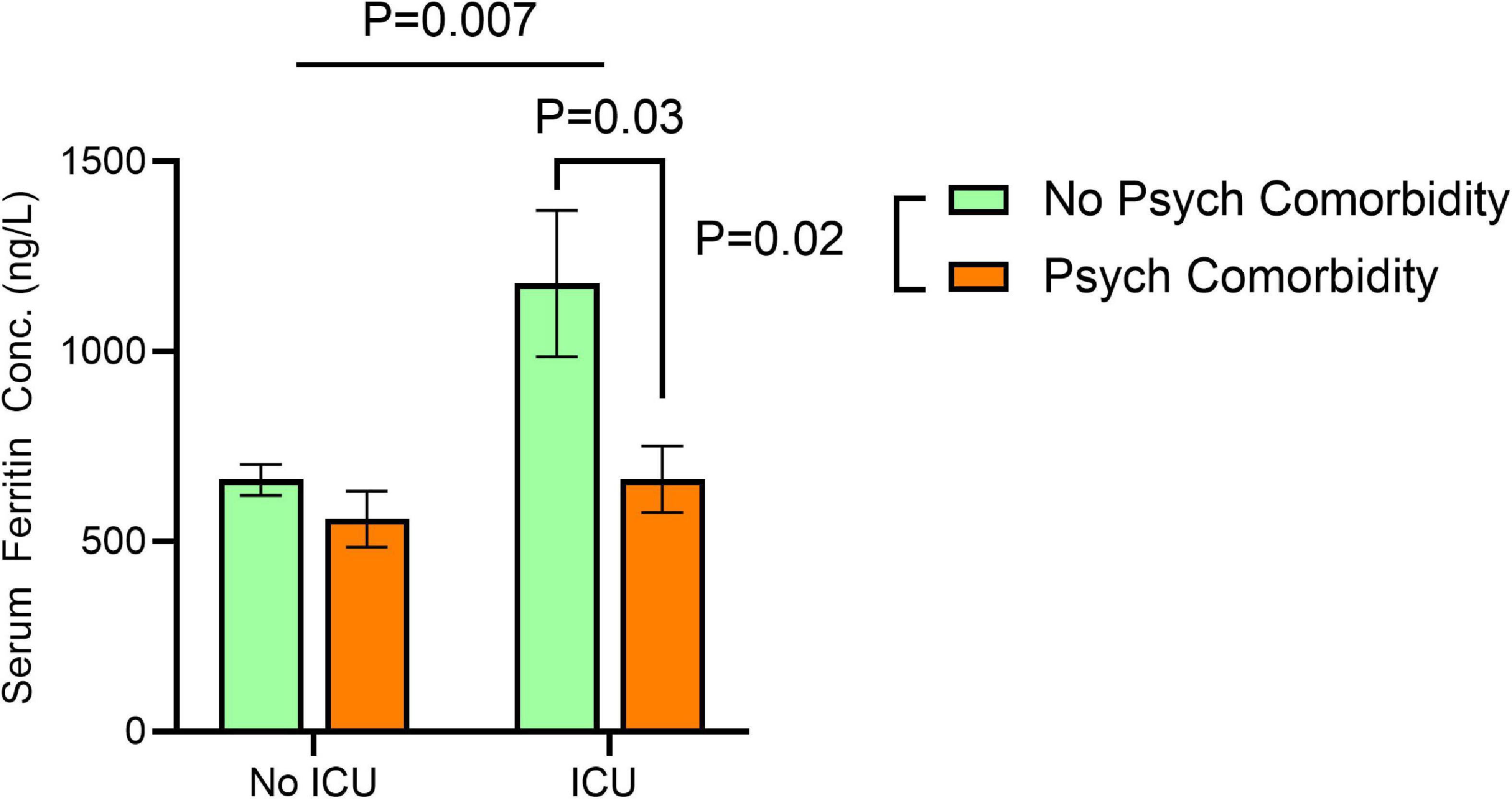
Figure 1. Effects of ICU admission and comorbid psychiatric conditions on serum ferritin concentrations. Significant effects for ICU admission [F(1, 601) = 7.344. P = 0.007], and the presence of comorbid psychiatric conditions [F(1, 601) = 5.205, P = 0.02] on serum ferritin concentration correcting for age at time of SARS-CoV-2 positive test by two-way ANOVA. Error bars represent 95% confidence interval.
The effects of mortality and delirium on serum ferritin
We have examined whether serum ferritin differs based on survival (vs. mortality) and the presence (vs. absence) of psychiatric comorbidity Mean serum ferritin was markedly elevated in non-survivors (compared to survivors) without psychiatric comorbidity (922.51 ± 1,401.51 vs. 672.68 ± 797.75), but the difference in serum ferritin between non-survivors and survivors among patients with psychiatric comorbidity was much smaller (686.12 ± 748.86 vs. 513.76 ± 950.64). A significant effect of mortality [F(1,620) = 6.080, P = 0.013] and psychiatric comorbidity [F(1, 620) = 5.33, P = 0.02] on serum ferritin was evident, but no interaction between the two factors was found [F(1,620) = 0.2047, P = 0.6 (Figure 2).
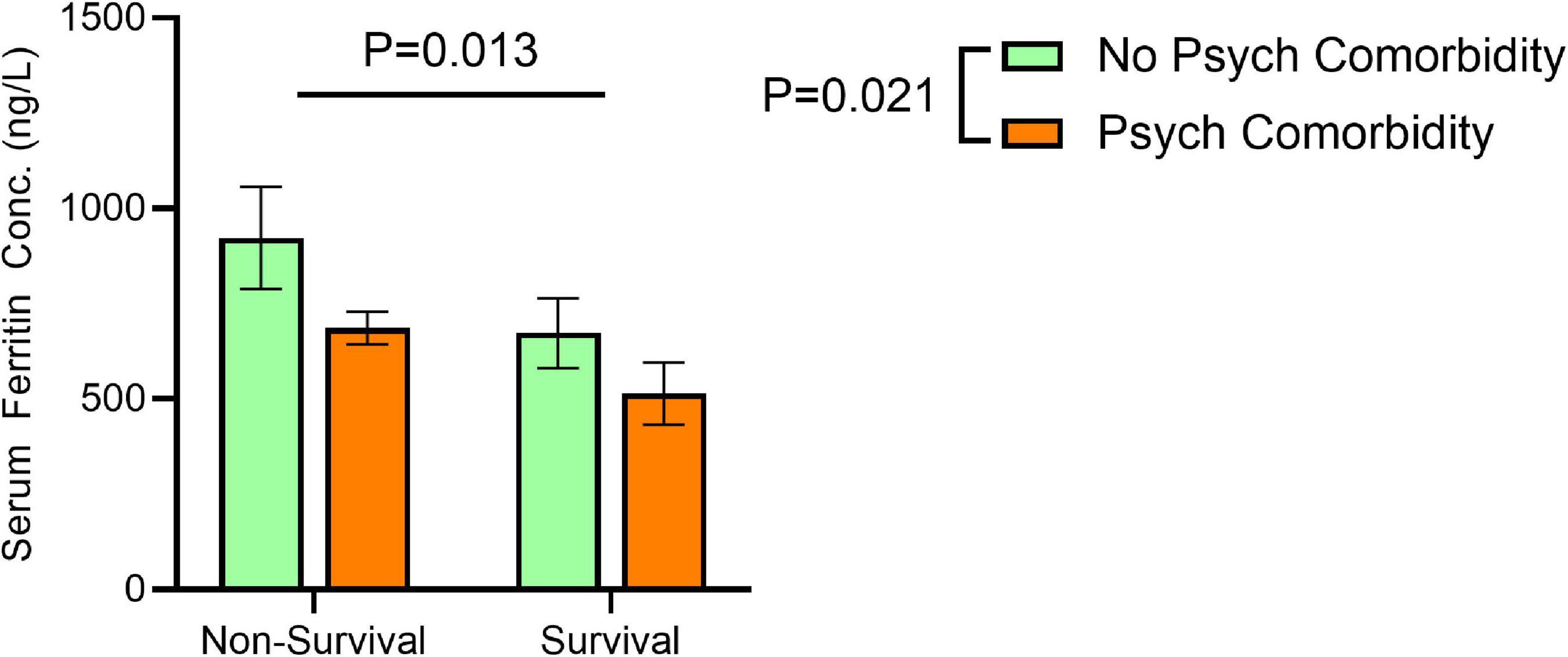
Figure 2. Effects of non-survival and comorbid psychiatric conditions on serum ferritin concentrations. Significant effects for non-survival [F(1,620) = 6.080, P = 0.013] and psychiatric comorbidity [F(1, 620) = 5.33, P = 0.02] on serum ferritin, but no interaction between the two factors [F(1,620) = 0.2047, P = 0.6 on serum ferritin concentration correcting for age at time of SARS-CoV-2 positive test by two-way ANOVA. Error bars represent 95% confidence interval.
Next, we assessed the association between serum ferritin and the interval between SARS-CoV-2 positive test and death using linear regression and found no significant association in patients with [F(1,73) = 0.2454, P = 0.6] or without psychiatric comorbidity [F(1,106) = 3.273, P = 0.073]. Further, we did not find significant effect of delirium on serum ferritin concentration [F(1,619) = 0.058, P = 0.8] among patients with or without psychiatric comorbidity [F(1,619) = 0.05, P = 0.8].
Peak serum ferritin
To examine whether peak serum ferritin concentrations differ between the two groups, we collected all available serum ferritin results and identified the highest value for those with more than one ferritin result and identified the day reaching the peak. About 13–14% had the peak on day 3 (without psych comorbidity) or day 4 (with psych comorbidity) (Supplementary Figure 1). Next, we calculated the percentage change in serum ferritin from baseline to peak and compared the two groups. We found no significant effect of psychiatric comorbidity [F(1, 201) = 3.681, P = 0.056] or time [F(12, 201) = 1.356, P = 0.19] on “peak” serum ferritin concentration (Supplementary Figure 2). Finally, we performed a two-way ANOVA (ICU admission and presence or absence of psychiatric comorbidity) and found no significant effect of psychiatric comorbidity on “peak” serum ferritin concentration [F(1, 238) = 2.377, P = 0.12]. However, it is important here to state that we don’t have solid data to be certain of peak serum ferritin results because (1) we don’t have repeated serum ferritin measurements except for 390 (out of 627), (2) among those we have 114 with only two measures and 276 with 3 or more measurements, and (3) among those with 3 or more measures we have 82 patients with peak serum ferritin as the first ferritin value, so, trying to interpret the results for our available “peak” serum ferritin is not going to be valid.
Discussion
The results of this study show that in the setting of COVID-19 infection, geriatric patients with and without comorbid psychiatric conditions have high serum ferritin concentrations. However, in critical illness requiring ICU admission, COVID-19 geriatric patients without psychiatric comorbidity had a higher, more robust increase in serum ferritin compared with patients with psychiatric comorbidity. It is possible that ferritin synthesis in these patients is blunted or that there is destruction of ferritin (ferritinophagy) and release of catalytic iron which may cause significant increase in oxidative stress and contribute to mortality. High serum ferritin could also be detrimental if it triggers nuclear receptor coactivator 4 (NCOA4)-mediated ferritinophagy, in which ferritin is degraded and iron is released. Excess iron released from ferritinophagy can increase oxidative stress and promote ferroptosis and cellular damage (64). The brain is highly sensitive to oxidative damage because of its high iron content (65). Failure to augment ferritin synthesis to sequester excessive iron during severe COVID-19 or the destruction of ferritin and rapid release of iron may leave the brain vulnerable to oxidative damage and neurotoxicity. Serum ferritin also plays a role in the regulation of immune response during acute inflammatory states, which may explain the attenuated increase in patients with comorbid psychiatric disorders.
Ferritin molecule, synthesis, and functions within the cell
Apoferritin (empty ferritin shell) is composed of 24 polypeptide chains arranged into 2 subunits: heavy (H) and light (L) subunits, each coded in a different gene (19). H-ferritin has ferroxidase activity that oxidizes ferrous iron into ferric iron (66). L-ferritin is found mostly in liver, spleen and serum and stores ferric iron up to 4,500 iron atoms can be stored inside the ferritin core (67–70). Tissues with high energy demand such as the brain have high iron content and high production of reactive oxygen species. For that reason, these tissues will have high concentration of H-ferritin to detoxify iron quickly and prevent ferroptosis. The brain has the second highest iron concentration after the liver because of its high metabolic activity and high oxidative stress. Brain iron is found mainly in neurons while ferritin is concentrated in oligodendrocytes (71). Mitochondrial ferritin is close to H-ferritin and has antioxidant properties and protects neurons against ferroptosis (72, 73), while nuclear ferritin protects DNA from oxidative damage (74).
Serum ferritin
Ferritin is secreted by macrophages, hepatocytes, and other cells into the serum to distribute iron to other cells (75–77). Most serum ferritin is L-ferritin (10). Cells uptake L-ferritin through the Scavenger Receptor Class A member 5 (SCARA5) (78), while H-ferritin receptors are transferrin receptor-1 (79). Serum ferritin is cleared by the liver and its concentration correlates with the concentration of intracellular iron under normal conditions (80). Serum ferritin has low iron content in healthy individuals (1,100–1,200 iron atoms/ferritin) and even lower in patients with inflammation (about 800 iron atoms/ferritin) (8). However, the low number of iron atoms in ferritin is still significantly higher than the iron content in transferrin (2 iron atoms/transferrin) which makes ferritin an important source for rapid delivery of iron at time of stress.
Severe acute respiratory syndrome coronavirus 2 increases oxidative stress, and the cell responds by upregulating ferritin synthesis
Viruses including SARS-CoV-2 generate a pro-oxidative environment in the host cell to optimize and sustain their replication (7). SARS-CoV-2 infection markedly stimulates nuclear factor-κB (NF-kB) protein expression and activity (81, 82). NF-κB is a central mediator of pro-inflammatory gene induction and functions in both innate and adaptive immune cells. It also targets inflammation not only directly by increasing the production of inflammatory cytokines, chemokines, and adhesion molecules, but also by regulating cell proliferation, apoptosis, morphogenesis, and differentiation. This dysregulated inflammatory response increases oxidative stress (83). In addition, SARS-CoV-2 interferes with the synthesis of antioxidant glutathione (GSH) through consumption of cystine into viral proteins during viral replication (7). Cytokines (TNF-α, IL-1α, IL-1β, IL-6) (84, 85) and oxidative stress upregulate the H-ferritin gene [reviewed in refs (19, 20)]. L-ferritin gene is regulated mostly by high iron content. Inflammation induces ferritin synthesis to divert labile iron into stored iron and reduce the availability of iron that can be used for bacterial growth (86). In addition, serum H-ferritin acts as an immuno-suppressor with some in vitro evidence that H- ferritin may directly suppress the differentiation of human B lymphocytes maturing into antibody producing cells (22, 87). Further, ferritin administration in mice protects against E. coli-induced sepsis (88).
Aging and psychiatric comorbidities affect ferritin response to inflammation
Aging is associated with progressive increase in neuronal iron deposition (71), serum ferritin (89, 90) and progressive decline in GSH concentration (91–94), increasing vulnerability to oxidative stress during infection (7). Psychiatric conditions also contribute to the increased vulnerability to oxidative stress. However, production of ferritin under stress conditions is variable. One study showed decreased hippocampal expression of H-ferritin in a mouse model of lipo poly saccharide (LPS)-induced depression (95), while another study showed increased expression of hippocampal ferritin (H and L subunits) in a mouse model of chronic unpredictable mild stress-induced depression (96).
Several factors influence ferritin synthesis in patients with psychiatric disorders such as comorbid substance use. Serum ferritin concentration correlated with alcohol intake (90) and heavy drinking was associated with increased serum ferritin concentration (97). Similarly, opioid use alters ferritin synthesis. μ-opioid agonists specifically elevate neuronal levels of H-ferritin (98–100). Comorbid diabetes and hypothyroidism blunt ferritin response to inflammation since the H-ferritin gene is upregulated by thyrotropin (101), T4 (102), T3 (103), insulin, and IGF-1 (19, 20)]. Sex and racial differences may also impact ferritin synthesis. One study found that black male patients responded to inflammation with a more robust rise in serum ferritin compared to white male patients (104).
High ferritin is reported in severely ill geriatric COVID-19 non-survivors who were hospitalized (n = 873) (105) or admitted to the ICU (n = 174) (106). Similarly, high ferritin was associated with a 1.7-fold (HR 1.709, 95% CI 1.017–2.871, p = 0.043) higher risk of ICU admission or in-hospital death in a cohort of 362 elderly patients (107).
Sex effect on serum ferritin
We found a significant effect of sex on serum ferritin in those without psychiatric comorbidity. Male patients without psychiatric comorbidity had significantly higher serum ferritin compared to female patients. However, we observed no sex differences in patients with psychiatric comorbidity. Hypothyroidism is more prevalent among psychiatric patients (108) specifically among women (109) and ferritin synthesis is regulated by thyroid hormones (101–103), so it is possible that patients with psychiatric comorbidities, specifically female patients are unable to augment the synthesis of ferritin under the stress of severe infection. Further research is needed to test this hypothesis. Women have significantly lower brain ferritin compared to men (55) suggesting sex-specific influence on iron regulation (110) that may not be related to female sex hormones (111). The reasons behind this sex difference have not yet been fully elucidated.
Patients with psychiatric comorbidity had significantly higher incidence of delirium and higher mortality
In our study, 22.6% of patients with psychiatric comorbidity developed delirium compared to 14.4% of those without psychiatric comorbidity (P = 0.014) and 35.8% died compared to 26.2% in the non-psychiatric comorbidity groups (P = 0.015). Delirium is common among COVID-19 patients admitted to the ICU, affecting 61% of patients in one study (112). Delirium is a risk factor for negative impact on recovery from various medical and surgical conditions (113, 114). Furthermore, delirium is associated with high mortality rates during hospitalization (115, 116), high rates of post hospitalization cognitive impairment (117, 118), and mortality (119). Whether disruption in brain iron homeostasis contributes to the development of delirium and overall mortality remains to be further investigated.
Mean serum ferritin was markedly elevated in non-survivors (compared to survivors) without psychiatric comorbidity (922.51 ± 1,401.51 vs. 672.68 ± 797.75), but the difference in serum ferritin between non-survivors and survivors among patients with psychiatric comorbidity was much smaller (686.12 ± 748.86 vs. 513.76 ± 950.64). Rich literature document high serum ferritin among COVID-19 non-survivors (1, 25, 33–39). Our results show that the marked elevation in serum ferritin typically observed in COVID-19 non-survivors, is not present in patients with psychiatric comorbidity who eventually died from severe illness. This new insight into the confounding effect of comorbid psychiatric conditions should be considered when interpreting serum ferritin results in COVID-19 mortality. Further studies to investigate the potential role of ferritin in protecting neuronal integrity during inflammatory-induced stress conditions are needed.
The confounding effect of metabolic syndrome
The prevalence of diabetes in both groups was similar (about 28%). However, geriatric patients with psychiatric comorbidities had significantly higher rates of obesity (BMI 30.1–40 kg/m2) (33 vs. 24%, P = 0.017). High ferritin levels are reported in a meta-analysis (n = 56,053) to be independently and positively associated with the presence of the metabolic syndrome with an odds ratio higher than 1.73 (120). Unfortunately, we do not have the baseline (before hospitalization) ferritin levels in these patients. Similarly, potential impacts of medication effects were not included in this study.
Limitations
This study has several limitations including its retrospective design and the documented clinical diagnosis of comorbid psychiatric conditions. Our current results do not answer the question about peak serum ferritin concentration timing or whether it could be different between patients with and without psychiatric comorbidity. Similarly, we are unable to comment on the effect of psychiatric comorbidity, if any, serum iron concentration or inflammatory markers such as IL-6 because of the small number of patients with these laboratory values. In addition, the data collection did not include the extent of substance use, which may have been a confounding factor, particularly in patients developing in-hospital delirium.
Conclusion
The results of this study show that critically ill, geriatric patients with comorbid psychiatric conditions have attenuated increase in serum ferritin concentration compared to those without comorbid psychiatric conditions. There are at least two possible mechanisms by which to explain this finding. The first could be a failure of psychiatric patients to augment ferritin synthesis due to higher prevalence of hypothyroidism, genetic, or other unknown factors. The second could be that high ferritin-triggered pathways cause rapid release of iron from ferritin, leading to more oxidative damage and cell death. Further research is needed to explore both possibilities and examine the role of psychiatric disorders and ferritin on illness severity and mortality during COVID-19 infection.
Data availability statement
The original contributions presented in this study are included in the article/Supplementary material, further inquiries can be directed to the corresponding author.
Ethics statement
The studies involving human participants were reviewed and approved by the Institutional Review Board of the Mayo Clinic and COVID-19 Research Task Force (ID: 21-010940). Written informed consent for participation was not required for this study in accordance with the national legislation and the institutional requirements.
Author contributions
OA: supervision, drafting of the manuscript, full access to all the data in the study, and takes responsibility for the integrity of the data and the accuracy of the data analysis. OA and AY: concept and design. OA, AY, CE, VN, MA, and YQ: acquisition, analysis, or interpretation of data. All authors: critical revision of the manuscript for important intellectual content, administrative, technical, or material support.
Funding
This research was supported by the Department of Psychiatry and Psychology at Mayo Clinic Arizona.
Acknowledgments
We would like to acknowledge Patricia Kennedy (Informatics Nurse Specialist) and Sharon Freimund (IT Tech Specialist I) for their work in extracting data from the medical records.
Conflict of interest
The authors declare that the research was conducted in the absence of any commercial or financial relationships that could be construed as a potential conflict of interest.
Publisher’s note
All claims expressed in this article are solely those of the authors and do not necessarily represent those of their affiliated organizations, or those of the publisher, the editors and the reviewers. Any product that may be evaluated in this article, or claim that may be made by its manufacturer, is not guaranteed or endorsed by the publisher.
Supplementary material
The Supplementary Material for this article can be found online at: https://www.frontiersin.org/articles/10.3389/fpsyt.2022.1035986/full#supplementary-material
Supplementary Figure 1 | Time to peak serum ferritin in patients with and without psychiatric comorbidity. About 13 and 14% of patients with and without psychiatric comorbidity respectively reach peak serum ferritin in days 4 and 3 post SARS-CoV-2 positive test.
Supplementary Figure 2 | Percentage change from initial to peak serum ferritin concentration in COVID-19 patients with and without psychiatric comorbidity. No significant effect of psychiatric comorbidity on the percentage change in serum ferritin concentration (from initial to peak).
References
1. Zhou F, Yu T, Du R, Fan G, Liu Y, Liu Z. Clinical course and risk factors for mortality of adult inpatients with COVID-19 in Wuhan, China: a retrospective cohort study. Lancet. (2020) 395:1054–62. doi: 10.1016/S0140-6736(20)30566-3
2. Vacheron CH, Bitker L, Thiolliére F, Subtil F, Abraham P, Collange V, et al. Prognosis of old intensive care COVID-19 patients at a glance: the senior COVID study. Turk J Anaesthesiol Reanim. (2022) 50:S57–61.
3. Tiwari L, Gupta P, N Y, Banerjee A, Kumar Y, Singh PK, et al. Clinicodemographic profile and predictors of poor outcome in hospitalised COVID-19 patients: a single-centre, retrospective cohort study from India. BMJ Open. (2022) 12:e056464. doi: 10.1136/bmjopen-2021-056464
4. Bag Soytas R, Ünal D, Arman P, Suzan V, Emiroðlu Gedik T, Can G, et al. Factors affecting mortality in geriatric patients hospitalized with COVID-19. Turk J Med Sci. (2021) 51:454–63. doi: 10.3906/sag-2008-91
5. Wu Z, McGoogan JM. Characteristics of and important lessons from the coronavirus disease 2019 (COVID-19) outbreak in china: summary of a report of 72314 cases from the chinese center for disease control and prevention. JAMA. (2020) 323:1239–42. doi: 10.1001/jama.2020.2648
6. Ghosh T, Dwivedi T, Agarwal H, Iyer H, Tiwari P, Mittal S, et al. Impact of various hematological and biochemical parameters on mortality in coronavirus disease 2019 (COVID-19): a single-center study from north India. Lung India. (2022) 39:230–3. doi: 10.4103/lungindia.lungindia_480_21
7. Galli F, Marcantonini G, Giustarini D, Albertini MC, Migni A, Zatini L, et al. How aging and oxidative stress influence the cytopathic and inflammatory effects of SARS-CoV-2 infection: the role of cellular glutathione and cysteine metabolism. Antioxidants. (2022) 11:1366. doi: 10.3390/antiox11071366
8. Watt RK. The many faces of the octahedral ferritin protein. Biometals. (2011) 24:489–500. doi: 10.1007/s10534-011-9415-8
9. Plays M, Muller S, Rodriguez R. Chemistry and biology of ferritin. Metallomics. (2021) 13:mfab021. doi: 10.1093/mtomcs/mfab021
10. Wang W, Knovich MA, Coffman LG, Torti FM, Torti SV. Serum ferritin: past, present and future. Biochim Biophys Acta. (2010) 1800:760–9. doi: 10.1016/j.bbagen.2010.03.011
11. Kernan KF, Carcillo JA. Hyperferritinemia and inflammation. Int Immunol. (2017) 29:401–9. doi: 10.1093/intimm/dxx031
12. Ahmed MS, Jadhav AB, Hassan A, Meng QH. Acute phase reactants as novel predictors of cardiovascular disease. ISRN Inflamm. (2012) 2012:953461. doi: 10.5402/2012/953461
13. Birgegard G, Hällgren R, Killander A, Strömberg A, Venge P, Wide L. Serum ferritin during infection. A longitudinal study. Scand J Haematol. (1978) 21:333–40. doi: 10.1111/j.1600-0609.1978.tb00374.x
14. Birgegard G. The source of serum ferritin during infection. Studies with concanavalin a–sepharose absorption. Clin Sci. (1980) 59:385–7. doi: 10.1042/cs0590385
15. Fahmy M, Young SP. Modulation of iron metabolism in monocyte cell line U937 by inflammatory cytokines: changes in transferrin uptake, iron handling and ferritin mRNA. Biochem J. (1993) 296:175–81. doi: 10.1042/bj2960175
16. Koorts AM, Viljoen M. Ferritin and ferritin isoforms II: protection against uncontrolled cellular proliferation, oxidative damage and inflammatory processes. Arch Physiol Biochem. (2007) 113:55–64. doi: 10.1080/13813450701422575
17. Orino K, Lehman L, Tsuji Y, Ayaki H, Torti SV. Ferritin and the response to oxidative stress. Biochem J. (2001) 357:241–7. doi: 10.1042/bj3570241
18. Alkhateeb AA, Connor JR. The significance of ferritin in cancer: anti-oxidation, inflammation and tumorigenesis. Biochim Biophys Acta. (2013) 1836:245–54. doi: 10.1016/j.bbcan.2013.07.002
19. Torti FM, Torti SV. Regulation of ferritin genes and protein. Blood. (2002) 99:3505–16. doi: 10.1182/blood.V99.10.3505
20. Zandman-Goddard G, Shoenfeld Y. Hyperferritinemia in autoimmunity. Isr Med Assoc J. (2008) 10:83–4.
21. Zandman-Goddard G, Shoenfeld Y. Ferritin in autoimmune diseases. Autoimmun Rev. (2007) 6:457–63. doi: 10.1016/j.autrev.2007.01.016
22. Recalcati S, Invernizzi P, Arosio P, Cairo G. New functions for an iron storage protein: the role of ferritin in immunity and autoimmunity. J Autoimmun. (2008) 30(1–2):84–9. doi: 10.1016/j.jaut.2007.11.003
23. Moreira AC, Teles MJ, Silva T, Bento CM, Alves IS, Pereira L, et al. Iron related biomarkers predict disease severity in a cohort of portuguese adult patients during COVID-19 acute infection. Viruses. (2021) 13:12. doi: 10.3390/v13122482
24. Hippchen T, Altamura S, Muckenthaler MU, Merle U. Hypoferremia is associated with increased hospitalization and oxygen demand in COVID-19 patients. Hemasphere. (2020) 4:e492. doi: 10.1097/HS9.0000000000000492
25. Deng F, Zhang L, Lyu L, Lu Z, Gao D, Ma X, et al. [Increased levels of ferritin on admission predicts intensive care unit mortality in patients with COVID-19]. Med Clin. (2021) 156:324–31. doi: 10.1016/j.medcli.2020.11.030
26. Alsafi RT, Minshawi F, Alshareef A, Althobiany E, Alqurashi A, Zawawi A, et al. Haematological, biochemical, and inflammatory biomarkers of COVID-19 patients hospitalized in critical unit: a retrospective study. Cureus. (2022) 14:e23691. doi: 10.7759/cureus.23691
27. Gurusamy E, Mahalakshmi S, Kaarthikeyan G, Ramadevi K, Arumugam P, Gayathr MS. Biochemical predictors for Sars-Cov-2 severity. Bioinformation. (2021) 17:834–9. doi: 10.6026/97320630017834
28. Dahan S, Segal G, Katz I, Hellou T, Tietel M, Bryk G, et al. Ferritin as a marker of severity in COVID-19 patients: a fatal correlation. Isr Med Assoc J. (2020) 22:494–500.
29. Zhou C, Chen Y, Ji Y, He X, Xue D, et al. Increased serum levels of hepcidin and ferritin are associated with severity of COVID-19. Med Sci Monit. (2020) 26:e926178. doi: 10.12659/MSM.926178
30. Yagci S, Serin E, Acicbe Ö, Zeren MÝ, Odabaşı MS. The relationship between serum erythropoietin, hepcidin, and haptoglobin levels with disease severity and other biochemical values in patients with COVID-19. Int J Lab Hematol. (2021) 43‘:142–51. doi: 10.1111/ijlh.13479
31. Melo AKG, Milby KM, Caparroz ALMA, Pinto ACPN, Santos RRP, Rocha AP, et al. Biomarkers of cytokine storm as red flags for severe and fatal COVID-19 cases: a living systematic review and meta-analysis. PLoS One. (2021) 16:e0253894. doi: 10.1371/journal.pone.0253894
32. Oksuz E, Malhan S, Gonen MS, Kutlubay Z, Keskindemirci Y, Tabak F, et al. COVID-19 healthcare cost and length of hospital stay in Turkey: retrospective analysis from the first peak of the pandemic. Health Econ Rev. (2021) 11:39. doi: 10.1186/s13561-021-00338-8
33. Cheng L, Li H, Li L, Liu C, Yan S, Chen H, et al. Ferritin in the coronavirus disease 2019 (COVID-19): a systematic review and meta-analysis. J Clin Lab Anal. (2020) 34:e23618. doi: 10.1002/jcla.23618
34. Zeng F, Huang Y, Guo Y, Yin M, Chen X, Xiao L, et al. Association of inflammatory markers with the severity of COVID-19: a meta-analysis. Int J Infect Dis. (2020) 96:467–74. doi: 10.1016/j.ijid.2020.05.055
35. Sonnweber T, Boehm A, Sahanic S, Pizzini A, Aichner M, Sonnweber B, et al. Persisting alterations of iron homeostasis in COVID-19 are associated with non-resolving lung pathologies and poor patients’ performance: a prospective observational cohort study. Respir Res. (2020) 21:276. doi: 10.1186/s12931-020-01546-2
36. Chakurkar V, Rajapurkar M, Lele S, Mukhopadhyay B, Lobo V, Injarapu R, et al. Increased serum catalytic iron may mediate tissue injury and death in patients with COVID-19. Sci Rep. (2021) 11:19618. doi: 10.1038/s41598-021-99142-x
37. Ramonfaur D, Aguirre-García GM, Diaz-Garza CA, Torre-Amione G, Sanchez-Nava VM, Lara-Medrano R, et al. Early increase of serum ferritin among COVID-19 patients is associated with need of invasive mechanical ventilation and with in-hospital death. Infect Dis. (2022) 2022:1–9. doi: 10.1080/23744235.2022.2101691
38. Yousaf MN, Sarwar S, Tarique S, Ahmed M, Tahir H. Mortality in patients of COVID-19 infection: biochemical markers and its cut-off values for predicting outcome. J Coll Physicians Surg Pak. (2022) 32:37–41. doi: 10.29271/jcpsp.2022.01.37
39. Rubio-Rivas M, Mora-Luján JM, Formiga F, Corrales González MÁ, García Andreu MDM, Moreno-Torres V, et al. Clusters of inflammation in COVID-19: descriptive analysis and prognosis on more than 15,000 patients from the Spanish SEMI-COVID-19 Registry. Intern Emerg Med. (2022) 17:1115–27. doi: 10.1007/s11739-021-02924-4
40. Beck AT, Epstein N, Brown G, Steer RA. An inventory for measuring clinical anxiety: psychometric properties. J Consult Clin Psychol. (1988) 56:893–7. doi: 10.1037/0022-006X.56.6.893
41. Beck AT, Ward CH, Mendelson M, Mock J, Erbaugh J. An inventory for measuring depression. Arch Gen Psychiatry. (1961) 4:561–71. doi: 10.1001/archpsyc.1961.01710120031004
42. Demiryurek E, De Lorenzo R, Conte C, Poletti S, Vai B, Bollettini I, et al. Depression and anxiety disorders in COVID-19 survivors: role of inflammatory predictors. Noro Psikiyatr Ars. (2022) 59:105–9.
43. Huang TL, Lee CT. Low serum albumin and high ferritin levels in chronic hemodialysis patients with major depression. Psychiatry Res. (2007) 152(:277–80. doi: 10.1016/j.psychres.2005.07.038
44. Zhu L, Han B, Wang L, Chang Y, Ren W, Gu Y, et al. The association between serum ferritin levels and post-stroke depression. J Affect Disord. (2016) 190:98–102. doi: 10.1016/j.jad.2015.09.074
45. Maes M, Van de Vyvere J, Vandoolaeghe E, Bril T, Demedts P, Wauters A, et al. Alterations in iron metabolism and the erythron in major depression: further evidence for a chronic inflammatory process. J Affect Disord. (1996) 40:23–33. doi: 10.1016/0165-0327(96)00038-9
46. Stelzhammer V, Haenisch F, Chan MK, Cooper JD, Steiner J, Steeb H, et al. Proteomic changes in serum of first onset, antidepressant drug-naive major depression patients. Int J Neuropsychopharmacol. (2014) 17:1599–608. doi: 10.1017/S1461145714000819
47. Baune BT, Neuhauser H, Ellert U, Berger K. The role of the inflammatory markers ferritin, transferrin and fibrinogen in the relationship between major depression and cardiovascular disorders - the German health interview and examination survey. Acta Psychiatr Scand. (2010) 121:135–42. doi: 10.1111/j.1600-0447.2009.01435.x
48. Vahdat Shariatpanaahi M, Vahdat Shariatpanaahi Z, Moshtaaghi M, Shahbaazi SH, Abadi A. The relationship between depression and serum ferritin level. Eur J Clin Nutr. (2007) 61:532–5. doi: 10.1038/sj.ejcn.1602542
49. Albacar G, Sans T, Martín-Santos R, García-Esteve L, Guillamat R, Sanjuan J, et al. An association between plasma ferritin concentrations measured 48 h after delivery and postpartum depression. J Affect Disord. (2011) 131:136–42. doi: 10.1016/j.jad.2010.11.006
50. Kuloglu M, Atmaca M, Ustündag B, Canatan H, Gecici O, Tezcan E, et al. Serum iron levels in schizophrenic patients with or without akathisia. Eur Neuropsychopharmacol. (2003) 13:67–71. doi: 10.1016/S0924-977X(02)00073-1
51. Keles Altun I, Atagün MÝ, Erdoðan A, Oymak Yenilmez D, Yusifova A, Şenat A, et al. Serum hepcidin/ferroportin levels in bipolar disorder and schizophrenia. J Trace Elem Med Biol. (2021) 68:126843. doi: 10.1016/j.jtemb.2021.126843
52. Casale G, Bonora C, Migliavacca A, Zurita IE, de Nicola P. Serum ferritin and ageing. Age Ageing. (1981) 10:119–22. doi: 10.1093/ageing/10.2.119
53. Roskams AJ, Connor JR. Iron, transferrin, and ferritin in the rat brain during development and aging. J Neurochem. (1994) 63:709–16. doi: 10.1046/j.1471-4159.1994.63020709.x
54. Focht SJ, Snyder BS, Beard JL, Van Gelder W, Williams LR, Connor JR, et al. Regional distribution of iron, transferrin, ferritin, and oxidatively-modified proteins in young and aged fischer 344 rat brains. Neuroscience. (1997) 79:255–61. doi: 10.1016/S0306-4522(96)00607-0
55. Bartzokis G, Tishler TA, Lu PH, Villablanca P, Altshuler LL, Carter M, et al. Brain ferritin iron may influence age- and gender-related risks of neurodegeneration. Neurobiol Aging. (2007) 28:414–23. doi: 10.1016/j.neurobiolaging.2006.02.005
56. Connor JR, Menzies SL, St Martin SM, Mufson EJ. Cellular distribution of transferrin, ferritin, and iron in normal and aged human brains. J Neurosci Res. (1990) 27:595–611. doi: 10.1002/jnr.490270421
57. Fairweather-Tait SJ, Wawer AA, Gillings R, Jennings A, Myint PK. Iron status in the elderly. Mech Ageing Dev. (2014) 13:22–8. doi: 10.1016/j.mad.2013.11.005
58. Joshi YB, Pratico D. Lipid peroxidation in psychiatric illness: overview of clinical evidence. Oxid Med Cell Longev. (2014) 2014:828702. doi: 10.1155/2014/828702
59. Mazhar M, Din AU, Ali H, Yang G, Ren W, Wang L, et al. Implication of ferroptosis in aging. Cell Death Discov. (2021) 7:149. doi: 10.1038/s41420-021-00553-6
60. Tian Y, Tian Y, Yuan Z, Zeng Y, Wang S, Fan X, et al. Iron metabolism in aging and age-related diseases. Int J Mol Sci. (2022) 23:7. doi: 10.3390/ijms23073612
61. Shakaroun DA, Lazar MH, Horowitz JC, Jennings JH. Serum ferritin as a predictor of outcomes in hospitalized patients with covid-19 pneumonia. J Intensive Care Med. (2022) 2022:8850666221113252. doi: 10.1177/08850666221113252
62. Milman N, Andersen HC, Pedersen NS. Serum ferritin and iron status in ‘healthy’ elderly individuals. Scand J Clin Lab Invest. (1986) 46:19–26. doi: 10.3109/00365518609086476
63. Vicente C, Porto G, de Sousa M. Method for establishing serum ferritin reference values depending on sex and age. J Lab Clin Med. (1990) 116:779–84.
64. Jia F, Liu H, Kang S. NCOA4-mediated ferritinophagy: a vicious culprit in COVID-19 pathogenesis? Front Mol Biosci. (2021) 8:761793. doi: 10.3389/fmolb.2021.761793
65. Ashraf A, Clark M, So PW. The aging of Iron man. Front Aging Neurosci. (2018) 10:65. doi: 10.3389/fnagi.2018.00065
66. Levi S, Luzzago A, Cesareni G, Cozzi A, Franceschinelli F, Albertini A, et al. Mechanism of ferritin iron uptake: activity of the H-chain and deletion mapping of the ferro-oxidase site. A study of iron uptake and ferro-oxidase activity of human liver, recombinant H-chain ferritins, and of two H-chain deletion mutants. J Biol Chem. (1988) 263:18086–92. doi: 10.1016/S0021-9258(19)81326-1
67. Paul BT, Manz DH, Torti FM, Torti SV. Mitochondria and Iron: current questions. Expert Rev Hematol. (2017) 10:65–79. doi: 10.1080/17474086.2016.1268047
68. Crichton RR. Structure and function of ferritin. Angew Chem Int Ed Engl. (1973) 12:57–65. doi: 10.1002/anie.197300571
69. Hintze KJ, Theil EC. Cellular regulation and molecular interactions of the ferritins. Cell Mol Life Sci. (2006) 63:591–600. doi: 10.1007/s00018-005-5285-y
70. Harrison PM, Arosio P. The ferritins: molecular properties, iron storage function and cellular regulation. Biochim Biophys Acta. (1996) 1275:161–203. doi: 10.1016/0005-2728(96)00022-9
71. Benkovic SA, Connor JR. Ferritin, transferrin, and iron in selected regions of the adult and aged rat brain. J Comp Neurol. (1993) 338:97–113. doi: 10.1002/cne.903380108
72. Langlois d’Estaintot B, Santambrogio P, Granier T, Gallois B, Chevalier JM, Précigoux G, et al. Crystal structure and biochemical properties of the human mitochondrial ferritin and its mutant Ser144Ala. J Mol Biol. (2004) 340:277–93. doi: 10.1016/j.jmb.2004.04.036
73. Yang H, Yang M, Guan H, Liu Z, Zhao S, Takeuchi S, et al. Mitochondrial ferritin in neurodegenerative diseases. Neurosci Res. (2013) 77:1–7. doi: 10.1016/j.neures.2013.07.005
74. Alkhateeb AA, Connor JR. Nuclear ferritin: a new role for ferritin in cell biology. Biochim Biophys Acta. (2010) 1800:793–7. doi: 10.1016/j.bbagen.2010.03.017
75. Cohen LA, Gutierrez L, Weiss A, Leichtmann-Bardoogo Y, Zhang DL, Crooks DR, et al. Serum ferritin is derived primarily from macrophages through a nonclassical secretory pathway. Blood. (2010) 116:1574–84. doi: 10.1182/blood-2009-11-253815
76. Truman-Rosentsvit M, Berenbaum D, Spektor L, Cohen LA, Belizowsky-Moshe S, Lifshitz L, et al. Ferritin is secreted via 2 distinct nonclassical vesicular pathways. Blood. (2018) 131:342–52. doi: 10.1182/blood-2017-02-768580
77. Meyron-Holtz EG, Moshe-Belizowski S, Cohen LA. A possible role for secreted ferritin in tissue iron distribution. J Neural Transm. (2011) 118:337–47. doi: 10.1007/s00702-011-0582-0
78. Li JY, Paragas N, Ned RM, Qiu A, Viltard M, Leete T, et al. Scara5 is a ferritin receptor mediating non-transferrin iron delivery. Dev Cell. (2009) 16:35–46. doi: 10.1016/j.devcel.2008.12.002
79. Li L, Fang CJ, Ryan JC, Niemi EC, Lebrón JA, Björkman PJ, et al. Binding and uptake of H-ferritin are mediated by human transferrin receptor-1. Proc Natl Acad Sci USA. (2010) 107:3505–10. doi: 10.1073/pnas.0913192107
80. Finch CA, Bellotti V, Stray S, Lipschitz DA, Cook JD, Pippard MJ, et al. Plasma ferritin determination as a diagnostic tool. West J Med. (1986) 145:657–63.
81. Bartolini D, Stabile AM, Vacca C, Pistilli A, Rende M, Gioiello A, et al. Endoplasmic reticulum stress and NF-kB activation in SARS-CoV-2 infected cells and their response to antiviral therapy. IUBMB Life. (2022) 74:93–100. doi: 10.1002/iub.2537
82. Ma Q, Pan W, Li R, Liu B, Li C, Xie Y, et al. Liu shen capsule shows antiviral and anti-inflammatory abilities against novel coronavirus SARS-CoV-2 via suppression of NF-kappaB signaling pathway. Pharmacol Res. (2020) 158:104850. doi: 10.1016/j.phrs.2020.104850
83. Liu T, Zhang L, Joo D, Sun SCNF. -κB signaling in inflammation. Signal Trans Target Ther. (2017) 2:17023. doi: 10.1038/sigtrans.2017.23
84. Torti SV, Kwak EL, Miller SC, Miller LL, Ringold GM, Myambo KB, et al. The molecular cloning and characterization of murine ferritin heavy chain, a tumor necrosis factor-inducible gene. J Biol Chem. (1988) 263:12638–44. doi: 10.1016/S0021-9258(18)37801-3
85. Kobune M, Kato J, Miyazaki E, Niitsu Y. Interleukin-6 enhances hepatic transferrin uptake and ferritin expression in rats. Hepatology. (1994) 19:1468–75. doi: 10.1002/hep.1840190623
86. Konijn AM, Hershko C. Ferritin synthesis in inflammation. I. Pathogenesis of impaired iron release. Br J Haematol. (1977) 37:7–16. doi: 10.1111/j.1365-2141.1977.tb08758.x
87. Morikawa K, Oseko F, Morikawa S. A role for ferritin in hematopoiesis and the immune system. Leuk Lymphoma. (1995) 18:429–33. doi: 10.3109/10428199509059641
88. Lipinski P, Jarzabek Z, Broniek S, Zagulski T. Protective effect of tissue ferritins in experimental Escherichia coli infection of mice in vivo. Int J Exp Pathol. (1991) 72:623–30.
89. Milman N. Serum ferritin in danes: studies of iron status from infancy to old age, during blood donation and pregnancy. Int J Hematol. (1996) 63:103–35.
90. Milman N, Byg KE, Ovesen L. Iron status in danes 1994. II: prevalence of iron deficiency and iron overload in 1319 Danish women aged 40-70 years. Influence of blood donation, alcohol intake and iron supplementation. Ann Hematol. (2000) 79:612–21. doi: 10.1007/s002770000209
91. Grubic Kezele T, Curko-Cofek B. Age-related changes and sex-related differences in brain Iron metabolism. Nutrients. (2020) 12:9. doi: 10.3390/nu12092601
92. Lang CA, Naryshkin S, Schneider DL, Mills BJ, Lindeman RD. Low blood glutathione levels in healthy aging adults. J Lab Clin Med. (1992) 120:720–5.
93. Erden-Inal M, Sunal E, Kanbak G. Age-related changes in the glutathione redox system. Cell Biochem Funct. (2002) 20:61–6. doi: 10.1002/cbf.937
94. Iskusnykh IY, Zakharova AA, Pathak D. Glutathione in brain disorders and aging. Molecules. (2022) 27:1. doi: 10.3390/molecules27010324
95. Wang Y, Li Y, Wu Z, Chen Z, Yu H, He Y, et al. Ferritin disorder in the plasma and hippocampus associated with major depressive disorder. Biochem Biophys Res Commun. (2021) 553:114–8. doi: 10.1016/j.bbrc.2021.03.059
96. Cao H. Hippocampal proteomic analysis reveals activation of necroptosis and ferroptosis in a mouse model of chronic unpredictable mild stress-induced depression. Behav Brain Res. (2021) 407:113261. doi: 10.1016/j.bbr.2021.113261
97. Leggett BA, Brown NN, Bryant SJ, Duplock L, Powell LW, Halliday JW, et al. Factors affecting the concentrations of ferritin in serum in a healthy Australian population. Clin Chem. (1990) 36:1350–5. doi: 10.1093/clinchem/36.7.1350
98. Pitcher J, Abt A, Myers J, Han R, Snyder M. Neuronal ferritin heavy chain and drug abuse affect HIV-associated cognitive dysfunction. J Clin Invest. (2014) 124:656–69. doi: 10.1172/JCI70090
99. Nash B, Tarn K, Irollo E, Luchetta J, Festa L, Halcrow P, et al. Morphine-induced modulation of endolysosomal iron mediates upregulation of ferritin heavy chain in cortical neurons. eNeuro. (2019) 6:4. doi: 10.1523/ENEURO.0237-19.2019
100. Sengupta R, Burbassi S, Shimizu S, Cappello S, Vallee RB, Rubin JB, et al. Morphine increases brain levels of ferritin heavy chain leading to inhibition of CXCR4-mediated survival signaling in neurons. J Neurosci. (2009) 29:2534–44. doi: 10.1523/JNEUROSCI.5865-08.2009
101. Thomson AM, Rogers JT, Leedman PJ. Thyrotropin-releasing hormone and epidermal growth factor regulate iron-regulatory protein binding in pituitary cells via protein kinase C-dependent and -independent signaling pathways. J Biol Chem. (2000) 275:31609–15. doi: 10.1074/jbc.M002354200
102. Levenson CW, Fitch CA. Effect of altered thyroid hormone status on rat brain ferritin H and ferritin L mRNA during postnatal development. Brain Res Dev Brain Res. (2000) 119:105–9. doi: 10.1016/S0165-3806(99)00163-7
103. Videla LA, Valenzuela R. Perspectives in liver redox imbalance: toxicological and pharmacological aspects underlying iron overloading, nonalcoholic fatty liver disease, and thyroid hormone action. Biofactors. (2022) 48:400–15. doi: 10.1002/biof.1797
104. Pan Y, Jackson RT. Ethnic difference in the relationship between acute inflammation and serum ferritin in US adult males. Epidemiol Infect. (2008) 136:421–31. doi: 10.1017/S095026880700831X
105. Ulugerger Avci G, Bektan Kanat B, Suzan V, Can G, Korkmazer B, Karaali R. Clinical outcomes of geriatric patients with COVID-19: review of one-year data. Aging Clin Exp Res. (2022) 34:465–74. doi: 10.1007/s40520-021-02047-y
106. Oba S, Altınay M, Salkaya A, Türk HŞ. Evaluation of the effect of clinical characteristics and intensive care treatment methods on the mortality of covid-19 patients aged 80 years and older. BMC Anesthesiol. (2021) 21:291. doi: 10.1186/s12871-021-01511-6
107. Bianconi V, Mannarino MR, Figorilli F, Cosentini E, Batori G, Marini E, et al. The detrimental impact of elevated ferritin to Iron ratio on in-hospital prognosis of patients with COVID-19. Expert Rev Mol Diagn. (2022) 22:469–78. doi: 10.1080/14737159.2022.2052047
108. Bauer M, London ED, Silverman DH, Rasgon N, Kirchheiner J, Whybrow PC, et al. Thyroid, brain and mood modulation in affective disorder: insights from molecular research and functional brain imaging. Pharmacopsychiatry. (2003) 36:S215–21. doi: 10.1055/s-2003-45133
109. Dunn D, Turner C. Hypothyroidism in women. Nurs Womens Health. (2016) 20:93–8. doi: 10.1016/j.nwh.2015.12.002
110. Hahn P, Ying GS, Beard J, Dunaief JL. Iron levels in human retina: sex difference and increase with age. Neuroreport. (2006) 17:1803–6. doi: 10.1097/WNR.0b013e3280107776
111. Berge LN, Bonaa KH, Nordoy A. Serum ferritin, sex hormones, and cardiovascular risk factors in healthy women. Arterioscler Thromb. (1994) 14:857–61. doi: 10.1161/01.ATV.14.6.857
112. Tasci I, Balgetir F, Mungen B, Demir CF, Gonen M, Delen LA, et al. Evaluation of neurological disorders that develop concurrently with COVID-19 pneumonia: a retrospective analysis. Arq Neuropsiquiatr. (2022) 80:375–83. doi: 10.1590/0004-282x-anp-2021-0059
113. Ely EW, Gautam S, Margolin R, Francis J, May L, Speroff T, et al. The impact of delirium in the intensive care unit on hospital length of stay. Intensive Care Med. (2001) 27:1892–900. doi: 10.1007/s00134-001-1132-2
114. McCusker J, Cole MG, Dendukuri N, Belzile E. Does delirium increase hospital stay? J Am Geriatr Soc. (2003) 51:1539–46. doi: 10.1046/j.1532-5415.2003.51509.x
115. Morandi A, Di Santo SG, Zambon A, Mazzone A, Cherubini A, Mossello E, et al. Delirium, dementia, and in-hospital mortality: the results from the Italian delirium day 2016, a national multicenter study. J Gerontol A Biol Sci Med Sci. (2019) 74:910–6. doi: 10.1093/gerona/gly154
116. Hapca S, Guthrie B, Cvoro V, Bu F, Rutherford AC, Reynish E, et al. Mortality in people with dementia, delirium, and unspecified cognitive impairment in the general hospital: prospective cohort study of 6,724 patients with 2 years follow-up. Clin Epidemiol. (2018) 10:1743–53. doi: 10.2147/CLEP.S174807
117. Bickel H, Gradinger R, Kochs E, Förstl H. High risk of cognitive and functional decline after postoperative delirium. A three year prospective study. Dement Geriatr Cogn Disord. (2008) 26:26–31. doi: 10.1159/000140804
118. Jackson JC, Gordon SM, Hart RP, Hopkins RO, Ely EW. The association between delirium and cognitive decline: a review of the empirical literature. Neuropsychol Rev. (2004) 14:87–98. doi: 10.1023/B:NERV.0000028080.39602.17
119. Lima DP, Ochiai ME, Lima AB, Curiati JA, Farfel JM, Filho WJ. Delirium in hospitalized elderly patients and post-discharge mortality. Clinics. (2010) 65:251–5. doi: 10.1590/S1807-59322010000300003
Keywords: ferritin, COVID-19, delirium, critical illness *mortality, geriatric, psychiatric disorders
Citation: Abulseoud OA, Yehia A, Egol CJ, Nettey VN, Aly M, Qu Y, Skolnik AB, Grill MF, Sen A and Schneekloth TD (2022) Attenuated initial serum ferritin concentration in critically ill coronavirus disease 2019 geriatric patients with comorbid psychiatric conditions. Front. Psychiatry 13:1035986. doi: 10.3389/fpsyt.2022.1035986
Received: 03 September 2022; Accepted: 20 October 2022;
Published: 09 November 2022.
Edited by:
Abdel Ali Belaidi, University of Melbourne, AustraliaReviewed by:
Darius John Rowland Lane, University of Melbourne, AustraliaCarlos M. Opazo, University of Melbourne, Australia
Copyright © 2022 Abulseoud, Yehia, Egol, Nettey, Aly, Qu, Skolnik, Grill, Sen and Schneekloth. This is an open-access article distributed under the terms of the Creative Commons Attribution License (CC BY). The use, distribution or reproduction in other forums is permitted, provided the original author(s) and the copyright owner(s) are credited and that the original publication in this journal is cited, in accordance with accepted academic practice. No use, distribution or reproduction is permitted which does not comply with these terms.
*Correspondence: Osama A. Abulseoud, QWJ1bHNlb3VkLm9zYW1hQE1heW8uZWR1