- 1Department of Magnetic Resonance Imaging, The First Affiliated Hospital of Zhengzhou University, Zhengzhou, China
- 2Key Laboratory for Functional Magnetic Resonance Imaging and Molecular Imaging of Henan Province, Zhengzhou, China
- 3Engineering Technology Research Center for Detection and Application of Brain Function of Henan Province, Zhengzhou, China
Background: Patients with Internet gaming disorder (IGD) and attention-deficit/hyperactivity disorder (ADHD) have high comorbidity but it is still unknown whether these disorders have shared and distinctive neuroimage alterations.
Objective: The aim of this meta-analysis was to identify shared and disorder-specific structural, functional, and multimodal abnormalities between IGD and ADHD.
Methods: A systematic literature search was conducted for whole-brain voxel-based morphometry (VBM) and functional magnetic resonance imaging (fMRI) studies comparing people with IGD or ADHD with healthy controls. Regional gray matter volume (GMV) and fMRI differences were compared over the patient groups and then a quantitative comparison was performed to find abnormalities (relative to controls) between IGD and ADHD using seed-based d mapping meta-analytic methods.
Result: The meta-analysis contained 14 IGD VBM studies (contrasts covering 333 IGDs and 335 HCs), 26 ADHD VBM studies (1,051 patients with ADHD and 887 controls), 30 IGD fMRI studies (603 patients with IGD and 564 controls), and 29 ADHD fMRI studies (878 patients with ADHD and 803 controls). Structurally, VBM analysis showed disorder-specific GMV abnormality in the putamen among IGD subjects and orbitofrontal cortex in ADHD and shared GMV in the prefrontal cortex. Functionally, fMRI analysis discovered that IGD-differentiating increased activation in the precuneus and shared abnormal activation in anterior cingulate cortex, insular, and striatum.
Conclusion: IGD and ADHD have shared and special structural and functional alterations. IGD has disorder-differentiating structural alterations in the putamen and ADHD has alterations in the orbitofrontal cortex. Disorder-differentiating fMRI activations were predominantly observed in the precuneus among IGD subjects and shared impairing function connection was in the rewards circuit (including ACC, OFC, and striatum).
Introduction
Internet gaming disorder (IGD) is characterized by difficulties in controlling online gaming behaviors, including symptoms such as craving (1, 2), loss of control, and excessive impulsivity (3, 4). Previous studies have indicated that the prevalence estimates of IGD range from 0.3 to 10.8%, depending on the country and age of the population (5–8). Attention-deficiency/hyperactivity disorder (ADHD) has a prevalence of 5–7% (9) and is typically characterized by symptoms of inattention, hyperactivity, and impulsivity (10).
Several comprehensive reviews reported a strong correlation between IGD and ADHD (11). These two disorders share some key features such as impulsivity, seeking immediate rewards, motivation deficit, and hostility (12, 13). A single prospective study followed over 2,000 adolescents for 2 years and found that ADHD was the most significant predictor for the development of internet addiction (14). Moreover, both IGD and ADHD have deficits in the reward circuit, which includes the prefrontal cortex (PFC), anterior cingulate cortex (ACC), orbitofrontal cortex (OFC), striatum (containing the caudate nucleus, putamen, globus pallidus), amygdaloid nucleus, and thalamus (15–17).
Current evidence shows that most addictive diseases exert initial reinforcing effects by activating reward circuits in the brain (18). Weinstein (19) has shown that individuals who are addicted to video-game playing obtain much pleasure during play because of extensive dopamine release. In addition, functional magnetic resonance imaging (fMRI) studies of the reward circuit showed hyperactivity in the bilateral dorsolateral prefrontal cortex (DLPFC), caudate nucleus, the supplementary motor cortex (SMA), and ACC among IGD people (2, 20). Moreover, people with IGD have abnormal structural alterations that include reduced gray matter volume (GMV) in the bilateral ACC, OFC, SMA, right putamen, and left dorsolateral prefrontal cortex through different studies (17, 21, 22).
In addition, Blum et al. (23) showed that ADHD is a reward deficiency disorder, and some theories considered that reward deficiency might predispose individuals to addictive, impulsive, and compulsive behavior. An ADHD, fMRI meta-analyses displayed hypoactivation in the right and left ventrolateral prefrontal cortex (VLPFC), anterior insular (AI), caudate nucleus, middle frontal gyrus (MFG) (24), SMA, and ACC. Moreover, whole-brain voxel-based morphometry (VBM) studies found common decreased GMV in the right globus pallidus and putamen, caudate nucleus, ventromedial prefrontal cortex (VMPFC), and ACC (25–27).
The above studies showed brain structural abnormalities were observed in the cingulate, striatum, frontal, and temporal lobes between these two disorders (15, 17). Moreover, both IGD and ADHD have abnormal whole-brain functional connectivity, such as deficits in the reward circuit (17, 28), although they may show much heterogeneous performance. However, only one study on VBM and no task fMRI compared these two disorders directly. The VBM study (29) showed that IGD subjects with a history of childhood ADHD symptoms had greater GMV in the angular gyrus, middle occipital gyrus, and lingual gyrus than IGD subjects who did not have childhood ADHD symptoms. However, the relatively small sample size of this study is statistically limited. This study aimed to establish the most consistent disorder-differentiating, shared structural, and functional deficits, which are important for developing disorder-specific or transdiagnostic treatment. A comprehensive meta-analysis was conducted, comparing structural and functional abnormalities between IGD and ADHD. Furthermore, multimodal structural and functional abnormalities were performed through conducting conjunction/disjunction analyses across VBM and fMRI studies.
According to previous studies, we hypothesized that disorder-specific GMV abnormality would be shown in the OFC among ADHD subjects (27) and in the putamen in IGD people, whereas we expected disorder-shared decreased GMV in the prefrontal cortex and striatum for both (29). As for fMRI, we hypothesized that IGD-differentiating increased activation in the prefrontal regions (e.g., OFC) (17) where ADHD patients show hypoactivation, and shared abnormal overactivation in the cingulate cortex in both disorders.
Methods
Publication Search and Study Inclusion
Systematic and comprehensive searches were performed in the PubMed, Web of Knowledge, and Science Direct databases from January 1, 2010, to October 31, 2020, using different combinations of the keywords “voxel-based morphometry” or “VBM” or “morphometry” or “gray matter” or “functional magnetic resonance imaging” or “fMRI” and “online-game” or “Internet gaming disorder” or “IGD” or “Attention-Deficit/Hyperactivity Disorder” or “ADHD.” We identified further papers by reference tracking and consulting retrieved high-quality meta-analysis and review articles.
The included studies had to meet the following criteria: (1) they provided whole-brain pairwise voxel-based comparisons of patient groups (IGD or ADHD) relative to controls; (2) they were a task-related fMRI or VBM study; (3) they provided peak coordinates in Montreal Neurological Institute (MNI) or Talairach spaces; (4) the diagnosis of ADHD patients had to be based on DSM-IV-TR, or DSM-5, or ICD-10 criteria, and IGD was diagnosed according to DSM-5 or YIAS or CIAS; and (5) there were no neurological or psychiatric comorbidities (such as depression, anxiety, autism, learning disorder, and epilepsy).
We excluded studies that had fewer than 10 patients, those that used only ROI analyses, duplicated patient data, or no eligible contrasts (25, 30–32). If studies did not report peak coordinates, corresponding authors were contacted for necessary details; otherwise, these studies were excluded from the meta-analysis.
The two authors (Gao and Zhang) assessed all articles and achieved 100% agreement.
Statistical Analysis
We used an anisotropic effect-size version of the Seed-based d Mapping software package (AES-SDM) (version 5.15) to conduct the voxel-wise meta-analysis (https://www.sdmproject.com/), following MOOSE guidelines for meta-analyses of observational studies. The AES-SDM data processing procedure is briefly summarized here (http://www.sdmproject.com/software/tutorial.pdf). AES-SDM uses an anisotropic non-normalized Gaussian kernel to recreate an effect-size map and an effect-size variance map for the contrast between patients and controls from peak coordinates and effect sizes for each VBM or fMRI study. Coordinates were converted to Montreal Neurological Institute (MNI) space for this analysis. Following this, a mean map is created by performing a voxel-wise calculation of the random-effects mean of the study maps, weighted by sample size and variance of each study and between-study heterogeneity. In addition, full width at half maximum (FWHM) was set to 20 mm because this setting was optimal to balance sensitivity and specificity and other parameters included voxel P = 0.005, peak height Z = 1, and cluster extent = 10 voxels (33).
First, separate analyses were conducted to examine regional GMV within each patient group (IGD and ADHD) relative to controls and then between the two disorders. Second, fMRI meta-analyses were conducted to examine the neural activation abnormalities observed within and between disorders using all available data. Then a conjunction analysis across both patient groups relative to controls was further performed to examine areas of shared/contrasting abnormalities; This conjunction method was also used within patient groups to conduct multimodal analyses, which showed regions of overlapping functional and structural abnormalities compared with controls. Some studies used multiple task contrasts, several functional tasks, or identical controls. Combined maps with reduced variance were calculated to avoid dependent data in the analyses (26). To examine the effects of age and gender, meta-regression analyses were performed. Finally, we also conducted additional reliability analyses to assess the robustness of the findings: a jackknife sensitivity analysis, which repeated the same analysis excluding one study each time, to assess the reproducibility of the results for each meta-analysis. Moreover, an Egger's test was used to examine possible publication bias.
A statistical threshold p < 0.005 was used for all meta-analyses (32, 34), and a reduced threshold p < 0.0005 and a cluster extent 20 voxels was used in the meta-regression to control for false positives (35).
Result
Search Results and Sample Characteristics
A pool of 2,174 retrieved publications was searched and 41 additional records were identified through other sources. After duplicates were removed, 1,103 records were screened and 289 full-text articles were assessed for eligibility. The final dataset comprised 14 IGD VBM studies (contrasts covering 333 IGDs and 335 HCs), 26 ADHD VBM studies (1,051 patients with ADHD and 887 controls), 30 IGD fMRI studies (603 patients with IGD and 564 controls), and 29 ADHD fMRI studies (878 patients with ADHD and 803 controls). See Figure 1 and Tables 1, 2 for more details.
In the VBM analysis, Wilcoxon W tests revealed that patient groups did not differ in age (z = −1.155; P = 0.248), and Chi-squared test showed both groups contained a significantly greater proportion of males (χ2 = 26.362; P = 0.001). In the fMRI meta-analysis, patient groups did not differ in age (z = −1.077; P = 0.282) but a large proportion of patients with IGD and ADHD were males (χ2 = 93.565; P = 0.001). Age and sex were consequently included as covariates in all between-group meta-analyses performed including only the adult studies, which were age and sex matched.
Disorder-Differentiating and Shared Brain Structure Abnormalities
Regional Differences in GMV
IGD VBM
Relative to healthy controls, IGD had reduced gray-matter volume (GMV) in the bilateral anterior cingulate cortex (ACC), median cingulate cortex (MCC), superior frontal gyrus (SFG), the supplementary motor cortex (SMA), right putamen/striatum, bilateral inferior frontal gyrus (IFG), and left middle frontal gyrus (MFG) (Table 3 and Figure 2).
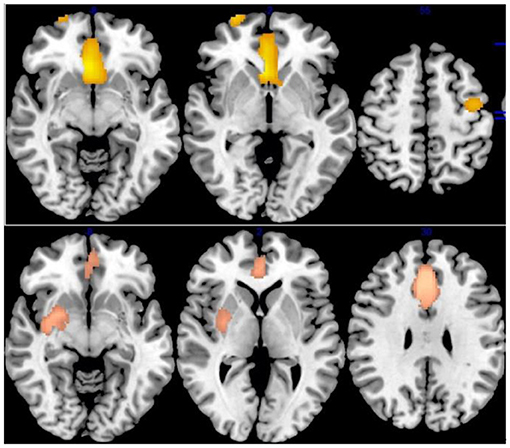
Figure 2. Results of Voxel-Based Morphometry (VBM) for IGD and ADHD. Results of VBM meta-analysis for, from top to bottom, patients with Internet gaming disorder (IGD) relative to controls, patients with attention-deficit/hyperactivity disorder (ADHD) relative to controls.
ADHD VBM
ADHD Patients compared with HCs showed significantly lower GMV in the bilateral ACC/olfactory cortex/median cingulate, bilateral striatum, left caudate nucleus, left precentral gyrus/postcentral gyrus, right superior frontal gyrus, and orbitofrontal cortex (OFC) (Table 3 and Figure 2).
IGD vs. ADHD VBM
People with IGD, relative to ADHD, had more reduced GMV in the right striatum (Montreal Neurological Institute [MNI]coordinates, 28, −4, −10; 44 voxels); while people with ADHD showed lower left caudate nucleus GMV (MNI coordinates, −12, 8, 6; 89 voxels), relative to IGD (Table 3).
Disorder-Differentiating and Shared Brain Functional Connectivity
IGD fMRI
Across all fMRI studies, people with IGD showed activation in the bilateral precuneus/cingulate cortex (CC), right OFC, left angular gyrus/middle temporal gyrus (MTG)/MOG, left precentral gyrus, bilateral IFG, right caudate nucleus. Moreover, IGDs had lower activation in the right precentral and postcentral gyri, right insular/rolandic operculum, compared with controls (Table 3 and Figure 3).
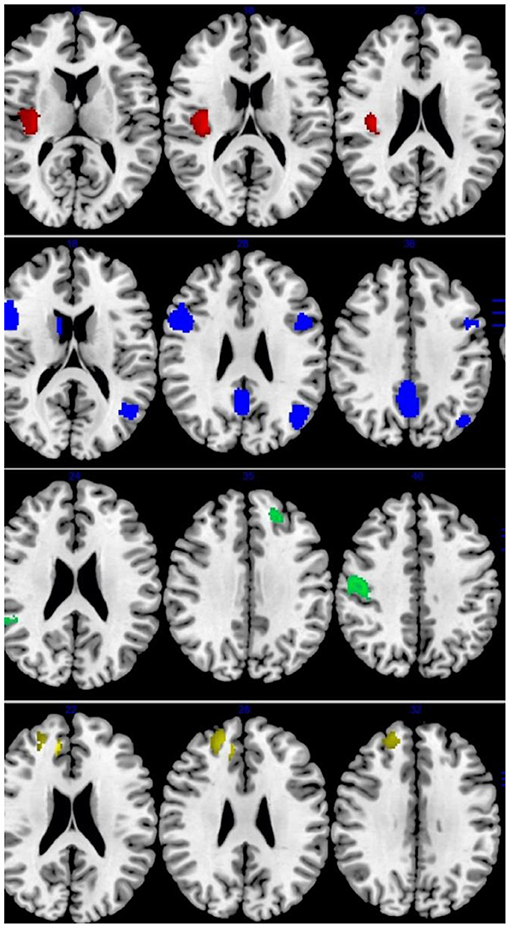
Figure 3. Results of Functional Magnetic Resonance Imaging (fMRI) for IGD and ADHD. Results of fMRI meta-analysis for, from top to bottom, patients with Internet gaming disorder (IGD) relative to controls (red: increased in IGD; blue: decreased in IGD), patients with attention-deficit/hyperactivity disorder (ADHD) relative to controls (green: increased in ADHD; yellow: decreased in ADHD).
ADHD fMRI
Patients with ADHD relative to controls showed overactivation in the right DLPFC, right MFG, and right ACC. Hypoactivation was observed in the right precentral gyrus (motor cortex), left STG/insula/OFC, right STG/MTG, left DLPFC/MFG (Table 3 and Figure 3).
IGD vs. ADHD fMRI
ADHD was associated with disorder-specific hypoactivation relative to IGD in the L MCC, R MTG, R caudate nucleus, and L MFG (Table 3).
Multimodal VBM and fMRI Analyses
Multimodal Analysis in IGD
In patients with IGD, decreased GMV and functional connection relative to controls overlapped in the right insular/putamen (MNI coordinates, 36, −8, 4; 240 voxels) while increased GMV overlapped with increased activation in the right angular gyrus/MOG and precuneus (MNI coordinates, 38, −70, 36 and 8, −56, 34; 456 voxels and 258 voxels, respectively). The left ACC and right IFG was decreased in volume and increased in function connection in patients with IGD relative to controls (MNI coordinates, 4, 16, 22 and 50, 24, 24; 832 and 445 voxels) (Figure 4).
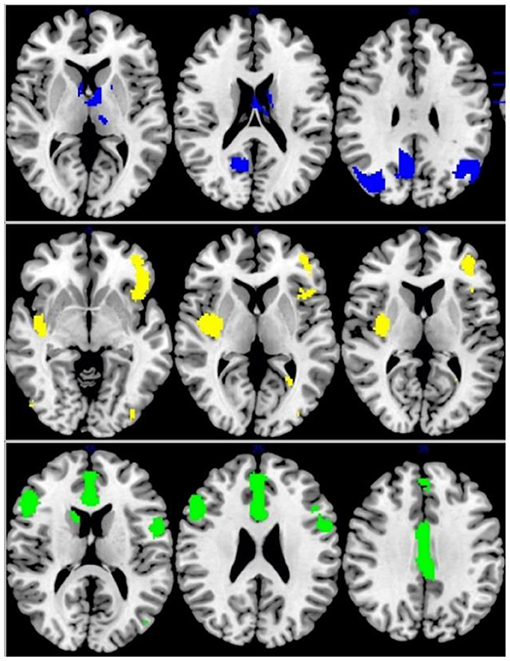
Figure 4. Multimodel analysis in the IGD. Blue: increased GMV and increased activation; Yellow: decreased GMV and functional connection; Green: decreased GMV and increased activation.
Multimodal Analysis in ADHD
As for ADHD patients, increased GMV and functional activation relative to controls overlapped in the right fusiform gyrus (MNI coordinates, 34, −8, −28; 39 voxels) while decreased GMV overlapped with decreased activation in the right superior temporal gyrus, left inferior frontal gyrus, and left postcentral gyrus (MNI coordinates: 58, −42, 16; −30, 16, −24 and −50, −14, 46; 810 voxels; 776 voxel and 222 voxels, respectively) (Figure 5).
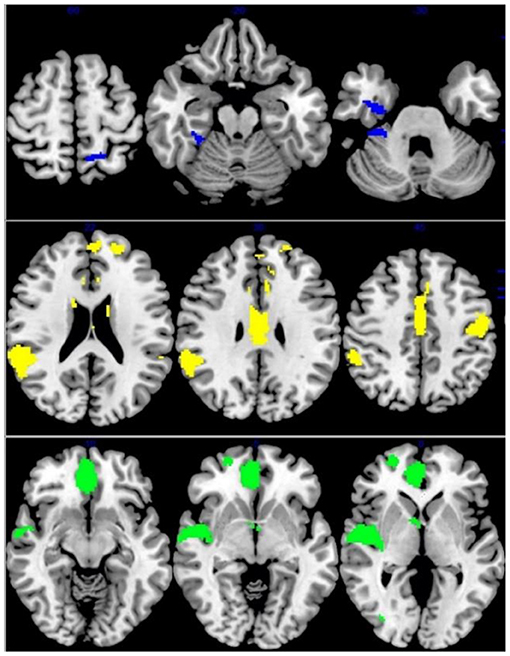
Figure 5. Multimodel analysis in the ADHD. Blue: increased GMV and increased activation; Yellow: decreased GMV and functional connection; Green: decreased GMV and increased activation.
Publication Bias
Egger's tests were performed to examine potential publication bias. The results of the Egger tests were non-significant (P > 0.05 for all comparisons, Bonferroni corrected), suggesting that there was no publication bias. Jack-knife reliability analyses suggested robust disorder-differentiating findings.
Discussion
The purpose of our meta-analytic comparison is to show that patients with IGD and ADHD have predominantly shared and disorder-specific patterns of structural and functional abnormalities, especially in reward function. Structurally, IGD people have decreased putamen GMV and ADHD patients have lower GMV in the orbitofrontal cortex. Functionally, precuneus was reported as disorder-special activation in IGD patients. Furthermore, functional alteration in the OFC was opposite, which is activated in IGDs and hypoactive in ADHD. Moreover, disorder-specific increased GMV and functional activation were found in the precuneus among IGD patients and in the fusiform gyrus in ADHD patients through multimodal analysis. Patients with IGD and ADHD showed commonly the same direction of change in the ACC (decreased GMV and hyperactivation) and insula (decreased GMV and lower activation). In addition, striatum, expecting abnormal structure in both two disorders, was reported to be reduced in GMV and functional connectivity in the IGD group and reduced in GMV and no significant change in fMRI for the ADHD group.
The key disorder-shared abnormality in two disorders both in structure and function is the prefrontal-striatum circuit. The circuit network contains the anterior cingulate cortex, the orbital prefrontal cortex, the ventral striatum, the ventral pallidum, the dorsal prefrontal cortex, amygdala, hippocampus (16). Attention-deficit/hyperactivity disorder (ADHD) has been conceptualized as a disorder of the prefrontal cortex for over 30 years (28) and IGD is found to be defective in PFC through various studies (22, 40), our results showed that parts of structural and functional alterations in ADHD and IGD patients concentrated on PFC areas. The main cortical areas in the PFC areas associated with reward are the anterior cingulate cortex and orbitofrontal cortex.
As predicted, the results of the main meta-analyses converged on the ACC, which showed functional hyperactivation and gray-matter reduction in IGDs and ADHD relative to HCs. This finding is in line with recent transdiagnostic meta-analyses that this region may serve as a common bio-marker across psychiatric disorders (128), possibly because it modulates the neural activity of the default-mode network and executive control network (129) and is critically involved in multiple processes including cognitive control (130), emotional regulation (131), and reward-relative decision-making (132). Bonath et al. found significantly smaller ACC gray matter volume in subjects with ADHD and reduced volume in ACC was directly associated with symptoms of attentional deficits (49). IGD participants cannot control their compulsion to play Internet games despite experiencing negative consequences due to impaired cognitive control of ACC (40). These studies are consistent with our findings. Neural alterations in the ACC area, consistent with the interaction of the Person-Affect-Cognition-Execution model, play an important role in cue-induced craving, rewards-seeking, (22) and cognitive control in the ACC among IGD and ADHD patients. Moreover, although the direction of the alteration of the ACC is inconsistent across modalities (fMRI and VBM), previous evidence suggests that gray-matter-volume increases or reductions may not simply correspond to functional neural activation or deactivation (132). In conclusion, fMRI and VBM may reflect the distinctive aspects of neural alterations, and the evidence converges to emphasize an important role for the ACC in IGD and ADHD.
The disorder-contrasting findings in OFC are worth discussion. Now it is commonly understood that the OFC contributes to psychotic dysfunction including impulse control and monitoring ongoing behavior and rewards-seeking behaviors (44). Our decreased OFC activation in ADHD is consistent with a previous study that showed decreased cognitive capacity, which is related to hyperactivity and impulsivity and is associated with reduced OFC activity during reward expectation in ADHD patients (133). Furthermore, the strong activation of OFC in IGD patients might be explained by pleasant objects and rewarding anticipation, which refers to internet games in IGD, so that IGD people are more eager to look for stimulation and rewards.
As for the striatum, which comprises the caudate nucleus and the putamen, the nucleus accumbens (NAc), and the olfactory tubercle, which appear in our result. When IGD or ADHD patients are exposed to cue-relative stimulation, the activation of glutamatergic projections from the ventral PFC, the ventral hippocampus, and the amygdala (and presumably medial thalamus) to striatal projections that increase DA signaling and release in the NAc and dorsal striatum will enhance reward craving and eventually result in game activity in IGD and distraction in ADHD (18). However, we found reduced striatum GMV in the IGD and ADHD group and lower functional connectivity in the IGD group, but there was no significant change in fMRI for the ADHD group. Using all kinds of checkout, there was still no significant functional connection alteration in striatum among ADHD patients. We speculate that the reasons could include ADHD fMRI studies that claim there was inconsistent striatum action, meaning there was no result when putting these studies together to conduct meta-analyses.
We found consistent changes in the insula, which had decreased GMV and lower activity in ADHD and IGD subjects. The insula are involved in motivation, rewards, salience detection, and cognitive control (98, 134, 135), modulated by dopaminergic activity (134), which is typically decreased in IGD and ADHD. Therefore, the insula is hypothesized to be a neural system that increases reward drivers and weakens cognitive control (136). In ADHD, deficient insula activation may result in reduced task-related salience detection and cognitive control, resulting in lower self-control ability and increased distractibility. IGD hypoactivation in the insula probably shows that they are habituated to gaming-related stimulation and insensitive to other conventional stimulation, which contributes to gaming addiction.
We find decreased putamen GMV in IGD people through VBM analysis. The dorsolateral putamen has been functionally linked to the sensorimotor cortices, forming the sensorimotor network. A recent research report that health controls show a significant positive correlation in the neural pathways connecting the putamen-MFG-insula when facing gaming cues, which is missing in individuals with IGD (136). Meanwhile, this study also demonstrated increased excitatory neuromodulation in the effective connections among the insula-putamen-OFC in IGD, a neural pathway involving reward-related activity. In conclusion, the putamen is part of the reward pathway, the declination of putamen gray matter may impact its function, which is part of the reason for game addiction.
In our study, disorder-specific activation is suggested in the precuneus among IGD patients. The precuneus is associated with visual imagery, attention, and memory retrieval by participating in the visual process and integrates related memory (137). A possible explanation is that high activation in the precuneus is relative to gaming urge, craving, and the severity of Internet addiction. This result suggests that the precuneus activates to process the gaming cue, and contributes to the cue-induced craving for online gaming. Furthermore, ADHD patients have special activation in the fusiform gyrus. The findings are consistent with a study of reward effect on brain structure and function in adults and children with ADHD (54). The fusiform gyrus (FG), which topographically connects the striate cortex to the inferior temporal lobe, plays a pivotal role in high-level visual/cognitive functions (138). Speculation is that fusiform mediate various stimuli that result in it being hard for people with ADHD to focus on what they are doing.
Limitation
This meta-analysis has several limitations. First, it was based primarily on peak coordinates rather than raw statistical brain maps. Besides, the heterogeneity of the methodologies among VBM studies could not be avoided, such as the differences in MRI machines, slice thickness, preprocessing protocols (traditional or optimized), and smoothing kernel size, which might have contributed to the inconsistent results (33). Moreover, the included studies have different proportions of males and diverse statistical thresholds, which may lead to discrepant results. Previous studies suggest that neural alterations in some regions may be more severe in female IGDs and ADHDs (17), but future studies are needed to shed more light on gender difference and conduct further research.
Conclusion
The comparative meta-analytic findings of this study stress the shared and distinctive brain structure and function in IGD and ADHD. Disorder-differentiating structure alterations are reported in the putamen for IGD and in the orbitofrontal cortex for ADHD subjects. Disorder-differentiating fMRI activation was predominantly observed in the precuneus among IGD subjects. The shared functional alterations focus on the frontal-striatum reward circuit, which is important for understanding the underlying pathophysiology and proves that these two disorders have a common neurological foundation. Disorder-shared neurofunctional biomarkers provide useful evidence that the drugs treat ADHD could be used on IGD. Disorder-specific neurofunctional biomarkers could ultimately aid in the development of future, disorder-differentiated behavioral, pharmacological, or neurotherapeutic treatments.
Data Availability Statement
The original contributions presented in the study are included in the article, further inquiries can be directed to the corresponding authors.
Author Contributions
XG and YZ designed the experiment. XG, MZ, and ZY performed the experiment. MW, HH, RZ, WW, YW, JC, SH, and YZ modified the experiment and paper. All authors contributed to the article and approved the submitted version.
Conflict of Interest
The authors declare that the research was conducted in the absence of any commercial or financial relationships that could be construed as a potential conflict of interest.
References
1. Ko C-H, Yen J-Y, Chen S-H, Wang P-W, Chen C-S, Yen C-F. Evaluation of the diagnostic criteria of Internet gaming disorder in the DSM-5 among young adults in Taiwan. J Psychiatr Res. (2014) 53:103–10. doi: 10.1016/j.jpsychires.2014.02.008
2. Liu L, Yip SW, Zhang JT, Wang LJ, Shen ZJ, Liu B, et al. Activation of the ventral and dorsal striatum during cue reactivity in Internet gaming disorder. Addict Biol. (2017) 22:791–801. doi: 10.1111/adb.12338
3. Choi SW, Kim HS, Kim GY, Jeon Y, Park SM, Lee JY, et al. Similarities and differences among Internet gaming disorder, gambling disorder and alcohol use disorder: a focus on impulsivity and compulsivity. J Behav Addict. (2014) 3:246–53. doi: 10.1556/jba.3.2014.4.6
4. Yao YW, Wang LJ, Yip SW, Chen PR, Li S, Xu J, et al. Impaired decision-making under risk is associated with gaming-specific inhibition deficits among college students with Internet gaming disorder. Psychiatry Res. (2015) 229:302–9. doi: 10.1016/j.psychres.2015.07.004
5. Müller KW, Janikian M, Dreier M, Wölfling K, Beutel ME, Tzavara C, et al. Regular gaming behavior and internet gaming disorder in European adolescents: results from a cross-national representative survey of prevalence, predictors, and psychopathological correlates. Eur Child Adolesc Psychiatry. (2015) 24:565–74. doi: 10.1007/s00787-014-0611-2
6. Przybylski AK, Weinstein N, Murayama K. Internet gaming disorder: investigating the clinical relevance of a new phenomenon. Am J Psychiatry. (2017) 174:230–6. doi: 10.1176/appi.ajp.2016.16020224
7. Rehbein F, Kliem S, Baier D, Mößle T, Petry NM. Prevalence of Internet gaming disorder in German adolescents: diagnostic contribution of the nine DSM-5 criteria in a state-wide representative sample. Addiction. (2015) 110:842–51. doi: 10.1111/add.12849
8. Wang HR, Cho H, Kim DJ. Prevalence and correlates of comorbid depression in a nonclinical online sample with DSM-5 internet gaming disorder. J Affect Disord. (2018) 226:1–5. doi: 10.1016/j.jad.2017.08.005
9. Polanczyk GV, Willcutt EG, Salum GA, Kieling C, Rohde LA. ADHD prevalence estimates across three decades: an updated systematic review and meta-regression analysis. Int J Epidemiol. (2014) 43:434–42. doi: 10.1093/ije/dyt261
10. Gallo EF, Posner J. Moving towards causality in attention-deficit hyperactivity disorder: overview of neural and genetic mechanisms. Lancet Psychiatry. (2016) 3:555–67. doi: 10.1016/s2215-0366(16)00096-1
11. Kim D, Lee D, Lee J, Namkoong K, Jung YC. Association between childhood and adult attention deficit hyperactivity disorder symptoms in Korean young adults with Internet addiction. J Behav Addict. (2017) 6:345–53. doi: 10.1556/2006.6.2017.044
12. Yen JY, Liu TL, Wang PW, Chen CS, Yen CF, Ko CH. Association between Internet gaming disorder and adult attention deficit and hyperactivity disorder and their correlates: impulsivity and hostility. Addict Behav. (2017) 64:308–13. doi: 10.1016/j.addbeh.2016.04.024
13. Ding WN, Sun JH, Sun YW, Chen X, Zhou Y, Zhuang ZG, et al. Trait impulsivity and impaired prefrontal impulse inhibition function in adolescents with internet gaming addiction revealed by a Go/No-Go fMRI study. Behav Brain Funct. (2014) 10:20. doi: 10.1186/1744-9081-10-20
14. Ko CH, Yen JY, Chen CS, Yeh YC, Yen CF. Predictive values of psychiatric symptoms for internet addiction in adolescents: a 2-year prospective study. Arch Pediatr Adolesc Med. (2009) 163:937–43. doi: 10.1001/archpediatrics.2009.159
15. Bush G. Cingulate, frontal, and parietal cortical dysfunction in attention-deficit/hyperactivity disorder. Biol Psychiatry. (2011) 69:1160–7. doi: 10.1016/j.biopsych.2011.01.022
16. Haber SN, Knutson B. The reward circuit: linking primate anatomy and human imaging. Neuropsychopharmacology. (2010) 35:4–26. doi: 10.1038/npp.2009.129
17. Yao YW, Liu L, Ma SS, Shi XH, Zhou N, Zhang JT, et al. Functional and structural neural alterations in Internet gaming disorder: A systematic review and meta-analysis. Neurosci Biobehav Rev. (2017) 83:313–24. doi: 10.1016/j.neubiorev.2017.10.029
18. Volkow ND, Morales M. The brain on drugs: from reward to addiction. Cell. (2015) 162:712–25. doi: 10.1016/j.cell.2015.07.046
19. Weinstein AM. Computer and video game addiction-a comparison between game users and non-game users. Am J Drug Alcohol Abuse. (2010) 36:268–76. doi: 10.3109/00952990.2010.491879
20. Sun Y, Ying H, Seetohul RM, Xuemei W, Ya Z, Qian L, et al. Brain fMRI study of crave induced by cue pictures in online game addicts (male adolescents). Behav Brain Res. (2012) 233:563–76. doi: 10.1016/j.bbr.2012.05.005
21. He Q, Turel O, Wei L, Bechara A. Structural brain differences associated with extensive massively-multiplayer video gaming. Brain Imag Behav. (2020) 15:364–74. doi: 10.1007/s11682-020-00263-0
22. Lee D, Namkoong K, Lee J, Jung Y-C. Abnormal gray matter volume and impulsivity in young adults with Internet gaming disorder. Addiction biology. (2018) 23:1160–1167. doi: 10.1111/adb.12552
23. Blum K, Chen AL, Braverman ER, Comings DE, Chen TJ, Arcuri V, et al. Attention-deficit-hyperactivity disorder and reward deficiency syndrome. Neuropsychiatric Dis Treatment. (2008) 4:893–918. doi: 10.2147/ndt.s2627
24. Han DH, Bae S, Hong J, Kim SM, Son YD, Renshaw P. Resting-state fMRI study of ADHD and internet gaming disorder. J Atten Disord. (2019) 2019:1087054719883022. doi: 10.1177/1087054719883022
25. Frodl T, Skokauskas N. Meta-analysis of structural MRI studies in children and adults with attention deficit hyperactivity disorder indicates treatment effects. Acta Psychiatr Scand. (2012) 125:114–26. doi: 10.1111/j.1600-0447.2011.01786.x
26. Norman LJ, Carlisi C, Lukito S, Hart H, Mataix-Cols D, Radua J, et al. Structural and functional brain abnormalities in attention-deficit/hyperactivity disorder and obsessive-compulsive disorder: a comparative meta-analysis. JAMA Psychiatry. (2016) 73:815–25. doi: 10.1001/jamapsychiatry.2016.0700
27. Hoogman M, Muetzel R, Guimaraes JP, Shumskaya E, Mennes M, Zwiers MP, et al. Brain imaging of the cortex in ADHD: a coordinated analysis of large-scale clinical and population-based samples. Am J Psychiatry. (2019) 176:531–42. doi: 10.1176/appi.ajp.2019.18091033
28. Durston S, van Belle J, de Zeeuw P. Differentiating frontostriatal and fronto-cerebellar circuits in attention-deficit/hyperactivity disorder. Biol Psychiatry. (2011) 69:1178–84. doi: 10.1016/j.biopsych.2010.07.037
29. Lee D, Namkoong K, Lee J, Jung YC. Preliminary evidence of altered gray matter volume in subjects with internet gaming disorder: associations with history of childhood attention-deficit/hyperactivity disorder symptoms. Brain Imaging Behav. (2019) 13:660–8. doi: 10.1007/s11682-018-9872-6
30. Hart H, Radua J, Nakao T, Mataix-Cols D, Rubia K. Meta-analysis of functional magnetic resonance imaging studies of inhibition and attention in attention-deficit/hyperactivity disorder: exploring task-specific, stimulant medication, and age effects. JAMA Psychiatry. (2013) 70:185–98. doi: 10.1001/jamapsychiatry.2013.277
31. McCarthy H, Skokauskas N, Frodl T. Identifying a consistent pattern of neural function in attention deficit hyperactivity disorder: a meta-analysis. Psychol Med. (2014) 44:869–80. doi: 10.1017/s0033291713001037
32. Radua J, Mataix-Cols D. Voxel-wise meta-analysis of grey matter changes in obsessive-compulsive disorder. Br J Psychiatry. (2009) 195:393–402. doi: 10.1192/bjp.bp.108.055046
33. Yang Z, Zhang Y, Cheng J, Zheng R. Meta-analysis of brain gray matter changes in chronic smokers. Eur J Radiol. (2020) 132:109300. doi: 10.1016/j.ejrad.2020.109300
34. Radua J, Mataix-Cols D, Phillips ML, El-Hage W, Kronhaus DM, Cardoner N, et al. A new meta-analytic method for neuroimaging studies that combines reported peak coordinates and statistical parametric maps. Eur Psychiatry. (2012) 27:605–11. doi: 10.1016/j.eurpsy.2011.04.001
35. Radua J, Borgwardt S, Crescini A, Mataix-Cols D, Meyer-Lindenberg A, McGuire PK, et al. Multimodal meta-analysis of structural and functional brain changes in first episode psychosis and the effects of antipsychotic medication. Neurosci Biobehav Rev. (2012) 36:2325–33. doi: 10.1016/j.neubiorev.2012.07.012
36. Du X, Qi X, Yang Y, Du G, Gao P, Zhang Y, et al. Altered Structural Correlates of Impulsivity in Adolescents with Internet Gaming Disorder. Front Hum Neurosci. (2016) 10:4. doi: 10.3389/fnhum.2016.00004
37. Han DH, Lyoo IK, Renshaw PF. Differential regional gray matter volumes in patients with on-line game addiction and professional gamers. J Psychiatr Res. (2012) 46:507–15. doi: 10.1016/j.jpsychires.2012.01.004
38. Jin C, Zhang T, Cai C, Bi Y, Li Y, Yu D, et al. Abnormal prefrontal cortex resting state functional connectivity and severity of internet gaming disorder. Brain Imag Behav. (2016) 10:719–29. doi: 10.1007/s11682-015-9439-8
39. Ko C-H, Hsieh T-J, Wang P-W, Lin W-C, Yen C-F, Chen C-S, et al. Altered gray matter density and disrupted functional connectivity of the amygdala in adults with Internet gaming disorder. Prog Neuro-Psychopharmacol Biol Psychiatry. (2015) 57:185–92. doi: 10.1016/j.pnpbp.2014.11.003
40. Lin X, Dong G, Wang Q, Du X. Abnormal gray matter and white matter volume in 'Internet gaming addicts'. Addict Behav. (2015) 40:137–43. doi: 10.1016/j.addbeh.2014.09.010
41. Mohammadi B, Szycik GR, Te Wildt B, Heldmann M, Samii A, Münte TF. Structural brain changes in young males addicted to video-gaming. Brain Cognit. (2020) 139:105518. doi: 10.1016/j.bandc.2020.105518
42. Seok J-W, Sohn J-H. Altered gray matter volume and resting-state connectivity in individuals with internet gaming disorder: a voxel-based morphometry and resting-state functional magnetic resonance imaging study. Front Psychiatry. (2018) 9:77. doi: 10.3389/fpsyt.2018.00077
43. Sun Y, Sun J, Zhou Y, Ding W, Chen X, Zhuang Z, et al. Assessment of in vivo microstructure alterations in gray matter using DKI in Internet gaming addiction. Behav Brain Funct: BBF. (2014) 10:37. doi: 10.1186/1744-9081-10-37
44. Weng C-B, Qian R-B, Fu X-M, Lin B, Han X-P, Niu C-S, et al. Gray matter and white matter abnormalities in online game addiction. Euro J Radiol. (2013) 82:1308–12. doi: 10.1016/j.ejrad.2013.01.031
45. Yoon EJ, Choi J-S, Kim H, Sohn BK, Jung HY, Lee J-Y, et al. Altered hippocampal volume and functional connectivity in males with Internet gaming disorder comparing to those with alcohol use disorder. Sci Rep. (2017) 7:5744. doi: 10.1038/s41598-017-06057-7
46. Zhou Y, Lin F-C, Du Y-S, Qin L-d, Zhao Z-M, Xu J-R, et al. Gray matter abnormalities in Internet addiction: a voxel-based morphometry study. Eur J Radiol. (2011) 79:92–5. doi: 10.1016/j.ejrad.2009.10.025
47. Ahrendts J, Rüsch N, Wilke M, Philipsen A, Eickhoff SB, Glauche V, et al. Visual cortex abnormalities in adults with ADHD: a structural MRI study. World J Biol Psychiatry. (2011) 12:260–70. doi: 10.3109/15622975.2010.518624
48. Amico F, Stauber J, Koutsouleris N, Frodl T. Anterior cingulate cortex gray matter abnormalities in adults with attention deficit hyperactivity disorder: a voxel-based morphometry study. Psychiatry Res. (2011) 191:31–5. doi: 10.1016/j.pscychresns.2010.08.011
49. Bonath B, Tegelbeckers J, Wilke M, Flechtner H-H, Krauel K. Regional gray matter volume differences between adolescents with ADHD and typically developing controls: further evidence for anterior cingulate involvement. J Attent Disord. (2018) 22:627–38. doi: 10.1177/1087054715619682
50. Bralten J, Greven CU, Franke B, Mennes M, Zwiers MP, Rommelse NNJ, et al. Voxel-based morphometry analysis reveals frontal brain differences in participants with ADHD and their unaffected siblings. J Psychiatry Neurosci: JPN. (2016) 41:272–9. doi: 10.1503/jpn.140377
51. Gehricke J-G, Kruggel F, Thampipop T, Alejo SD, Tatos E, Fallon J, et al. The brain anatomy of attention-deficit/hyperactivity disorder in young adults - a magnetic resonance imaging study. PLoS ONE. (2017) 12:e0175433. doi: 10.1371/journal.pone.0175433
52. He N, Li F, Li Y, Guo L, Chen L, Huang X, et al. Neuroanatomical deficits correlate with executive dysfunction in boys with attention deficit hyperactivity disorder. Neurosci Lett. (2015) 600:45–9. doi: 10.1016/j.neulet.2015.05.062
53. Jagger-Rickels AC, Kibby MY, Constance JM. Global gray matter morphometry differences between children with reading disability, ADHD, and comorbid reading disability/ADHD. Brain Lang. (2018) 185:54–66. doi: 10.1016/j.bandl.2018.08.004
54. Kappel V, Lorenz RC, Streifling M, Renneberg B, Lehmkuhl U, Ströhle A, et al. Effect of brain structure and function on reward anticipation in children and adults with attention deficit hyperactivity disorder combined subtype. Soc Cogn Affect Neurosci. (2015) 10:945–51. doi: 10.1093/scan/nsu135
55. Sutcubasi Kaya B, Metin B, Tas ZC, Buyukaslan A, Soysal A, Hatiloglu D, et al. Gray matter increase in motor cortex in pediatric ADHD: a voxel-based morphometry study. J Atten Disord. (2018) 22:611–8. doi: 10.1177/1087054716659139
56. Klein M, Souza-Duran FL, Menezes AKPM, Alves TM, Busatto G, Louzã MR. Gray matter volume in elderly adults with ADHD: associations of symptoms and comorbidities with brain structures. J Atten Disord. (2019) 2019:1087054719855683. doi: 10.1177/1087054719855683
57. Kobel M, Bechtel N, Specht K, Klarhöfer M, Weber P, Scheffler K, et al. Structural and functional imaging approaches in attention deficit/hyperactivity disorder: does the temporal lobe play a key role? Psychiatry Res. (2010) 183:230–6. doi: 10.1016/j.pscychresns.2010.03.010
58. Kumar U, Arya A, Agarwal V. Neural alterations in ADHD children as indicated by voxel-based cortical thickness and morphometry analysis. Brain Dev. (2017) 39:403–10. doi: 10.1016/j.braindev.2016.12.002
59. Li X, Cao Q, Pu F, Li D, Fan Y, An L, et al. Abnormalities of structural covariance networks in drug-naïve boys with attention deficit hyperactivity disorder. Psychiatry Res. (2015) 231:273–8. doi: 10.1016/j.pscychresns.2015.01.006
60. Lim L, Marquand A, Cubillo AA, Smith AB, Chantiluke K, Simmons A, et al. Disorder-specific predictive classification of adolescents with attention deficit hyperactivity disorder (ADHD) relative to autism using structural magnetic resonance imaging. PLoS ONE. (2013) 8:e63660. doi: 10.1371/journal.pone.0063660
61. Almeida Montes LG, Ricardo-Garcell J, Barajas De La Torre LB, Prado Alcántara H, Martínez García RB, Fernández-Bouzas A, et al. Clinical correlations of grey matter reductions in the caudate nucleus of adults with attention deficit hyperactivity disorder. J Psychiatry Neurosci. (2010) 35:238–46. doi: 10.1503/jpn.090099
62. Moreno-Alcázar A, Ramos-Quiroga JA, Radua J, Salavert J, Palomar G, Bosch R, et al. Brain abnormalities in adults with attention deficit hyperactivity disorder revealed by voxel-based morphometry. Psychiatry Res Neuroimag. (2016) 254:41–7. doi: 10.1016/j.pscychresns.2016.06.002
63. Ramesh MG, Rai KS. Region-wise gray matter volume alterations in brain of adolescents with attention deficit hyperactive disorder: a voxel based morphometric analysis. Ind J Physiol Pharmacol. (2013). 57:270–9.
64. Roman-Urrestarazu A, Lindholm P, Moilanen I, Kiviniemi V, Miettunen J, Jääskeläinen E, et al. Brain structural deficits and working memory fMRI dysfunction in young adults who were diagnosed with ADHD in adolescence. Eur Child Adolesc Psychiatry. (2016) 25:529–38. doi: 10.1007/s00787-015-0755-8
65. Seidman LJ, Biederman J, Liang L, Valera EM, Monuteaux MC, Brown A, et al. Gray matter alterations in adults with attention-deficit/hyperactivity disorder identified by voxel based morphometry. Biol Psychiatry. (2011) 69:857–66. doi: 10.1016/j.biopsych.2010.09.053
66. Sethi A, Evelyn-Rahr E, Dowell N, Jain S, Voon V, Critchley HD, et al. Magnetization transfer imaging identifies basal ganglia abnormalities in adult ADHD that are invisible to conventional T1 weighted voxel-based morphometry. NeuroImage Clin. (2017) 15:12. doi: 10.1016/j.nicl.2017.03.012
67. Shimada K, Fujisawa TX, Takiguchi S, Naruse H, Kosaka H, Okazawa H, et al. Ethnic differences in COMT genetic effects on striatal grey matter alterations associated with childhood ADHD: A voxel-based morphometry study in a Japanese sample. World J Biol Psychiatry. (2017) 18:322–8. doi: 10.3109/15622975.2015.1102325
68. van Wingen GA, van den Brink W, Veltman DJ, Schmaal L, Dom G, Booij J, et al. Reduced striatal brain volumes in non-medicated adult ADHD patients with comorbid cocaine dependence. Drug Alcohol Depend. (2013) 131:198–203. doi: 10.1016/j.drugalcdep.2013.05.007
69. Vilgis V, Sun L, Chen J, Silk TJ, Vance A. Global and local grey matter reductions in boys with ADHD combined type and ADHD inattentive type. Psychiatry Res Neuroimag. (2016) 254:119–26. doi: 10.1016/j.pscychresns.2016.06.008
70. Villemonteix T, De Brito SA, Slama H, Kavec M, Balériaux D, Metens T, et al. Structural correlates of COMT Val158Met polymorphism in childhood ADHD: a voxel-based morphometry study. World J Biol Psychiatry. (2015) 16:190–9. doi: 10.3109/15622975.2014.984629
71. Wang L-J, Li S-C, Kuo H-C, Chou W-J, Lee M-J, Chou M-C, et al. Gray matter volume and microRNA levels in patients with attention-deficit/hyperactivity disorder. Eur Arch Psychiatry Clin Neurosci. (2019) 270:1037–45. doi: 10.1007/s00406-019-01032-x
72. Zhao Y, Cui D, Lu W, Li H, Zhang H, Qiu J. Aberrant gray matter volumes and functional connectivity in adolescent patients with ADHD. J Magnet Resonance Imaging: JMRI. (2020) 51:719–26. doi: 10.1002/jmri.26854
73. Chen CY, Huang MF, Yen JY, Chen CS, Liu GC, Yen CF, et al. Brain correlates of response inhibition in Internet gaming disorder. Psychiatry Clin Neurosci. (2015) 69:201–9. doi: 10.1111/pcn.12224
74. Chun JW, Choi J, Cho H, Lee SK, Kim DJ. Dysfunction of the frontolimbic region during swear word processing in young adolescents with Internet gaming disorder. Transl Psychiatry. (2015) 5:e624. doi: 10.1038/tp.2015.106
75. Dieter J, Hill H, Sell M, Reinhard I, Vollstädt-Klein S, Kiefer F, et al. Avatar's neurobiological traces in the self-concept of massively multiplayer online role-playing game (MMORPG) addicts. Behav Neurosci. (2015) 129:25. doi: 10.1037/bne0000025
76. Dong G, Li H, Wang L, Potenza MN. Cognitive control and reward/loss processing in Internet gaming disorder: Results from a comparison with recreational Internet game-users. Eur Psychiatry. (2017) 44:30–8. doi: 10.1016/j.eurpsy.2017.03.004
77. Dong G, Hu Y, Lin X, Lu Q. What makes Internet addicts continue playing online even when faced by severe negative consequences? Possible explanations from an fMRI study. Biol Psychol. (2013) 94:282–9. doi: 10.1016/j.biopsycho.2013.07.009
78. Dong G, Huang J, Du X. Enhanced reward sensitivity and decreased loss sensitivity in Internet addicts: an fMRI study during a guessing task. J Psychiatr Res. (2011) 45:1525–9. doi: 10.1016/j.jpsychires.2011.06.017
79. Dong G, Potenza MN. Risk-taking and risky decision-making in Internet gaming disorder: Implications regarding online gaming in the setting of negative consequences. J Psychiatric Res. (2016) 73:1–8. doi: 10.1016/j.jpsychires.2015.11.011
80. Dong G, Lin X, Zhou H, Lu Q. Cognitive flexibility in internet addicts: fMRI evidence from difficult-to-easy and easy-to-difficult switching situations. Addict Behav. (2014) 39:677–83. doi: 10.1016/j.addbeh.2013.11.028
81. Han DH, Kim SM, Lee YS, Renshaw PF. The effect of family therapy on the changes in the severity of on-line game play and brain activity in adolescents with on-line game addiction. Psychiatry Res. (2012) 202:126–31. doi: 10.1016/j.pscychresns.2012.02.011
82. Kim SM, Han DH, Lee YS, Kim JE, Renshaw PF. Changes in brain activity in response to problem solving during the abstinence from online game play. J Behav Addict. (2012) 1:41–9. doi: 10.1556/JBA.1.2012.2.1
83. Ko C-H, Liu G-C, Yen J-Y, Chen C-Y, Yen C-F, Chen C-S. Brain correlates of craving for online gaming under cue exposure in subjects with Internet gaming addiction and in remitted subjects. Addict Biol. (2013) 18:559–69. doi: 10.1111/j.1369-1600.2011.00405.x
84. Ko C-H, Hsieh T-J, Chen C-Y, Yen C-F, Chen C-S, Yen J-Y, et al. Altered brain activation during response inhibition and error processing in subjects with Internet gaming disorder: a functional magnetic imaging study. Eur Arch Psychiatry Clin Neurosci. (2014) 264:661–72. doi: 10.1007/s00406-013-0483-3
85. Lee D, Lee J, Yoon KJ, Kee N, Jung Y-C. Impaired anterior insular activation during risky decision making in young adults with internet gaming disorder. Neuroreport. (2016) 27:605–9. doi: 10.1097/WNR.0000000000000584
86. Lee J, Lee S, Chun JW, Cho H, Kim D-j, Jung Y-C. Compromised prefrontal cognitive control over emotional interference in adolescents with internet gaming disorder. Cyberpsychol Behav Soc Networ. (2015) 18:661–8. doi: 10.1089/cyber.2015.0231
87. Leménager T, Dieter J, Hill H, Koopmann A, Reinhard I, Sell M, et al. Neurobiological correlates of physical self-concept and self-identification with avatars in addicted players of Massively Multiplayer Online Role-Playing Games (MMORPGs). Addict Behav. (2014) 39:1789–97. doi: 10.1016/j.addbeh.2014.07.017
88. Lin X, Zhou H, Dong G, Du X. Impaired risk evaluation in people with Internet gaming disorder: fMRI evidence from a probability discounting task. Prog Neuro-Psychopharmacol Biol Psychiatry. (2015) 56:142–8. doi: 10.1016/j.pnpbp.2014.08.016
89. Liu G-C, Yen J-Y, Chen C-Y, Yen C-F, Chen C-S, Lin W-C, et al. Brain activation for response inhibition under gaming cue distraction in internet gaming disorder. Kaohsiung J Med Sci. (2014) 30:43–51. doi: 10.1016/j.kjms.2013.08.005
90. Liu L, Xue G, Potenza MN, Zhang J-T, Yao Y-W, Xia C-C, et al. Dissociable neural processes during risky decision-making in individuals with Internet-gaming disorder. NeuroImage Clin. (2017) 14:741–9. doi: 10.1016/j.nicl.2017.03.010
91. Lorenz RC, Krüger J-K, Neumann B, Schott BH, Kaufmann C, Heinz A, et al. Cue reactivity and its inhibition in pathological computer game players. Addict Biol. (2013) 18:134–46. doi: 10.1111/j.1369-1600.2012.00491.x
92. Ma S-S, Worhunsky PD, Xu J-S, Yip SW, Zhou N, Zhang J-T, et al. Alterations in functional networks during cue-reactivity in Internet gaming disorder. J Behav Addict. (2019) 8:277–87. doi: 10.1556/2006.8.2019.25
93. Qi X, Du X, Yang Y, Du G, Gao P, Zhang Y, et al. Decreased modulation by the risk level on the brain activation during decision making in adolescents with internet gaming disorder. Front Behav Neurosci. (2015) 9:296. doi: 10.3389/fnbeh.2015.00296
94. Qi X, Yang Y, Dai S, Gao P, Du X, Zhang Y, et al. Effects of outcome on the covariance between risk level and brain activity in adolescents with internet gaming disorder. NeuroImage Clin. (2016) 12:845-51. doi: 10.1016/j.nicl.2016.10.024
95. Shin Y-B, Kim H, Kim S-J, Kim J-J. A neural mechanism of the relationship between impulsivity and emotion dysregulation in patients with Internet gaming disorder. Addict Biol. (2020) 2020:e12916. doi: 10.1111/adb.12916
96. Wang Y, Wu L, Wang L, Zhang Y, Du X, Dong G. Impaired decision-making and impulse control in Internet gaming addicts: evidence from the comparison with recreational Internet game users. Addict Biol. (2017) 22:1610–21. doi: 10.1111/adb.12458
97. Zhang Y, Lin X, Zhou H, Xu J, Du X, Dong G. Brain activity toward gaming-related cues in internet gaming disorder during an addiction stroop task. Front Psychol. (2016) 7:714. doi: 10.3389/fpsyg.2016.00714
98. Zhang JT, Yao YW, Potenza MN, Xia CC, Lan J, Liu L, et al. Effects of craving behavioral intervention on neural substrates of cue-induced craving in Internet gaming disorder. Neuroimage Clin. (2016) 12:591–9. doi: 10.1016/j.nicl.2016.09.004
99. Cubillo A, Halari R, Ecker C, Giampietro V, Taylor E, Rubia K. Reduced activation and inter-regional functional connectivity of fronto-striatal networks in adults with childhood Attention-Deficit Hyperactivity Disorder (ADHD) and persisting symptoms during tasks of motor inhibition and cognitive switching. J Psychiatr Res. (2010) 44:629–39. doi: 10.1016/j.jpsychires.2009.11.016
100. Dibbets P, Evers E, Hurks P, Bakker K, Jolles J. Differential brain activation patterns in adult attention-deficit hyperactivity disorder (ADHD) associated with task switching. Neuropsychology. (2010) 24:413–23. doi: 10.1037/a0018997
101. Kooistra L, van der Meere J, Edwards J, Kaplan B, Crawford S, Goodyear B. Preliminary fMRI findings on the effects of event rate in adults with ADHD. J Neural Transm. (2010) 117:655–62. doi: 10.1007/s00702-010-0374-y
102. Passarotti A, Sweeney J, Pavuluri M. Neural correlates of response inhibition in pediatric bipolar disorder and attention deficit hyperactivity disorder. Psychiatry Res. (2010) 181:36–43. doi: 10.1016/j.pscychresns.2009.07.002
103. Cubillo A, Halari R, Giampietro V, Taylor E, Rubia K. Fronto-striatal underactivation during interference inhibition and attention allocation in grown up children with attention deficit/hyperactivity disorder and persistent symptoms. Psychiatry Res. (2011) 193:17–27. doi: 10.1016/j.pscychresns.2010.12.014
104. Rubia K, Halari R, Cubillo A, Smith A, Mohammad A, Brammer M, et al. Methylphenidate normalizes fronto-striatal underactivation during interference inhibition in medication-naïve boys with attention-deficit hyperactivity disorder. Neuropsychopharmacology. (2011) 36:1575–86. doi: 10.1038/npp.2011.30
105. Rubia K, Halari R, Mohammad A, Taylor E, Brammer M. Methylphenidate normalizes frontocingulate underactivation during error processing in attention-deficit/hyperactivity disorder. Biol Psychiatry. (2011) 70:255–62. doi: 10.1016/j.biopsych.2011.04.018
106. Spinelli S, Joel S, Nelson T, Vasa R, Pekar J, Mostofsky S. Different neural patterns are associated with trials preceding inhibitory errors in children with and without attention-deficit/hyperactivity disorder. J Am Acad Child Adolesc Psychiatry. (2011) 50:705–15.e3. doi: 10.1016/j.jaac.2011.03.014
107. Ma J, Lei D, Jin X, Du X, Jiang F, Li F, et al. Compensatory brain activation in children with attention deficit/hyperactivity disorder during a simplified Go/No-go task. J Neural Transmission. (2012) 119:613–9. doi: 10.1007/s00702-011-0744-0
108. Sebastian A, Gerdes B, Feige B, Klöppel S, Lange T, Philipsen A, et al. Neural correlates of interference inhibition, action withholding and action cancelation in adult ADHD. Psychiatry Res. (2012) 202:132–41. doi: 10.1016/j.pscychresns.2012.02.010
109. Siniatchkin M, Glatthaar N, von Müller G, Prehn-Kristensen A, Wolff S, Knöchel S, et al. Behavioural treatment increases activity in the cognitive neuronal networks in children with attention deficit/hyperactivity disorder. Brain Topograp. (2012) 25:332–44. doi: 10.1007/s10548-012-0221-6
110. Bhaijiwala M, Chevrier A, Schachar R. Withholding and canceling a response in ADHD adolescents. Brain Behav. (2014) 4:602–14. doi: 10.1002/brb3.244
111. Chantiluke K, Barrett N, Giampietro V, Santosh P, Brammer M, Simmons A, et al. Inverse fluoxetine effects on inhibitory brain activation in non-comorbid boys with ADHD and with ASD. Psychopharmacology. (2015) 232:2071–82. doi: 10.1007/s00213-014-3837-2
112. Cubillo A, Smith A, Barrett N, Giampietro V, Brammer M, Simmons A, et al. Shared and drug-specific effects of atomoxetine and methylphenidate on inhibitory brain dysfunction in medication-naive ADHD boys. Cereb Cortex. (2014) 24:174–85. doi: 10.1093/cercor/bhs296
113. Schulz K, Bédard A, Fan J, Clerkin S, Dima D, Newcorn J, et al. Emotional bias of cognitive control in adults with childhood attention-deficit/hyperactivity disorder. NeuroImage Clin. (2014) 5:1–9. doi: 10.1016/j.nicl.2014.05.016
114. Chen C, Yen J, Yen C, Chen C, Liu G, Liang C, et al. Aberrant brain activation of error processing among adults with attention deficit and hyperactivity disorder. Kaohsiung J Med Sci. (2015) 31:179–87. doi: 10.1016/j.kjms.2015.01.001
115. Janssen T, Heslenfeld D, van Mourik R, Logan G, Oosterlaan J. Neural correlates of response inhibition in children with attention-deficit/hyperactivity disorder: A controlled version of the stop-signal task. Psychiatry Res. (2015) 233:278–84. doi: 10.1016/j.pscychresns.2015.07.007
116. Rasmussen J, Casey B, van Erp T, Tamm L, Epstein J, Buss C, et al. ADHD and cannabis use in young adults examined using fMRI of a Go/NoGo task. Brain Imag Behav. (2016) 10:761–71. doi: 10.1007/s11682-015-9438-9
117. van Rooij D, Hoekstra PJ, Mennes M, von Rhein D, Thissen AJ, Heslenfeld D, et al. Distinguishing adolescents with ADHD from their unaffected siblings and healthy comparison subjects by neural activation patterns during response inhibition. Am J Psychiatry. (2015) 172:674–83. doi: 10.1176/appi.ajp.2014.13121635
118. Ma I, van Holstein M, Mies G, Mennes M, Buitelaar J, Cools R, et al. Ventral striatal hyperconnectivity during rewarded interference control in adolescents with ADHD. Cortex. (2016) 82:225–36. doi: 10.1016/j.cortex.2016.05.021
119. Zamorano F, Billeke P, Kausel L, Larrain J, Stecher X, Hurtado J, et al. Lateral prefrontal activity as a compensatory strategy for deficits of cortical processing in attention deficit hyperactivity disorder. Sci Rep. (2017) 7:7181. doi: 10.1038/s41598-017-07681-z
120. Fan L, Shang C, Tseng W, Gau S, Chou T. Visual processing as a potential endophenotype in youths with attention-deficit/hyperactivity disorder: A sibling study design using the counting Stroop functional MRI. Human Brain Mapp. (2018) 39:3827–35. doi: 10.1002/hbm.24214
121. Shang C, Sheng C, Yang L, Chou T, Gau S. Differential brain activations in adult attention-deficit/ hyperactivity disorder subtypes: a counting Stroop functional MRI study. Brain Imag Behav. (2018) 12:882–90. doi: 10.1007/s11682-017-9749-0
122. Thornton S, Bray S, Langevin L, Dewey D. Functional brain correlates of motor response inhibition in children with developmental coordination disorder and attention deficit/hyperactivity disorder. Hum. Movement Sci. (2018) 59:134–42. doi: 10.1016/j.humov.2018.03.018
123. Materna L, Wiesner CD, Shushakova A, Trieloff J, Weber N, Engell A, et al. Adult patients with ADHD differ from healthy controls in implicit, but not explicit, emotion regulation. J Psychiatry Neurosci. (2019) 44:340–9. doi: 10.1503/jpn.180139
124. Mehren A, Özyurt J, Thiel CM, Brandes M, Lam AP, Philipsen A. Effects of acute aerobic exercise on response inhibition in adult patients with ADHD. Sci Rep. (2019) 9:19884. doi: 10.1038/s41598-019-56332-y
125. Yang D-Y, Chi MH, Chu C-L, Lin C-Y, Hsu S-E, Chen KC, et al. Orbitofrontal dysfunction during the reward process in adults with ADHD: An fMRI study. Clin Neurophysiol. (2019) 130:627–33. doi: 10.1016/j.clinph.2019.01.022
126. Albajara Sáenz A, Septier M, Van Schuerbeek P, Baijot S, Deconinck N, Defresne P, et al. ADHD and ASD: distinct brain patterns of inhibition-related activation? Translational Psychiatry. (2020) 10:24. doi: 10.1038/s41398-020-0707-z
127. Pretus C, Picado M, Ramos-Quiroga A, Carmona S, Richarte V, Fauquet J, et al. Presence of distractor improves time estimation performance in an adult ADHD sample. J Attent Disord. (2020) 24:1530–7. doi: 10.1177/1087054716648776
128. Goodkind M, Eickhoff SB, Oathes DJ, Jiang Y, Chang A, Jones-Hagata LB, et al. Identification of a common neurobiological substrate for mental illness. JAMA Psychiatry. (2015) 72:305–15. doi: 10.1001/jamapsychiatry.2014.2206
129. Sutherland MT, McHugh MJ, Pariyadath V, Stein EA. Resting state functional connectivity in addiction: Lessons learned and a road ahead. Neuroimage. (2012) 62:2281–95. doi: 10.1016/j.neuroimage.2012.01.117
130. Meng Y, Deng W, Wang H, Guo W, Li T. The prefrontal dysfunction in individuals with Internet gaming disorder: a meta-analysis of functional magnetic resonance imaging studies. Addict Biol. (2015) 20:799–808. doi: 10.1111/adb.12154
131. Etkin A, Egner T, Kalisch R. Emotional processing in anterior cingulate and medial prefrontal cortex. Trends Cogn Sci. (2011) 15:85–93. doi: 10.1016/j.tics.2010.11.004
132. Kennerley SW, Walton ME, Behrens TE, Buckley MJ, Rushworth MF. Optimal decision making and the anterior cingulate cortex. Nat Neurosci. (2006) 9:940–7. doi: 10.1038/nn1724
133. Tegelbeckers J, Kanowski M, Krauel K, Haynes JD, Breitling C, Flechtner HH, et al. Orbitofrontal signaling of future reward is associated with hyperactivity in attention-deficit/hyperactivity disorder. J Neurosci. (2018) 38:6779–86. doi: 10.1523/jneurosci.0411-18.2018
134. Volkow ND, Wang GJ, Fowler JS, Ding YS. Imaging the effects of methylphenidate on brain dopamine: new model on its therapeutic actions for attention-deficit/hyperactivity disorder. Biol Psychiatry. (2005) 57:1410–5. doi: 10.1016/j.biopsych.2004.11.006
135. Chikama M, McFarland NR, Amaral DG, Haber SN. Insular cortical projections to functional regions of the striatum correlate with cortical cytoarchitectonic organization in the primate. J Neurosci. (1997) 17:9686–705. doi: 10.1523/jneurosci.17-24-09686.1997
136. Wang M, Dong H, Zheng H, Du X, Dong GH. Inhibitory neuromodulation of the putamen to the prefrontal cortex in Internet gaming disorder: How addiction impairs executive control. J Behav Addict. (2020) 9:312–24. doi: 10.1556/2006.2020.00029
137. Cavanna AE, Trimble MR. The precuneus: a review of its functional anatomy and behavioural correlates. Brain. (2006) 129:564–83. doi: 10.1093/brain/awl004
Keywords: internet gaming disorder, attention-deficit/hyperactivity disorder, rewards circuit, voxel-based morphometry, functional connectivity
Citation: Gao X, Zhang M, Yang Z, Wen M, Huang H, Zheng R, Wang W, Wei Y, Cheng J, Han S and Zhang Y (2021) Structural and Functional Brain Abnormalities in Internet Gaming Disorder and Attention-Deficit/Hyperactivity Disorder: A Comparative Meta-Analysis. Front. Psychiatry 12:679437. doi: 10.3389/fpsyt.2021.679437
Received: 11 March 2021; Accepted: 21 May 2021;
Published: 01 July 2021.
Edited by:
Holly Moore, National Institute on Drug Abuse (NIDA), United StatesReviewed by:
Georgios Demetrios Kotzalidis, Sapienza University of Rome, ItalyFengchun Wu, Guangzhou Medical University, China
Copyright © 2021 Gao, Zhang, Yang, Wen, Huang, Zheng, Wang, Wei, Cheng, Han and Zhang. This is an open-access article distributed under the terms of the Creative Commons Attribution License (CC BY). The use, distribution or reproduction in other forums is permitted, provided the original author(s) and the copyright owner(s) are credited and that the original publication in this journal is cited, in accordance with accepted academic practice. No use, distribution or reproduction is permitted which does not comply with these terms.
*Correspondence: Yong Zhang, zzuzhangyong2013@163.com; Jingliang Cheng, fccchengjl@zzu.edu.cn; Shaoqiang Han, shaoqianghan@163.com