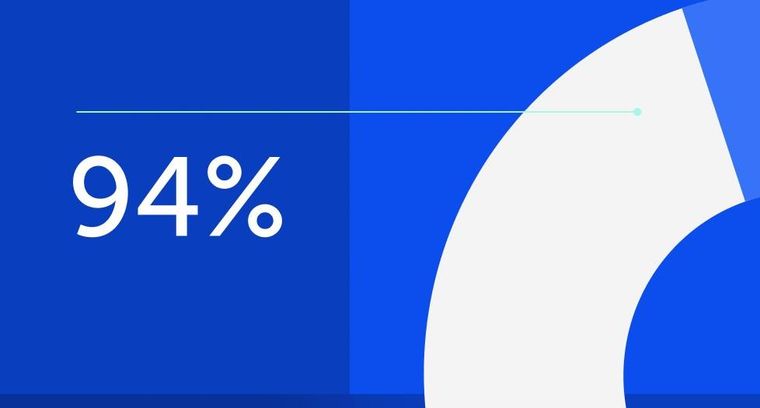
94% of researchers rate our articles as excellent or good
Learn more about the work of our research integrity team to safeguard the quality of each article we publish.
Find out more
MINI REVIEW article
Front. Psychiatry, 11 May 2021
Sec. Mood Disorders
Volume 12 - 2021 | https://doi.org/10.3389/fpsyt.2021.659052
This article is part of the Research TopicMechanisms Underlying Mood DisordersView all 12 articles
The anesthetic drug ketamine has been successfully repurposed as an antidepressant in human subjects. This represents a breakthrough for clinical psychopharmacology, because unlike monoaminergic antidepressants, ketamine has rapid onset, including in Major Depressive Disorder (MDD) that is resistant to conventional pharmacotherapy. This rapid therapeutic onset suggests a unique mechanism of action, which continues to be investigated in reverse translational studies in rodents. A large fraction of rodent and human studies of ketamine have focused on the effects of only a single administration of ketamine, which presents a problem because MDD is typically a persistent illness that may require ongoing treatment with this drug to prevent relapse. Here we review behavioral studies in rodents that used repeated dosing of ketamine in the forced swim test (FST), with an eye toward eventual mechanistic studies. A subset of these studies carried out additional experiments with only a single injection of ketamine for comparison, and several studies used chronic psychosocial stress, where stress is a known causative factor in some cases of MDD. We find that repeated ketamine can in some cases paradoxically produce increases in immobility in the FST, especially at high doses such as 50 or 100 mg/kg. Several studies however provide evidence that repeated dosing is more effective than a single dose at decreasing immobility, including behavioral effects that last longer. Collectively, this growing literature suggests that repeated dosing of ketamine has prominent depression-related effects in rodents, and further investigation may help optimize the use of this drug in humans experiencing MDD.
In the last two decades, the anesthetic drug (R,S)-ketamine (this racemic mixture is referred to as “ketamine” hereafter) has been successfully repurposed as an antidepressant in humans suffering from Major Depressive Disorder (MDD) (1–4). This has been a significant advance in clinical psychopharmacology because, unlike commonly used monoaminergic antidepressants such as selective serotonin reuptake inhibitors (SSRIs), ketamine has rapidly acting antidepressant properties, including in treatment resistant MDD (2, 4, 5). Monoaminergic antidepressants are also not therapeutically effective in all individuals, and can have significant side effects, creating demand for novel agents such as ketamine (6). The initial repurposing of ketamine was based on a foundation of rodent behavioral studies largely conducted in the 1990's, using a variety of compounds that, like ketamine, block glutamatergic NMDA receptors (7–11), although the detailed molecular mechanisms of ketamine (a non-competitive NMDA receptor antagonist) began being elucidated later (8, 12). Thus, a combination of preclinical and clinical studies, a large number of which continue to be carried out, has established that use of ketamine is an important pharmacological option for clinicians in the treatment of MDD.
Rodent neuroscience models offer an excellent opportunity to elucidate the neural network mechanisms of new or repurposed medications such as ketamine. This in turn would enable us to understand limbic circuitry itself in new ways. Many studies have focused on this and an ongoing debate, particularly in the rodent preclinical literature, concerns the precise molecular and circuit-based mechanisms through which ketamine produces its favorable effects (13–16). This ongoing debate includes investigating the efficacy of the R enantiomer of ketamine in a rat learned helplessness model (15), and also potentially dose-dependent sex differences in response to ketamine (16). It is not clear at this time whether the molecular or circuit-based mechanisms of single dose ketamine differ just in magnitude or instead qualitatively from those of repeated administration, but qualitative differences are possible (17).
To date most rodent studies have used only single doses of ketamine, whereas in clinical settings repeated dosing is increasingly used to treat MDD (18–20). These rodent studies typically administer a single, systemic injection of ketamine, and then monitor behavior in the acute period afterward, or 24 h or more later (14). It is important to measure behavior beyond the acute time window, such as at the 24 h point, since ketamine has acute dissociative-like properties that can result in hyperlocomotion (21, 22) and be confounded with immobility-related behavior in the FST. For these reasons, when studying any drug in the FST it is important to also measure locomotor activity in an assay such as the open field test, to gauge whether changes observed in the FST are confounded with generalized hyperactivity (or hypoactivity). The topic of dosing frequency of ketamine is of particular importance, since in clinical settings multiple doses are often given over the course of weeks to months in individuals with MDD (18–20, 23) to prevent relapse (19, 20). While understanding the effects of repeated dosing of ketamine is important clinically, this topic is only beginning to be addressed preclinically in rodent settings where neural mechanisms can be deciphered. We can then ask, why does ketamine initially produce favorable effects? And why do these favorable effects then typically fade? These questions can only be answered if we have effective and reliable behavioral models in rodents to allow us to subsequently delve deeper into neural mechanisms.
In this brief review, we investigate the behavioral effects of repeated dosing of ketamine in rodents, focusing on the widely used behavioral assay of antidepressant compounds, the forced swim test (FST) (24, 25). We focus on the FST since at this time there are only a limited number of studies on the behavioral effects of repeated dose ketamine and those using the FST are the most numerous. That said, this appears to be a growing literature, which we summarize below. Importantly, some of these studies use chronic psychosocial stress, such as chronic unpredictable stress (CUS) [also known as unpredictable chronic mild stress (UCMS)], to determine how this affects treatment with ketamine. This is crucial since chronic stress often triggers MDD (26, 27) and stress models in rodents are one of our primary means for studying mood-related brain circuits (28). The single injection ketamine literature in the FST provides conflicting evidence as to whether prior chronic stress exposure modulates the behavioral response to this drug, with some studies showing stress-sensitivity (28, 29) and a number of others not (16, 30). This topic remains to be adequately addressed in future studies that use repeated ketamine administration.
We should point out that in recent years, the FST has been increasingly criticized as not being a direct measure of depression-related behavior (31). For example, it has been suggested that immobility in the FST represents a passive coping strategy that is a behavioral adaptation to an inescapable acute stressor, rather than modeling depression-like behavior (32–34), or represents an extinction-like response (35). In this scenario, the FST may be more of a screening test for putative antidepressant compounds in response to an acute stressor, although questions remain regarding the utility of the FST for predicting response to non-monoaminergic, fast-acting glutamatergic agents (33, 35). For all of these reasons, future rodent studies aimed at investigating the putative antidepressant properties of ketamine (or other drugs) should consider including a battery of other behavioral tests [sucrose preference, novelty suppressed feeding, splash; as well as open field (to test generalized hyperactivity)] in addition to the FST. Repeated ketamine administration is only beginning to be investigated in these other tests (36–38).
As recently as July 6, 2020, we conducted a literature search of PubMed using the following terms: ketamine + repeated/twice/subchronic/chronic + “forced swim”/“forced swimming.” We identified 24 relevant studies that used repeated administration of ketamine to mice or rats. These FST data are summarized in Table 1, and comprise immobility as a behavioral readout. In the studies described in Table 1, ketamine was injected from 2 to 30 times (and in one study was orally administered), over a period of up to 7 weeks, at a dose that varied from 0.1 to 100 mg/kg. The time delay between the final administration of ketamine and when the FST was conducted varied greatly, from 30 min to 2 months, which should be considered when interpreting acute versus sustained effects of this drug. A subset of these publications also used a single injection of ketamine for comparison with the multiple injections, and these data were also included in Table 1. During some of the experiments, chronic unpredictable stress (CUS) or unpredictable chronic mild stress (UCMS) was used, prior to the FST. The table consists of mice and rats of both sexes and various strains, ranging in age from adolescents to adults. The studies primarily used racemic (R,S)-ketamine, although one study (42) as noted in the table only used the (S)-ketamine enantiomer.
While the literature on repeated ketamine administration in the FST is somewhat limited at this time, we summarize these findings in Table 1. This table may already yield several principles or themes. One principle is that when the effects of ketamine are acutely measured in the FST (within an hour of final administration), whether after only a single injection or multiple injections, this drug tends to decrease immobility [for example: (41, 53)]. However, as noted above, this time window comprises the acutely intoxicating or dissociative-like effects of ketamine, and one remarkable aspect of this drug is its capacity to affect brain and behavior for hours or days after it has been largely systemically eliminated in vivo (in about 4 h). Therefore, we focus on the time period in the FST beginning 24 h or later after the last drug administration (14). While a number of the studies listed in Table 1 investigated acute effects of ketamine, many examined longer time delays after drug injection.
Inspection of Table 1 also reveals that not many studies of repeated ketamine have used chronic stress, even though as described earlier chronic stress or trauma is an etiological factor in human MDD (26, 27). While we identified only four studies (all carried out in rats) that used chronic stress in Table 1, in each of the experiments carried out there was a decrease in immobility, after either a single injection or multiple injections, that was statistically significant in most cases and lasted up to 2 months for repeated injections (36, 49, 50, 58). These daily unpredictable stress procedures varied in length from 15 (36) to 42 days (50, 58), and used either one (58) or two (36, 49, 50) stressors per day, such as cage tilting or placing wet bedding in the cage. These behavioral findings after chronic stress was administered are consistent with experiments from our laboratory that used C57BL/6J mice, and found that chronic stress was either necessary for (28) or amplified (29) the increases in swimming or decreases in immobility produced by a single injection of ketamine. Future studies should further investigate how repeated ketamine modulates the behavioral and neural effects of chronic stress.
In comparing mice with rats in Table 1: while studies often used different parameters such as varied dosing and none of the mouse studies used chronic stress, we can conclude that repeated ketamine is capable of producing either decreases or increases in immobility in the FST in both species, under various experimental conditions, with higher doses such 50 or 100 mg/kg favoring immobility. Regarding different strains of mice or rats: the three studies that used CD-1 and CF-1 mice only found decreases in immobility (40–42), whereas studies of other albino mouse strains and C57BL/6J mice showed either increases or decreases in immobility depending on the study parameters and strain used (39, 44–48). Likewise, Sprague-Dawley or WKY rats were only observed to have decreases in immobility (36, 49–51, 59, 60). One of these studies directly compared female Wistar and WKY rats, and found that the latter strain was more responsive to the immobility decreasing effects of this drug (59). As mentioned above, Neves et al. (42) was the only study in Table 1 that used the enantiomer, (S)-ketamine. It is noteworthy that this study showed an advantage of repeated over single dose ketamine at 30 mg/kg administered daily for 2 weeks, although the favorable effect of repeated drug did not persist beyond 2 days (42).
These preliminary observations on species and strain should be tested in further studies in a controlled manner. It is not clear whether these differences in the FST reflect differential response to ketamine or qualitatively different engagement by the strains with the FST itself. For example, in one strain, higher mobility may indicate more motivation or exploratory drive, but in another it may indicate depressive-like behavior that resembles psychomotor agitation—-which are on opposing ends of the typical spectrum of neuropsychiatric health states. Some of our own recent data suggest that male C57BL/6J mice, for example, differ in FST behavior from other common inbred and outbred strains, perhaps showing greater sensitivity to the immobility enhancing effects of one injection of 10 mg/kg ketamine in unstressed animals (14). We have also recently demonstrated that female C57BL/6J mice can be more sensitive than males to the favorable effects of a single dose of 30 mg/kg ketamine in the FST, an effect that is amplified by prior exposure to chronic stress (29). Finally, there is growing evidence that, in addition to factors such as animal strain, sex, and age, the sex of the experimenter may play a prominent role in the outcomes of behavioral experiments such as the FST (61, 62). These factors need to be considered in future studies that investigate repeated dosing of ketamine and other drugs.
Most of the experiments in Table 1 used male rather than female mice and rats. Inspection of the table reveals that both male and female mice and rats are capable of exhibiting either increases or decreases in immobility in response to repeated ketamine under different experimental conditions. For example, in females repeated doses as low as 10 mg/kg can produce increases in immobility (39, 55). There appears to be only a limited literature directly comparing males and females after repeated dosing of ketamine in the FST, although studies such as Thelen et al. (39) and Krimmel et al. (41) suggest that female mice may be less sensitive than males to the immobility decreasing effects of repeated ketamine. Several studies in the single injection ketamine literature suggest that female mice or rats show decreased immobility after lower doses (such as 10 mg/kg or less), but the duration of action may be longer in males, although our recent study may be an exception to this latter point (29, 63–65).
Is there an optimal dose for repeated ketamine that emerges from these studies? High doses, such as 50 or 100 mg/kg, can be associated with increases in immobility in Table 1 both acutely and up to 10 days later (44, 46, 54), although 50 mg/kg is still a subanesthetic dose. While we have previously suggested (14) that the field has converged on using 10 mg/kg as a standard dose in single injection rodent FST experiments (16), our own data have suggested that 30 mg/kg is more effective at reducing immobility in male C57BL/6J mice (28). A repeated dose as low as 10 mg/kg in Table 1 has actually been associated with increases in immobility in mice (38, 39, 55). The field has not converged on a single repeated dose found to be generally efficacious in all labs and contradictory results are frequent. This will encumber efforts to consistently study these phenomena. The variety of results may stem from strain differences (including between inbred versions of the same strain from different vendors or laboratories), handling differences (both by laboratory staff and animal housing staff), environmental differences, or differences in the testing and quantification itself. These problems are not new to behavioral pharmacology, but ketamine research has not avoided them.
Lastly and perhaps most importantly, are multiple administrations of ketamine more effective in the FST than a single administration of this drug, based on the limited data set described in Table 1? As stated above, chronic treatment with ketamine can increase immobility in some studies, especially at moderate to high doses (30–100 mg/kg). Whether repeated use of lower doses is more effective at reducing immobility than a single administration of that same dose or a different one, seems to be unclear at this time. Some studies that used both single and repeated injections show an advantage for chronic dosing over a single dose (40, 42, 58), whereas others show the opposite pattern (47, 54) or no clear advantage for either dosing regimen (41). The Chindo et al. (54) and Hou et al. (47) studies found immobility increasing effects of repeated ketamine at relatively high doses of this drug (30–100 mg/kg), which may suggest that repeated dosing can be more favorable than a single dose if a sufficiently low dose (such as 10 mg/kg or lower) is used repeatedly, or perhaps if there is a longer interval between administrations. Beyond there being some degree of difference in dose used in these above five studies, it is difficult to draw further conclusions from them on the efficacy of repeated dosing due to different experimental parameters used, such as (S)-ketamine (42) or chronic stress (58). In general, the scarcity of studies reviewed here that have used repeated ketamine in the FST precludes definitive conclusions on the potential interactions between variables such as strain, sex, stress, and dose. To better compare a single dose with repeated dosing, future studies should keep all other experimental variables the same, including the time lag between the last (or only) dose of ketamine and the start of the FST or other behavioral tests [sucrose preference, novelty suppressed feeding, splash; as well as open field (to test generalized hyperactivity)]. It should be noted that in a recent study using rats selectively bred for depressive-like behavior, repeated ketamine (10 mg/kg/day for 7 consecutive days) did not produce favorable brain network topological effects relative to vehicle or a single ketamine injection, calling into question the general approach of using repeated ketamine in this animal model (17).
A related topic is whether the immobility decreasing effects of repeated ketamine last longer than a single dose. For example, studies such as Clarke et al. (40) and Neves et al. (42), which directly compare in mice a single injection vs. multiple injections at the same dose, suggest that the immobility decreasing effects of repeated ketamine may last longer than those of one injection. Another point is that repeated dosing regimens are capable of producing a decrease in immobility when ketamine is given at different fixed intervals, including less frequently than once a day (40, 41, 49), exactly once a day (39, 42, 49, 50, 52, 53, 58–60), or twice a day (36). In Sprague-Dawley rats weekly administration of ketamine, for 5 weeks at 20 or 50 mg/kg, has previously been shown to induce locomotor sensitization (66). All of the experiments in Table 1 that repeatedly administered ketamine used a fixed interval. It would also be informative to study this drug when given at a variable interval. One possibility is that a variable interval may be less likely to produce mood cycling or therapeutic habituation, since drug administration would not occur at a fixed, repeating interval.
Future reverse translational studies of ketamine in rodents may benefit from investigating dosing regimens, such as three times a week for 2 weeks and related protocols, that have been used effectively in human MDD studies (1, 19, 67, 68). Another consideration in future rodent studies, as well as clinical trials, would be to test whether monoaminergic antidepressants can amplify or further sustain the antidepressant properties of ketamine (69), including the combination of esketamine with SSRIs or SNRIs in rodents or treatment resistant human subjects (70). Likewise, if treatment with a monoaminergic antidepressant is initiated but only results in a weak response for a given individual, perhaps ketamine can then be added to amplify the response. It has already been demonstrated in MDD that repeated intranasal esketamine plus a conventional antidepressant can be more effective than an antidepressant alone (71). Several studies in the rodent literature also suggest that SSRIs modulate the behavioral effects of ketamine. For example, chronically administered citalopram can hasten and sustain the anti-immobility effect of repeated ketamine (10 mg/kg once every 7 days for 3 weeks) (49), although another study that gave daily ketamine for 2 weeks at a higher dose (30 mg/kg/day) found no effect of a single dose of fluoxetine (72). A study that reported an increase in immobility with 30 mg/kg/day ketamine for 5 days showed that a single dose of paroxetine could attenuate this effect (54). One or two injections of sertraline can also counteract hyperlocomotion induced by 10 consecutive days of 15 mg/kg ketamine (73). Combining repeated ketamine with conventional monoaminergic antidepressants is a promising line of inquiry that should be investigated further.
Another approach has been suggested for prolonging the antidepressant properties of a single administration of ketamine: using (R)-ketamine instead of the racemic mixture of this drug or (S)-ketamine. A number of rodent studies that used a variety of depression-related tests including the FST, have suggested that a single administration of (R)-ketamine has greater therapeutic potency and more sustained effects than (S)-ketamine (74–76). These studies also suggest that (R)-ketamine has fewer side effects, such as dissociative-like properties and hyperlocomotion, than (S)-ketamine. A body of repeated dosing studies in rodents also suggests that (R)-ketamine has more favorable effects on: bone mineral density in ovariectomized mice (77), MPTP-induced dopaminergic neurotoxicity (78), and phencyclidine-induced cognitive deficits (79).
Based on our literature search, we find that administering ketamine multiple times has prominent effects in the rodent FST. Perhaps most importantly, several studies that directly compared single with multiple injections of ketamine found evidence for greater efficacy at decreasing immobility, that is possibly of longer duration, with repeated drug administration, especially when lower doses (10 mg/kg or lower) are used. Further, repeated injections of this drug are capable of producing both increases in immobility (especially at higher doses such as 30–100 mg/kg) and decreases, both acutely and in a more sustained fashion. Within the 1st h after administration, ketamine more reliably reduces immobility, but this may be confounded with dissociative-related hyperactivity. In the few studies that used chronic psychosocial stress, either single or repeated ketamine tended to reduce immobility. Both mice and rats (including males and females) are capable of exhibiting either increases or decreases in immobility after repeated ketamine (depending on experimental parameters such as dose or administration interval), and there is some evidence that this could differ by strain. Two studies suggest that female mice are less sensitive than males to the favorable effects of this drug in the FST, which is an important topic with respect to higher rates of MDD in women. Future studies should further investigate the optimal dosing interval (and dose) of repeated ketamine treatment in the rodent FST and related tests. The potential sex differences in response to ketamine have been particularly understudied at this time, and we are also only beginning to appreciate whether certain strains such as C57BL/6J mice are better than others for modeling depression-related behavior and its response to ketamine (14). These future studies should be conducted with an eye toward maximizing the sustained antidepressant effects of this unique pharmacological agent in the treatment of MDD in human subjects.
RW, PF, and BW conceived of this review, wrote, and edited the manuscript. RW performed the literature search and constructed the table, with assistance from PF. All authors contributed to the article and approved the submitted version.
BW: Collaboration Grant from The Kavli Neuroscience Innovators at University of Michigan, Neuroscience Fellows at the University of Michigan, The Taubman Emerging Scholars Award at the University of Michigan, The Pritzker Neuropsychiatric Disorders Research Consortium. PF: University of Michigan Depression Center STAR Award.
The authors declare that the research was conducted in the absence of any commercial or financial relationships that could be construed as a potential conflict of interest.
1. Aan Het Rot M. Safety and efficacy of repeated-dose intravenous ketamine for treatment-resistant depression. Biol Psychiatry. (2010) 67:139–45. doi: 10.1016/j.biopsych.2009.08.038
2. Berman RM, Cappiello A, Anand A, Oren DA, Heninger GR, Charney DS, et al. Antidepressant effects of ketamine in depressed patients. Biol Psychiatry. (2000) 47:351–54. doi: 10.1016/S0006-3223(99)00230-9
3. Krystal JH, Abdallah CG, Sanacora G, Charney DS, Duman RS. Ketamine: a paradigm shift for depression research and treatment. Neuron. (2019) 101:774–8. doi: 10.1016/j.neuron.2019.02.005
4. Zarate CA, Singh JB, Carlson PJ, Brutsche NE, Ameli R, Luckenbaugh DA, et al. A randomized trial of an N-methyl-D-aspartate antagonist in treatment-resistant major depression. Arch Gen Psychiatry. (2006) 63:856–64. doi: 10.1001/archpsyc.63.8.856
5. Duman RS. Ketamine and rapid-acting antidepressants: a new era in the battle against depression and suicide. F1000Research. (2018) 7:F1000 Faculty Rev−659. doi: 10.12688/f1000research.14344.1
6. Ruhe HG, Huyser J, Swinkels JA, Schene AH. Switching antidepressants after a first selective serotonin reuptake inhibitor in major depressive disorder: a systematic review. J Clin Psychiatry. (2006) 67:1836–55. doi: 10.4088/JCP.v67n1203
7. Layer RT, Popik P, Olds T, Skolnick P. Antidepressant-like actions of the polyamine site NMDA antagonist, eliprodil (SL-82.0715). Pharmacol Biochem Behav. (1995) 52:621–7. doi: 10.1016/0091-3057(95)00155-P
8. Maeng S, Zarate CA, Du J, Schloesser RJ, McCammon J, Chen G, et al. Cellular mechanisms underlying the antidepressant effects of ketamine: role of α-Amino-3-Hydroxy-5-Methylisoxazole-4-Propionic acid receptors. Biol Psychiatry. (2008) 63:349–52. doi: 10.1016/j.biopsych.2007.05.028
9. Meloni D, Carla Gambarana I, Graziella Montis MDE, Dal Pra P, Taddei I, Tagliamonte A, et al. Dizocilpine antagonizes the effect of chronic imipramine on learned helplessness in rats. Pharmacol Biochem Behav. (1993) 46:423–6. doi: 10.1016/0091-3057(93)90374-3
10. Papp M, Moryl E. Antidepressant-like effects of 1-aminocyclopropanecarboxylic acid and D-cycloserine in an animal model of depression. Eur J Pharmacol. (1996) 316:145–51. doi: 10.1016/S0014-2999(96)00675-9
11. Trullas R, Skolnick P. Functional antagonists at the NMDA receptor complex exhibit antidepressant actions. Eur J Pharmacol. (1990) 185:1–10. doi: 10.1016/0014-2999(90)90204-J
12. Li N, Lee B, Liu RJ, Banasr M, Dwyer JM, Iwata M, et al. mTOR-dependent synapse formation underlies the rapid antidepressant effects of NMDA antagonists. Science. (2010) 329:959–64. doi: 10.1126/science.1190287
13. Autry AE, Adachi M, Nosyreva E, Na ES, Los MF, Cheng PF, et al. NMDA receptor blockade at rest triggers rapid behavioural antidepressant responses. Nature. (2011) 475:91–5. doi: 10.1038/nature10130
14. Polis AJ, Fitzgerald PJ, Hale PJ, Watson BO. Rodent ketamine depression-related research: Finding patterns in a literature of variability. Behav Brain Res. (2019) 376:112153. doi: 10.1016/j.bbr.2019.112153
15. Shirayama Y, Hashimoto K. Lack of antidepressant effects of (2R,6R)-hydroxynorketamine in a rat learned helplessness model: comparison with (R)-ketamine. Int J Neuropsychopharmacol. (2018) 21:84–8. doi: 10.1093/ijnp/pyx108
16. Zanos P, Moaddel R, Morris PJ, Georgiou P, Fischell J, Elmer GI, et al. NMDAR inhibition-independent antidepressant actions of ketamine metabolites. Nature. (2016) 533:481–6. doi: 10.1038/nature17998
17. Gass N, Becker R, Reinwald J, Cosa-Linan A, Sack M, Weber-Fahr W, et al. The influence of ketamine's repeated treatment on brain topology does not suggest an antidepressant efficacy. Transl Psychiatry. (2020) 10:56. doi: 10.1038/s41398-020-0727-8
18. Daly EJ, Singh JB, Fedgchin M, Cooper K, Lim P, Shelton RC, et al. Efficacy and safety of intranasal esketamine adjunctive to oral antidepressant therapy in treatment-resistant depression: a randomized clinical trial. JAMA Psychiatry. (2018) 75:139–48. doi: 10.1001/jamapsychiatry.2017.3739
19. Murrough JW, Perez AM, Pillemer S, Stern J, Parides MK, Aan Het Rot M, et al. Rapid and longer-term antidepressant effects of repeated ketamine infusions in treatment-resistant major depression. Biol Psychiatry. (2013) 74:250–6. doi: 10.1016/j.biopsych.2012.06.022
20. Wilkinson ST, Katz RB, Toprak M, Webler R, Ostroff RB, Sanacora G. Acute and longer-term outcomes using ketamine as a clinical treatment at the yale psychiatric hospital. J Clin Psychiatry. (2018) 79:1–16. doi: 10.4088/JCP.17m11731
21. McDougall SA, Park GI, Ramirez GI, Gomez V, Adame BC, Crawford CA. Sex-dependent changes in ketamine-induced locomotor activity and ketamine pharmacokinetics in preweanling, adolescent, adult rats. Eur Neuropsychopharmacol. (2019) 29:740–55. doi: 10.1016/j.euroneuro.2019.03.013
22. Wilson C, Cone K, Kercher M, Hibbitts J, Fischer J, Van Lake A, et al. Naloxone increases ketamine-induced hyperactivity in the open field in female rats. Pharmacol Biochem Behav. (2005) 81:530–4. doi: 10.1016/j.pbb.2005.03.018
23. Singh JB, Fedgchin M, Daly E, Xi L, Melman C, De Bruecker G, et al. Intravenous esketamine in adult treatment-resistant depression: a double-blind, double-randomization, placebo-controlled study. Biol Psychiatry. (2016) 80:424–31. doi: 10.1016/j.biopsych.2015.10.018
24. Porsolt RD, Bertin A, Jalfre M. Behavioral despair in mice: a primary screening test for antidepressants. Arch Int Pharmacodyn Ther. (1977) 229:327–36.
25. Slattery DA, Cryan JF. Using the rat forced swim test to assess antidepressant-like activity in rodents. Nat Protocols. (2012) 7:1009–14. doi: 10.1038/nprot.2012.044
26. Bonde JP, Utzon-Frank N, Bertelsen M, Borritz M, Eller NH, Nordentoft M, et al. Risk of depressive disorder following disasters and military deployment: systematic review with meta-analysis. Br J Psychiatry. (2016) 208:330–6. doi: 10.1192/bjp.bp.114.157859
27. Hosang GM, Shiles C, Tansey KE, McGuffin P, Uher R. Interaction between stress and the BDNF Val66Met polymorphism in depression: a systematic review and meta-analysis. BMC Med. (2014) 12:7. doi: 10.1186/1741-7015-12-7
28. Fitzgerald PJ, Yen JY, Watson BO. Stress-sensitive antidepressant-like effects of ketamine in the mouse forced swim test. PLoS ONE. (2019) 14:e0215554. doi: 10.1371/journal.pone.0215554
29. Fitzgerald PJ, Kounelis-Wuillaume SK, Gheidi A, Morrow JD, Spencer-Segal JL, Watson BO. Sex- and stress-dependent effects of a single injection of ketamine on open field and forced swim behavior. Stress. (2021) 1:1–9. doi: 10.1080/10253890.2021.1871600
30. Pham TH, Defaix C, Xu X, Deng SX, Fabresse N, Alvarez JC, et al. Common neurotransmission recruited in (R,S)-ketamine and (2R,6R)-hydroxynorketamine–induced sustained antidepressant-like effects. Biol Psychiatry. (2018) 84:e3–6. doi: 10.1016/j.biopsych.2017.10.020
31. Reardon S. Depression researchers rethink popular mouse swim tests. Nature. (2019) 571:456–7. doi: 10.1038/d41586-019-02133-2
32. Commons KG, Cholanians AB, Babb JA, Ehlinger DG. The rodent forced swim test measures stress-coping strategy, not depression-like behavior. ACS Chem Neurosci. (2017) 8:955–60. doi: 10.1021/acschemneuro.7b00042
33. Molendijk ML, de Kloet ER. Immobility in the forced swim test is adaptive and does not reflect depression. Psychoneuroendocrinology. (2015) 62:389–91. doi: 10.1016/j.psyneuen.2015.08.028
34. Molendijk ML, de Kloet ER. Coping with the forced swim stressor: current state-of-the-art. Behav Brain Res. (2019) 364:1–10. doi: 10.1016/j.bbr.2019.02.005
35. Campus P, Colelli V, Orsini C, Sarra D, Cabib S. Evidence for the involvement of extinction-associated inhibitory learning in the forced swimming test. Behav Brain Res. (2015) 278:348–55. doi: 10.1016/j.bbr.2014.10.009
36. Parise EM, Alcantara LF, Warren BL, Wright KN, Hadad R, Sial OK, et al. Repeated ketamine exposure induces an enduring resilient phenotype in adolescent and adult rats. Biol Psychiatry. (2013) 74:750–9. doi: 10.1016/j.biopsych.2013.04.027
37. Schumacher A, Sivanandan B, Tolledo EC, Woldegabriel J, Ito R. Different dosing regimens of repeated ketamine administration have opposite effects on novelty processing in rats. Prog Neuro-Psychopharmacol Biol Psychiatry. (2016) 69:1–10. doi: 10.1016/j.pnpbp.2016.03.007
38. Suárez-Santiago JE, Briones-Aranda A, Espinosa-Raya J, Picazo O. Agonist E-6837 and antagonist SB-271046 of 5-HT 6 receptors both reverse the depressive-like effect induced in mice by subchronic ketamine administration. Behav Pharmacol. (2017) 28:582–5. doi: 10.1097/FBP.0000000000000327
39. Thelen C, Sens J, Mauch J, Pandit R, Pitychoutis PM. Repeated ketamine treatment induces sex-specific behavioral and neurochemical effects in mice. Behav Brain Res. (2016) 312:305–12. doi: 10.1016/j.bbr.2016.06.041
40. Clarke M, Razmjou S, Prowse N, Dwyer Z, Litteljohn D, Pentz R, et al. Ketamine modulates hippocampal neurogenesis and pro-inflammatory cytokines but not stressor induced neurochemical changes. Neuropharmacology. (2017) 112:210–20. doi: 10.1016/j.neuropharm.2016.04.021
41. Krimmel SR, Zanos P, Georgiou P, Colloca L, Gould TD. Classical conditioning of antidepressant placebo effects in mice. Psychopharmacology. (2020) 237:93–102. doi: 10.1007/s00213-019-05347-4
42. Neves G, Borsoi M, Antonio CB, Pranke MA, Betti AH, Rates SMK. Is forced swimming immobility a good endpoint for modeling negative symptoms of schizophrenia? - study of sub-anesthetic ketamine repeated administration effects. An Acad Bras Cienc. (2017) 89:1655–69. doi: 10.1590/0001-3765201720160844
43. Kara NZ, Agam G, Anderson GW, Zitron N, Einat H. Lack of effect of chronic ketamine administration on depression-like behavior and frontal cortex autophagy in female and male ICR mice. Behav Brain Res. (2017) 317:576–80. doi: 10.1016/j.bbr.2016.09.056
44. Chatterjee M, Jaiswal M, Palit G. Comparative evaluation of forced swim test and tail suspension test as models of negative symptom of schizophrenia in rodents. ISRN Psychiatry. (2012) 2012:1–5. doi: 10.5402/2012/595141
45. Popik P, Kos T, Sowa-Kućma M, Nowak G. Lack of persistent effects of ketamine in rodent models of depression. Psychopharmacology. (2008) 198:421–30. doi: 10.1007/s00213-008-1158-z
46. Singh S, Choudhury A, Gusain P, Parvez S, Palit G, Shukla S, et al. Oral acetate supplementation attenuates N-methyl D-aspartate receptor hypofunction-induced behavioral phenotypes accompanied by restoration of acetyl-histone homeostasis. Psychopharmacology. (2016) 233:1257–68. doi: 10.1007/s00213-016-4213-1
47. Hou Y, Zhang H, Xie G, Cao X, Zhao YN, Liu Y, et al. Neuronal injury, but not microglia activation, is associated with ketamine-induced experimental schizophrenic model in mice. Prog Neuro-Psychopharmacol Biol Psychiatry. (2013) 45:107–16. doi: 10.1016/j.pnpbp.2013.04.006
48. Owolabi RA, Akanmu MA, Adeyemi OI. Effects of ketamine and N-methyl-d-aspartate on fluoxetine-induced antidepressant-related behavior using the forced swimming test. Neurosci Lett. (2014) 566:172–6. doi: 10.1016/j.neulet.2014.01.015
49. Zhang GF, Liu WX, Qiu LL, Guo J, Wang XM, Sun HL, et al. Repeated ketamine administration redeems the time lag for citalopram's antidepressant-like effects. Eur Psychiatry. (2015) 30:504–10. doi: 10.1016/j.eurpsy.2014.11.007
50. Jiang Y, Wang Y, Sun X, Lian B, Sun H, Wang G, et al. Short- and long-term antidepressant effects of ketamine in a rat chronic unpredictable stress model. Brain Behav. (2017) 7:1–11. doi: 10.1002/brb3.749
51. Li Q, Wu HR, Fan SJ, Liu DX, Jiang H, Zhang Q, et al. The effects of sub-anesthetic ketamine plus ethanol on behaviors and apoptosis in the prefrontal cortex and hippocampus of adolescent rats. Pharmacol Biochem Behav. (2019) 184:172742. doi: 10.1016/j.pbb.2019.172742
52. Getachew B, Tizabi Y. Both ketamine and NBQX attenuate alcohol-withdrawal induced depression in male rats. J Drug Alcohol Res. (2019) 8:236069. doi: 10.4303/jdar/236069
53. Garcia LS, Comim CM, Valvassori SS, Réus GZ, Andreazza AC, Stertz L, et al. Chronic administration of ketamine elicits antidepressant-like effects in rats without affecting hippocampal brain-derived neurotrophic factor protein levels. Basic Clin Pharmacol Toxicol. (2008) 103:502–6. doi: 10.1111/j.1742-7843.2008.00210.x
54. Chindo BA, Adzu B, Yahaya TA, Gamaniel KS. Ketamine-enhanced immobility in forced swim test: a possible animal model for the negative symptoms of schizophrenia. Prog Neuro-Psychopharmacol Biol Psychiatry. (2012) 38:310–6. doi: 10.1016/j.pnpbp.2012.04.018
55. De Carvalho Cartágenes S, Fernandes LMP, Carvalheiro TCVS, De Sousa TM, Gomes ARQ, Monteiro MC, et al. “Special K” drug on adolescent rats: Oxidative damage and neurobehavioral impairments. Oxid Med Cell Longevity. (2019) 2019:5452727. doi: 10.1155/2019/5452727
56. Réus GZ, Abelaira HM, dos, Santos MAB, Carlessi AS, Tomaz DB, et al. Ketamine and imipramine in the nucleus accumbens regulate histone deacetylation induced by maternal deprivation and are critical for associated behaviors. Behav Brain Res. (2013) 256:451–6. doi: 10.1016/j.bbr.2013.08.041
57. Ecevitoglu A, Canbeyli R, Unal G. Oral ketamine alleviates behavioral despair without cognitive impairment in Wistar rats. Behav Brain Res. (2019) 372:112058. doi: 10.1016/j.bbr.2019.112058
58. Aricioglu F, Yalcinkaya C, Ozkartal CS, Tuzun E, Sirvanci S, Kucukali CI, et al. NLRP1-mediated antidepressant effect of ketamine in chronic unpredictable mild stress model in rats. Psychiatry Investig. (2020) 17:283–91. doi: 10.30773/pi.2019.0189
59. Tizabi Y, Bhatti BH, Manaye KF, Das JR, Akinfiresoye L. Antidepressant-like effects of low ketamine dose is associated with increased hippocampal AMPA/NMDA receptor density ratio in female Wistar-Kyoto rats. Neuroscience. (2012) 213:72–80. doi: 10.1016/j.neuroscience.2012.03.052
60. Akinfiresoye L, Tizabi Y. Antidepressant effects of AMPA and ketamine combination: role of hippocampal BDNF. synapsin, and mTOR. Psychopharmacology. (2013) 230:291–8. doi: 10.1007/s00213-013-3153-2
61. Chapman CD, Benedict C, Schiöth HB. Experimenter gender and replicability in science. Sci Adv. (2018) 4:e1701427. doi: 10.1126/sciadv.1701427
62. Georgiou P, Zanos P, Jenne C, Highland J, Gerhard D, Duman R, et al. Human experimenter sex modulates mouse behavioral responses to stress and to the antidepressant ketamine. Biol Psychiatry. (2018) 83:S277. doi: 10.1016/j.biopsych.2018.02.715
63. Carrier N, Kabbaj M. Sex differences in the antidepressant-like effects of ketamine. Neuropharmacology. (2013) 70:27–34. doi: 10.1016/j.neuropharm.2012.12.009
64. Franceschelli A, Sens J, Herchick S, Thelen C, Pitychoutis PM. Sex differences in the rapid and the sustained antidepressant-like effects of ketamine in stress-naïve and “depressed” mice exposed to chronic mild stress. Neuroscience. (2015) 290:49–60. doi: 10.1016/j.neuroscience.2015.01.008
65. Sarkar A, Kabbaj M. Sex differences in effects of ketamine on behavior, spine density, and synaptic proteins in socially isolated rats. Biol Psychiatry. (2016) 80:448–56. doi: 10.1016/j.biopsych.2015.12.025
66. Trujillo KA, Zamora JJ, Warmoth KP. Increased response to ketamine following treatment at long intervals: implications for intermittent use. Biol Psychiatry. (2008) 63:178–83. doi: 10.1016/j.biopsych.2007.02.014
67. Ionescu DF. Repeat-dose ketamine augmentation for treatment-resistant depression with chronic suicidal ideation: a randomized, double blind, placebo controlled trial. J Affect Disord. (2019) 243:516–24. doi: 10.1016/j.jad.2018.09.037
68. Phillips JL, Norris S, Talbot J, Birmingham M, Hatchard T, Ortiz A, et al. Single, repeated, and maintenance ketamine infusions for treatment-resistant depression: a randomized controlled trial. Am J Psychiatry. (2019) 176:401–9. doi: 10.1176/appi.ajp.2018.18070834
69. Melo A, Kokras N, Dalla C, Ferreira C, Ventura-Silva AP, Sousa N, et al. The positive effect on ketamine as a priming adjuvant in antidepressant treatment. Transl Psychiatry. (2015) 5:1–10. doi: 10.1038/tp.2015.66
70. Fedgchin M, Trivedi M, Daly EJ, Melkote R, Lane R, Lim P, et al. Efficacy and safety of fixed-dose esketamine nasal spray combined with a new oral antidepressant in treatment-resistant depression: results of a randomized, double-blind, active-controlled study (TRANSFORM-1). Int J Neuropsychopharmacol. (2019) 22:616–30. doi: 10.1093/ijnp/pyz039
71. Popova V, Daly EJ, Trivedi M, Cooper K, Lane R, Lim P, et al. Efficacy and safety of flexibly dosed esketamine nasal spray combined with a newly initiated oral antidepressant in treatment-resistant depression: a randomized double-blind active-controlled study. Am J Psychiatry. (2019) 176:428–38. doi: 10.1176/appi.ajp.2019.19020172
72. Borsoi M, Nunes LED, Barbosa AR, Lima MS, Medeiros I, Pranke MA, et al. Intermittent repeated stress but not ketamine changes mice response to antidepressants. Neurosci Lett. (2021) 741:135452. doi: 10.1016/j.neulet.2020.135452
73. Onaolapo OJ, Paul TB, Onaolapo AY. Comparative effects of sertraline, haloperidol or olanzapine treatments on ketamine-induced changes in mouse behaviours. Metab Brain Dis. (2017) 32:1475–89. doi: 10.1007/s11011-017-0031-3
74. Fukumoto K, Toki H, Iijima M, Hashihayata T, Yamaguchi JI, Hashimoto K, et al. Antidepressant potential of (R)-ketamine in rodent models: comparison with (S)-ketamine. J Pharmacol Exp Therap. (2017) 361:9–16. doi: 10.1124/jpet.116.239228
75. Yang C, Shirayama Y, Zhang JC, Ren Q, Yao W, Ma M, et al. R-ketamine: a rapid-onset and sustained antidepressant without psychotomimetic side effects. Transl Psychiatry. (2015) 5:e632. doi: 10.1038/tp.2015.136
76. Zhang JC, Li SX, Hashimoto K. R (-)-ketamine shows greater potency and longer lasting antidepressant effects than S (+)-ketamine. Pharmacol Biochem Behav. (2014) 116:137–41. doi: 10.1016/j.pbb.2013.11.033
77. Fujita Y, Hashimoto K. Decreased bone mineral density in ovariectomized mice is ameliorated after subsequent repeated intermittent administration of (R)-ketamine, but not (S)-ketamine. Neuropsychopharmacol Rep. (2020) 40:401–6. doi: 10.1002/npr2.12132
78. Fujita A, Fujita Y, Pu Y, Chang L, Hashimoto K. MPTP-induced dopaminergic neurotoxicity in mouse brain is attenuated after subsequent intranasal administration of (R)-ketamine: a role of TrkB signaling. Psychopharmacology. (2020) 237:83–92. doi: 10.1007/s00213-019-05346-5
79. Tan Y, Fujita Y, Qu Y, Chang L, Pu Y, Wang S, et al. Phencyclidine-induced cognitive deficits in mice are ameliorated by subsequent repeated intermittent administration of (R)-ketamine, but not (S)-ketamine: role of BDNF-TrkB signaling. Pharmacol Biochem Behav. (2020) 188:172839. doi: 10.1016/j.pbb.2019.172839
Keywords: chronic ketamine, forced swim test, major depression, literature search, sex differences, strain differences, sustained effects, subchronic
Citation: Weston RG, Fitzgerald PJ and Watson BO (2021) Repeated Dosing of Ketamine in the Forced Swim Test: Are Multiple Shots Better Than One? Front. Psychiatry 12:659052. doi: 10.3389/fpsyt.2021.659052
Received: 26 January 2021; Accepted: 12 April 2021;
Published: 11 May 2021.
Edited by:
Polymnia Georgiou, University of Maryland, Baltimore, United StatesReviewed by:
Katherine Wright, University of Florida, United StatesCopyright © 2021 Weston, Fitzgerald and Watson. This is an open-access article distributed under the terms of the Creative Commons Attribution License (CC BY). The use, distribution or reproduction in other forums is permitted, provided the original author(s) and the copyright owner(s) are credited and that the original publication in this journal is cited, in accordance with accepted academic practice. No use, distribution or reproduction is permitted which does not comply with these terms.
*Correspondence: Brendon O. Watson, brendonw@umich.edu
Disclaimer: All claims expressed in this article are solely those of the authors and do not necessarily represent those of their affiliated organizations, or those of the publisher, the editors and the reviewers. Any product that may be evaluated in this article or claim that may be made by its manufacturer is not guaranteed or endorsed by the publisher.
Research integrity at Frontiers
Learn more about the work of our research integrity team to safeguard the quality of each article we publish.