- 1Michigan Neuroscience Institute, University of Michigan, Ann Arbor, MI, United States
- 2IUNICS, University of the Balearic Islands, Palma, Spain
- 3Health Research Institute of the Balearic Islands (IdISBa), Palma, Spain
- 4Department of Psychiatry, University of Michigan, Ann Arbor, MI, United States
Adolescent drug use reliably predicts increased addiction liability in adulthood, but not all individuals are equally impacted. To explore the biological bases of this differential reactivity to early life drug experience, we used a genetic rat model of temperament and evaluated the impact of adolescent cocaine exposure on adult psychomotor sensitization. Relative to adult bred low-responder (bLR) rats, bred high-responders (bHR) are more sensitive to the psychomotor-activating effects of cocaine and reinstate drug-seeking behavior more readily following prolonged cocaine exposure and/or abstinence. We found that a 7-day sensitizing cocaine regimen (15 mg/kg/day) during either adolescence or adulthood produced psychomotor sensitization in bHRs only, while a dual cocaine exposure prevented further sensitization, suggesting limits on neuroplasticity. By contrast, adolescent cocaine in bLRs shifted their resilient phenotype, rendering them more responsive to cocaine in adulthood following adolescent cocaine. To begin to explore the neural correlates of these behavioral phenotypes, we assessed two functionally opposite epigenetic chromatin modifications implicated in addiction liability, permissive acetylation (ac) and repressive tri-methylation (me3) on Histone 3 Lysine 9 (H3K9), in four striatal sub-regions. In bHRs, decreased H3K9me3 and increased acH3K9 in the nucleus accumbens (NAc) core associated with cocaine sensitization. In bLRs, the combination of cocaine exposure in adolescence and adulthood, which lead to an increased response to a cocaine challenge, also increased acH3K9 in the core. Thus, adolescent cocaine experience interacts with genetic background to elicit different behavioral profiles relevant to addiction in adulthood, with concurrent modifications in the epigenetic histone profiles in the NAc that associate with cocaine sensitization and with metaplasticity.
Introduction
Substance use disorder (SUD) is a complex, pernicious and pervasive condition negatively impacting millions around the world and costing hundreds of billions of dollars in the United States alone (1, 2). However, despite years of research, widely effective treatments remain elusive1 (3). One reason may be the confounding contribution of individual differences. SUD is highly heritable (30–70%) (4), and interrelated with other psychiatric diseases, often sharing common neurobiology (5–7). Twin studies suggest SUD and externalizing disorders, including novelty seeking, have shared genetic influence (8, 9), while early-onset depressive or internalizing disorders appear to be inherent predisposing factors, often co-morbid in persons with SUD and predictive drug dependence problems later in adulthood (10–12). This suggests that temperamental tendencies might contribute to drug use and misuse likely through multiple paths—e.g., novelty seeking in some cases, and self-medication for negative affect in others. Moreover, environmental factors, such as drug availability, childhood adversity, socioeconomic distress, and the impact of chronic drug experience are strongly associated with addiction outcome. Understanding how gene × environment interactions contribute to addiction liability is therefore an important area of investigation that will help improve treatment options for individuals with SUD as well as informing prevention strategies.
Most individuals with SUD initiate drug use before the age of 18 and then quickly escalate use, resulting in a life-long problem by adulthood (13–15). Younger age of onset often predicts greater risk of SUD later in life (12, 16, 17) and drug abuse during adolescence, a sensitive period when these environmental and stress-related effects are most formative, seems to be a consistent factor in developing long-term addiction liability (18), and could be modeled in rodents [reviewed by (19–21)]. However, despite these relationships, only a small minority of individuals who use illicit drugs develop SUD. Therefore, it is likely that a critical combination of individual differences (i.e., inherent predisposing and environmental risk factors) work in concert to establish lifelong addiction vulnerability (22).
The field of neuroepigenetics provides biological evidence that genes are accompanied by heritable epigenomic profiles (e.g., chromatin structure) that can become altered by life experiences, including chronic drug use (23, 24). Thus, under the right circumstances even inborn genetic transcription profiles can become modified by environmental experience, altering inherent affective phenotypes (25–28). In agreement, several groups have shown that a history of drug experience can induce epigenetic modifications that influence gene expression and contribute to addiction vulnerability both within a lifetime (29–31) and across generations (32–34). While epigenetic modifications occur throughout the brain, changes in particular regions within the striatum (e.g., the nucleus accumbens, NAc, core) have been shown to directly influence dopamine-rich mesolimbic medium spiny neurons, which play a role in addiction-related behaviors (35). For example, selective histone modification on two opposing Histone 3 Lysine 9 (H3K9) modifications (acetylation and tri-methylation) in the NAc core, manipulated via engineered zinc finger proteins targeting Cdk5 gene expression, mediates cocaine conditioned place preference and psychomotor sensitization in mice (36). In particular, overexpressing permissive acH3K9 produced greater sensitization, while overexpressing similar restrictive di-methylation H3K9 blunted sensitization in mice (36).
Adolescence is a critical period where chromatin structure is being modified by hormonal and other factors, and extensive drug use during adolescence is likely to have a strong effect on chromatin structure, possibly impacting the neurobiological substrates that contribute to addiction-related phenotypes. However, the interplay between genetic background and the epigenetic response to chronic drug exposure, especially during adolescence, remains understudied. Here, we used our bred High-responder (bHR) and Low-responder (bLR) rat model to investigate whether genetic predisposition intersects with adolescent cocaine experience to alter addiction-related behaviors and epigenetic profiles in adulthood.
Our two lines of rats are bred based on their locomotor response to a novel environment, but concurrently differ on a number or addiction-related measures, including psychomotor sensitization, acquisition of drug-taking, response to drug cues, and dopamine receptor 1 (D1) and 2 (D2) RNA profiles in the NAc (37–40). Now bred across multiple generations, these rats reliably exhibit distinct and extreme novelty-seeking and addiction-related phenotypes that are predicted with 99% certainty (40). This a priori knowledge allowed us to test whether adolescent cocaine history augments or redirects their respective inborn phenotypes and track changes to their epigenetic profiles. We measured psychomotor sensitization and profiled two opposing H3K9 modifications (acetylation and tri-methylation) previously implicated in addiction-related behaviors across four striatal sub-regions of bHR and bLR rats following exposure to cocaine (or saline): 1) in adolescence only, 2) in adulthood only, or 3) in both adolescence and adulthood. A priori we expected to find associations between signs of psychomotor sensitization and the regulation of epigenetic markers (i.e., decreased restrictive H3K9me3 and increased permissive acH3K9) in a region-specific manner (changes expected in NAc core). While most other studies examined epigenetic changes in bulk, at the regional level, we sought to examine the changes at a more cellular level within the striatum. Thus, we relied on a combination of immunohistochemistry and unbiased stereology to more precisely visualize the epigenomic changes associated with exposure to cocaine.
Materials and methods
Animals
A total of 150 male Sprague–Dawley rats (70 bHR and 80 bLR) from generation F43 were used in this study. For unknown reasons, one bLR rat died prior to initial locomotor testing and his cage-mate was subsequently excluded. Rats were housed in pairs in standard controlled environmental conditions (22°C, 70% humidity, and 12-h light/dark cycle, lights on at 06:00 h) with food and water available ad libitum. All animal procedures complied with the ARRIVE guidelines and were performed in accordance with the University of Michigan animal care committee’s regulations and followed the Guide for the Care and Use of Laboratory Animals: Eight Edition (41). Ideally, we would have included both sexes in our study, however, no female rats were available at the time of our experiments, and therefore the effect of sex as a biological variable could not be included in the experimental design.
Basal locomotor response to novelty
All rats were tested for locomotor response to a novel environment, the phenotype for which bHR and bLR rats were bred, during early life (P25) between 9:30 AM and 12:30 PM in a different room from where rats were housed. Rats were placed into clear acrylic 43.0 × 21.5 × 25.5 cm tall cages equipped with infrared photocell emitters mounted 2.3 and 6.5 cm above a grid floor placed on top of standard bedding. A customized computer with a locomotion-testing rig and motion-recording software created in-house at the University of Michigan was used to measure lateral and rearing activity. Each locomotor test session lasted 60 min and the data were collected and recorded in 5-min bins. Locomotion scores for each rat were calculated as the sum of horizontal and rearing movements for the entire session. Recorded locomotor scores were used to counterbalance all subsequent testing conditions within bHR or bLR phenotypes (see Supplementary Figure 1 and Supplementary Table 1).
Drug treatments and psychomotor activating effects of cocaine
A total of 60 bHR and 78 bLR rats were intraperitoneally (i.p.) administered with either cocaine (15 mg/kg) or saline once daily for 7 consecutive days during adolescence (P33-39) (Figure 1). While a cohort of rats was sacrificed 24 h after treatment (P40) (20 bHR and 38 bLR; see Figure 1), the other cohort was exposed to the same regimen of either saline or cocaine administration in adulthood (P76-84), providing 8 experimental groups (see n/group in Figure 1). Another group of 10 bHR rats was only exposed to cocaine in adulthood (no drug treatment during adolescence; ND-Cocaine; Figure 1) in an attempt to evaluate whether adolescent saline treatment (and/or the stress associated with the procedure) could alter the behavioral outcome in bHR adult rats (i.e., bHR-Sal-Cocaine vs. bHR-ND-Cocaine comparisons). Since no behavioral differences were observed between both groups (data not shown), rats were combined in a group of 20 rats in which to evaluate the impact of adult cocaine exposure with no prior history of cocaine on the emergence of psychomotor sensitization. This selected cocaine regimen was previously shown to induce psychomotor sensitization in adult outbred (42) and bHR rats (43), and to induce long-term behavioral changes [e.g., (44–47)] when given at this time-window during early-mid adolescence.
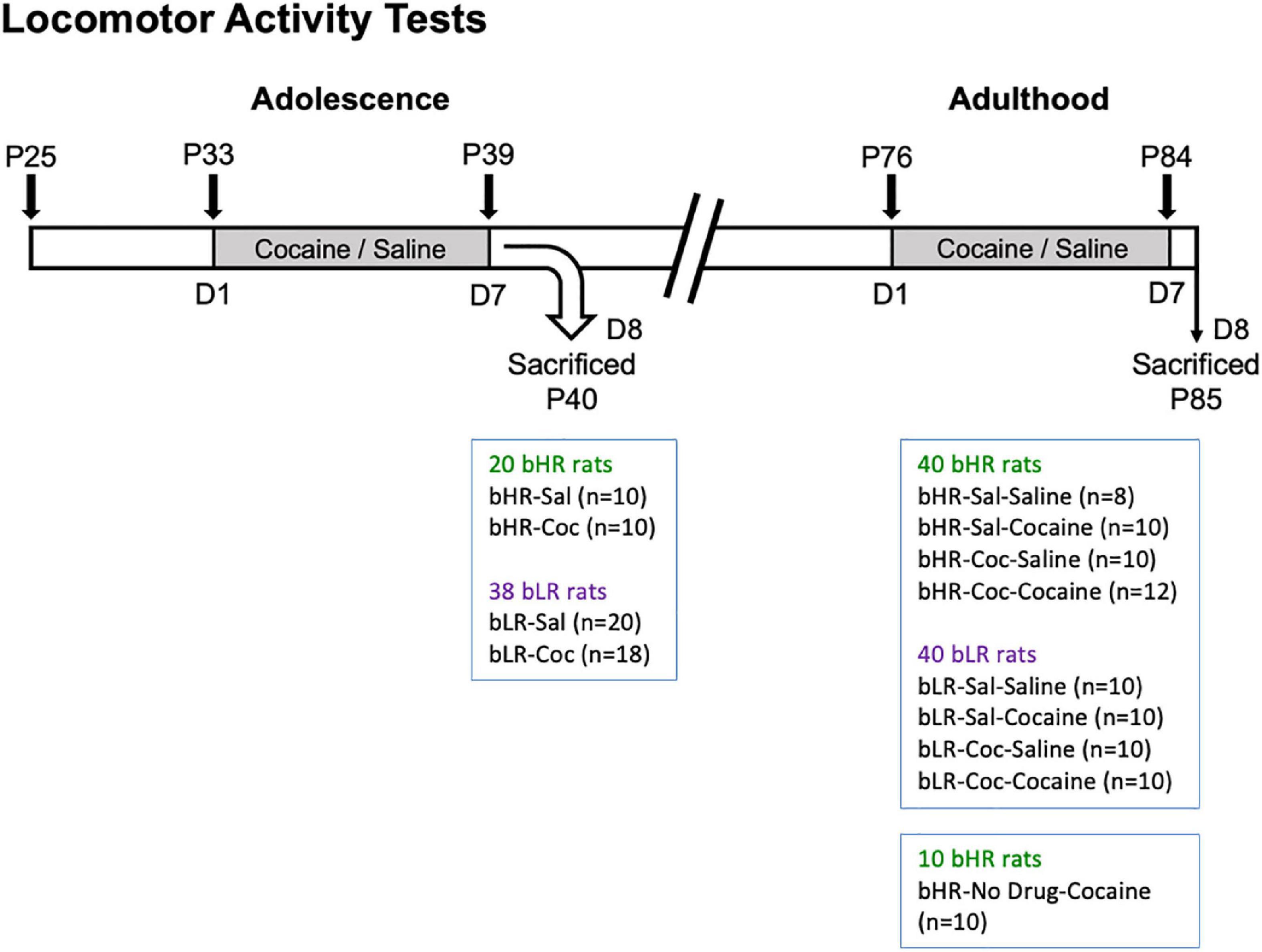
Figure 1. Drug treatments and psychomotor activating effects of cocaine. Locomotor Activity Tests. P: post-natal day; Gray: drug treatment period. bHR and bLR rats were initially tested for locomotor exploration of a novel environment (P25, Supplementary Figure 1). bHRs and bLRs were then assigned to different treatment conditions where either saline or cocaine was administered in adolescence (P33-39), adulthood (P76-84), or both. A subset of these bHRs and bLRs were sacrificed 24 h (D8, P40) following the adolescent cocaine sensitization regimen to assess epigenetic changes at this timepoint. All other conditions were exposed to the second regimen of either saline or cocaine later in adulthood (P76-P84) and subsequently sacrificed (P85).
Psychomotor sensitization tests were performed during adolescence, adulthood, or both. On the first day (D1) of each sensitization regimen (P33 or P76), rats were transferred to a dedicated testing room where they were placed into custom-made activity chambers (45) and were allowed to habituate for 2 h before receiving an injection of either cocaine (15 mg/kg, i.p.) or saline (0.9% NaCl, 1 ml/kg, i.p.). This was immediately followed by 1 h of locomotor testing before being returned to their home cages. For the next 5 days (P34-38, and/or P77-83), rats were injected once daily with 15 mg/kg of cocaine or saline in an alternate room at the same time of day as the first test. Rats were placed back into their home cages immediately following injections and returned to their colony room. On D7 of testing (P39 and/or P84), rats were again returned to the activity chambers where they were tested on D1 and allowed to habituate for 2 h before receiving an injection of either cocaine or saline followed by 1 h of behavioral scoring.
Test equipment and analysis of signs of psychomotor sensitization
As previously described (45) locomotor activity test chambers were made of expanded PVC (33.0 × 68.6 × 61.0 cm tall) with stainless steel woven-wire cloth grid floors (30.5 × 61.0, 7.6 × 7.6 cm squares), placed above a metal catch tray. Animal behavior was digitally video recorded using IC Capture 2.2 software2 and USB 2.0 monochrome industrial video cameras (The Imaging Source LLC, Charlotte, NC, United States) installed directly above each activity chamber. Recordings captured behavior during a 2-h habituation period and for 1 h following drug administration. These video files were transferred to an offline computer server for subsequent behavior analysis using TopScan 2.0 software (Clever Sys, Inc., Reston, VA, United States3). Several measurements were used to ascertain signs of psychomotor sensitization as described by Flagel and Robinson (48). Locomotor activity was measured as the distance traveled in mm from one end of the chamber to the other based on user-defined areas of the activity chamber, and the velocity (mm/s). The number of darting bouts, which is defined as an uninterrupted instance of fast locomotion (≥ 95 mm/s as the predetermined velocity) was also measured. Finally, stereotypy was measured by TopScan using the frequency of head-waving movements: the total number of lateral head-waving movements divided by the time spent in place (no./s).
Tissue collection
Rats were killed by rapid decapitation using a guillotine 24 h following the last day of cocaine or saline administration during adolescence (D8: P40) or in adulthood (D8: P85; see Figure 1). The extracted brains were quickly frozen in a −30°C isopentane solution and stored at −80°C until further processing. For each rat, 40 μm coronal sections were cryostat cut through the rostro-caudal extent from cortex to the brainstem and slide-mounted (Superfrost Plus, VWR). Mounted sections were then stored at −80°C for subsequent immunohistochemistry (IHC) assays.
Histone modification quantification visualization
Tri-methyl and acetylated H3K9 epigenetic marks (H3K9me3 and acH3K9 respectively) were systematically quantified following 3,3’-diaminobenzidine (DAB) immunohistochemistry for regional quantification in striatal regions (Figure 2). We employed 6 level-matched coronal hemi-sections per animal (of 2 slides covering the full rostral-to caudal extent of striatum). Immunohistochemistry experiments followed a standardized protocol (43) where tissue was post-fixed in 4% paraformaldehyde and incubated at 90°C for 50 min in 0.1 M sodium citrate (Fisher, #S379-3, pH 6.0) for epitope retrieval. Sections were then rinsed with Tris-buffered saline (TBS), washed in 0.3% peroxide, blocked with bovine serum albumin containing 1% goat serum and 0.05% Triton X-100, and incubated overnight with either monoclonal rabbit anti-H3K9me3 (1:2,000; Abcam, ab8988, United States) or polyclonal anti-acH3K9 (1:5,000; Cell Signaling, #9649, United States). After PBS washes, sections were incubated in biotinylated anti-rabbit secondary antibody 1:500 (Vectors Laboratories, #BA-1000) followed by Avidin/Biotin complex amplification (Vectastain Elite ABC kit; Vectors Laboratories, #PK-6100) and DAB (Sigma Life Science, #5905) mixed with 3% hydrogen peroxide for signal amplification. Finally, all slides underwent dehydration through graded alcohols, xylene immersion, and were subsequently coverslipped using Permount® mounting medium (Fisher, #SP15).
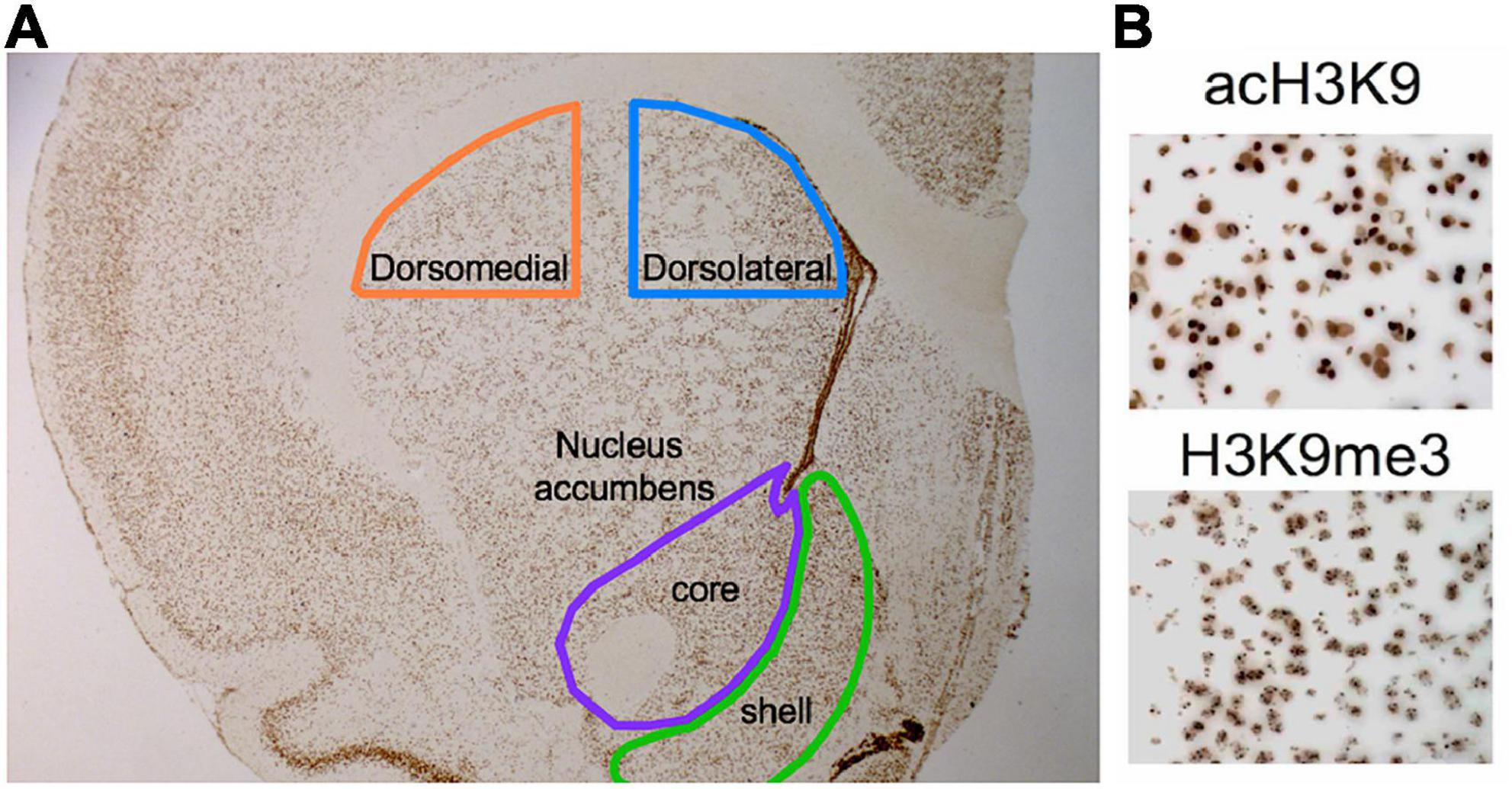
Figure 2. Histone modification quantification visualization. A modified combination of immunohistochemistry and unbiased stereology was used to quantify acH3K9 and H3K9me3 histone modifications within defined anatomical sub-regions of the striatum: dorsomedial (dmSTR), dorsal lateral (dlSTR), nucleus accumbens (NAc) core and shell. Each area was outlined with hand-drawn contours based on Paxinos and Watson brain atlas (2012) (A), and then quantified using unbiased stereology (see section “Materials and methods”). Antibody concentrations for acH3K9 and H3K9me3 were optimized using a titration curve and visualized using diaminobenzidine (DAB) for easy counting (B).
Unbiased stereology
Unbiased stereology was performed using a Zeiss Axio Imager M2 motorized fluorescent microscope at 40x or 63x oil. DAB immunohistochemistry-processed slides representing every animal were randomized and coded prior to analysis. Trained personnel blind to the treatment conditions performed all of the stereology. Systematic Random Sampling (SRS) was performed on each section using Stereo Investigator software (MBF) with frames (60 × 60 μm2) on a counting grid (150 × 150 μm2). Optical dissector height was 20 μm with 2 μm upper and lower guard zones to avoid quantification of partial cells and cutting artifacts. All counting protocols used a Gundersen coefficient (m = 1) of < 0.1 and near equal values between treatment conditions (CE = ∼0.04) to ensure count accuracy and consistency (49). Some brains did not stain well enough for optimal stereological quantification, but attrition was random, small, and no condition had fewer than 6 rats for analysis of epigenetic modifications. Only cells showing unambiguous DAB signal were counted. Each “count” reflects a cell with a modification present.
Statistical analysis
All graphical representations of the data and the corresponding statistical analysis were performed with GraphPad Prism, Version 9 (GraphPad Software, San Diego, CA, United States). Results are expressed as mean values ± standard error of the mean (SEM) and individual symbols for each rat are shown within bar graphs. Given the baseline differences in locomotor activity between bHR and bLR rats (Supplementary Figure 1), the statistical comparisons evaluating the psychomotor effects of cocaine were performed in each phenotype separately. In particular, following the adolescent treatment, two-way repeated measures (RM) ANOVAs or mixed-effects models (if there were any missing values) were used with the following independent variables: Adolescent Treatment and Day of testing (D1 vs. D7), and followed by Sidak’s multiple comparison tests when appropriate. Following the adult treatment, three-way RM ANOVAs were performed, which included 3 independent variables (Adolescent Treatment, Adult Treatment, Day of testing; see Supplementary Table 1). When evaluating the changes in epigenetic markers (H3K9me3 and acH3K9) in the NAc (core and shell), Student t-tests were performed to assess the effects of adolescent cocaine (D8, 24 h post-adolescent treatment), while two-way ANOVAs were utilized (independent variables: Adolescent Treatment and Adult Treatment) following the adult treatment (D8, 24 h post-adult treatment). Sidak’s multiple comparisons tests were used for post hoc comparisons when appropriate. For statistical analysis during adulthood 4 experimental groups (Sal-Saline, Sal-Cocaine, Coc-Saline, Coc-Cocaine) were included; however, graphs were split accordingly to history of cocaine for simplicity and visualization. The level of significance was set at p ≤ 0.05.
Results
Adolescent cocaine differentially impacts psychomotor sensitization in divergent affective phenotypes
Effects during adolescence: During adolescence, both acute (D1) and chronic (D7) cocaine treatment regimens elicited psychomotor activation in bHR (Figures 3A,B) and bLR (Figures 3C,D) rats as compared to saline-treated rats (***p < 0.001 or **p < 0.01; Figures 3A–D; complete statistical results are reported in Supplementary Table 1). For each phenotype there was a significant main effect of Treatment, supported by increased locomotor activity (i.e., distance traveled, Figures 3A,C; locomotor velocity, darting bouts, Supplementary Figure 2) and head-waving frequency (Figures 3B,D) on D1 and D7. However, only for bHR rats was there a significant effect of Day (D7 vs. D1, #p < 0.05) for locomotor activity (see Supplementary Table 1). Thus, following repeated drug exposure in adolescence only bHRs show evidence of psychomotor sensitization (Figure 3A).
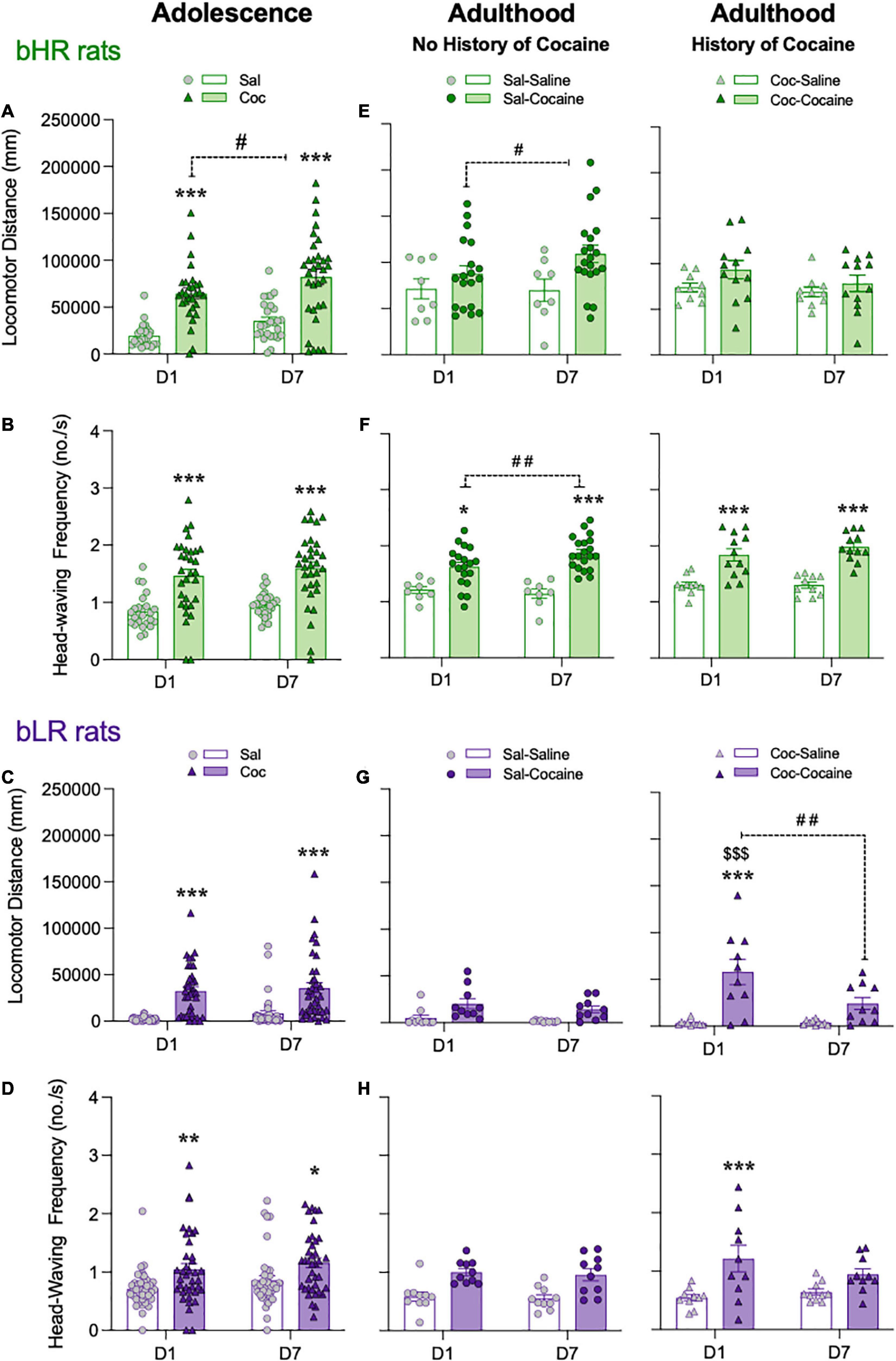
Figure 3. Adolescent cocaine differentially impacts psychomotor sensitization in divergent affective phenotypes. Acute (D1) and chronic (D7) psychomotor activating effects of cocaine administration in adolescent (A–D) and/or in adulthood [with no or with a prior history of adolescent cocaine; (E–H)] in bHR and bLR rats. Data represents the mean values ± SEM of the locomotor distance traveled in mm (A,C,E,G) and the frequency of head-waving (B,D,F,H). Individual values are shown for each rat (symbols). Two- or three-way RM ANOVAs were used for statistical analysis (see Supplementary Table 1 for more details). *p < 0.05, **p < 0.01 and ***p < 0.001 when comparing cocaine-treated rats with the corresponding saline-treated group (main Effect of Treatment). ##p < 0.01 and #p < 0.05 when comparing D7 vs. D1 responses for the same treatment group (main Effect of Day). p < 0.001 when comparing the response on D7 of the adolescent treatment vs. the one following an acute challenge dose in adulthood (D1 for Coc-Cocaine group).
Effects during adulthood: In bHRs, the psychomotor effects of adult cocaine exposure where only observed for locomotor distance (Figure 3E) and head-waving frequency (Figure 3F) (see the overall main effects of Treatment and other statistical results reported in Supplementary Table 1). In bHR rats there was a significant Day × Adult Treatment interaction when evaluating head-waving frequency (see Supplementary Table 1), and Sidak’s multiple comparisons test revealed an increase in locomotor distance and head-waving frequency on D7 as compared to D1 for rats treated with cocaine (#p < 0.05 and ##p < 0.01 respectively; see Figures 3E,F). However, bHRs exposed to adult cocaine and with a prior history of adolescent cocaine showed similar locomotor activity levels (i.e., locomotor distance and velocity, darting bouts) and head-waving frequency movements on D1 and D7 in adulthood, and as compared to rats that only received cocaine in adulthood (Figures 3E,F and Supplementary Figure 2). Thus, bHR rats that were exposed to cocaine in adolescence do not exhibit further sensitization in adulthood.
In bLR rats with a history of cocaine exposure during adolescence, the psychomotor effects of adult cocaine treatment were observed for all measurements (i.e., locomotor distance and velocity, darting bouts, head frequency; see main effects of Adult Treatment on Supplementary Table 1, Figures 3G,H, and Supplementary Figure 2). These rats showed greater locomotor activity and head-waving frequency in response to a single cocaine injection (D1 for Coc-Cocaine group; Figures 3G,H) relative to those treated with saline in adulthood (***p < 0.001) or those treated with a repeated cocaine treatment during adolescence (p < 0.001 vs. the locomotor distance on D7 of the adolescent treatment). Notably, the increase in locomotor activity on D1 was followed by a reduction on D7 (##p < 0.01; Figure 3G), suggesting a lack in the classic pattern of adult sensitization (increased activity from D7 vs. D1).
Adolescent cocaine differentially impacts epigenetic levels in the nucleus accumbens core of divergent affective phenotypes
Prior to evaluating the impact of drug treatments in adolescence and/or adulthood, we ascertained potential baseline differences in the levels of H3K9me3 and acH3K9 with age (adolescence P40 vs. adulthood P85) in saline-treated rats. There were no significant differences between bHR and bLR rats at baseline in either histone marker in the NAc core or NAc shell (Supplementary Figure 3).
Effects during adolescence: Chronic adolescent cocaine treatment reduced H3K9me3 in the NAc core of bHR rats (−42,476 ± 15,169, *p = 0.0150 vs. Sal treated rats; Figure 4A), but not bLR rats (Figure 4B), when measured 24 h after the last dose (D8) (see Supplementary Table 1). In contrast, there was an increase in acH3K9 in the NAc core (+ 24,757 ± 12914, *p = 0.0421 vs. Sal treated rats) in bHR rats treated with cocaine (Figure 4E), but not bLR rats (Figure 4F). There were no significant effects of cocaine treatment on either H3K9me3 (Figures 4C,D) or acH3K9 (Figures 4G,H) in the NAc shell in bHR or bLR rats 24 h after the last treatment.
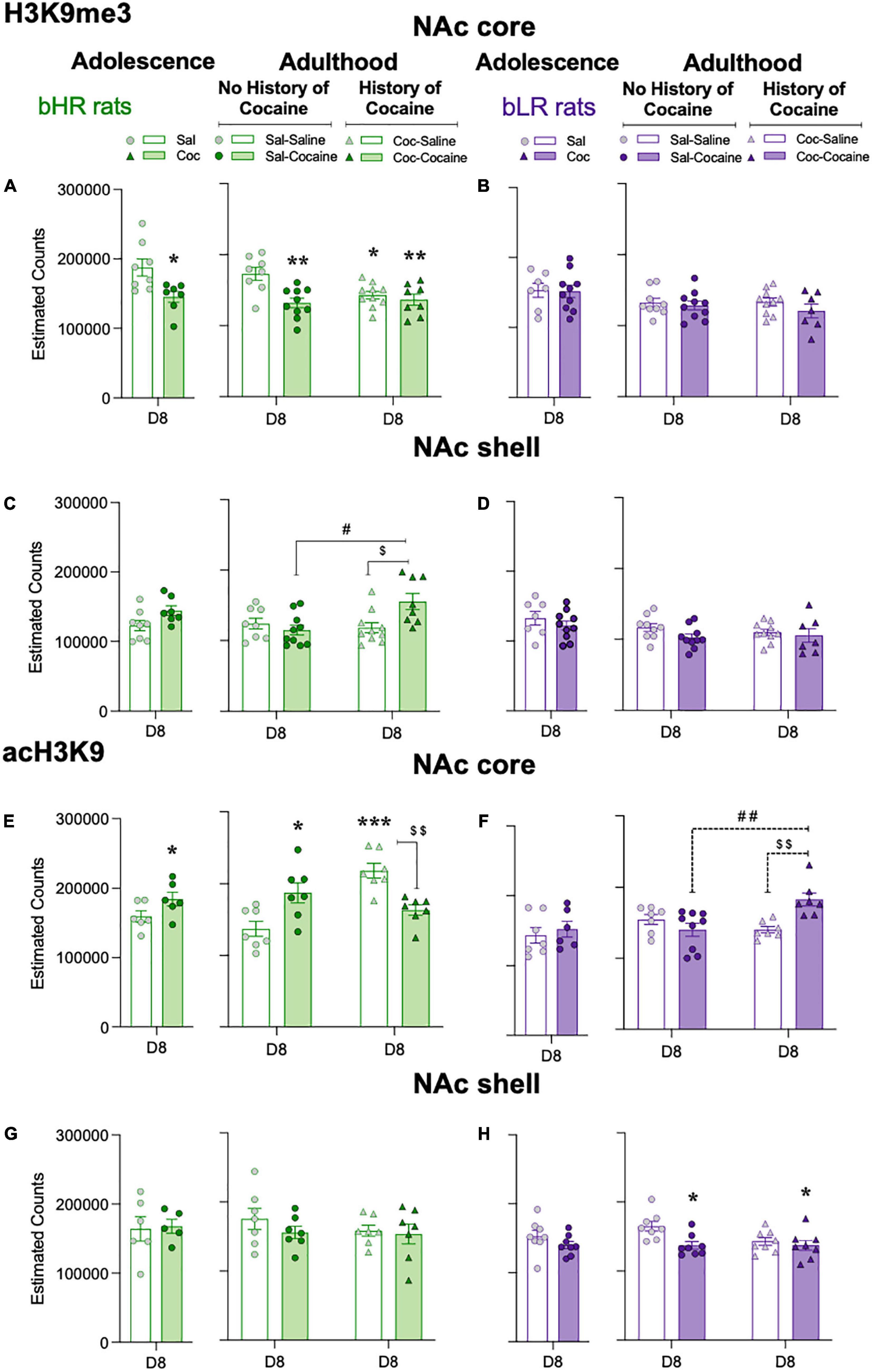
Figure 4. Adolescent cocaine differentially impacts epigenetic levels in the nucleus accumbens core of divergent affective phenotypes. Repressive H3K9me3 (A–D) and permissive acH3K9 (E–H) cell counts in the NAc core and shell of bHRs and bLRs treated with adolescent and/or adult cocaine. Data represents the mean values ± SEM of the estimated cell counts of each marker as measured 24 h post-adolescent or adult treatment (D8). Individual values are shown for each rat (symbols). Student t-tests were performed to assess the effects of adolescent cocaine, while two-way ANOVAs were utilized following the adult treatment (see Supplementary Table 1 for more details). *p < 0.05, **p < 0.01, and ***p < 0.001 when comparing cocaine-treated rats with the corresponding saline-treated group (main Effect of Treatment). ##p < 0.01 and #p < 0.05 when comparing rats with and without a prior history of adolescent cocaine (Coc-Cocaine vs. Sal-Cocaine). p < 0.01 and $p < 0.05 when comparing Coc-Cocaine vs. Coc-Saline groups.
Effects during adulthood: In adulthood, H3K9me3 was again decreased in the NAc exclusively in bHR rats (Figure 4A). Specifically, two-way ANOVAs revealed Adolescent × Adult Treatment interactions in both the NAc core and NAc shell (see Supplementary Table 1). In the NAc core (see Figure 4A), Sidak’s multiple comparisons tests showed that adult cocaine administration reduced the levels of H3K9me3 in bHR rats (Sal-Cocaine: −41,123 ± 6,579, **p = 0.0017 vs. Sal-Saline). Moreover, rats with a history of adolescent cocaine and treated with saline in adulthood still showed reduced H3K9me3 levels in the NAc core (Coc-Saline: −30,162 ± 5,372, *p = 0.0317 vs. Sal-Saline). The combination of cocaine exposure during both adolescence and adulthood resulted in decreased levels of H3K9me3 (Coc-Cocaine −36,574 ± 7,745, **p = 0.0098 vs. Sal-Saline), and with a similar magnitude of change between those groups that received cocaine during adolescence and those that received cocaine in adulthood (Figure 4A). In the NAc shell (Figure 4C), the combination of adolescent and adult cocaine treatment (Coc-Cocaine) resulted in higher levels of H3K9me3 in bHR rats, compared to those that received cocaine only during adolescence (+ 37,223 ± 11,252, $p = 0.0227 vs. Coc-Saline) or in adulthood (+ 40,755 ± 11,935, #p = 0.0105 vs. Sal-Cocaine).
For acH3K9 in the NAc, two-way ANOVAs revealed Adolescent × Adult Treatment interactions in the NAc core for both bHR (Figure 4E) and bLR (Figure 4F) rats (see Supplementary Table 1 for statistical analysis). In bHR rats, a single regimen of cocaine exposure (either in adolescence or adulthood) increased acH3K9 (Sal-Cocaine: + 50,299 ± 14,069, *p = 0.0179; Coc-Saline: + 81,239 ± 10,299, ***p = 0.0001) relative to saline-treated (Sal-Saline) rats (Figure 4E). However, acH3K9 was not affected in bHR rats that received a combination of adolescent and adult cocaine treatment (Coc-Cocaine: −55,097 ± 7,227, ##p = 0.0082 vs. rats that just receiving adolescent cocaine, Coc-Saline; Figure 4E). In bLR rats, the combination of adolescent and adult cocaine (Coc-Cocaine) treatment resulted in higher levels of acH3K9 in the NAc core when compared to those that only received cocaine during adolescence (+ 42,325 ± 11,627, p = 0.0071 vs. Coc-Saline) or in adulthood (Sal-Cocaine: + 42,494 ± 10,962, ##p = 0.0039) (Figure 4F). In the NAc shell, acH3K9 was decreased in bLR rats following cocaine treatment in adulthood (Figure 4H).
To further examine the long-term impact of adolescent cocaine exposure on brain regions involved in psychomotor sensitization, we also quantified the number of cells levels of acH3K9 in the dorsomedial (dmSTR) and dorsolateral (dlSTR) regions of the striatum in adult bHR and bLR rats (Supplementary Figure 4). There were no significant changes induced by cocaine exposure (adolescent, adult or both) in these subregions in either bHR or bLR rats (Supplementary Figure 4 and Supplementary Table 1).
Discussion
In the present study, we analyzed the interplay of genetic predisposition and exposure to cocaine during adolescence in shaping behavioral responsiveness to cocaine during adulthood. We asked whether the unique behavioral phenotypes that are shaped by these gene × environment interactions are associated with distinct epigenetic profiles. In particular, we studied whether exposure to chronic cocaine alters the balance of a repressive and a permissive modified histone differentially, as a function of genetic background.
Our behavioral findings show the following: a) bHR rats, which are vulnerable to psychostimulant use and exhibit a number of addiction-related behaviors (38, 40, 50) are prone to sensitization in both adolescence and adulthood across several measures of locomotor behavior; b) In bHRs, previous exposure to cocaine sensitization during adolescence abrogates the development of sensitization in adulthood. This phenomenon is termed “metaplasticity,” and refers to the concept that a history of neuroplasticity alters the organism’s subsequent ability to mount subsequent neuroplastic changes (51); c) Adult bLRs, which are comparatively resilient to psychostimulant use, are only mildly reactive to either acute or chronic cocaine. By contrast, adolescent bLRs show greater locomotor activation in response to acute and chronic cocaine, although they are still less responsive than bHRs. However, at neither age do bLRs show cocaine sensitization in the sense of an increased locomotor response on D7 relative to D1; d) In bLRs, a previous exposure to cocaine during adolescence produces an unusual pattern of reactivity in adulthood. The initial cocaine challenge in adulthood elicits a pronounced locomotor response in comparison to bLRs with no history of cocaine. This is evidence of sensitization triggered by adolescent exposure that becomes manifest on the initial cocaine challenge in adulthood. However, following 7 days of exposure to cocaine in adulthood, this enhanced initial response appears resistant to further sensitization on most measures of locomotion, and shows a significant reduction in locomotor distance traveled. Thus, the adolescence-induced sensitization to cocaine in adult bLRs appears to be transient. Overall, cocaine exposure during adolescence caused enduring effects into adulthood with distinct sensitization phenotypes as a function of genetic predisposition.
Our epigenetic findings show the following: a) In bHRs, sensitization was associated with a decrease in the repressive H3K9me3 and an increase in the permissive acH3K9 in the NAc core, a combination expected to lead to an increase in net transcriptional activation. These sensitization-induced changes appear to be persistent (i.e., bHR with a history of adolescent cocaine who receive saline as adults have a profile resembling the adolescent sensitization profile, with decreased H3K9me3 and increased acH3K9); b) In bHRs with dual exposure to cocaine in adolescence and adulthood, the modified histones showed either no additional changes (H3K9me3) or a reversal (acH3K9), consistent with metaplasticity; c) In bLRs, there are no decreases in H3K9me3 in the NAc core in any of the experimental groups; d) In the bLRs, there is an increase in the permissive acH3K9 in the NAc core only in adults with a history of cocaine exposure during adolescence. It is notable that this group showed evidence of transient sensitization to cocaine.
Overall, sensitization in its typical manifestation seen in bHRs is associated with a decrease in the repressive H3K9me3 and increase in a the permissive acH3K9. Limits on their changes are associated with limits to behavioral sensitization (see Figure 5). By contrast, an increase in acH3K9 alone, while associated with an increased responsiveness to an initial cocaine challenge in bLRs is not sufficient to support the expression of sustained sensitization. These findings, and additional behavioral and epigenetic observations, are further discussed below.
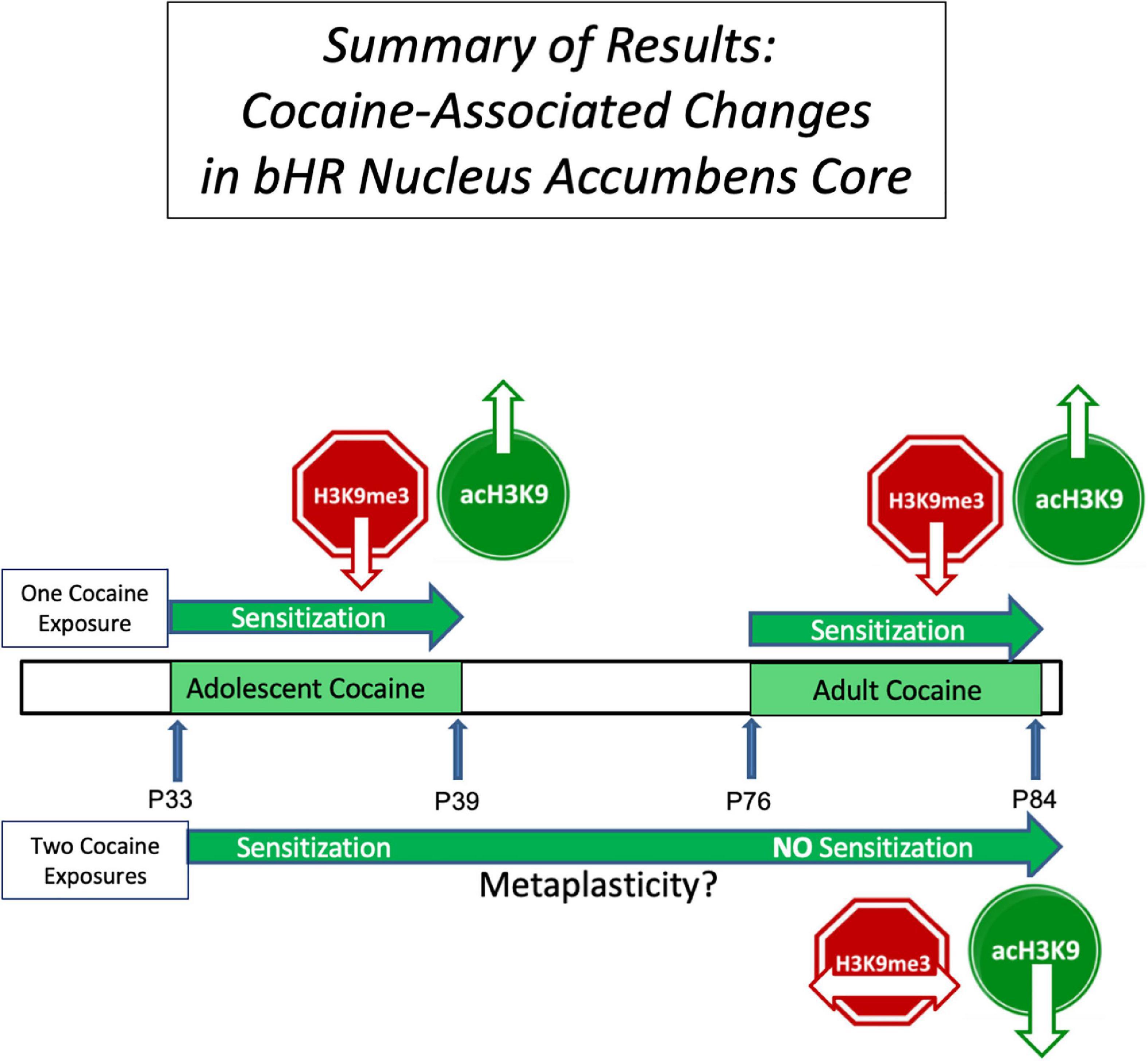
Figure 5. Summary of the Results: This figure summarizes some of the results of this study and serves as a framework for further work testing the potential causative role of these epigenetic changes in cocaine sensitization. It focuses on the nucleus accumbens core of bHR rats (downward arrow indicates a decrease, upward arrow an increase, and horizontal arrow no change). The top bar illustrates the findings in bHRs receiving cocaine only once, either in adolescence or in adulthood, compared to those receiving saline at the same time. We observed a decrease in repressive histone, H3K9me3 (red) and increased permissive histone, acH3K9 (green), associated with cocaine sensitization in both adolescence and adulthood. The lower bar shows bHR rats with a history of cocaine exposure in adolescence. Thus, they received cocaine twice (Coc-Cocaine), and are compared to those who received saline in adolescence (Sal-Cocaine). bHRs with a history of adolescent cocaine exposure show no evidence of behavioral sensitization in adulthood and no change in H3K9me3. This decreased evidence of behavioral and neural plasticity following previous experience is consistent with the notion of metaplasticity.
The impact of adolescent and adult exposure to cocaine on behavioral sensitization
bHR rats are largely considered more addiction liable as they inherently exhibit greater risk-taking and exploratory behaviors, more readily acquire self-administration of cocaine, show greater spontaneous dopamine release, higher D2 mRNA expression in the dorsal striatum, and greater locomotor responsiveness to psychostimulants, which may facilitate initiation of drug seeking (38, 40, 50). However, bLRs, the more anxious and depressive-like phenotype, may be more responsive to drugs that alleviate these symptoms, and are generally more sensitive to the neurobiological impact of aversive stimuli. Since either phenotype could exhibit drug abuse liability, we wanted to assess the impact of adolescent cocaine experience on each phenotype trajectory, measure whether cocaine’s impact during adolescence caused different neurobiological and epigenetic changes in bHRs vs. bLRs, and determine if these changes persist into adulthood and associate with adult psychomotor sensitization, an indicator of responsiveness to psychostimulants.
In particular, bHR rats sensitized both in adolescence and adulthood to this particular repeated cocaine paradigm, and this is consistent with our prior studies performed in adult rats (e.g., 43). This is the first study showing that adolescent bHR rats also sensitize following repeated cocaine, and as shown in the results, the magnitude of increase on D7, both for locomotor distance and head-waving frequency, is similar to the one observed in adult bHR rats exposed to cocaine (and with or without a prior history of cocaine). This suggest that the limits on drug-induced changes might be preventing bHR rats with a double hit of cocaine (adolescence and adulthood) to show even higher levels of activity, a term that fits the definition of “metaplasticity” in the context of our results, and that can justify why bHR rats with a prior history of adolescent cocaine no longer exhibit sensitization in adulthood. In contrast, although bLRs did not display psychomotor sensitization when given cocaine in either adolescence or adulthood alone (43), a prior history of cocaine exposure during adolescence appeared to have “primed” bLRs such that they express increased psychomotor activation later in adulthood following an acute challenge. Thus, the drug experience during adolescence appeared sufficient to override an inherent resistance to expressing psychomotor sensitization in those less addiction liable (i.e., bLRs). However, this acute effect appeared transient, with repeated exposure to cocaine either showing no increase or even a decrease in psychomotor activation on D7 relative to D1.
The fact that identical cocaine treatment regimens in either adolescence or adulthood produced such different behavioral profiles underscore the importance of considering genetic background and associated neurobiological differences in shaping drug responsiveness. The idea that bHRs and bLRs have unique drug responses is consistent with our previous findings showing that an acute cocaine challenge in unmanipulated bHRs and bLRs produces different neurochemical responses in NAc dopamine and norepinephrine levels (52). However, the biological mechanisms that shape their contrasting responses to repeated drug exposure across two stages of life are far from understood.
The impact of adolescent and adult exposure to cocaine on the balance of a repressive and a permissive modified histone
In this study, we asked whether the differential impact of adolescent cocaine on the bHR vs. bLR adult phenotype is associated with distinct epigenetic changes in the two lines. We used an anatomical, cell-count-based approach to identify epigenetic consequences of adolescent drug experience on subsequent adult drug sensitization in bHR and bLR rats. We focused on specific sub-regions of the striatum and NAc and characterized their profiles by quantifying the levels of the repressive H3K9me3 vs. the permissive acH3K9 at the cellular level. This approach assesses, with single cell granularity, the total change in the level of these histones that can modify the transcription of multiple target genes in those cells. It represents an alternative to techniques such as CHIP-seq that are typically carried out at the level of an entire brain region and aim at identifying the binding sites of a histone mark to specific genes. Focusing on select sub-regions of the striatum we found specific bHR-bLR differences in epigenetic markers, particularly notable in the NAc core.
The two epigenetic marks we examined have been previously shown to be important in cocaine sensitization. Overexpressing permissive acH3K9 produced greater sensitization, while overexpressing similar restrictive di-methylation H3K9 blunted sensitization in mice (36). However, these chromatin modifications were directly manipulated on the Cdk5 gene to produce these effects, which is known to modulate DARPP-32 dopamine signaling (53) and phosphorylate D2, regulating its downstream signaling (54). In our model, we have found basal differences in bHR-bLR epigenetic profiles (55). In particular, H3K9me3 was found to negatively regulate D2 receptors in the striatum of bHRs following a history of cocaine self-administration (50).
In this study, we found that decreases in H3K9me3 track with the behavioral manifestation of sensitization. In the NAc core of bHRs, H3K9me3 exhibits a pattern consistent with limits on plasticity described at the behavioral level. Thus, its levels are decreased by chronic cocaine in both adolescence and adulthood, and since it is usually repressive, this change is consistent with increased behavioral activation. Similarly in outbred rats, the number of cells levels of repressive H3K9me3 was reduced in the NAc following cocaine sensitization (31). Moreover, our findings show that the decrease in this histone is stable in that it is seen in saline-treated adults with a history of adolescent cocaine exposure. However, the additional cocaine exposure in adulthood does not further impact to the change in NAc core. Thus, one explanation for why bHRs did not show further augmentation of locomotor sensitization is that the plasticity of their neuroplasticity, or “metaplasticity,” may become diminished by the adolescent cocaine experience, weakening the brain’s response to subsequent drug experience. Alternatively, plasticity might remain intact, but the response takes on a different form to reduce subsequent sensitization to the adult drug exposure (i.e., reduced H3K9me3 in NAc core). Moreover, in the NAc shell of bHRs, there were no cocaine-induced changes in H3K9me3 observed in either adolescents or adults with no prior drug history. Only the double hit exposure (adolescence + adulthood) triggered a change which was in the opposite direction (i.e., toward increased repression), which may contribute to the lack of behavioral sensitization in the Coc-Cocaine group. In bLR rats, there were no changes observed in the levels of this modified histone in the NAc core or shell, in line with their non-sensitized response at the end of the adulthood treatment.
As for the regulation of the permissive mark in bHRs rats, the changes observed in acH3K9 were region-specific (only in NAc core) and showed a consistent pattern with sensitization in both adolescent and adults with no cocaine history. These findings are in agreement with the literature showing that repeated psychostimulant administration increases histone acetylation in the NAc of mice (35).
The effects on acH3K9 were also persistent, as seen in the Coc-Saline group. However, a double hit cocaine exposure actively reversed this elevation, and decreased the level of this modified histone, which would also be inhibitory (i.e., no further psychomotor sensitization beyond the levels elicited by the first regimen of adolescent cocaine). The change in profile of H3K9me3 and acH3K9 during sensitization and metaplasticity is illustrated in Figure 5. Overall, if a bHR animal has a dual exposure to cocaine and is showing a limit to further sensitization, several epigenetics changes are consistent with limiting activation or enhancing repression: a) No further inhibition of H3K9me3 in the NAc core; b) Activation of H3K9me3 in the shell; and c) Inhibition of acH3K9 in the core. All of the changes are consistent with the notion of metaplasticity.
As for bLR rats, acH3K9 was increased in the NAc core, but only after a double hit of cocaine, suggesting it might not be sufficient to cause sensitization in these rats. Interestingly, these changes were restricted to the NAc area, since acH3K9 was not altered in dmSTR or dlSTR of bHR or bLR rats following cocaine treatments. Altogether, the current findings proved that these changes were anatomically (e.g., NAc core vs. shell or STR regions) and histone (methylation vs. acetylation) specific, and different levels were observed in bHRs and bLRs following the same history of adolescent cocaine experience. This suggests these histone modifications are situated to have an active and circuitry-specific regulatory role in the expression of cocaine sensitization, since decreased repressive histone, H3K9me3, and increased permissive histone, acH3K9, associated with cocaine sensitization in the NAc core of bHR rats.
General considerations
These findings reflect that adult epigenetic function is both sub-region specific and phenotype specific. The lens of epigenetics offers novel perspectives on how genetics and environment share common mechanisms for ensuring adaptive neurodevelopment, but also the potential for exacerbating maladaptive risk. While we have focused in this study on changes in two modified histones, it is important to note the existence of hundreds of identified posttranslational chromatin modifications which likely interact with several other classes of epigenetic modifications (e.g., DNA methylation, lncRNAs). These modifications likely work in concert as a “histone code” to enact substantive downstream events via transcription factors to drive gene regulation (56, 57). Thus, vulnerability to psychostimulant use and abuse is likely shaped by broad patterns of gene expression modified by epigenetic and enzymatic modifications in critical neuronal networks that shape responsiveness to the drugs (58). The advent of technologies such as the use of Transposase Accessible Chromatin with high-throughput sequencing (ATAC-Seq) (59) coupled with RNAseq promises to be powerful in the context of drug abuse research for fully characterizing chromatin accessibility, broadly defining epigenetic profiles in a given tissue and cell types to identify which genes are available for tissue-specific transcription. The use of these coupled techniques to understand the interplay between genetic background and adolescent exposure to drugs in modifying vulnerability to drug use or abuse should prove especially powerful.
Our study clearly underscores the importance of adolescence as a critical developmental window for substantial life-long changes that can shape the circuitry for emotion, motivation and addiction (e.g., 44–47, reviewed by 19–21). There is growing evidence that epigenetic time windows may accompany critical periods of development, playing a role in the long-term trajectory of the brain (26). This may be particularly relevant for addiction liability and outcome given the prevalence of prescribed psychostimulant medication in younger ages and increased accessibility potential for adolescent drug abuse. Epigenetic mechanisms that normally play a functional role in neurodevelopment at that time, including cortical organization, pruning, and coordination of gene expression, may become substantially altered by adolescent drug abuse, causing life-long consequences on subsequent addiction liability.
In conclusion, we have shown the clear interplay between genetic background and exposure to cocaine during adolescence in altering behavioral phenotypes in adulthood in response to a psychostimulant drug. In particular, we demonstrated that early experience with psychostimulant drugs can limit plasticity in adulthood. Finally, we have demonstrated how epigenetic mechanisms within the NAc, that can affect the broad level of gene activation or repression, are associated with cocaine sensitization and metaplasticity.
Data availability statement
The original contributions presented in this study are included in the article/Supplementary material, further inquiries can be directed to the corresponding author.
Ethics statement
All animal procedures complied with the ARRIVE guidelines and were performed in accordance with the University of Michigan Animal Care Committee’s Regulations and followed the Guide for the Care and Use of Laboratory Animals: Eight Edition (2011).
Author contributions
AP, MJG-F, SF, and HA designed this research and wrote the manuscript. AP and MJG-F performed the research and analyzed the data. MJG-F plotted the figures displayed. EH-B and SW contributed unpublished reagents/analytic tools critical to these experiments. All authors contributed to the article and approved the submitted version.
Funding
This work was funded in part by: National Institute of Drug Abuse 5P01DA021633, Biology of Drug Abuse Postdoctoral Training Program (University of Michigan Medical School, Grant: T32 DA007268) and Office of Naval Research N00014-09-1-0598 and N00014-12-1-0366 to HA. This work was also partly funded by: “Delegación del Gobierno para el Plan Nacional sobre Drogas” (Grants: 2012/011, 2016/002, and 2020/001, Ministerio de Sanidad, Servicios Sociales e Iqualdad, Spain) to MJG-F.
Acknowledgments
We would like to thank Anna Gencay, Jesse Klein, and Natalie Tarrant for their assistance quantifying stereological results, Alex Stefanov for oversight of the selective-breeding colony, and Amy Tan, Fei Li, and Jennifer Fitzpatrick for their technical assistance.
Conflict of interest
The authors declare that the research was conducted in the absence of any commercial or financial relationships that could be construed as a potential conflict of interest.
Publisher’s note
All claims expressed in this article are solely those of the authors and do not necessarily represent those of their affiliated organizations, or those of the publisher, the editors and the reviewers. Any product that may be evaluated in this article, or claim that may be made by its manufacturer, is not guaranteed or endorsed by the publisher.
Supplementary material
The Supplementary Material for this article can be found online at: https://www.frontiersin.org/articles/10.3389/fpsyt.2022.1024617/full#supplementary-material
Footnotes
References
1. Volkow ND, Koob GF, McLellan AT. Neurobiologic advances from the brain disease model of addiction. N Engl J Med. (2016) 374:363–71. doi: 10.1056/NEJMra1511480
2. National Survey of Drug Use and Health [NSDUH]. 2020 National Survey of Drug Use and Health (NSDUH) Releases. (2020). Available online at: https://www.samhsa.gov/data/release/2020-national-survey-drug-use-and-health-nsduh-releases (accessed July 1, 2022).
3. Lipari RN, Williams MR, Copello EAP, Pemberton MR. Risk and Protective Factors and Estimates of Substance Use Initiation: Results from the 2015 National Survey on Drug Use and Health. Rockville, MD: Substance Abuse and Mental Health Services Administration (US) (2012).
4. Ducci F, Goldman D. The genetic basis of addictive disorders. Psychiatr Clin North Am. (2012) 35:495–519. doi: 10.1016/j.psc.2012.03.010
5. Goldman D, Oroszi G, Ducci F. The genetics of addictions: uncovering the genes. Nat Rev Genet. (2005) 6:521–32. doi: 10.1038/nrg1635
6. Kreek MJ, Nielsen DA, Butelman ER, LaForge KS. Genetic influences on impulsivity, risk taking, stress responsivity and vulnerability to drug abuse and addiction. Nat Neurosci. (2005) 8:1450–7. doi: 10.1038/nn1583
7. Hawkins EHA. Tale of two systems: co-occurring mental health and substance abuse disorders treatment for adolescents. Ann Rev Psychol. (2009) 60:197–227. doi: 10.1146/annurev.psych.60.110707.163456
8. Hicks BM, Krueger RF, Iacono WG, McGue M, Patrick CJ. Family transmission and heritability of externalizing disorders. Arch Gen Psychiatry. (2004) 61:922. doi: 10.1001/archpsyc.61.9.922
9. Hettema JM, Prescott CA, Myers JM, Neale MC, Kendler KS. The structure of genetic and environmental risk factors for anxiety disorders in men and women. Arch Gen Psychiatry. (2005) 62:182. doi: 10.1001/archpsyc.62.2.182
10. Crews F, He J, Hodge C. Adolescent cortical development: a critical period of vulnerability for addiction. Pharmacol Biochem Behav. (2007) 86:189–99. doi: 10.1016/j.pbb.2006.12.001
11. Sihvola E, Rose RJ, Dick DM, Pulkkinen L, Marttunen M, Kaprio J. Early-onset depressive disorders predict the use of addictive substances in adolescence: a prospective study of adolescent finnish twins. Addiction. (2008) 103:2045–53. doi: 10.1111/j.1360-0443.2008.02363.x
12. Chen C-Y, Storr CL, Anthony JC. Early-onset drug use and risk for drug dependence problems. Addict Behav. (2009) 34:319–22. doi: 10.1016/j.addbeh.2008.10.021
13. Warner LA. Prevalence and correlates of drug use and dependence in the united states. Arch Gen Psychiatry. (1995) 52:219. doi: 10.1001/archpsyc.1995.03950150051010
14. Degenhardt L, Coffey C, Carlin JB, Moran P, Patton GC. Who are the new amphetamine users? A 10-year prospective study of young Australians. Addiction. (2007) 102:1269–79. doi: 10.1111/j.1360-0443.2007.01906.x
15. Burstein M. Use and abuse of alcohol and illicit drugs in US adolescents. Arch Gen Psychiatry. (2012) 69:390. doi: 10.1001/archgenpsychiatry.2011.1503
16. McCabe SE, West BT, Morales M, Cranford JA, Boyd CJ. Does early onset of non-medical use of prescription drugs predict subsequent prescription drug abuse and dependence? Results from a national study. Addiction. (2007) 102:1920–30. doi: 10.1111/j.1360-0443.2007.02015.x
17. Palmer RHC, Young SE, Hopfer CJ, Corley RP, Stallings MC, Crowley TJ, et al. Developmental epidemiology of drug use and abuse in adolescence and young adulthood: evidence of generalized risk. Drug Alcohol Depend. (2009) 102:78–87. doi: 10.1016/j.drugalcdep.2009.01.012
18. Dickinson JK, Forsyth PA. Preconditioned conjugate gradient methods for three-dimensional linear elasticity. Int J Numer Methods Eng. (1994) 37:2211–34. doi: 10.1002/nme.1620371305
19. Spear LP. Consequences of adolescent use of alcohol and other drugs: studies using rodent models. Neurosci Biobehav Rev. (2016) 70:228–43. doi: 10.1016/j.neubiorev.2016.07.026
20. Salmanzadeh H, Ahmadi-Soleimani SM, Pachenari N, Azadi M, Halliwell RF, Rubino T, et al. Adolescent drug exposure: a review of evidence for the development of persistent changes in brain function. Brain Res Bull. (2020) 156:105.117. doi: 10.1016/j.brainresbull.2020.01.007
21. Kantak KM. Adolescent-onset vs. adult-onset cocaine use: impact on cognitive functioning in animal models and opportunities for translation. Pharmacol Biochem Behav. (2020) 196:172994. doi: 10.1016/j.pbb.2020.172994
22. Rao U, Chen LA. Neurobiological and psychosocial processes associated with depressive and substance-related disorders in adolescents. Curr Drug Abuse Rev. (2008) 1:68–80. doi: 10.2174/1874473710801010068
23. Nestler EJ. Epigenetic mechanisms of drug addiction. Neuropharmacology. (2014) 76:259–68. doi: 10.1016/j.neuropharm.2013.04.004
24. Pierce RC, Fant B, Swinford-Jackson SE, Heller EA, Berrettini WH, Wimmer ME. Environmental, genetic and epigenetic contributions to cocaine addiction. Neuropsychopharmacology. (2018) 43:1471–80. doi: 10.1038/s41386-018-0008-x
25. McGowan PO, Szyf M. Environmental epigenomics: understanding the effects of parental care on the epigenome. Essays Biochem. (2010) 48:275–87. doi: 10.1042/bse0480275
26. Kanherkar RR, Bhatia-Dey N, Csoka AB. Epigenetics across the human lifespan. Front Cell Dev Biol. (2014) 2:1–19. doi: 10.3389/fcell.2014.00049
27. Kundakovic M, Champagne FA. Early-life experience, epigenetics, and the developing brain. Neuropsychopharmacology. (2015) 40:141–53. doi: 10.1038/npp.2014.140
28. McEwen BS. Redefining neuroendocrinology: epigenetics of brain-body communication over the life course. Front Neuroendocrinol. (2018) 49:8–30. doi: 10.1016/j.yfrne.2017.11.001
29. LaPlant Q, Nestler EJ. CRAC king the histone code: cocaine’s effects on chromatin structure and function. Horm Behav. (2011) 59:321–30. doi: 10.1016/j.yhbeh.2010.05.015
30. Feng J, Nestler EJ. Epigenetic mechanisms of drug addiction. Curr Opin Neurobiol. (2013) 23:521–8. doi: 10.1016/j.conb.2013.01.001
31. Maze I, Feng J, Wilkinson MB, Sun HS, Shen L, Nestler EJ. Cocaine dynamically regulates heterochromatin and repetitive element unsilencing in nucleus accumbens. Proc Natl Acad Sci USA. (2011) 108:3035–40. doi: 10.1073/pnas.1015483108
32. Vassoler FM, Byrnes EM, Pierce RC. The impact of exposure to addictive drugs on future generations: physiological and behavioral effects. Neuropharmacology. (2014) 76:269–75. doi: 10.1016/j.neuropharm.2013.06.016
33. Vassoler FM, Sadri-Vakili G. Mechanisms of transgenerational inheritance of addictive-like behaviors. Neuroscience. (2014) 264:198–206. doi: 10.1016/j.neuroscience.2013.07.064
34. Le Q, Yan B, Yu X, Li Y, Song H, Zhu H, et al. Drug-seeking motivation level in male rats determines offspring susceptibility or resistance to cocaine-seeking behaviour. Nat Commun. (2017) 8:15527. doi: 10.1038/ncomms15527
35. Kennedy PJ, Feng J, Robison AJ, Maze I, Badimon A, Mouzon E, et al. Class I HDAC inhibition blocks cocaine-induced plasticity by targeted changes in histone methylation. Nat Neurosci. (2013) 16:434–40. doi: 10.1038/nn.3354
36. Heller EA, Hamilton PJ, Burek DD, Lombroso SI, Peña CJ, Neve RL, et al. Targeted epigenetic remodeling of the cdk5 gene in nucleus accumbens regulates cocaine- and stress-evoked behavior. J Neurosci. (2016) 36:4690–7. doi: 10.1523/JNEUROSCI.0013-16.2016
37. Davis BA, Clinton SM, Akil H, Becker JB. The effects of novelty-seeking phenotypes and sex differences on acquisition of cocaine self-administration in selectively bred high-responder and low-responder rats. Pharmacol Biochem Behav. (2008) 90:331–8. doi: 10.1016/j.pbb.2008.03.008
38. Flagel SB, Robinson TE, Clark JJ, Clinton SM, Watson SJ, Seeman P, et al. An animal model of genetic vulnerability to behavioral disinhibition and responsiveness to reward-related cues: implications for addiction. Neuropsychopharmacology. (2010) 35:388–400. doi: 10.1038/npp.2009.142
39. Clinton SM, Kerman IA, Orr HR, Bedrosian TA, Abraham AD, Simpson DN, et al. Pattern of forebrain activation in high novelty-seeking rats following aggressive encounter. Brain Res. (2011) 1422:20–31. doi: 10.1016/j.brainres.2011.08.033
40. Flagel SB, Waselus M, Clinton SM, Watson SJ, Akil H. Antecedents and consequences of drug abuse in rats selectively bred for high and low response to novelty. Neuropharmacology. (2014) 76:425–36. doi: 10.1016/j.neuropharm.2013.04.033
41. Committee for the Update of the Guide for the Care and Use of Laboratory Animals.Guide for the Care and Use of Laboratory Animals. Washington, D.C: The National Academies Press (2011).
42. Gosnell BA, Blake AG, Gosnell BA. Sucrose intake enhances behavioral sensitization produced by cocaine. Brain Res. (2005) 1031:194–201. doi: 10.1016/j.brainres.2004.10.037
43. García-Fuster MJ, Perez JA, Clinton SM, Watson SJ, Akil H. Impact of cocaine on adult hippocampal neurogenesis in an animal model of differential propensity to drug abuse. Eur J Neurosci. (2010) 31:79–89. doi: 10.1111/j.1460-9568.2009.07045.x
44. García-Cabrerizo R, Keller B, Garcia-Fuster MJ. Hippocampal cell fate regulation by chronic cocaine during periods of adolescent vulnerability: consequences of cocaine exposure during adolescence on behavioral despair in adulthood. Neuroscience. (2015) 304:302–15. doi: 10.1016/j.neuroscience.2015.07.040
45. García-Fuster MJ, Parsegian A, Watson SJ, Akil H, Flagel SB. Adolescent cocaine exposure enhances goal-tracking behavior and impairs hippocampal cell genesis selectively in adult bred low-responder rats. Psychopharmacology. (2017) 234:1293–305. doi: 10.1007/s00213-017-4566-0
46. García-Cabrerizo R, García-Fuster MJ. Adolescent cocaine exposure enhanced negative affect following drug re-exposure in adult rats: attenuation of c-fos activation. J Psychopharmacol. (2019) 33:154–62. doi: 10.1177/0269881118812353
47. Bis-Humbert C, García-Fuster MJ. Adolescent cocaine induced persistent negative affect in female rats exposed to early-life stress. Psychopharmacology. (2021) 238:3399–410. doi: 10.1007/s00213-021-05955-z
48. Flagel SB, Robinson TE. Quantifying the psychomotor activating effects of cocaine in the rat. Behav Pharmacol. (2007) 18:297–302. doi: 10.1097/FBP.0b013e3281f522a4
49. Gundersen HJ, Jensen EB, Kieu K, Nielsen J. The efficiency of systematic sampling in stereology–reconsidered. J Microsci. (1999) 193:199–211. doi: 10.1046/j.1365-2818.1999.00457.x
50. Flagel SB, Chaudhury S, Waselus M, Kelly R, Sewani S, Clinton SM, et al. Genetic background and epigenetic modifications in the core of the nucleus accumbens predict addiction-like behavior in a rat model. Proc Natl Acad Sci USA. (2016) 113:E2861–70. doi: 10.1073/pnas.1520491113
51. Abraham WC, Bear MF. Metaplasticity: the plasticity of synaptic plasticity. Trends Neurosci. (1996) 19:126–30. doi: 10.1016/S0166-2236(96)80018-X
52. Mabrouk OS, Han JL, Wong JMT, Akil H, Kennedy RT, Flagel SB. The in vivo neurochemical profile of selectively bred high-responder and low-responder rats reveals baseline, cocaine-evoked, and novelty-evoked differences in monoaminergic systems. ACS Chem Neurosci. (2018) 9:715–24. doi: 10.1021/acschemneuro.7b00294
53. Bibb JA, Snyder GL, Nishi A, Yan Z, Meijer L, Fienberg AA, et al. Phosphorylation of DARPP-32 by Cdk5 modulates dopamine signalling in neurons. Nature. (1999) 402:669–71. doi: 10.1038/45251
54. Jeong J, Park YU, Kim DK, Lee S, Kwak Y, Lee SA, et al. Cdk5 phosphorylates dopamine D2 receptor and attenuates downstream signaling. PLoS One. (2013) 8:e84482. doi: 10.1371/journal.pone.0084482
55. Chaudhury S, Aurbach EL, Sharma V, Blandino P, Turner CA, Watson SJ, et al. FGF2 is a target and a trigger of epigenetic mechanisms associated with differences in emotionality: partnership with H3K9me3. Proc Natl Acad Sci USA. (2014) 111:11834–9. doi: 10.1073/pnas.1411618111
56. Strahl BD, Allis CD. The language of covalent histone modifications. Nature. (2000) 403:41–5. doi: 10.1038/47412
57. Matthews SG, McGowan PO. Developmental programming of the HPA axis and related behaviours: epigenetic mechanisms. J Endocrinol. (2019) 61:154–62.
58. Lud Cadet J. Dysregulation of acetylation enzymes inanimal models of psychostimulant use disorders: evolving stories. Curr Neuropharmacol. (2016) 14:10–6. doi: 10.2174/1570159X13666150121230133
Keywords: adolescence, cocaine, epigenetics, addiction, psychomotor sensitization, nucleus accumbens
Citation: Parsegian A, García-Fuster MJ, Hebda-Bauer E, Watson SJ, Flagel SB and Akil H (2022) Adolescent cocaine differentially impacts psychomotor sensitization and epigenetic profiles in adult male rats with divergent affective phenotypes. Front. Psychiatry 13:1024617. doi: 10.3389/fpsyt.2022.1024617
Received: 22 August 2022; Accepted: 26 September 2022;
Published: 12 October 2022.
Edited by:
Amy Lasek, University of Illinois at Chicago, United StatesReviewed by:
Giordano de Guglielmo, University of California, San Diego, United StatesAnna Brancato, University of Palermo, Italy
Copyright © 2022 Parsegian, García-Fuster, Hebda-Bauer, Watson, Flagel and Akil. This is an open-access article distributed under the terms of the Creative Commons Attribution License (CC BY). The use, distribution or reproduction in other forums is permitted, provided the original author(s) and the copyright owner(s) are credited and that the original publication in this journal is cited, in accordance with accepted academic practice. No use, distribution or reproduction is permitted which does not comply with these terms.
*Correspondence: M. Julia García-Fuster, j.garcia@uib.es