- 1Institute of Behavioral Science in Medicine and Department of Psychiatry, Yonsei University College of Medicine, Seoul, South Korea
- 2Department of Neuropsychiatry, Veterans Health Service Medical Center, Seoul, South Korea
Introduction: Dynamic proteolysis, through the ubiquitin-proteasome system, has an important role in DNA transcription and cell cycle, and is considered to modulate cell stress response and synaptic plasticity. We investigated whether genetic variants in the ubiquitin carboxyl-terminal hydrolase 46 (USP46) would be associated with post-traumatic stress disorder (PTSD) in people with exposure to combat trauma using a case-control candidate gene association design.
Methods: Korean male veterans exposed to the Vietnam War were grouped into those with (n = 128) and without (n = 128) PTSD. Seven tagging SNPs of USP46 were selected, and single-marker and haplotype-based association analyses were performed. All analyses were adjusted for sociodemographic factors and levels of combat exposure severity and alcohol problem.
Results: One single-marker (rs2244291) showed nominal evidence of association with PTSD status and with the “re-experiencing” cluster, although the association was not significant after Bonferroni correction. No significant association with the other SNPs or the haplotypes was detected.
Conclusion: The present finding suggests preliminarily that genetic vulnerability regarding the ubiquitin-proteasome system may be related to fear memory processes and the development of PTSD symptoms after trauma exposure. Further studies with a larger sample size will be needed to examine the role of the ubiquitin-proteasome system including USP46 in PTSD.
Introduction
Post-traumatic stress disorder (PTSD) is a chronic and debilitating condition with characteristic symptoms, including re-experience of fear memory and severe anxiety as long-term responses to life-threatening traumatic exposure (1). However, not all people who are exposed to trauma develop PTSD. For instance, only around 10–20% of veterans exposed to combat trauma develop PTSD (2–4). The reason why certain individuals are more likely to develop PTSD than others after similar trauma exposure has not been elucidated. The molecular determinants of individual differences in vulnerability or resilience to stressors are still not well-understood. Twin studies have shown that PTSD is moderately heritable, with approximately 40% of the variance in PTSD attributable to genetic variance (5–7). Accummulating evidence shows that genetic factors contribute to the PTSD susceptibility among people who have experienced trauma (8, 9).
There is emerging literature on genetic variations in mechanisms responsible for development and maintenance of PTSD (9–11). Many studies on detection of candidate genes associated with PTSD have focused on genetic variations of the dopaminergic and serotonergic systems (12–15), but robust common genetic variants have yet to be identified. Since ubiquitin-proteasome-mediated proteolysis plays a crucial role in synaptic development and long-term synaptic plasticity in neural circuits (16–20), the ubiquitin-proteasome system is an interesting biological target for the pathophysiology of human neuropsychiatric disorders related to stress-related synaptic plasticity, such as PTSD. Dynamic and reversible processes via the ubiquitin-proteasome system regulate synaptic Alpha-amino-3-hydroxy-5-Methyl-4-isoxazolepropionic acid—type glutamate receptors (AMPARs) levels, which is known to be important for controlling development and function of glutamatergic synapses and long-term potentiation of synaptic transmission in the brain (21, 22). Substantial evidence from animal studies suggests that ubiquitin-mediated proteolysis is an important regulation process for fear memory formation and reconsolidation (23–26). To date, little is known about the genetic evidence of the role of ubiquitin-proteasome system in clinical samples of individuals with PTSD.
Ubiquitin carboxyl-terminal hydrolase 46 (USP46), a deubiquitinating enzyme that is widely expressed throughout the brain (19), was identified in Caenorhabditis elegans as the first deubiquitinating enzyme to regulate degradation of glutamate receptors and reported to have several roles in the nervous system (27). In mammalian studies, both in vivo and in vitro, USP46 has been implicated in regulating the AMPA glutamatergic system (28, 29), which is important for inter-neuronal communication and higher brain functions such as learning and memory. For example, altered AMPAR expression has been shown to modulate contextual fear memory reconsolidation (30, 31) and endocytosis of AMPARs has been demonstrated to be required for the loss of fear response during adaptive reconsolidation of contextual fear (32). In addition, USP46 has been implicated in regulating the GABAergic system (33–36), which also has a crucial role in fear memory formation, reconsolidation, and extinction (37–42). Notably, Ebihara and colleagues reported that Usp46 knockout mice display shortened immobility times in the tail suspension test (36) and long-term memory deficits in the object recognition test (33). They also found out that Usp46 mutant mice were more sensitive to stress and developed impaired maternal behaviors (43). The findings on the possible regulatory role of the USP46 in synaptic plasticity and fear memory processing suggest that USP46 may be an interesting candidate for the development and recovery of fear-related disorders such as PTSD. Although USP46 single nucleotide polymorphism (SNP) has been reported to be associated with major depressive disorder (44) and with depressive temperament (45) in human, no study has been conducted to examine the association of the USP46 gene with PTSD in a clinical sample.
We investigated whether the genetic variants of the USP46 would be associated with chronic PTSD status in Korean male veterans with exposure to combat trauma using a case-control candidate gene association design. Our main hypothesis was that susceptibility to PTSD might be associated with genetic polymorphisms of the USP46.
Materials and Methods
Participants and Procedure
According to the DSM-IV-TR diagnostic criteria (1) for PTSD, 128 subjects with PTSD and 128 (non-PTSD) controls were recruited from a psychiatric outpatient clinic at the Veterans Health Service (VHS) Medical Center. All subjects were of Korean ethnicity and male veterans who had served on active duty during the Vietnam War. Individuals with a history of head trauma, organic brain syndrome including cerebrovascular accidents or dementia, major psychiatric disorders including psychosis or bipolar disorder, or substance dependence other than alcohol and nicotine were excluded. The study was approved by the institutional review board of the VHS Medical Center, South Korea (BOHUN 2016-02-007). All subjects gave their written informed consent before participating in this study.
Measures
For assessing PTSD, we used the Clinician-Administered PTSD Scale (CAPS), a structured clinical interview, which is considered the gold standard for diagnosing PTSD (46, 47). The diagnosis of PTSD was determined by symptom frequency and intensity based on the liberal scoring rule of the CAPS (48). In addition, the Combat Exposure Scale (CES), a self-reporting scale, was administered for measuring the level of wartime traumatic stressors experienced by the combatants (49). The total CES scores were divided into five categories of combat exposure: light (1–8), light–moderate (9–16), moderate (17–24), moderate–heavy (25–32), and heavy (33–41). The Alcohol Use Disorders Identification Test (AUDIT) was also used to assess hazardous and harmful alcohol use (50).
Genotyping
Seven tagging SNPs covering all regions of USP46 (rs346005, rs10034164, rs2244291, rs12646800, rs6554557, rs17675844, and rs10517263) were selected with the criteria of an r2 threshold >0.8 based on a prior genetic association study in a Japanese population in which gene-based approach was used involving all common SNPs (minor allele frequency >5%) (44). Subjects donated a blood sample through venipuncture, and the DNA of each subject was isolated using extraction protocol with QG-810/800 of Quickgene DNA whole blood kit-S after lysate preparation. The genotyping procedures were carried out using single base primer extension assay using the ABI PRISM SNaPShot multiplex kit (ABI, Foster City, CA, USA) according to the manufacturer's recommendations. The forward and reverse primer pairs used for the SNaPshot assay and genetic information for all tested SNPs are presented in Supplementary Table 1. Analysis was performed using Genemapper software (version 3.0; Applied Biosystems) in the DNA Link, Inc. (Seoul, South Korea).
Data Analyses
Demographic and clinical characteristics between subjects with and without PTSD were compared using χ2-test or Student's t-test on the Statistical Package for the Social Sciences version 25.0 (SPSS Inc., Chicago, IL, USA). The Hardy–Weinberg equilibrium for each SNP in the control group was calculated by χ2-test. Statistical power was calculated with Genetic Association Study Power Calculator (https://csg.sph.umich.edu/abecasis/gas_power_calculator). Given the available sample size, the statistical power for detecting a risk allele with an effect size of 1.6 is 88%, depending on 10% minor allele frequency, 40% lifetime disease prevalence of war veterans, and 5% alpha level.
Single-marker analyses were performed using the R package SNPassoc (51). Between-group comparisons of genotype frequency differences for diagnostic status were performed by logistic regression analysis considering different genetic inheritance models. For five SNPs whose genotype frequencies of homozygous with minor alleles were <5%, dominant genetic model was assumed. The outcome variable was analyzed yielding odds ratios (ORs) with 95% confidence intervals (CIs) and p-values. Associations between haplotype distributions and PTSD status were examined using the “haplo.score” function of R package haplo.stats (52). This package computes score statistics to test associations between haplotypes and a trait allowing adjustment for other determinants. This analysis was corrected for multiple testing by applying the simulate = TRUE parameter in haplo.score which gives simulated p-values. These simulated haplotype score statistics are calculated from a permuted re-ordering of the trait (PTSD status) and USP46 polymorphisms. We used 100,000 permutations for all the analyses. Haploblock structure and linkage disequilibrium (LD) patterns obtained from the seven SNPs were constructed using the Haploview ver.4.2 (http://www.broad.mit.edu/mpg/haploview). In further analysis, we conducted linear regression analyses for three clusters (re-experiencing, avoidance, and hyperarousal) of PTSD symptoms considering PTSD as continuous phenotypes. Demographic and clinical characteristics which were different between cases and controls in χ2-test or t-test (p < 0.1) or which have been reported as a risk factor of PTSD in previous studies (53, 54) were selected as potential confounders in analyses. As a result, all analyses were adjusted for demographic factors including age, education year, socio-economic status, and marital status; the five levels of CES, and AUDIT scores (harmful alcohol drinking). In all analyses, p-value of <0.05 was considered as nominally significant (uncorrected p < 0.05). The statistical threshold was corrected using the Bonferroni method for the total number of SNPs (α = 0.05/7 = 0.0071).
Results
The demographic and clinical characteristics of subjects with and without PTSD are presented in Table 1. The groups with and without PTSD were not significantly different in terms of age, education level, marital status, and socioeconomic status. For combat exposure, the distribution of the five CES categories showed a significant difference between PTSD and non-PTSD groups (χ2 = 48.54, df = 4, p < 0.001), with a higher proportion of heavy trauma experience in subjects with PTSD than those without PTSD. For alcohol problem, subjects with PTSD had significantly harmful alcohol consumption based on the AUDIT score, compared to those without PTSD (11.66 ± 10.92 vs. 6.84 ± 7.53, p < 0.001).
The allelic distributions of the seven SNPs in the control group were in accordance with the Hardy–Weinberg equilibrium (Supplementary Table 2). In single-marker analyses under multiple genetic models, only one single-marker (rs2244291) showed a significant association at the nominal significance level of 5% (p = 0.0193 in over-dominant model and p = 0.0497 in Co-dominant model), but the association did not remain significant after stringent correction for multiple comparisons (Table 2). For the other SNPs in the USP46 region, no significant association was found between the groups (Table 2).
The analysis of LD and haplotype block for the USP46 revealed one haplotype block (Figure 1). In haplotype analyses, the permutation test of the seven SNP haplotypes showed no significant difference in the estimated haplotype frequency distributions between both groups (Table 3).
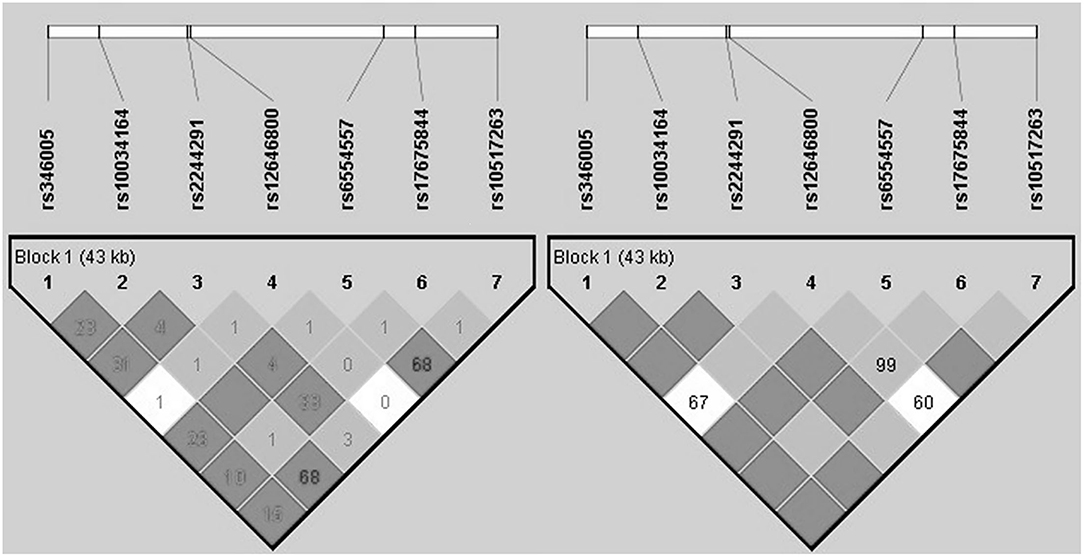
Figure 1. Haploblock structure and linkage disequilibrium for the non-PTSD group from tagging SNPs of USP46. The color scheme is based on r2-value (left) and D prime value (right).
In further analyses considering PTSD as continuous phenotypes, the rs2244291, which was shown to be nominally significantly associated with PTSD status in the main analysis, was associated with the “re-experiencing” cluster of PTSD symptoms (p = 0.014 in over-dominant model and p= 0.041 in co-dominant model) (Table 4).
Discussion
The present study examined a genetic association between the USP46 genetic variants and chronic PTSD among Korean male combat veterans. Single-marker analysis resulted in a nominally significant association only for rs2244291 with PTSD status, although the association did not remain significant after stringent correction for multiple comparisons. In addition, the rs2244291 was found to be associated with the “re-experiencing” cluster of PTSD symptoms. The present finding suggests preliminarily that some underlying genetic vulnerability regarding the ubiquitin-proteasome system such as USP46 may be related to fear memory processes and the development of some PTSD symptoms after trauma exposure.
To the best of our knowledge, the present study is the first to investigate the possible genetic association of the deubiquitinating enzyme in genetic susceptibility for PTSD. There is indirect evidence supporting the role of USP46 in PTSD and fear memory processes. In animal studies, Ebihara and colleagues suggested that Usp46 might be a quantitative trait gene responsible for immobility time reflecting behavioral despair under inescapable stress conditions (34). They showed that Usp46 knockout mice exhibited shorter immobility times in the tail-suspension test, assessing depression-like behavior; reduced sucrose consumption in the sucrose preference test, assessing anhedonia-like symptoms; and lower locomotor activity levels in the open field test, assessing exploratory behavior and anxiety compared to wild type mice (33), which suggests the involvement of Usp46 in stress-related phenotypes. In addition, ubiquitin-mediated protein degradation has been shown as important regulatory process in consolidation and extinction of memory in animal studies (23, 55, 56). Recent in vitro and in vivo findings showed that USP46 regulates glutamatergic receptor ubiquitination and turnover, as well as the strength of synaptic transmission, which suggest the involvement of USP46 in synaptic plasticity and fear memory processes (29, 57). These findings are compatible with our finding that the USP46 rs2244291 is associated with the “re-experiencing (having sudden and intrusive traumatic memories)” cluster, the core PTSD symptom, although fear memory processes themselves were not evaluated in the current study, when considering that re-experiencing of the traumatic event is closely related with abnormalities in fear memory processes including conditioning, reconsolidation and extinction of fear memory (58, 59). Particularly, rs2244291 has been reported to be involved in a haplotype pattern of susceptibility to major depression in a Japanese population by Fukuo et al. (44). Substantial genetic overlap between PTSD and depression has been reported (6, 60), which implies that genes implicated in the pathophysiology of depression are candidates for PTSD. When considering shared genetic components between PTSD and depression, genetic variations of ubiquitin-proteasome system, such as rs2244291, may be involved in regulating dynamic and reversible processes in synaptic plasticity and long-term potentiation after stress exposures in stress-related conditions, rather than in a disease-specific manner.
However, since no significant association with PTSD for the SNPs or the haplotype in the USP46 region was detected after stringent correction for multiple comparisons in this Korean population, the present findings should be interpreted cautiously and preliminarily until confirmed. One possible reason for a weak association is that any one genetic polymorphism may confer a small genetic contribution to PTSD due to multi-factorial polygenic involvement in the pathophysiology of PTSD. The present negative findings in the main analysis should not be interpreted as conclusive for no association because the present sample might be too small for adequate statistical power to detect genetic variants with extremely small effect. Another possibility is that the weak association might be related to functional impact of other potential unmeasured genetic factors, such as the role of rare and structural genetic variations with strong effect. Although the role of rare and structural variation is not known in PTSD (61), rare variants were found to play unique roles in the genetics of complex diseases and research of rare variants require larger sample sizes than common variants to ensure sufficient statistical power (62). Further genetic studies in much larger samples will be helpful in unraveling the genetic contribution of common variants and rare variants to PTSD, its clusters, and broader phenotypes.
The strength of the present genetic association study is that case (trauma-exposed PTSD subjects)—control (trauma-exposed non-PTSD controls) design was applied for a relatively homogenous sample with exposure to similar trauma in a racially uniform population. However, limitations of this study should be noted. First, environmental factors such as early-life trauma were not controlled. Considering possible gene–environment interactions, some environments may have confounding effects that influence chronic PTSD status. Second, although our subjects are likely to comprise a more homogeneous sample with similar age and a single ethnic origin, the present study can only be regarded as a preliminary study in the Korean elderly population. Therefore, it should be replicated in larger sample sets, including populations with diverse ages and different ethnic backgrounds. Third, although we selected the USP46 as a candidate gene based on a priori hypothesis on the possible role of the USP46 in synaptic plasticity and fear memory formation from previous studies, the functions of the present seven tag SNPs are unknown and no evidence of USP46 has been reported from the GWAS studies on PTSD. Fourth, copy number variants or rare variants of USP46 were not examined. In addition, gene expression analysis of the USP46 was not conducted. Finally, psychiatric comorbidities such as depression might affect the present results. Since comorbidity of PTSD and depression could be largely explained by common genetic influences (60), we did not exclude the comorbidities. In the context of common genetic liability among PTSD, anxiety disorders, and depression, further research is required to determine genetic and environmental factors that influence the development of PTSD.
In summary, we investigated the clinical relevance of the genetic factors in the USP46 using a case-control association design in Korean male veterans with or without PTSD after exposure to combat trauma. While the present findings suggest preliminarily that USP46 rs2244291 may potentially be involved in re-experiencing symptoms and PTSD status after exposure to traumatic events, the limited sample size warrants caution for over-interpreting nominally significant genetic findings. Further research in large cohorts is needed to better understand the role of ubiquitin-proteasome system in genetic susceptibility to PTSD.
Data Availability Statement
The raw data supporting the conclusions of this article will be made available by the authors, without undue reservation.
Ethics Statement
The studies involving human participants were reviewed and approved by institutional review board of the VHS Medical Center, South Korea (BOHUN 2016-02-007). The patients/participants provided their written informed consent to participate in this study.
Author Contributions
JK and TK designed the study. TK, JC, and HS collected the data. JS, JK, SK, and HS undertook the statistical analyses and interpreted the findings. JS and JK prepared the manuscript. All authors contributed to, and approved, the final manuscript.
Funding
This work was supported by the National Research Foundation of Korea (NRF) grant funded by the Korea government (NRF-2019R1A2C1084611). The funding source did not give any influences on the study design, data collection, analysis and interpretation of data, the writing of the report, and the decision to submit the paper for publication.
Conflict of Interest
The authors declare that the research was conducted in the absence of any commercial or financial relationships that could be construed as a potential conflict of interest.
Publisher's Note
All claims expressed in this article are solely those of the authors and do not necessarily represent those of their affiliated organizations, or those of the publisher, the editors and the reviewers. Any product that may be evaluated in this article, or claim that may be made by its manufacturer, is not guaranteed or endorsed by the publisher.
Acknowledgments
We would like to thank the Veterans Health Service Medical Center for their support in this study.
Supplementary Material
The Supplementary Material for this article can be found online at: https://www.frontiersin.org/articles/10.3389/fpsyt.2021.663647/full#supplementary-material
Abbreviations
USP46, ubiquitin carboxyl-terminal hydrolase 46; PTSD, post-traumatic stress disorder; USP, ubiquitin specific peptidase; GABA, gamma-aminobutyric acid; AMPA, alpha-amino-3-hydroxy-5-methyl-4-isoxazolepropionic acid; VHS, veterans health service; CAPS, clinician-administered PTSD scale; CES, combat exposure scale; AUDIT, alcohol use disorders identification test; SNPs, single nucleotide polymorphisms; LD, linkage disequilibrium.
References
1. First MB, Spitzer R, L GM, Williams JBW. Structured Clinical Interview for DSM-IV Axis I Disorders, Clinical Version (SCID-CV). Washington, DC: American Psychiatric Press, Inc. (1996).
2. Hines LA, Sundin J, Rona RJ, Wessely S, Fear NT. Posttraumatic stress disorder post Iraq and Afghanistan: prevalence among military subgroups. Can J Psychiatry. (2014) 59:468–79. doi: 10.1177/070674371405900903
3. Gates MA, Holowka DW, Vasterling JJ, Keane TM, Marx BP, Rosen RC. Posttraumatic stress disorder in veterans and military personnel: epidemiology, screening, and case recognition. Psychol Serv. (2012) 9:361–82. doi: 10.1037/a0027649
4. Magruder K, Serpi T, Kimerling R, Kilbourne AM, Collins JF, Cypel Y, et al. Prevalence of posttraumatic stress disorder in Vietnam-era women veterans: the health of Vietnam-era women's study (HealthVIEWS). JAMA Psychiatry. (2015) 72:1127–34. doi: 10.1001/jamapsychiatry.2015.1786
5. True WR, Rice J, Eisen SA, Heath AC, Goldberg J, Lyons MJ, et al. A twin study of genetic and environmental contributions to liability for posttraumatic stress symptoms. Arch Gen Psychiatry. (1993) 50:257–64. doi: 10.1001/archpsyc.1993.01820160019002
6. Sartor CE, Grant JD, Lynskey MT, McCutcheon VV, Waldron M, Statham DJ, et al. Common heritable contributions to low-risk trauma, high-risk trauma, posttraumatic stress disorder, and major depression. Arch Gen Psychiatry. (2012) 69:293–9. doi: 10.1001/archgenpsychiatry.2011.1385
7. Stein MB, Jang KL, Taylor S, Vernon PA, Livesley WJ. Genetic and environmental influences on trauma exposure and posttraumatic stress disorder symptoms: a twin study. Am J Psychiatry. (2002) 159:1675–81. doi: 10.1176/appi.ajp.159.10.1675
8. Voisey J, Young RM, Lawford BR, Morris CP. Progress towards understanding the genetics of posttraumatic stress disorder. J Anxiety Disord. (2014) 28:873–83. doi: 10.1016/j.janxdis.2014.09.014
9. Almli LM, Fani N, Smith AK, Ressler KJ. Genetic approaches to understanding post-traumatic stress disorder. Int J Neuropsychopharmacol. (2014) 17:355–70. doi: 10.1017/S1461145713001090
10. Wilker S, Schneider A, Conrad D, Pfeiffer A, Boeck C, Lingenfelder B, et al. Genetic variation is associated with PTSD risk and aversive memory: evidence from two trauma-exposed African samples and one healthy European sample. Transl Psychiatry. (2018) 8:251. doi: 10.1038/s41398-018-0297-1
11. Banerjee SB, Morrison FG, Ressler KJ. Genetic approaches for the study of PTSD: advances and challenges. Neurosci Lett. (2017) 649:139–46. doi: 10.1016/j.neulet.2017.02.058
12. Voisey J, Swagell CD, Hughes IP, Morris CP, van Daal A, Noble EP, et al. The DRD2 gene 957C>T polymorphism is associated with posttraumatic stress disorder in war veterans. Depress Anxiety. (2009) 26:28–33. doi: 10.1002/da.20517
13. Goçi Uka A, Agani F, Blyta A, Hoxha B, Haxhibeqiri S, Haxhibeqiri V, et al. Role of the allelic variation in the 5-Hydroxytryptamine Receptor 1A (HTR1A) and the Tryptophan Hydroxylase 2 (TPH2) genes in the development of PTSD. Psychiatr Danub. (2019) 31:256–62. doi: 10.24869/psyd.2019.256
14. Wolf EJ, Mitchell KS, Logue MW, Baldwin CT, Reardon AF, Aiello A, et al. The dopamine D3 receptor gene and posttraumatic stress disorder. J Trauma Stress. (2014) 27:379–87. doi: 10.1002/jts.21937
15. Miller MW, Sperbeck E, Robinson ME, Sadeh N, Wolf EJ, Hayes JP, et al. 5-HT2A gene variants moderate the association between PTSD and reduced default mode network connectivity. Front Neurosci. (2016) 10:299. doi: 10.3389/fnins.2016.00299
16. Dong C, Bach SV, Haynes KA, Hegde AN. Proteasome modulates positive and negative translational regulators in long-term synaptic plasticity. J Neurosci. (2014) 34:3171–82. doi: 10.1523/JNEUROSCI.3291-13.2014
17. Li Q, Korte M, Sajikumar S. Ubiquitin-proteasome system inhibition promotes long-term depression and synaptic tagging/capture. Cereb Cortex. (2016) 26:2541–8. doi: 10.1093/cercor/bhv084
18. Cai F, Frey JU, Sanna PP, Behnisch T. Protein degradation by the proteasome is required for synaptic tagging and the heterosynaptic stabilization of hippocampal late-phase long-term potentiation. Neuroscience. (2010) 169:1520–6. doi: 10.1016/j.neuroscience.2010.06.032
19. Kowalski JR, Juo P. The role of deubiquitinating enzymes in synaptic function and nervous system diseases. Neural Plast. (2012) 2012:892749. doi: 10.1155/2012/892749
20. Todi SV, Paulson HL. Balancing act: deubiquitinating enzymes in the nervous system. Trends Neurosci. (2011) 34:370–82. doi: 10.1016/j.tins.2011.05.004
21. Colledge M, Snyder EM, Crozier RA, Soderling JA, Jin Y, Langeberg LK, et al. Ubiquitination regulates PSD-95 degradation and AMPA receptor surface expression. Neuron. (2003) 40:595–607. doi: 10.1016/S0896-6273(03)00687-1
22. Hegde AN, DiAntonio A. Ubiquitin and the synapse. Nat Rev Neurosci. (2002) 3:854–61. doi: 10.1038/nrn961
23. Reis DS, Jarome TJ, Helmstetter FJ. Memory formation for trace fear conditioning requires ubiquitin-proteasome mediated protein degradation in the prefrontal cortex. Front Behav Neurosci. (2013) 7:150. doi: 10.3389/fnbeh.2013.00150
24. Sol Fustiñana M. de la Fuente V, Federman N, Freudenthal R, Romano A. Protein degradation by ubiquitin-proteasome system in formation and labilization of contextual conditioning memory. Learn Mem. (2014) 21:478–87. doi: 10.1101/lm.035998.114
25. Orsi SA, Devulapalli RK, Nelsen JL, McFadden T, Surineni R, Jarome TJ. Distinct subcellular changes in proteasome activity and linkage-specific protein polyubiquitination in the amygdala during the consolidation and reconsolidation of a fear memory. Neurobiol Learn Mem. (2019) 157:1–11. doi: 10.1016/j.nlm.2018.11.012
26. Jarome TJ, Werner CT, Kwapis JL, Helmstetter FJ. Activity dependent protein degradation is critical for the formation and stability of fear memory in the amygdala. PLoS ONE. (2011) 6:e24349. doi: 10.1371/journal.pone.0024349
27. Kowalski JR, Dahlberg CL, Juo P. The deubiquitinating enzyme USP-46 negatively regulates the degradation of glutamate receptors to control their abundance in the ventral nerve cord of Caenorhabditis elegans. J Neurosci. (2011) 31:1341–54. doi: 10.1523/JNEUROSCI.4765-10.2011
28. Hodul M, Dahlberg CL, Juo P. Function of the deubiquitinating enzyme USP46 in the nervous system and its regulation by WD40-repeat proteins. Front Synaptic Neurosci. (2017) 9:16. doi: 10.3389/fnsyn.2017.00016
29. Huo Y, Khatri N, Hou Q, Gilbert J, Wang G, Man HY. The deubiquitinating enzyme USP46 regulates AMPA receptor ubiquitination and trafficking. J Neurochem. (2015) 134:1067–80. doi: 10.1111/jnc.13194
30. Bhattacharya S, Kimble W, Buabeid M, Bhattacharya D, Bloemer J, Alhowail A, et al. Altered AMPA receptor expression plays an important role in inducing bidirectional synaptic plasticity during contextual fear memory reconsolidation. Neurobiol Learn Mem. (2017) 139:98–108. doi: 10.1016/j.nlm.2016.12.013
31. Yamada D, Wada K, Sekiguchi M. Facilitating actions of an AMPA receptor potentiator upon extinction of contextually conditioned fear response in stressed mice. Neurosci Lett. (2011) 488:242–6. doi: 10.1016/j.neulet.2010.11.038
32. Rao-Ruiz P, Rotaru DC, van der Loo RJ, Mansvelder HD, Stiedl O, Smit AB, et al. Retrieval-specific endocytosis of GluA2-AMPARs underlies adaptive reconsolidation of contextual fear. Nat Neurosci. (2011) 14:1302–8. doi: 10.1038/nn.2907
33. Imai S, Kano M, Nonoyama K, Ebihara S. Behavioral characteristics of ubiquitin-specific peptidase 46-deficient mice. PLoS ONE. (2013) 8:e58566. doi: 10.1371/journal.pone.0058566
34. Tomida S, Mamiya T, Sakamaki H, Miura M, Aosaki T, Masuda M, et al. Usp46 is a quantitative trait gene regulating mouse immobile behavior in the tail suspension and forced swimming tests. Nat Genet. (2009) 41:688–95. doi: 10.1038/ng.344
35. Zhang W, Tian QB Li QK, Wang JM, Wang CN, Liu T, et al. Lysine 92 amino acid residue of USP46, a gene associated with 'behavioral despair' in mice, influences the deubiquitinating enzyme activity. PLoS ONE. (2011) 6:e26297. doi: 10.1371/journal.pone.0026297
36. Imai S, Mamiya T, Tsukada A, Sakai Y, Mouri A, Nabeshima T, et al. Ubiquitin-specific peptidase 46 (Usp46) regulates mouse immobile behavior in the tail suspension test through the GABAergic system. PLoS ONE. (2012) 7:e39084. doi: 10.1371/journal.pone.0039084
37. Makkar SR, Zhang SQ, Cranney J. Behavioral and neural analysis of GABA in the acquisition, consolidation, reconsolidation, and extinction of fear memory. Neuropsychopharmacology. (2010) 35:1625–52. doi: 10.1038/npp.2010.53
38. Luft T, Pereira GS, Cammarota M, Izquierdo I. Different time course for the memory facilitating effect of bicuculline in hippocampus, entorhinal cortex, and posterior parietal cortex of rats. Neurobiol Learn Mem. (2004) 82:52–6. doi: 10.1016/j.nlm.2004.03.002
39. Bustos SG, Maldonado H, Molina VA. Midazolam disrupts fear memory reconsolidation. Neuroscience. (2006) 139:831–42. doi: 10.1016/j.neuroscience.2005.12.064
40. Berlau DJ, McGaugh JL. Enhancement of extinction memory consolidation: the role of the noradrenergic and GABAergic systems within the basolateral amygdala. Neurobiol Learn Mem. (2006) 86:123–32. doi: 10.1016/j.nlm.2005.12.008
41. Akirav I, NMDA. Partial agonist reverses blocking of extinction of aversive memory by GABA(A) agonist in the amygdala. Neuropsychopharmacology. (2007) 32:542–50. doi: 10.1038/sj.npp.1301050
42. Bustos SG, Maldonado H, Molina VA. Disruptive effect of midazolam on fear memory reconsolidation: decisive influence of reactivation time span and memory age. Neuropsychopharmacology. (2009) 34:446–57. doi: 10.1038/npp.2008.75
43. Umemura S, Imai S, Mimura A, Fujiwara M, Ebihara S. Impaired maternal behavior in Usp46 mutant mice: a model for trans-generational transmission of maternal care. PLoS ONE. (2015) 10:e0136016. doi: 10.1371/journal.pone.0136016
44. Fukuo Y, Kishi T, Kushima I, Yoshimura R, Okochi T, Kitajima T, et al. Possible association between ubiquitin-specific peptidase 46 gene and major depressive disorders in the Japanese population. J Affect Disord. (2011) 133:150–7. doi: 10.1016/j.jad.2011.04.020
45. Boo YJ, Park CI, Kim HW, Kim SJ, Kang JI. Possible association of the Ubiquitin-Specific Peptidase 46 Gene (USP46) with affective temperamental traits in healthy Korean volunteers. Psychiatry Investig. (2019) 16:87–92. doi: 10.30773/pi.2018.10.02
46. Blake DD, Weathers FW, Nagy LM, Kaloupek DG, Gusman FD, Charney DS, et al. The development of a clinician-administered PTSD scale. J Trauma Stress. (1995) 8:75–90. doi: 10.1002/jts.2490080106
47. Weathers FW, Keane TM, Davidson JR. Clinician-administered PTSD scale: a review of the first ten years of research. Depress Anxiety. (2001) 13:132–56. doi: 10.1002/da.1029
48. Weathers FW, Ruscio AM, Keane TM. Psychometric properties of nine scoring rules for the Clinian-Administered PTSD Scale (CAPS). Psychol Assess. (1999) 11:124–33. doi: 10.1037/1040-3590.11.2.124
49. Keane TM, Fairbank JA, Caddell JM, Zimering RT, Taylor KL, Mora CA. Clinical evaluation of a measure to assess combat exposure. Psychol Assess. (1989) 1:53–5. doi: 10.1037/1040-3590.1.1.53
50. Babor TF, Higgins-Biddle JC, Saunders JB, Monteiro MG. The Alcohol Use Disorders Identification Test: Guidelines for Use in Primary Care. Geneva: World Health Organization (2001).
51. Gonzalez JR, Armengol L, Sole X, Guino E, Mercader JM, Estivill X, et al. SNPassoc: an R package to perform whole genome association studies. Bioinformatics. (2007) 23:644–5. doi: 10.1093/bioinformatics/btm025
52. Schaid DJ, Rowland CM, Tines DE, Jacobson RM, Poland GA. Score tests for association between traits and haplotypes when linkage phase is ambiguous. Am J Hum Genet. (2002) 70:425–34. doi: 10.1086/338688
53. Pejuskovic B, Lecic-Tosevski D, Toskovic O. Longitudinal study of posttraumatic stress disorder in the community: risk and recovery factors. J Nerv Ment Dis. (2017) 205:77–82. doi: 10.1097/NMD.0000000000000624
54. DiGrande L, Perrin MA, Thorpe LE, Thalji L, Murphy J, Wu D, et al. Posttraumatic stress symptoms, PTSD, and risk factors among lower Manhattan residents 2-3 years after the September 11, 2001 terrorist attacks. J Trauma Stress. (2008) 21:264–73. doi: 10.1002/jts.20345
55. Felsenberg J, Dombrowski V, Eisenhardt D A. role of protein degradation in memory consolidation after initial learning and extinction learning in the honeybee (Apis mellifera). Learn Mem. (2012) 19:470–7. doi: 10.1101/lm.026245.112
56. Furini CR, Myskiw Jde C, Schmidt BE, Zinn CG, Peixoto PB, Pereira LD, et al. The relationship between protein synthesis and protein degradation in object recognition memory. Behav Brain Res. (2015) 294:17–24. doi: 10.1016/j.bbr.2015.07.038
57. Ribeiro FC, Santos LE, Ferreira ST. USP46: a new piece of the memory puzzle? J Neurochem. (2015) 134:979–81. doi: 10.1111/jnc.13227
58. Wessa M, Flor H. Failure of extinction of fear responses in posttraumatic stress disorder: evidence from second-order conditioning. Am J Psychiatry. (2007) 164:1684–92. doi: 10.1176/appi.ajp.2007.07030525
59. Orr SP, Metzger LJ, Lasko NB, Macklin ML, Peri T, Pitman RK, et al. De novo conditioning in trauma-exposed individuals with and without posttraumatic stress disorder. J Abnorm Psychol. (2000) 109:290–8. doi: 10.1037/0021-843X.109.2.290
60. Koenen KC, Fu QJ, Ertel K, Lyons MJ, Eisen SA, True WR, et al. Common genetic liability to major depression and posttraumatic stress disorder in men. J Affect Disord. (2008) 105:109–15. doi: 10.1016/j.jad.2007.04.021
61. Smoller JW. The genetics of stress-related disorders: PTSD, depression, and anxiety disorders. Neuropsychopharmacology. (2016) 41:297–319. doi: 10.1038/npp.2015.266
Keywords: post-traumatic stress disorder, ubiquitin proteasome system, ubiquitin specific peptidase, USP46, genetic association study
Citation: Seo JH, Kim TY, Kim SJ, Choi JH, So HS and Kang JI (2021) Possible Association of Polymorphisms in Ubiquitin Specific Peptidase 46 Gene With Post-traumatic Stress Disorder. Front. Psychiatry 12:663647. doi: 10.3389/fpsyt.2021.663647
Received: 22 February 2021; Accepted: 14 July 2021;
Published: 11 August 2021.
Edited by:
Gianluca Serafini, San Martino Hospital (IRCCS), ItalyReviewed by:
Yogesh Dwivedi, University of Alabama at Birmingham, United StatesLaiana Quagliato, Federal University of Rio de Janeiro, Brazil
Copyright © 2021 Seo, Kim, Kim, Choi, So and Kang. This is an open-access article distributed under the terms of the Creative Commons Attribution License (CC BY). The use, distribution or reproduction in other forums is permitted, provided the original author(s) and the copyright owner(s) are credited and that the original publication in this journal is cited, in accordance with accepted academic practice. No use, distribution or reproduction is permitted which does not comply with these terms.
*Correspondence: Jee In Kang, jeeinkang@yuhs.ac