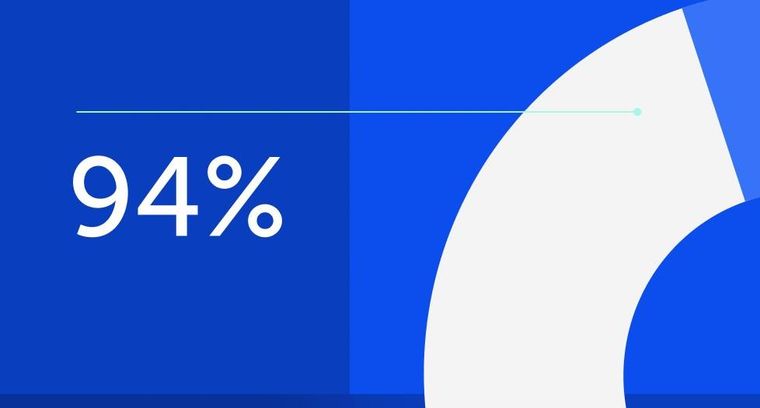
94% of researchers rate our articles as excellent or good
Learn more about the work of our research integrity team to safeguard the quality of each article we publish.
Find out more
CLINICAL TRIAL article
Front. Psychiatry, 02 June 2021
Sec. Psychopharmacology
Volume 12 - 2021 | https://doi.org/10.3389/fpsyt.2021.653026
This article is part of the Research TopicModulation of NMDA Receptors: From Bench Side to Clinical Applications in PsychiatryView all 11 articles
N-methyl-D-aspartate glutamate-receptor (NMDAR) antagonists such as ketamine have demonstrated efficacy in both major depressive disorder (MDD) and bipolar disorder depression (BP-D). We have previously reported that reduction in Glx (glutamate + glutamine) in the ventromedial prefrontal cortex/anterior cingulate cortex (vmPFC/ACC), measured by proton magnetic resonance spectroscopy (1H MRS) at 3T during a ketamine infusion, mediates the relationship of ketamine dose and blood level to improvement in depression. In the present study, we assessed the impact of D-cycloserine (DCS), an oral NMDAR antagonist combined with lurasidone in BP-D on both glutamate and Glx. Subjects with DSM-V BP-D-I/II and a Montgomery-Asberg Depression Rating Scale (MADRS) score>17, underwent up to three 1H MRS scans. During Scan 1, subjects were randomized to receive double-blind lurasidone 66 mg or placebo. During Scan 2, all subjects received single-blind DCS 950 mg + lurasidone 66 mg, followed by 4 weeks of open label phase of DCS+lurasidone and an optional Scan 3. Five subjects received lurasidone alone and three subjects received placebo for Scan 1. Six subjects received DCS+lurasidone during Scan 2. There was no significant baseline or between treatment-group differences in acute depression improvement or glutamate response. In Scan 2, after a dose of DCS+lurasidone, peak change in glutamate correlated negatively with improvement from baseline MADRS (r = −0.83, p = 0.04). There were no unexpected adverse events. These preliminary pilot results require replication but provide further support for a link between antidepressant effect and a decrease in glutamate by the NMDAR antagonist class of antidepressants.
Bipolar disorder affects 2% of the population in the United States (1). Despite overall effectiveness of FDA approved compounds, many individuals with bipolar depression (BP-D) experience persistent depression despite antidepressant medication treatment, either alone or combined with mood stabilizers. For example, across several recent registration studies, ~40-50% of subjects were non-responders based upon Montgomery-Asberg Depression Rating Scale (MADRS) (2) scores ≥50% of baseline (3–5).
Recently, the N-methyl-D-asparate glutamate-receptor (NMDAR) antagonist, ketamine, has emerged as a potential treatment option for both major depressive disorder (MDD) (6, 7) and (BP-D) (8). Although the antidepressant mechanism of action of ketamine remains unclear, convergent evidence suggests that dysfunction of glutamatergic systems plays a role in the pathophysiology of BP-D (9, 10).
However, intravenous ketamine use is limited by loss of benefit after about 5–7 days and transient psychotomimetic side effects during administration. Intranasal ketamine is easier to administer but may have more side effects (11, 12). D-cycloserine (DCS), an FDA-approved anti-tuberculosis drug, is an NMDAR antagonist at higher doses. It is primarily an antagonist at >500 mg (13–15), via the glycine co-receptor of the NMDAR and may have a more favorable safety profile than ketamine. Potential antidepressant effects of DCS were first reported in 1959 (16) but not formally studied until recently. Efficacy of DCS in a dose of >500 mg in MDD, including an anti-suicidal effect, is supported by two double-blind studies (15, 17). Recently, we reported an open label study of treatment resistant BP-D—a single infusion of ketamine followed by 8 weeks of a combination of DCS + FDA approved medications for BP-D (including lurasidone). This combination was employed seeking a treatment where an atypical antipsychotic prevented any potential psychomimetic effect of DCS and perhaps had an additional antidepressant action. Indeed, a sustained benefit for the duration of treatment was seen (46% symptom reduction, p = 0.019 vs. baseline) without significant safety concerns (18). Of note, there was a decline in benefit over the first 2 weeks post ketamine, that reversed with the ongoing combination of DCS and lurasidone or other FDA-approved treatments for BP-D.
In previous studies, we used proton magnetic resonance spectroscopy (1H MRS) to quantify ketamine effects on Glx (a combination of glutamate (Glu) and glutamine resonance signals: Glu+glutamine) in the ventromedial prefrontal cortex, along with the adjacent anterior cingulate cortex (vmPFC/ACC) in both healthy (19) and depressed (20, 21) individuals. Our focus on the vmPFC and the ACC stems from extensive research implicating these regions in the pathogenesis of mood disorders (22–24) and microdialysis rodent (25, 26) studies suggesting that the glutamatergic surge in response to NMDAR antagonists is maximal in the vmPFC.
In our recently published, placebo-controlled, dose-finding, randomized clinical trial of ketamine (21), we found that improvement in MDD had a positive linear relationship with ketamine dose and blood level, and a negative correlation with Glx response. Reduction of Glx mediated the relationship of ketamine dose and level with antidepressant response. In the present report, we sought to determine whether the same relationship is found within BP-D for DCS combined with lurasidone. This combination of medications seeks to preserve the antidepressant effect of DCS and block its potential psychotomimetic effect with lurasidone. Lurasidone may also augment the antidepressant effect of DCS since it an FDA approved medication for BP-D (3, 4).
To determine the independent biological effect of lurasidone, prior to the DCS/lurasidone scan, all subjects underwent an 1H MRS scan while receiving double-blind lurasidone 66 mg or placebo. We have previously shown that this 1H MRS method is sensitive to DCS-induced changes in Glx in healthy controls (27). Due to upgrades in both scanner quality and 1H MRS methodology (28–30), we now report the more specific 1H MRS outcome of Glu, in place of Glx. We hypothesized that we would find a similar relationship between Glu and DCS+lurasidone mediated antidepressant response, thus adding to our understanding of NMDAR antagonist mechanism of action in depression.
The study was conducted under a biomarker letter of support (IND 129194) from the US Food and Drug Administration and posted on www.clinicaltrials.gov (NCT03402152).
Enrollment criteria included DSM-V current BP-D-I/II, confirmed by a SCID (31). Subjects had at least moderate depression symptoms, as defined by a MADRS score>17, with no current or chronic psychosis or substance use disorder. To minimize further acute clinical deterioration, subjects were permitted to remain on all current pre-study psychotropics, with the exception that prior antipsychotics and fluoxetine were discontinued at least 24 h before the first MRI to mitigate the effect of such medications on the Glu response to acute administration of DCS or lurasidone.
After screening, each eligible subject underwent up to three 1H MRS scans, on three different days (referred to as Scan 1, Scan 2, and Scan 3, respectively). During Scan 1, all subjects were randomized to receive double-blind lurasidone 66 mg or placebo, and during Scan 2 all subjects received single-blind one dose of NRX-101 (DCS 950 mg + lurasidone 66 mg). All subjects received a dose of pyridoxine (200 mg) along with the study medication to prevent DCS related reductions in Vitamin B6 (32). After Scan 2, subjects were started on a combination of open-label flexibly dosed DCS/lurasidone and daily pyridoxine 200 mg for 4 weeks, culminating in an optional final 1H MRS scan (Scan 3).
Scans 1 and 2 were at least 1 day apart, and subjects and data analysts, including 1H MRS data processing, were blind to treatment order (e.g., unaware that DCS+lurasidone was always administered immediately prior to Scan 2). The mean time between Scans 1 and 2 was 3.3 days (range 1–7 days). After structural MRI and baseline 1H MRS scans (~30 min), subjects were briefly removed from the scanner for study drug administration, followed by serial 1H MRS frame acquisitions for up to 70 min following drug administration. Subjects were assessed using a side effects checklist, the C-SSRS and MADRS at baseline, ~30 min before and after the imaging on the 1H MRS days and weekly during the 4-week follow-up.
The study was terminated by the sponsor after eight randomized subjects due to a corporate decision to pursue a different approach. Thus, we only report pilot results due to the limited sample size.
Six subjects were scanned on a Siemen Prisma 3.0T MR scanner equipped with a 32-channel surface coil array and two subjects were scanned on a General Electric SIGNA Premier 3T MR scanner equipped with a 21-channel surface coil array. MR data were acquired with the same protocols on both scanners. The protocols for voxel placement and 1H MRS data acquisition for both sessions of before and after medication were the same. First, three-plane scout images were acquired, followed by a high-resolution structural MRI scan in the sagittal planes; Then, high resolution structural MRI images in the oblique axial planes parallel to the AC-PC line were acquired. We placed the 1H MRS voxel (3.0 × 2.5 × 2.5 cm3) based on the sagittal and axial MR images in the vmPFC and ACC, with the center of the posterior side of the voxel close to the frontal tip of the cingulate gyrus (Figure 1). The 1H MRS data were acquired from the voxel using a commercial version of the PRESS sequence (33) implemented on both scanners with following parameters: TR/TE = 1,500/120 ms (28, 29), spectral width = 2,000 Hz, free induction decay (FID) datapoints = 1,024, number of excitations (NEX) for water unsuppressed 1H MRS scan = 16, and NEX for water suppressed 1H MRS = 240. Total scan time for each 1H MRS frame, including pre-scan, was ~8 min.
We combined the multichannel 1H MRS data, using the unsuppressed water signal as a reference for correcting phase errors and for calculating weighting factors of S/N, where S is the amplitude of water signal and N is the standard deviation of noise of each channel (34). We then corrected frequency and phase shifts among the FIDs in each 1H MRS data file and combined them into a single FID for each baseline and dynamic 1H MRS scan. We removed the residual water signal using a singular value decomposition-based matrix-pencil method (35).
We quantified the 1H MRS data in the time domain using the software packages AMARES (36) imbedded in jMRUI (37). To improve accuracy of the quantification of the metabolites of interest via spectral fitting, we fit all peaks with major contributions, including metabolic peaks of N-acetylaspartate at 2.01 ppm, total creatine (Cr) at 3.02 ppm, total choline at 3.24 ppm and Glu around 2.26 ppm were included in the spectral fitting. In the present paper, we focus on the role of Glu/Cr and Glx/Cr, and did not analyze NAA and Ch. For accurate spectral fitting of Glu, we incorporated prior knowledge in the model of relative frequencies, phases, and amplitudes of the major peaks Glu obtained by fitting the simulated spectra of Glu using AMARES, similar to the approach in the reference (38), where the prior knowledge was obtained from phantom 1H MRS data of Glu. The simulations of Glu spectra for both Siemens and GE data were performed using the MARSS software package (30).
Due to higher quality measurements on our upgraded scanner, we modified the initial analysis plan posted on clinicaltrials.gov, and utilized Glu peak, as opposed to Glx AUC, as the primary metabolite outcome. Glx was analyzed as a secondary measure. Based on pharmacokinetics (39–41) of DCS, our prior finding of the 1H MRS peak at ~35 min DCS post-dose (27) and our prior ketamine study using peak level (21), Glu 1H MRS peak level was used, defined as a mean from 30 to 46 min post drug. We used Cr as a reference for the relative quantification of Glu and expressed the outcome measure from 1H MRS as Glu/Cr. The rationale for using Cr as a standard is as follows: (1). The Cr level is assumed to be stable over the course of drug administration; and (2). The tissue volumes for Glu and Cr are the same in the voxel and a partial volume effect of using water as a reference is avoided. We used the ratio of standard deviation to estimated amplitude given in the fitting by the jMRUI software, as a metric for quality control and set the threshold to be 20%. No data were excluded (42).
Prior to analysis, all variables were examined for distribution and outliers. Due to the small sample sizes, parametric tests were utilized only for repeated-measures modeling of change in MADRS, for which residuals were sufficiently normal.
Wilcoxon sign rank tests were used to test for significant Scan 1 percent change in MADRS and Glu within treatment group, and Scan 2 percent change in MADRS and Glu in the overall sample. Additionally, Wilcoxon sign rank tests were also used to assess both baseline and change in MADRS and Glu response from Scan 1 to Scan 2, within subject.
Mixed effects linear regression models were fit to model MADRS change from baseline over the four follow-up weeks. First, an intercept-only model was fit to assess mean change across the 4 weeks. Next, week was added to the model as a categorical predictor to estimate change from baseline at each week. Both models featured a random intercept for subject. Spearman's correlations were used to assess the association between MADRS and Glu responses, on Scan 1 and on Scan 2, separately. Due to the small sample sizes, descriptive statistics are provided in the text.
Significance level was set at α = 0.05, with results reported as mean ± standard deviation (SD) and median with interquartile ranges (IQR, 25th percentile, 75th percentile). All analyses were performed using SAS version 9.4 (Cary, NC: SAS Institute Inc.; 2014). The data that support the findings of this study are available on request from the corresponding author if accompanied by a reasonable plan for their use. The data are not publicly available due to privacy or ethical restrictions.
Subjects: 9 subjects consented to participate (Figure 2), eight met study criteria and were randomized (Table 1). On entering the study, three randomized subjects were unmedicated, and the remaining five randomized subjects were on stable doses of mood stabilizers and antidepressants for at least 1 month, including one subject on oxcarbazepine 600 mg and escitalopram 20 mg, one subject on lithium 450 mg and fluoxetine 60 mg (discontinued prior to scan), one subject on lamotrigine 50 mg and diphenhydramine 50 mg, one subject on sertraline 200 mg, zolpidem 10 mg, gabapentin 1,000 mg and diazepam 10 mg and one subject on valproic acid 1,000 mg, paroxetine 20 mg and dextroamphetamine and amphetamine 20 mg. Six out of eight randomized subjects completed the first two scans, and both non-completers received lurasidone during Scan 1. Four subjects completed the four-week open-label phase, with 1H MRS available for three subjects.
Figure 2. Examples of voxel placement (red outline) on the axial and sagittal localizer images showing the size and location in the medial ventral prefrontal cortex (Left) and the original, estimated, and their difference spectra from the voxel (Right).
Five subjects were randomized to lurasidone and three to placebo on Scan 1. Overall, subjects exhibited a comparable degree of acute improvement from the baseline MADRS after one dose of lurasidone alone (57.0% ± 31.7, p = 0.06, n = 5) or placebo (72.7% ± 32.6, p = 0.25, n = 3) at Scan 1; and sustained this improvement after a mean of 3.3 days of one dose of DCS+lurasidone at Scan 2 (67.2% ± 22.6, p = 0.03, n = 6). Only the DCS+lurasidone improvement at Scan 2 relative to baseline reached statistical significance. Among subjects that completed both scans, there was no significant difference between baseline MADRS on scan days, suggesting a lack of carryover effect from Scan 1 (Signed-Rank Test p = 0.31).
Using mixed-effects linear modeling, a significant overall MADRS improvement over time was seen (t4 = −6.38, p = 0.0031) over the 4-week treatment, with the final MADRS total decreasing to 17.5 ± 12.0. Weekly contrasts demonstrated significant improvement from baseline at all rating points except at 2 weeks (p = 0.0038, Figure 3 Right). No subjects achieved euthymia, defined by MADRS <8.
Figure 3. A scatter plot of mean change in Glu vs. improvement from baseline MADRS (rs = −0.83, p = 0.04) on the DCS/Lurasidone days (Left). Spaghetti plot of MADRS over time by subject (Right).
No patients exhibited active suicidal ideation, intent or behavior during the study on the C-SSRS (all C-SSRS scores <3). There were no unexpected side effects. There was one serious adverse event involving a patient who was observed overnight in hospital for moderate dystonia thought to be related to lurasidone. This subject remained in the study with a reduction in dose.
1H MRS: As previously (27), the Glu peak was ~35 min post oral drug administration. Consistent with our previous work with ketamine in MDD (21), Glu increase was seen after placebo only. Both lurasidone alone and the DCS+lurasidone combination attenuated the Glu response. However, neither the within nor the between group changes in Glu levels were significant statistically. The changes observed were small: a decrease after lurasidone [Median (IQR) = −6.6% (−16.9%,−1.9%), n = 5, p = 0.31]; and increase after placebo [Median (IQR)= +12.9% (−9.0%,34.8%), n = 3, p = 1.0]; and a decrease after DCS+lurasidone treatment [Median (IQR)= −2.7% (−7.6%,−2.2%), n = 6, p = 0.31] on the 1H MRS scan days (Wilcoxon sign rank test). Within the same subjects, Glu response decreased after DCS+lurasidone and lurasidone alone (median: −3.9% vs. −7.4%, n = 4) and increased after placebo (median: 20.3% vs. −6.7%, n = 2) but none of these effects were statistically significant.
On the DCS+lurasidone treatment day (Scan 2), change of Glu from baseline on that day correlated negatively with improvement from baseline MADRS (rs = −0.83, p = 0.04, Figure 3, Left), using Spearman's correlation coefficient. By contrast, on the placebo/lurasidone day (Scan 1), Glu change did not correlate with MADRS change (rs = 0.29, p = 0.53). Controlling for Scan 1 treatment-type did not change results for Scan 1 or 2 correlations. There were no significant differences in baseline Glu levels on Scan 1 and Scan 2 [Day 1 Med (IQR) = 0.31 (0.20–0.35); Day 2 Med (IQR) = 0.29 (0.22–0.37); Signed-Rank Test p = 0.69], supporting the assumption that this relationship was not due to carryover effects from Scan 1. Only 3 subjects completed Scan 3 (Week 4) 1H MRS, without significant results.
Glx did not show any statistically significant results in Day 1 or Day 2 analysis. Change in Glx on Day 2 did not correlate with either change in Glu on Day 2 (rs = 0.54, p = 0.27), nor with MADRS change (rs = −0.37, p = 0.47).
Due to the small sample size, particularly for the placebo and lurasidone alone groups, the present findings are presented as a preliminary pilot study. Nevertheless, we did observe that lower mean Glu level post treatment with an NMDAR antagonist combined with lurasidone predicts better antidepressant response in BP-D, consistent with prior findings (21) in MDD when ketamine was employed. This relationship was seen despite a lack of significant between-treatment group differences for symptoms or 1H MRS outcomes, and was not seen after treatment with lurasidone alone or placebo. Of course, the small sample size precluded an adequately powered statistical analysis.
In a secondary finding, we demonstrate tolerability and potential efficacy of acute, high-dose DCS in BP-D when combined with lurasidone and no reports of psychotomimetic symptoms when receiving this medication combination. While the analysis was limited by the small sample, statistically significant improvement in depression was seen after an acute dose of DCS+lurasidone, but not with lurasidone alone or placebo. Similarly, the degree of clinical response was comparable to our previous open label findings of efficacy over 8 weeks of DCS combined with atypical antipsychotics (27).
We previously proposed that an increase in Glu may be a stress response because it is most robust in the placebo and healthy control groups (19, 21). While meta-analysis of medicated MDD patients indicate lower levels of Glx, when medication status is considered, the data indicate that medicated MDD has lower Glx or glutamate and untreated MDD may have elevated levels (43). Studies of BP-D have reported higher Glu levels (44–46), as have studies in other relatively treatment resistant populations such as postpartum depression (47). Similarly, a large mega-analysis found that while medial frontal cortex Glu and Glx are generally lower in schizophrenia compared to healthy controls, higher Glu and Glx levels were associated with more severe symptoms and lower levels were associated with antipsychotic treatment (48).
While our small sample size limited the ability to assess between group differences in the Glu response between scan days, we replicate our previous findings with ketamine (21), finding that DCS combined with lurasidone, appears to diminish the Glx or glutamate response and this effect correlates with degree of antidepressant effect. A reduced stress response is consistent with preclinical studies, indicating that NMDAR antagonist related antidepressant response may produce a resilience effect (49). Similarly, putative glutamatergic treatments in schizophrenia also appear to reduce NMDAR antagonist induced glutamate increases (50). Thus, we have previous proposed that elevated Glu or Glx may be a marker of depressive illness severity (51), and a reduction is an indicator of antidepressant response to NMDAR antagonists.
In our previous study of DCS alone in healthy controls (27), we found a positive peak at ~35 min post-dose (23 ± 5% increase). In the present report of BP-D patients, we found a small decrease in Glu after DCS+lurasidone treatment, consistent with a blunting of the elevation seen in other studies including those employing the NMDAR antagonist ketamine. Similar to our ketamine study (21), this blunting was correlated with degree of antidepressant effect.
The use of target engagement biomarkers early in drug development can facilitate dose selection and initial proof-of-mechanism assessments (50, 52–54). While the present report was not designed to assess dose response, our results do further support that target engagement at the NMDAR and the NMDAR glycine site, as measured by 1H MRS, is necessary for antidepressant response. Exemplary of this, a recent study of treatment resistant depression (55), found neither antidepressant nor 1H MRS Glu changes in response to AV-101, a competitive antagonist at the NMDAR glycine site. A subsequent study of AV-101 in healthy controls found evidence for a dose response for AV-101 using the auditory steady state response (56), and suggested that higher doses may be needed.
Our study has several limitations, and we emphasize its presentation as a pilot study. The small sample is the principal study limitation. A second concern is the potential carry-over effects from Scan 1 treatment with lurasidone or pre-study medications, especially concomitant mood stabilizers or those with a long half-life such as fluoxetine. Although the discontinuation of other antipsychotics and fluoxetine lowered the blood and brain levels of these medications, this was not for long enough to allow them to wash out of the brain completely. Consequently, although this step reduced the potential impact of such medications, it did not eliminate the possibility of an effect. These limitations are minimized by the lack of baseline Glu and MADRS differences between Scan 1 and 2, and only one subject was taking fluoxetine pre-study. Furthermore, potential variability in Glu from the use of two scanners or pre-study medication differences was minimized because we focused on the acute percentage change in Glu post study drug administration within each day for each subject, not absolute Glu values.
Finally, we focused on Glu in the present report instead of the composite measure of Glx by taking advantages of data acquisition parameter TE = 120 ms, which is optimized for Glu separation (28, 29) and spectral fitting prior knowledge obtained from simulated model spectra (30, 38). While this optimized our 1H MRS sequence for Glu measurements, the spectral overlapping between Glu and Gln might result in a “competition” or a “compensation” between them in the fitting, limiting the accuracy of Gln. Therefore, the variation of Glx may be smaller than that of Gln itself but may still larger than Glu. For this reason, we did not focus on Glx, nor report Gln. This limits the direct comparison to our Glx results in prior studies (21, 27). Better spectral fitting methods need to be developed to improve the fitting of Glu, Gln, and Glx.
In conclusion, our preliminary pilot results are consistent with our previous work. Attenuation of the Glu response being correlated with antidepressant response to NMDAR antagonists requires replication in a larger, multi-dose, controlled study. If replicated, this biomarker may prove to be a method for screening NMDAR antagonists for antidepressant potential.
The raw data supporting the conclusions of this article will be made available by the authors, without undue reservation.
The studies involving human participants were reviewed and approved by NYSPI IRB. The patients/participants provided their written informed consent to participate in this study.
ZD, JM, and JK had full access to all of the data in the study and take responsibility for the integrity of the data and the accuracy of the data analysis. JK, ZD, MM, and JM: substantial contributions to conception and design. JK, ZD, MG, ML, VW, T-HC, TS, and JM: acquisition, analysis, or interpretation of data. JK, T-HC, JM, and ZD: drafting of the manuscript. JK, T-HC, JM, ML, and ZD: critical revision of the manuscript for important intellectual content. All authors reviewed the final submission and gave final approval of the submitted version.
This work was supported by a grant from NeuroRx to JK. The funding agency had no role in manuscript preparation or decision to submit for publication.
JK reports having received consulting payments within the last 24 months from Alphasights, Charles River Associates, Medscape, Putnam, techspert.io, Third Bridge, MEDACorp, Parexel, GroupH, Simon Kucher, ECRI Institute, ExpertConnect, Parexel, Schlesinger Group, CelloHealth, Acsel Health, Strafluence, Guidepoint, L.E.K. and System Analytic. He serves on the MedinCell Psychiatry and Karuna Mechanism of Action (MOA) Advisory Boards. He has conducted clinical research supported by the NIMH, Sunovion, Roche, Alkermes, Cerevance, Corcept, Takeda, Taisho, Lundbeck, Boehringer Ingelheim, NeuroRX and Teva within the last 24 months. JK was a co-investigator on a study that receives lumeteperone and reimbursement for safety testing for an investigator-initiated research from Intra-Cellular Therapies Inc. He owns a small number of shares of common stock from GSK. JM receives royalties for commercial use of the C-SSRS from the Research Foundation for Mental Hygiene.
The remaining authors declare that the research was conducted in the absence of any commercial or financial relationships that could be construed as a potential conflict of interest.
We are grateful to Ms. Anina Klein at the Nathan Kline Institute for her invaluable help providing study monitoring.
1. Merikangas KR, Akiskal HS, Angst J, Greenberg PE, Hirschfeld RM, Petukhova M, et al. Lifetime and 12-month prevalence of bipolar spectrum disorder in the national comorbidity survey replication. Arch Gen Psychiatry. (2007) 64:543–52. doi: 10.1001/archpsyc.64.5.543
2. Montgomery SA, Asberg M. A new depression scale designed to be sensitive to change. Br J Psychiatry. (1979) 134:382–9. doi: 10.1192/bjp.134.4.382
3. Loebel A, Cucchiaro J, Silva R, Kroger H, Hsu J, Sarma K, et al. Lurasidone monotherapy in the treatment of bipolar i Depression: a Randomized, double-Blind, placebo-Controlled study. Am J Psychiatry. (2014) 171:160–8. doi: 10.1176/appi.ajp.2013.13070984
4. Loebel A, Cucchiaro J, Silva R, Kroger H, Sarma K, Xu J, et al. Lurasidone as adjunctive therapy with lithium or valproate for the treatment of bipolar i Depression: a Randomized, double-Blind, placebo-Controlled study. Am J Psychiatry. (2014) 171:169–77. doi: 10.1176/appi.ajp.2013.13070985
5. Earley W, Burgess MV, Rekeda L, Dickinson R, Szatmari B, Nemeth G, et al. Cariprazine treatment of bipolar depression: a Randomized double-Blind placebo-Controlled phase 3 study. Am J Psychiatry. (2019) 176:439–48. doi: 10.1176/appi.ajp.2018.18070824
6. Diazgranados N, Ibrahim LA, Brutsche NE, Ameli R, Henter ID, Luckenbaugh DA, et al. Rapid resolution of suicidal ideation after a single infusion of an n-methyl-D-aspartate antagonist in patients with treatment-resistant major depressive disorder. J Clin Psychiatry. (2010) 71:1605–11. doi: 10.4088/JCP.09m05327blu
7. Grunebaum MF, Galfalvy HC, Choo TH, Keilp JG, Moitra VK, Parris MS, et al. Ketamine for rapid reduction of suicidal thoughts in major depression: a Midazolam-Controlled randomized clinical trial. Am J Psychiatry. (2018) 175:327–35. doi: 10.1176/appi.ajp.2017.17060647
8. Grunebaum MF, Ellis SP, Keilp JG, Moitra VK, Cooper TB, Marver JE, et al. Ketamine versus midazolam in bipolar depression with suicidal thoughts: a pilot midazolam-controlled randomized clinical trial. Bipolar Disord. (2017) 19:176–83. doi: 10.1111/bdi.12487
9. Lener MS, Niciu MJ, Ballard ED, Park M, Park LT, Nugent AC, et al. Glutamate and gamma-Aminobutyric acid systems in the pathophysiology of major depression and antidepressant response to ketamine. Biol Psychiatry. (2017) 81:886–97. doi: 10.1016/j.biopsych.2016.05.005
10. Duman RS, Sanacora G, Krystal JH. Altered connectivity in depression: gABA and glutamate neurotransmitter deficits and reversal by novel treatments. Neuron. (2019) 102:75–90. doi: 10.1016/j.neuron.2019.03.013
11. Daly EJ, Trivedi MH, Janik A, Li H, Zhang Y, Li X, et al. Efficacy of esketamine nasal spray plus oral antidepressant treatment for relapse prevention in patients with treatment-Resistant depression: a Randomized clinical trial. JAMA Psychiatry. (2019) 76:893–903. doi: 10.1001/jamapsychiatry.2019.1189
12. Gastaldon C, Raschi E, Kane JM, Barbui C, Schoretsanitis G. Post-Marketing safety concerns with esketamine: a Disproportionality analysis of spontaneous reports submitted to the fDA adverse event reporting system. Psychother Psychosom. (2021) 90:41–8. doi: 10.1159/000510703
13. Van Berckel BN, Lipsch C, Gispen-De Wied C, Wynne HJ, Blankenstein MA, Van Ree JM, et al. The partial nMDA agonist D-Cycloserine stimulates lH secretion in healthy volunteers. Psychopharmacology (Berl). (1998) 138:190–7. doi: 10.1007/s002130050662
14. Heresco-Levy U, Javitt DC, Gelfin Y, Gorelik E, Bar M, Blanaru M, et al. Controlled trial of D-Cycloserine adjuvant therapy for treatment-resistant major depressive disorder. J Affect Disord. (2006) 93:239–43. doi: 10.1016/j.jad.2006.03.004
15. Heresco-Levy U, Gelfin G, Bloch B, Levin R, Edelman S, Javitt DC, et al. A randomized add-on trial of high-dose D-Cycloserine for treatment-resistant depression. Int J Neuropsychopharmacol. (2013) 16:501–6. doi: 10.1017/S1461145712000910
16. Crane GE. Cyloserine as an antidepressant agent. Am J Psychiatry. (1959) 115:1025–6. doi: 10.1176/ajp.115.11.1025
17. Chen MH, Cheng CM, Gueorguieva R, Lin WC, Li CT, Hong CJ, et al. Maintenance of antidepressant and antisuicidal effects by d-cycloserine among patients with treatment-resistant depression who responded to low-dose ketamine infusion: a double-blind randomized placebo-control study. Neuropsychopharmacology. (2019) 44:2112–8. doi: 10.1038/s41386-019-0480-y
18. Kantrowitz JT, Halberstam B, Gangwisch J. Single dose ketamine followed by daily D-Cycloserine in treatment resistant bipolar depression. J Clin Psychiatry. (2015) 76:737–8. doi: 10.4088/JCP.14l09527
19. Javitt DC, Carter CS, Krystal JH, Kantrowitz JT, Girgis RR, Kegeles LS, et al. Utility of imaging-Based biomarkers for glutamate-Targeted drug development in psychotic disorders: a Randomized clinical trial. JAMA Psychiatry. (2018) 75:11–9. doi: 10.1001/jamapsychiatry.2017.3572
20. Milak MS, Proper CJ, Mulhern ST, Parter AL, Kegeles LS, Ogden RT, et al. A pilot in vivo proton magnetic resonance spectroscopy study of amino acid neurotransmitter response to ketamine treatment of major depressive disorder. Mol Psychiatry. (2016) 21:320–7. doi: 10.1038/mp.2015.83
21. Milak MS, Rashid R, Dong Z, Kegeles LS, Grunebaum MF, Ogden RT, et al. Assessment of relationship of ketamine dose with magnetic resonance spectroscopy of glx and gABA responses in adults with major depression: a Randomized clinical trial. JAMA Netw Open. (2020) 3:e2013211. doi: 10.1001/jamanetworkopen.2020.13211
22. Hanford LC, Nazarov A, Hall GB, Sassi RB. Cortical thickness in bipolar disorder: a systematic review. Bipolar Disord. (2016) 18:4–18. doi: 10.1111/bdi.12362
23. Hibar DP, Westlye LT, Doan NT, Jahanshad N, Cheung JW, Ching CRK, et al. Cortical abnormalities in bipolar disorder: an mRI analysis of 6503 individuals from the eNIGMA bipolar disorder working group. Mol Psychiatry. (2018) 23:932–42. doi: 10.1038/mp.2017.73
24. Hiser J, Koenigs M. The multifaceted role of the ventromedial prefrontal cortex in emotion, decision making, social cognition, and psychopathology. Biol Psychiatry. (2018) 83:638–47. doi: 10.1016/j.biopsych.2017.10.030
25. Moghaddam B, Adams B, Verma A, Daly D. Activation of glutamatergic neurotransmission by ketamine: a novel step in the pathway from nMDA receptor blockade to dopaminergic and cognitive disruptions associated with the prefrontal cortex. J Neurosci. (1997) 17:2921–7. doi: 10.1523/JNEUROSCI.17-08-02921.1997
26. Chowdhury GM, Behar KL, Cho W, Thomas MA, Rothman DL, Sanacora G. (1)H-[(1)(3)C]-nuclear magnetic resonance spectroscopy measures of ketamine's effect on amino acid neurotransmitter metabolism. Biol Psychiatry. (2012) 71:1022–5. doi: 10.1016/j.biopsych.2011.11.006
27. Kantrowitz JT, Milak MS, Mao X, Shungu DC, Mann JJ. D-Cycloserine, an nMDA glutamate receptor glycine site partial agonist, induces acute increases in brain glutamate plus glutamine and gABA comparable to ketamine. Am J Psychiatry. (2016) 173:1241–2. doi: 10.1176/appi.ajp.2016.16060735
28. Snyder J, Wilman A. Field strength dependence of pRESS timings for simultaneous detection of glutamate and glutamine from 1.5 to 7T. J Magn Reson. (2010) 203:66–72. doi: 10.1016/j.jmr.2009.12.002
29. Choi C, Ganji SK, Deberardinis RJ, Hatanpaa KJ, Rakheja D, Kovacs Z, et al. 2-hydroxyglutarate detection by magnetic resonance spectroscopy in IDH-mutated patients with gliomas. Nat Med. (2012) 18:624–9. doi: 10.1038/nm.2682
30. Landheer K, Swanberg KM, Juchem C. Magnetic resonance spectrum simulator (MARSS), a novel software package for fast and computationally efficient basis set simulation. NMR Biomed. (2019) 34:e4129. doi: 10.1002/nbm.4129
31. American Psychiatric Association. Diagnostic and Statistical Manual of Mental Disorders: DSM-5. Fifth edition. Arlington, VA: American Psychiatric Association (2013). doi: 10.1176/appi.books.9780890425596
32. Cohen AC. Pyridoxine in the prevention and treatment of convulsions and neurotoxicity due to cycloserine. Ann N Y Acad Sci. (1969) 166:346–9. doi: 10.1111/j.1749-6632.1969.tb54286.x
33. Bottomley PA. Spatial localization in nMR spectroscopy in vivo. Ann N Y Acad Sci. (1987) 508:333–48. doi: 10.1111/j.1749-6632.1987.tb32915.x
34. Dong Z, Peterson B. The rapid and automatic combination of proton mRSI data using multi-channel coils without water suppression. Magn Reson Imaging. (2007) 25:1148–54. doi: 10.1016/j.mri.2007.01.005
35. Dong Z, Dreher W, Leibfritz D. Toward quantitative short-echo-time in vivo proton mR spectroscopy without water suppression. Magn Reson Med. (2006) 55:1441–6. doi: 10.1002/mrm.20887
36. Vanhamme L, Van Den Boogaart A, Van Huffel S. Improved method for accurate and efficient quantification of mRS data with use of prior knowledge. J Magn Reson. (1997) 129:35–43. doi: 10.1006/jmre.1997.1244
37. Naressi A, Couturier C, Devos JM, Janssen M, Mangeat C, De Beer R, et al. Java-based graphical user interface for the mRUI quantitation package. MAGMA. (2001) 12:141–52. doi: 10.1007/BF02668096
38. Kraguljac NV, Reid MA, White DM, Den Hollander J, Lahti AC. Regional decoupling of n-acetyl-aspartate and glutamate in schizophrenia. Neuropsychopharmacology. (2012) 37:2635–42. doi: 10.1038/npp.2012.126
39. Zitkova L, Tousek J. Pharmacokinetics of cycloserine and terizidone. A comparative study. Chemotherapy. (1974) 20:18–28. doi: 10.1159/000221787
40. Zhu M, Nix DE, Adam RD, Childs JM, Peloquin CA. Pharmacokinetics of cycloserine under fasting conditions and with high-fat meal, orange juice, and antacids. Pharmacotherapy. (2001) 21:891–7. doi: 10.1592/phco.21.11.891.34524
41. Patel DS, Sharma N, Patel MC, Patel BN, Shrivastav PS, Sanyal M. Development and validation of a selective and sensitive lC-MS/MS method for determination of cycloserine in human plasma: application to bioequivalence study. J Chromatogr B Analyt Technol Biomed Life Sci. (2011) 879:2265–73. doi: 10.1016/j.jchromb.2011.06.011
42. Kreis R. The trouble with quality filtering based on relative cramer-Rao lower bounds. Magn Reson Med. (2016) 75:15–8. doi: 10.1002/mrm.25568
43. Moriguchi S, Takamiya A, Noda Y, Horita N, Wada M, Tsugawa S, et al. Glutamatergic neurometabolite levels in major depressive disorder: a systematic review and meta-analysis of proton magnetic resonance spectroscopy studies. Mol Psychiatry. (2019) 24:952–64. doi: 10.1038/s41380-018-0252-9
44. Chitty KM, Lagopoulos J, Lee RS, Hickie IB, Hermens DF. A systematic review and meta-analysis of proton magnetic resonance spectroscopy and mismatch negativity in bipolar disorder. Eur Neuropsychopharmacol. (2013) 23:1348–136. doi: 10.1016/j.euroneuro.2013.07.007
45. Taylor MJ. Could glutamate spectroscopy differentiate bipolar depression from unipolar? J Affect Disord. (2014) 167:80–4. doi: 10.1016/j.jad.2014.05.019
46. Scotti-Muzzi E, Umla-Runge K, Soeiro-De-Souza MG. Anterior cingulate cortex neurometabolites in bipolar disorder are influenced by mood state and medication: a meta-analysis of (1)H-MRS studies. Eur Neuropsychopharmacol. (2021). doi: 10.1016/j.euroneuro.2021.01.096
47. Mcewen AM, Burgess DT, Hanstock CC, Seres P, Khalili P, Newman SC, et al. Increased glutamate levels in the medial prefrontal cortex in patients with postpartum depression. Neuropsychopharmacology. (2012) 37:2428–35. doi: 10.1038/npp.2012.101
48. Merritt K, Mcguire PK, Egerton A, Investigators HMIS, Aleman A. Association of age, antipsychotic medication, and symptom severity in schizophrenia with proton magnetic resonance spectroscopy brain glutamate level: a Mega-analysis of individual participant-Level data. JAMA Psychiatry. (2021). doi: 10.1001/jamapsychiatry.2021.0380
49. Brachman RA, Mcgowan JC, Perusini JN, Lim SC, Pham TH, Faye C, et al. Ketamine as a prophylactic against stress-Induced depressive-like behavior. Biol Psychiatry. (2016) 79:776–86. doi: 10.1016/j.biopsych.2015.04.022
50. Kantrowitz JT, Grinband J, Goff DC, Lahti AC, Marder SR, Kegeles LS, et al. Proof of mechanism and target engagement of glutamatergic drugs for the treatment of schizophrenia: rCTs of pomaglumetad and tS-134 on ketamine-induced psychotic symptoms and pharmacoBOLD in healthy volunteers. Neuropsychopharmacology. (2020) 45:1842–1850. doi: 10.1038/s41386-020-0706-z
51. Kantrowitz JT, Dong Z, Milak MS, Rashid R, Kegeles LS, Javitt DC, et al. Higher Ventromedial Prefrontal Glx and Glutamate Levels in Unmedicated Major Depressive Disorder (under review).
52. Grabb MC, Cross AJ, Potter WZ, Mccracken JT. Derisking psychiatric drug development: the nIMH's fast fail program, a Novel precompetitive model. J Clin Psychopharmacol. (2016) 36:419–21. doi: 10.1097/JCP.0000000000000536
53. Krystal AD, Pizzagalli DA, Mathew SJ, Sanacora G, Keefe R, Song A, et al. The first implementation of the nIMH fAST-FAIL approach to psychiatric drug development. Nat Rev Drug Discov. (2018) 18:82–4. doi: 10.1038/nrd.2018.222
54. Krystal AD, Pizzagalli DA, Smoski M, Mathew SJ, Nurnberger J Jr, Lisanby SH, et al. A randomized proof-of-mechanism trial applying the 'fast-fail' approach to evaluating kappa-opioid antagonism as a treatment for anhedonia. Nat Med. (2020) 26:760–8. doi: 10.1038/s41591-020-0806-7
55. Park LT, Kadriu B, Gould TD, Zanos P, Greenstein D, Evans JW, et al. A randomized trial of the n-Methyl-d-Aspartate receptor glycine site antagonist prodrug 4-Chlorokynurenine in treatment-Resistant depression. Int J Neuropsychopharmacol. (2020) 23:417–25. doi: 10.1093/ijnp/pyaa025
Keywords: N-methyl-D-aspartate, glutamate, MRS—1H nuclear magnetic resonance spectra, biomarker, bipolar depression, D-Cycloserine, lurasidone
Citation: Dong Z, Grunebaum MF, Lan MJ, Wagner V, Choo T-H, Milak MS, Sobeih T, Mann JJ and Kantrowitz JT (2021) Relationship of Brain Glutamate Response to D-Cycloserine and Lurasidone to Antidepressant Response in Bipolar Depression: A Pilot Study. Front. Psychiatry 12:653026. doi: 10.3389/fpsyt.2021.653026
Received: 13 January 2021; Accepted: 10 May 2021;
Published: 02 June 2021.
Edited by:
Nevena V. Radonjic, Upstate Medical University, United StatesReviewed by:
Bashkim Kadriu, National Institute of Mental Health, National Institutes of Health (NIH), United StatesCopyright © 2021 Dong, Grunebaum, Lan, Wagner, Choo, Milak, Sobeih, Mann and Kantrowitz. This is an open-access article distributed under the terms of the Creative Commons Attribution License (CC BY). The use, distribution or reproduction in other forums is permitted, provided the original author(s) and the copyright owner(s) are credited and that the original publication in this journal is cited, in accordance with accepted academic practice. No use, distribution or reproduction is permitted which does not comply with these terms.
*Correspondence: Joshua T. Kantrowitz, amszMzgwQGN1bWMuY29sdW1iaWEuZWR1
Disclaimer: All claims expressed in this article are solely those of the authors and do not necessarily represent those of their affiliated organizations, or those of the publisher, the editors and the reviewers. Any product that may be evaluated in this article or claim that may be made by its manufacturer is not guaranteed or endorsed by the publisher.
Research integrity at Frontiers
Learn more about the work of our research integrity team to safeguard the quality of each article we publish.