- 1Department of Psychiatry and Weill Institute for Neurosciences, University of California, San Francisco, San Francisco, CA, United States
- 2Department of Psychology, Stanford University, Stanford, CA, United States
- 3Center for Neuroscience, University of Pittsburgh, Pittsburgh, PA, United States
- 4Department of Radiology, Stanford University, Stanford, CA, United States
- 5Center for Depression, Anxiety, and Stress Research, McLean Hospital and Harvard Medical School, Belmont, MA, United States
- 6Department of Epidemiology and Biostatistics, University of California, San Francisco, San Francisco, CA, United States
- 7Department of Microbiology and Immunology, Stanford University, Stanford, CA, United States
Animal models of stress and related conditions, including depression, have shown that elevated peripheral levels of inflammatory cytokines have downstream consequences on glutamate (Glu) in the brain. Although studies in human adults with depression have reported evidence of higher inflammation but lower Glu in the anterior cingulate cortex (ACC), the extent to which peripheral inflammation contributes to glutamatergic abnormalities in adolescents with depression is not well-understood. It is also unclear whether antioxidants, such as ascorbate (Asc), may buffer against the effects of inflammation on Glu metabolism. Fifty-five depressed adolescents were recruited in the present cross-sectional study and provided blood samples, from which we assayed pro-inflammatory cytokines, and underwent a short-TE proton magnetic spectroscopy scan at 3T, from which we estimated Glu and Asc in the dorsal ACC. In the 31 adolescents with usable cytokine and Glu data, we found that IL-6 was significantly positively associated with dorsal ACC Glu (β = 0.466 ± 0.199, p = 0.029). Of the 16 participants who had usable Asc data, we found that at higher levels of dorsal ACC Asc, there was a negative association between IL-6 and Glu (interaction effect: β = −0.906 ± 0.433, p = 0.034). Importantly, these results remained significant when controlling for age, gender, percentage of gray matter in the dorsal ACC voxel, BMI, and medication (antidepressant and anti-inflammatory) usage. While preliminary, our results underscore the importance of examining both immune and neural contributors to depression and highlight the potential role of anti-inflammatory compounds in mitigating the adverse effects of inflammation (e.g., glutamatergic neuroexcitotoxicity). Future studies that experimentally manipulate levels of inflammation, and of ascorbate, and that characterize these effects on cortical glutamate concentrations and subsequent behavior in animals and in humans are needed.
Introduction
Depression is an impairing and prevalent disorder that commonly emerges during adolescence (1, 2). While there is growing evidence that elevated inflammation, measured by peripheral levels of pro-inflammatory cytokines and proteins, are implicated in the development and maintenance of depression in adults [(3, 4); for reviews see also (5, 6)], the role of these immune markers in adolescent depression is unclear [although see (7, 8) for recent research in non-depressed adolescents]. It is well recognized both that life stress is one of the most potent risk factors for depression during adolescence (9–11), and that the effects of stress on the immune and nervous systems are critical in understanding the onset and persistence of depression. Extensive research has shown that psychosocial stressors activate the immune system by initiating a cascade of inflammatory responses, including increased production of pro-inflammatory cytokines in the peripheral nervous system (12). Importantly, peripheral cytokines can cross and alter the permeability of the blood-brain barrier (13) and influence processes that affect glutamate metabolism through a variety of mechanisms, including, but not limited to, increased production of quinolinic acid-which binds to glutamatergic NMDAreceptors and provokes release of glutamate into the synapse-and the impediment of astrocyte reuptake of glutamate (5, 12, 14, 15). Indeed, preclinical data indicate that inflammatory cytokines stimulate glutamate release that, over time, leads to cell apoptosis and to damage to oligodendrocytes (16, 17). Thus, glutamatergic excitotoxicity may be one mechanism by which inflammatory cytokines effect depression-related changes both in the brain and in behavior (18).
Consistent with this framework, investigators have conducted studies in adults of translocator protein 18 kDA (TSPO)-positron emission topography (PET) binding that index microglial activation by measuring TSPO expression; these researchers report higher TSPO binding in the ACC in patients with depression compared to healthy controls (19, 20). Neuroinflammation and mediators of the immune response (e.g., microglia) in the central nervous system cannot be measured non-invasively; however, technologies such as proton magnetic resonance spectroscopy (MRS) can be used to measure non-invasively the downstream effects of peripheral cytokines on levels of neurotransmitters, including glutamate. Studies using MRS to examine depression-related alterations in glutamate have primarily focused on the anterior cingulate cortex [ACC; for reviews and meta-analyses, see (21, 22)], a large brain region with distinct subdivisions (23). While these recent reviews report that the published literature thus far has identified lower glutamate in the ACC in depressed individuals compared to healthy controls, these patterns have been equivocal in the nine MRS studies conducted with depressed adolescents. Prior studies have focused primarily on adults with depression, in which measures of glutamate are often confounded by medication usage, chronicity of illness, and age-related effects on overall brain development (e.g., thinner gray matter) that likely complicate the nature of how, and the extent to which, inflammation affects glutamate in the context of MDD. Importantly, with the exception of one study (24), all MRS studies of depressed adolescents conducted to date have each recruited fewer than 17 depressed adolescents (22), and many have acquired spectral data on MR scanners at 1.5 Tesla (22), which have poorer signal-to-noise ratios and more limited resolution for resolving spectral resonances than do scanners at 3 Tesla.
The present study was designed to examine the role of glutamatergic abnormalities in adolescent depression by testing associations between peripheral levels of inflammation, indexed by pro-inflammatory cytokines, and levels of Glu in the dorsal ACC (dACC) in clinically depressed adolescents scanned at 3 Tesla. Our focus on the dACC is motivated in part by previous studies that have identified functional and structural abnormalities in this division of the ACC in depressed vs. non-depressed adolescents. From a neurodevelopmental perspective, the dACC has been shown to be a key region in which there are dramatic changes in connectivity with limbic and cortical regions that underlie affective processing and cognitive control during adolescence (25, 26). Thus, it is likely that insults in the form of psychosocial stress and psychiatric disease have a profound impact on dACC development and, subsequently, on psychosocial skills associated with adaptive emotion regulation (23, 27). Indeed, our group has reported that depressed adolescents exhibit lower dACC network connectivity than do psychiatrically healthy controls and, further, that dACC network connectivity is associated with an earlier age of depression onset (28). Together, these results suggest that the dACC is a critically important region to examine in understanding the neurobiological substrates of adolescent depression.
An exploratory aim of the study was to examine the role of antioxidants in a model of inflammatory contributions to glutamatergic metabolism in adolescent depression. Basic researchers have begun to report that antioxidants, such as ascorbate (Asc, also known as Vitamin C), buffer against the neurotoxic effects of excessive glutamate in neurons, and may serve a neuroprotective role against glutamatergic excitotoxicity (29–32). These findings, however, have yet to be replicated in humans.
To address these gaps, we recruited 55 clinically depressed adolescents who underwent short-TE MRS scans that permitted modeling of Glu and Asc resonances at 3 Tesla, and who provided blood samples from which we assayed inflammatory cytokines. We tested whether peripheral levels of inflammation were positively associated with glutamate concentrations in the dorsal ACC, and explored whether levels of ascorbate in the dACC moderated the associations between peripheral levels of inflammation and dACC glutamate.
Methods and Materials
Participants
Fifty-five adolescents between the ages of 13 and 18 years were recruited from the San Francisco Bay Area community as part of a longitudinal study examining neurobiological mechanisms underlying adolescent stress and depression (18). We interviewed participants and their caregiver at an initial session to assess study eligibility (see below) using the Kiddie Schedule for Affective Disorders and Schizophrenia–Present and Lifetime [K-SADS-PL; (33, 34)], the Children's Depressive Rating Scale–Revised [CDRS-R; (35)], and the Family Interview for Genetics Studies [FIGS; (36)]. Eligible participants were invited to complete additional questionnaires, and to complete an MRI scan and provide blood samples at a subsequent session held ~2 weeks after the initial baseline session (interval: 10.85 ± 6.08 days). Inclusion criteria for potentially depressed adolescents included being 13–18 years of age, being fluent in English, and having a depressive disorder (Major Depressive Disorder, Dysthymia, or Depressive Disorder Not Otherwise Specified) based on a combination of the K-SADS-PL and CDRS-R (i.e., t-scores ≥55 or raw scores ≥30) for those who did not meet full criteria for MDD or Dysthymia in the K-SADS-PL screening, provided that the participant also endorsed at least 2 symptoms of MDD or Dysthymia in the K-SADS-PL screening [see (18) for more details]. Exclusion criteria included meeting lifetime or current criteria for Mania, Psychosis, or Alcohol Dependence (based on DSM-IV) or Moderate Substance Use Disorder with substance-specific threshold for withdrawal (based on DSM-V), premenarchal status (for females), history of concussion within the past 6 weeks or history of any concussion with loss of consciousness, contraindications for MRI scanning (e.g., braces, metal implants, or claustrophobia), and any serious neurological or intellectual disorders that could interfere with the ability to complete study components. The study was approved by the Institutional Review Boards (IRBs) at Stanford University and the University of California, San Francisco. All participants and their legal guardian(s) gave written assent and informed consent, respectively, in accordance with the Declaration of Helsinki, and were compensated for their participation.
Depression Symptom Severity
Trained research assistants administered the CDRS-R to participants and their parents/legal guardians as a measure of depression symptom severity. The CDRS-R is a clinician-rated scale composed of 17 questions; both participants and caregivers were administered the first 14 questions to assess depressive symptomology, while the last 3 questions were rated based on the interviewer's observation of the adolescent participant to assess non-verbal characteristics of depression including depressed affect, listless speech, and hypoactivity. Interviewers integrated responses from both parent and child interviews to produce summary items ratings, which were then summed to attain a total summary score for each participant. All item ratings were discussed by a subset of the co-authors to ensure consistency across interviewers to maximize reliability and validity. The CDRS-R is the most widely used rating scale in clinical research trials for assessing the severity of depression and change in depressive symptoms in children and adolescents with depression. While there are no thresholds for distinguishing mild, moderate, and severe levels of depression beyond the cutoff score of 30 that is used to determine depression and the score of ≤28 that is used to determine remission, there is evidence that scores of 35–40 indicate mild depression (37). In our full sample, 37 participants (67.3%) met the clinical cutoff score of 40 on the CDRS-R, 15 participants (27.3%) scored 35–40, 2 participants (3.6%) scored 28–35, and 1 participant (1.8%) had a score <28. In our final analytic sample with usable ACC Glu and cytokine data, 20 participants (64.5%) had scores higher than 40 and 11 participants (35.5%) scored 35–40; no participants had scores <35.
Pro-inflammatory Cytokines
Peripheral levels of pro-inflammatory cytokines were assayed from dried blood spot (DBS) samples. Blood samples were collected using mini contact-activated lancets (BD 366594 Microtainer, BD Biosciences, San Jose, CA) that were used to prick the participant's finger from their non-dominant hand after running their hand under hot water for 2 min. Blood spots were collected on 1.3 cm filter paper cards (Whatman #903, GE Healthcare, Piscataway, NJ) with ~150–250 μL amount of blood per spot; spots were then dried overnight at room temperature before being transferred to Ziplock bags with a desiccant for storage in a −20°C freezer. Sample extraction and Luminex analysis was performed by the Human Immune Monitoring Center at Stanford University. Samples were extracted and diluted 3-fold in the Luminex assay buffer prior to being run on a 62-plex Procarta plex assay (Thermo Fisher, Santa Clara, CA) on the Luminex FlexMap 3D. Custom Assay Chex control beads were added to all wells (Radix Biosolutions, Georgetown, Texas). Data were analyzed using MasterPlex software (Hitachi Software Engineering America Ltd., MiraiBio Group). Both median fluorescence intensity (MFI) and calculated concentration values (in pg/mL) were estimated for each analyte. Based on prior work demonstrating advantages of using MFI over concentration values for low abundant analytes from multiplex assays (38), we conducted all analyses using median fluorescence intensity (MFI) values (log-transformed). To correct for non-specific binding, we employed ordinary non-linear least squares (ONLS) regression by regressing cytokine values (log MFI) on CHEX4 values; the resulting residualized scores were thus used in subsequent statistical analyses (39). To minimize multiple comparisons, we focused our analyses on the pro-inflammatory cytokines IL-1β, IL-6, TNF-α, as these cytokines have been previously linked with depression (4, 7, 8, 40–43) and have been shown to be assayed reliably from DBS (44, 45). Of the 55 participants, 1 participant did not return after their initial assessment, and 1 did not feel comfortable providing a blood sample. Of the 53 participants who provided blood samples, 6 had samples that were collected and assayed differently (for the purposes of piloting protocols), and 9 did not provide sufficient blood volume for the assay.
MR Scanning Acquisition
All MRI scans were acquired at the Stanford Center for Cognitive and Neurobiological Imaging (CNI) with a 3T GE Discovery MR750 (General Electric Healthcare, Milwaukee, WI, USA) and Nova 32-channel head coil (Nova Medical, Wilmington, MA, USA). Participants completed a T1-weighted anatomical scan based on the GE BRAVO IR-prep, fast spoiled gradient (SPGR) sequence: TR/TE/TI = 8.2/3.2/600 ms; flip angle = 12°; 156 axial slices; FOV = 256 mm; matrix = 256 mm × 256 mm, voxel resolution = 1 × 1 × 1 mm3; total scan time = 3 min 40 s. Participants also completed proton MRS scans based on a modification of the GE Healthcare PRESS product sequence, PROBE-p™. Two features were added to the product PROBE-p™ sequence for improved localization: (1) 16 step phase cycling (EXORCYCLE on the two refocusing RF pulses) and (2) application of a sensitive point echo planar (EP) waveform during acquisition to further eliminate out-of-slice artifact in the logical z direction (46–48). The bandwidth of the CHESS RF water-suppression pulses was reduced from 150 to 75 Hz to avoid suppression of the ascorbate peak at 4.1 ppm. We performed single voxel spectroscopy using this sequence with TE/TR = 35/2,000 ms, 128 averages, total scan time = 5 min 4 s on the graphically prescribed region of the dACC based on the 3D T1-weighted anatomical images using anatomical landmarks (all prescriptions confirmed by TCH).
MR Spectral Processing
Concentrations of Glu, Asc, and other metabolites in the dACC were quantified and expressed as ratios to total creatine (i.e., creatine and phosphocreatine) levels using LCModel (49), which models in vivo spectrum as a linear combination of basis in vitro spectra from individual metabolites. Experimental Asc basis spectrum was acquired using the same sequence as for the in vivo studies from a custom built 50 mM Asc spherical phantom with pH of 7.2 at 37°C in an otherwise synthetic basis set to improve accuracy, due to the complexity of the Asc resonances and their dependence on temperature. Previous studies have indicated that Asc can be reliably measured from the human brain at 3 Tesla (50); independent data from our group also indicate that Asc at physiological concentrations can be reliably estimated from LCModel using this method at 3 Tesla with a mean Cramer-Rao Lower Bound (CRLB) of 16% in cortical gray matter (51). We used CRLB, a measure of the reliability of the fit, with a quality criterion set at ≤35% for each individual metabolite. All T1-weighted MR images were run through FreeSurfer 6.0 [(52); http://surfer.nmr.mgh.harvard.edu/fswiki/recon-all] to perform tissue segmentation and to estimate percentage of gray matter, white matter, and cerebrospinal fluid in the dACC voxel. For all statistical analyses involving Glu or Asc, we included percentage of gray matter in the prescribed dACC voxel as a covariate (see below). See Figure 1 for exemplary spectra from a representative subject. Of the 55 participants, 1 participant did not return after their initial assessment and 2 did not feel comfortable completing the MR scan. Of the 52 participants who completed the MRS scans, 44 provided usable data for Glu and 19 for Asc, based on CRLB criteria.
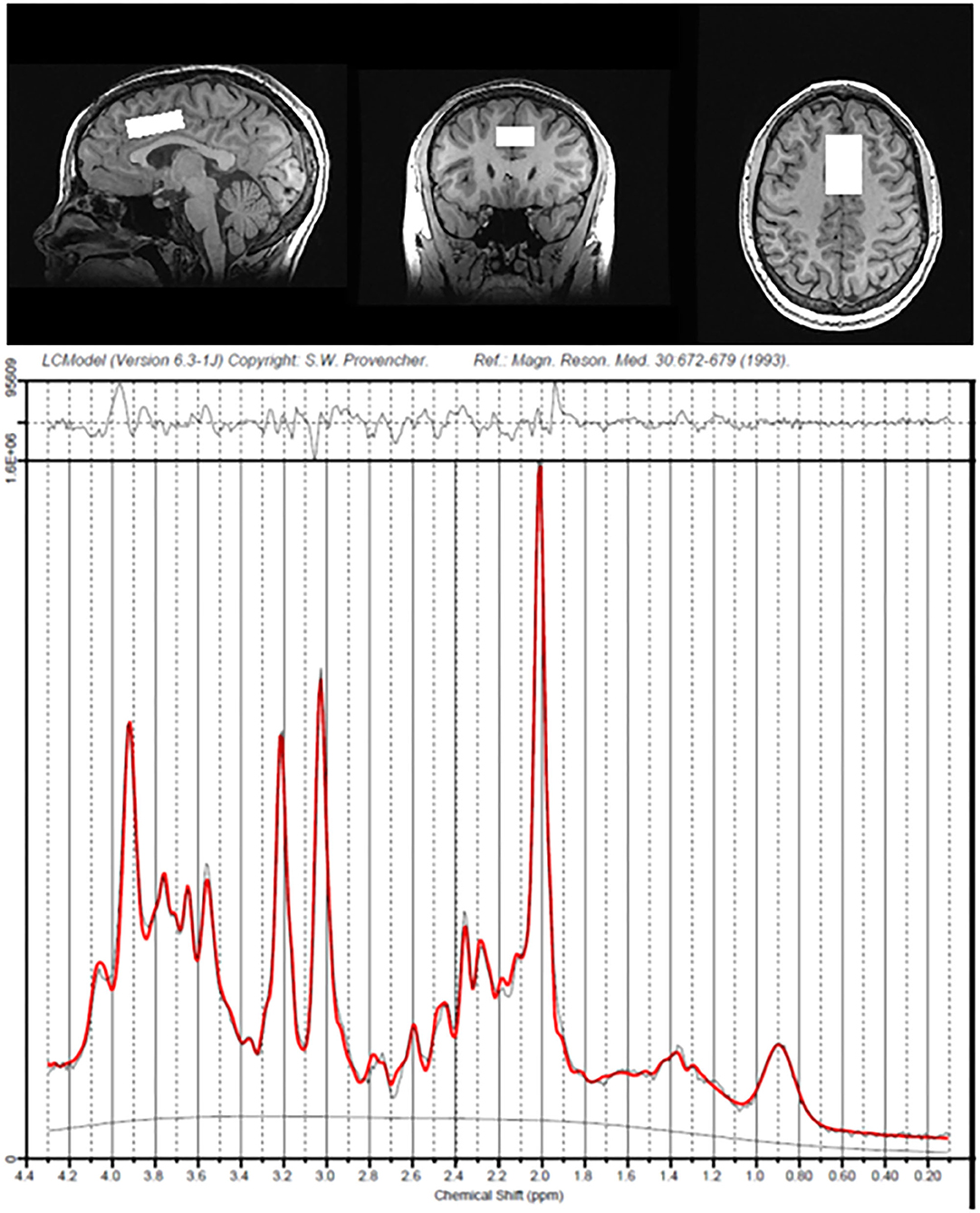
Figure 1. Voxel placement of dorsal anterior cingulate cortex and spectra data from a representative participant.
Statistical Approach
All analyses were conducted in R (version 3.5.3; R Core Team). All associations between main variables of interest were tested using Pearson's correlations. We conducted multiple linear regressions to test whether baseline levels of pro-inflammatory cytokines were associated with concentrations of Glu in the dACC and whether levels of Asc in the dACC moderated any of these associations. To probe significant interaction effects, we applied the Johnson-Neyman procedure for continuous predictors using sim_slopes in R. In all statistical models, we covaried for age, gender (male, female, non-binary), psychotropic medication use (coded as a dichotomous variable), percentage of gray matter in the dACC voxel, Body Mass Index (BMI), and anti-inflammatory medication usage (coded as a dichotomous variable). We selected these covariates based on previous work identifying potential influences of these factors on both peripheral cytokine levels and MRS-based estimates of neurometabolites. To estimate standardized coefficient weights (β), all predictor and response variables were z-scored.
Code Availability
Scripts for conducting functional clustering and statistical analyses can be found at: https://github.com/tiffanycheingho/TIGER/.
Results
Demographic and Clinical Characteristics
Demographic and clinical characteristics of participants at baseline are presented in Table 1. Of the 55 participants enrolled in the study, 11 did not provide usable Glu data, 36 did not provide usable Asc data, and 17 did not provide usable blood data. We compared the 16 participants who were not missing any data on any measure to the 39 participants who were missing data on at least one measure of interest on baseline demographic and clinical characteristics. None of the effect sizes of the differences were statistically significant (all ps > 0.081) and all were small or negligible, with the exception of highest level of parental education, which had a large effect size [Cramer's ϕ = 0.586, = 17.193, p < 0.01].
Descriptive statistics of the main variables of interest are presented in Table 2. A correlation matrix of the primary predictors and outcomes of interest, covariates, and clinical characteristics is presented in Figure 2. As expected, the inflammatory cytokines of interest were all highly intercorrelated (all rs > 0.67, all ps < 0.0001). IL-6 was positively correlated with Glu in the dACC (r = 0.41, p = 0.02), and both IL-6 and TNF-α were negatively correlated with Asc in the dACC (both rs < −0.54, both ps < 0.03). Finally, age of study assessment was associated with age of onset for current depressive episode (r = 0.46, p < 0.001).
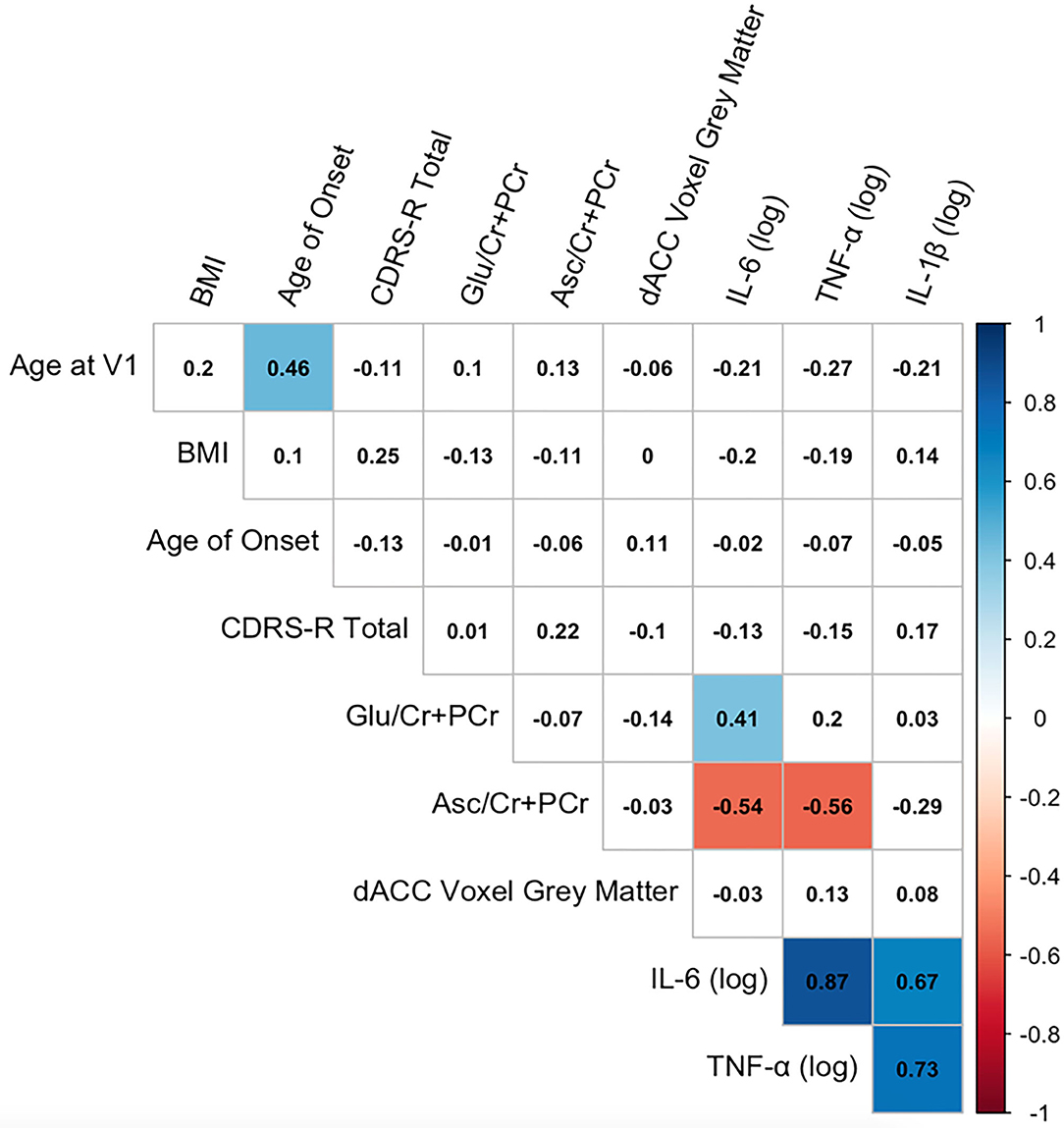
Figure 2. Correlation matrix among key variables of interest. Correlation matrix of primary predictor and outcome variables of interest, covariates, and clinical characteristics. Pearson correlation coefficients are presented in black text. Significant correlations at p < 0.05 (uncorrected) are highlighted in color (blue, positive correlations; red, negative correlations).
Higher Levels of IL-6 Are Associated With Higher Concentrations of Glutamate in the Dorsal Anterior Cingulate Cortex
Thirty-one participants provided both usable cytokine and Glu data. Levels of IL-6 were significantly positively associated with concentrations of dACC Glu (β = 0.466 ± 0.199, t22 = 2.339, p = 0.029, ΔR2 = 0.199). Levels of IL-1β and TNF-α were not significantly associated with concentrations of Glu in the dorsal ACC (all ps > 0.288). See Figure 3 for more details.
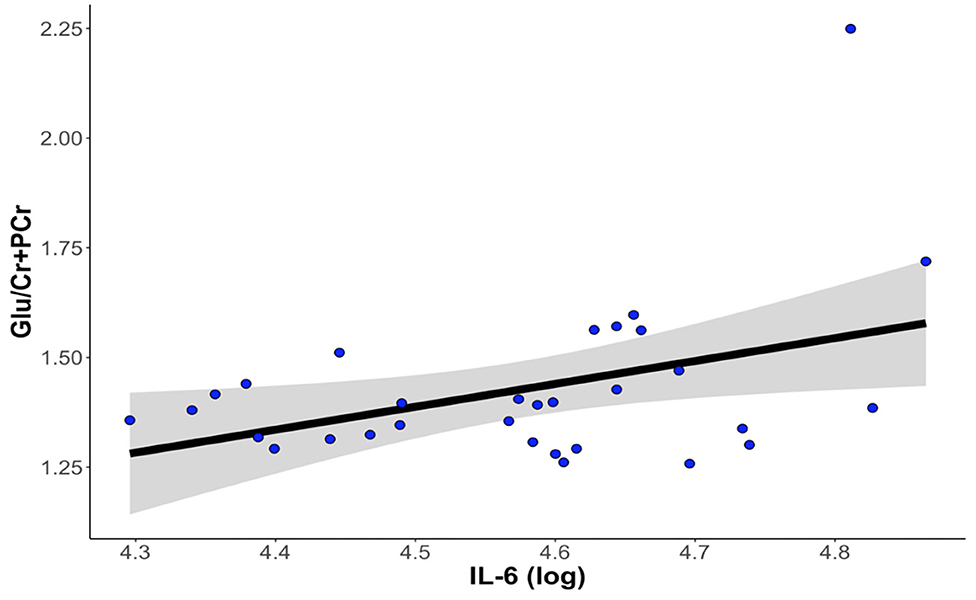
Figure 3. Higher levels of IL-6 at baseline are associated with higher levels of Glu in the dorsal anterior cingulate cortex. The correlation between IL-6 and dACC Glu remained statistically significant after controlling for age, gender, percentage of gray matter volume in the dACC voxel, antidepressant medication usage, and anti-inflammatory medication usage. dACC, dorsal anterior cingulate cortex; Glu, glutamate; IL-6, interleukin-6.
One participant who exhibited abnormally high levels of Glu in the dACC was a statistical outlier despite having adequate CRLB for estimates of Glu. Although winsorizing the outlier data (based on a median-unbiased estimator interpolated from the sample without strict distribution assumptions, see quantile in R) did not change the significance of the association between IL-6 and dACC Glu (p < 0.05), removal of the outlier changed the statistical significance (p = 0.235). The associations between IL-1β and TNF-α with dACC Glu remained non-significant regardless of the treatment of the outlier (all ps > 0.397 when winsorizing, all ps > 0.764 when removing the statistical outlier).
Exploring the Moderating Role of Ascorbate in the Dorsal Anterior Cingulate Cortex on the Association Between IL-6 and Glutamate in the Dorsal Anterior Cingulate Cortex
Sixteen participants provided usable cytokine, Glu, and Asc data. Higher levels of Asc in the dorsal ACC moderated the associations between levels of IL-6 and Glu in the dorsal ACC, even after accounting for age, gender, BMI, percentage of gray matter in the voxel, and antidepressant and anti-inflammatory medication usage (interaction effect: β = −0.906 ± 0.433, t6 = −2.74, p = 0.034, ΔR2 = 0.557). We used the Johnson-Neyman procedure to compute the values of dACC Asc where the linear correlation between IL-6 and dACC Glu was statistically significant. We found that whereas Asc levels lower than 0.16 were associated with a significantly positive correlation between IL-6 and Glu, Asc levels higher than 0.32 were associated with a significantly negative correlation between IL-6 and Glu; Asc values between 0.16 and 0.32 were not associated with significant correlations between IL-6 and Glu. See Figure 4 for more details.
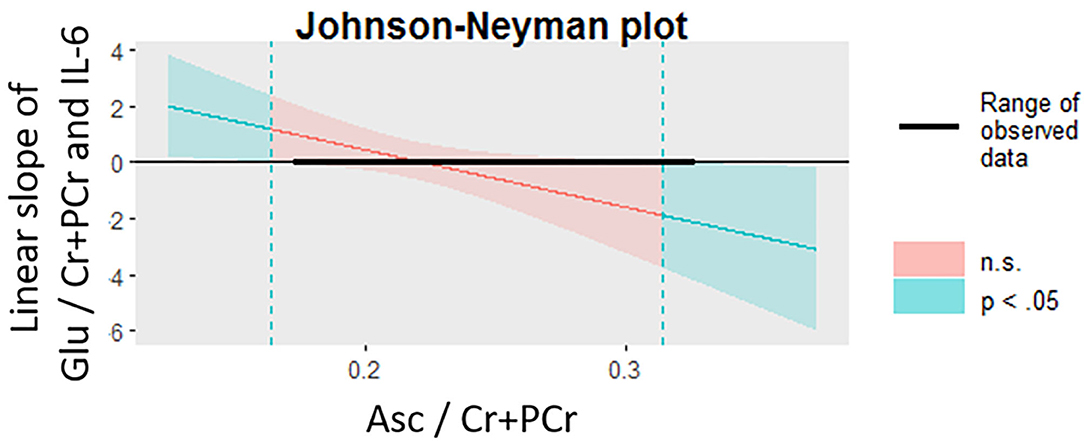
Figure 4. Levels of Asc in the dACC moderate the association between IL-6 and glutamate in the dACC. The Johnson-Neyman procedure was used to probe the interaction effect of dACC Asc and IL-6 on dACC Glu. At lower levels of Asc (<0.16, indicated by dotted teal line, left), the correlation between IL-6 and Glu was significantly positive (p < 0.05) whereas at higher levels of Asc (>0.32, indicated by dotted teal line, right), the correlation between IL-6 and Glu was significantly negative (p < 0.05). Asc, ascorbate; dACC, dorsal anterior cingulate cortex; Glu, glutamate; IL-6, interleukin-6.
Discussion
The present study was designed to examine, in depressed adolescents, associations between peripheral levels of inflammation, as indexed by pro-inflammatory cytokines, and concentrations of glutamate (Glu) in the dorsal anterior cingulate cortex (dACC), a region that has been implicated in adolescent depression in a range of structural and functional MRI studies but that has been relatively neglected in studies using MRS. We found that levels of IL-6, but not of IL-1β or TNF-α, were positively associated with concentrations of Glu in the dACC. In an exploratory analysis, we also found that higher concentrations of Asc in the dACC moderated the association between IL-6 and concentrations of Glu in the dACC, such that these markers were negatively correlated at higher levels of Asc only.
Our primary finding that higher levels of inflammatory cytokines, specifically IL-6, are associated with higher concentrations of glutamate in the dACC significantly advances our understanding of glutamatergic abnormalities in adolescent depression, particularly given the sparse and conflicting research in this area. First, our results are consistent with animal models demonstrating the role of inflammatory cytokines in contributing to glutamatergic excitotoxicity (12, 18); they appear to be inconsistent, however, with findings from MRS studies indicating lower concentrations of Glu in the ACC of depressed adults (22). One explanation for this discrepancy involves the specific region examined in this study. We focused on the dorsal division of the ACC based on findings of previous studies that abnormalities in this region may be a neurodevelopmentally sensitive marker of adolescent depression (28), in contrast to the rostral (pregenual) or medial ACC (22). Studies focused on the rostral and medial ACC in depressed adolescents, however, have found evidence of higher Glx (sum of glutamate and glutamine, a precursor to glutamate and GABA) in depressed adolescents with suicidal ideation than in healthy controls (53), as well as reductions in the ratio of glutamine to glutamate (Gln/Glu) in response to treatment-related symptom improvement (54). Although these studies examined Glx and Gln/Glu rather than solely Glu, their data are nonetheless consistent with our findings that higher levels of Glu may contribute to depressive symptomatology. Thus, a second explanation of the discrepancy between our findings and those of previous studies is that higher levels of Glu in the ACC may characterize depression that occurs at earlier developmental stages, when the consequences of chronically high levels of glutamate on brain structure (e.g., cell apoptosis, cortical thinning, etc.) have not yet had as large an impact. Future research with larger samples that include both adolescents and adults and that image the distinct portions of the ACC are needed to test these hypotheses more explicitly and to determine whether higher Glu in the ACC is an adolescent-specific indicator of depression.
Our finding that the positive association IL-6 and Glu in the dACC was found only at lower levels of Asc is broadly consistent with basic science work showing that Asc prevents excitotoxic damage to cells by inhibiting the binding of Glu to NMDA receptors and by limiting the effects of oxidative stress markers, including quercetin (29–32). Other studies have also shown that Asc is pharmacologically effective against glutamate-induced phosphorylation of AMPK, a mechanism of neuronal cell death (55). While preliminary, these results highlight the potential role of antioxidants in mitigating the downstream effects of inflammation on the brain, including glutamate metabolism. Indeed, several studies have reported that ascorbic acid supplements produce an antidepressant (and not anxiolytic, suggesting specificity) effect [for a review, see (56)]. The low toxicity and high tolerance of Asc may make Asc a possible adjuvant to first-line antidepressant treatments. An important direction of future research will be to test the extent to which anti-inflammatory strategies—including pharmacological agents, psychosocial therapies targeting stress reduction, or lifestyle and behavioral changes (e.g., changes in sleep, exercise, and/or diet)—affect levels of Asc in the brain and whether they help to reduce or prevent depression in adolescents.
Although the effect size of the association between IL-1β as well as TNF-α and Glu in the dACC was in the same direction as that of IL-6 and Glu, it is notable that we detected effects that were specific to IL-6. IL-1β, IL-6, and TNF-α are similar in their role in mediating immune responses; all three are released into circulation by innate immune cells to act on organs throughout the body [e.g., signaling the release of acute phase reactants such as C-reactive protein; (57)]. All three have also been shown to alter glutamate production (58, 59); in particular, TNF-α, has been strongly linked with glutamate-mediated oxidative stress and impairment of glutamate reuptake from astrocytes (59). Importantly, however, in vivo studies conducted with rodents have found that chronic stress paradigms that upregulate levels of IL-6 do so in the absence of increases in IL-1b and, further, that administration of IL-6 specifically produces depressive-like phenotypes in rodents (60). In vivo studies in human adolescents who are not clinically depressed have also showed that IL-6 predicts subsequent changes in depressive symptoms, although the extent of these effects appears to be conditional on other factors, including sex, stressful life events, and time or, possibly, developmental stage (7, 61). Our findings are consistent with much of the emerging human research in adults (12, 14, 15), by showing that in depressed adolescents higher levels of IL-6 specifically are associated with higher levels of glutamate. Nevertheless, it is critical that future studies with larger sample sizes replicate our findings and reconcile important differences between humans and animals in neuroimmune systems in order to facilitate forward and backward translation.
There are several limitations of our investigation that warrant additional discussion and that should be addressed in future research. First, our sample size was relatively small with a high degree of psychiatric comorbidity and medication use (including agents with some anti-inflammatory effects); it is important to note, however, that our study is one of the largest MRS studies to date conducted with depressed adolescents and the first to focus on the dACC (22), and also includes a sample that is representative of the type of patients who often present at clinics. Second, we did not exclude participants on the basis of medication history or usage. While we included both antidepressant and anti-inflammatory medications as statistical covariates in all of our analyses, it is possible that the effects of these medications nevertheless influenced our results. Bearing in mind issues concerning the generalizability of the findings (for example, many depressed adolescents seek treatment and are medicated), it will be important for future studies to consider recruiting unmedicated adolescents with depression. Third, we did not assess healthy control participants in our study. Future research will benefit from including psychiatrically healthy adolescents to clarify the extent to which the associations we observed are specific to adolescents who are depressed. Fourth, the reliability of Asc at 3 Tesla was significantly worse than the reliability of Glu; the advent of ultra-field MR imaging (e.g., 7 Tesla) may prove to be especially fruitful in improving detection and quantification of Asc. Finally, our study was a naturalistic observational study, which precluded our ability to make causal interpretations of the associations among IL-6, Glu, Asc, and depressive symptoms. Studies are needed that experimentally manipulate levels of inflammation, and of ascorbate, and that characterize these effects on cortical glutamate concentrations and subsequent behavior in samples of both depressed and non-depressed adolescents.
Despite these limitations, this study is important in establishing the associations among peripheral levels of inflammation and concentrations of glutamate and ascorbate in the dACC in humans with depression. In a cohort of depressed adolescents, we found that higher levels of IL-6 were associated with higher concentrations of Glu in the dACC and, further, that higher concentrations of Asc in the dACC moderated this effect, such that the positive association between IL-6 and Glu in the dACC was present only at lower levels of Asc. Although preliminary, our results underscore the importance of examining both immune and neural contributors to depression in adolescence and highlight the potential role of anti-inflammatory compounds in mitigating the adverse effects of inflammation (e.g., glutamatergic neuroexcitotoxicity) in depressed adolescents.
Data Availability Statement
The datasets used and analyzed during the current study will be made available by the corresponding author upon reasonable request.
Ethics Statement
The studies involving human participants were reviewed and approved by Institution Review Board at Stanford University. Written informed consent to participate in this study was provided by the participants' legal guardian/next of kin.
Author Contributions
TH designed the research. GT, JS, AO, and JW helped perform research. TH, GT, JS, AO, and YR-H analyzed the data. MG, DS, MS, FJ, YR-H, and HM provided additional analytic tools to assist with data analysis. TH and IG obtained funding support. All authors contributed to the writing of the manuscript.
Funding
This research was supported by the National Institutes of Health (K01MH117442 to TH, R37MH101495 to IG), the Klingenstein Third Generation Foundation (Child and Adolescent Depression Fellow Award to TH), the Stanford Maternal Child and Health Institute (Early Career Award and K Support Award to TH), the Stanford Center for Cognitive and Neurobiological Imaging Center (Seed Grant to TH), and the Ray and Dagmar Dolby Family Fund (to TH). The funding agencies played no role in the design and conduct of the study; collection, management, analysis, and interpretation of the data; and preparation, review, or approval of the manuscript.
Conflict of Interest
The authors declare that the research was conducted in the absence of any commercial or financial relationships that could be construed as a potential conflict of interest.
Acknowledgments
We thank Anna Cichocki, Miranda Edwards, Holly Pham, Michelle Sanabria, Lucinda Sisk, Alexess Sosa, and Rachel Weisenburger for assistance with data collection and organization. Finally, we thank the participants and their families for contributing to this study.
References
1. World Health Organization. Depression and Other Common Mental Disorders: Global Health Estimates. World Health Organization (2017).
2. Breslau J, Gilman SE, Stein BD, Ruder T, Gmelin T, Miller E. Sex differences in recent first-onset depression in an epidemiological sample of adolescents. Transl Psychiatry. (2017) 7:e1139. doi: 10.1038/tp.2017.105
3. Huang M, Su S, Goldberg J, Miller AH, Levantsevych OM, Shallenberger L, et al. Longitudinal association of inflammation with depressive symptoms: a 7-year cross-lagged twin difference study. Brain Behav Immun. (2019) 75:200–7. doi: 10.1016/j.bbi.2018.10.007
4. Mac Giollabhui N, Ng TH, Ellman LM, Alloy LB. The longitudinal associations of inflammatory biomarkers and depression revisited: systematic review, meta-analysis, and meta-regression. Mol Psychiatry. (2020). doi: 10.1038/s41380-020-00867-4. [Epub ahead of print].
5. Miller AH, Raison CL. The role of inflammation in depression: from evolutionary imperative to modern treatment target. Nat Rev Immunol. (2016) 16:22. doi: 10.1038/nri.2015.5
6. Dooley LN, Kuhlman KR, Robles TF, Eisenberger NI, Craske MG, Bower JE. The role of inflammation in core features of depression: Insights from paradigms using exogenously-induced inflammation. Neurosci Biobehav Rev. (2018) 94:219–37. doi: 10.1016/j.neubiorev.2018.09.006
7. Moriarity DP, Mac Giollabhui N, Ellman LM, Klugman J, Coe CL, Abramson LY, et al. Inflammatory proteins predict change in depressive symptoms in male and female adolescents. Clin Psychol Sci. (2019) 7:754–67. doi: 10.1177/2167702619826586
8. Moriarity DP, Kautz MM, Mac Giollabhui N, Klugman J, Coe CL, Ellman LM, et al. Bidirectional associations between inflammatory biomarkers and depressive symptoms in adolescents: potential causal relationships. Clin Psychol Sci. (2020) 8:690–703. doi: 10.1177/2167702620917458
9. Hammen C. Adolescent depression: stressful interpersonal contexts and risk for recurrence. Curr Direct Psychol Sci. (2009) 18:200–4. doi: 10.1111/j.1467-8721.2009.01636.x
10. LeMoult J, Humphreys KL, Tracy A, Hoffmeister JA, Ip E, Gotlib IH. Meta-analysis: exposure to early life stress and risk for depression in childhood and adolescence. J Am Acad Child Adolesc Psychiatry. (2019) 59:842–55. doi: 10.1016/j.jaac.2019.10.011
11. Patton GC, Coffey C, Posterino M, Carlin JB, Bowes G. Life events and early onset depression: cause or consequence? Psychol Med. (2003) 33:1203–10. doi: 10.1017/S0033291703008626
12. Miller RJ, Jung H, Bhangoo SK, White FA. Cytokine and chemokine regulation of sensory neuron function. Handb Exp Pharmacol. (2009) 417–49. doi: 10.1007/978-3-540-79090-7_12
13. Banks WA. Characteristics of compounds that cross the blood-brain barrier. BMC Neurol. (2009) 9(Suppl. 1):S3. doi: 10.1186/1471-2377-9-S1-S3
14. Haroon E, Miller AH, Sanacora G. Inflammation, glutamate, and glia: a trio of trouble in mood disorders. Neuropsychopharmacology. (2017) 42:193–215. doi: 10.1038/npp.2016.199
15. Haroon E, Miller AH. Inflammation effects on brain glutamate in depression: mechanistic considerations and treatment implications. Curr Top Behav Neurosci. (2017) 31:173–98. doi: 10.1007/7854_2016_40
16. Káradóttir R, Attwell D. Neurotransmitter receptors in the life and death of oligodendrocytes. Neuroscience. (2007) 145:1426–38. doi: 10.1016/j.neuroscience.2006.08.070
17. Matute C. Glutamate and ATP signalling in white matter pathology. J Anat. (2011) 219:53–64. doi: 10.1111/j.1469-7580.2010.01339.x
18. Walker JC, Teresi GI, Weisenburger RL, Segarra JR, Ojha A, Kulla A, et al. Study protocol for teen inflammation glutamate emotion research (TIGER). Front Hum Neurosci. (2020) 14:414. doi: 10.3389/fnhum.2020.585512
19. Richards EM, Zanotti-Fregonara P, Fujita M, Newman L, Farmer C, Ballard ED, et al. PET radioligand binding to translocator protein (TSPO) is increased in unmedicated depressed subjects. EJNMMI Res. (2018) 8:57. doi: 10.1186/s13550-018-0401-9
20. Setiawan E, Attwells S, Wilson AA, Mizrahi R, Rusjan PM, Miler L, et al. Association of translocator protein total distribution volume with duration of untreated major depressive disorder: a cross-sectional study. Lancet Psychiatry. (2018) 5:339–47. doi: 10.1016/S2215-0366(18)30048-8
21. Luykx JJ, Laban KG, van den Heuvel MP, Boks MP, Mandl RC, Kahn RS, et al. Region and state specific glutamate downregulation in major depressive disorder: a meta-analysis of (1)H-MRS findings. Neurosci Biobehav Rev. (2012) 36:198–205. doi: 10.1016/j.neubiorev.2011.05.014
22. Moriguchi S, Takamiya A, Noda Y, Horita N, Wada M, Tsugawa S, et al. Glutamatergic neurometabolite levels in major depressive disorder: a systematic review and meta-analysis of proton magnetic resonance spectroscopy studies. Mol Psychiatry. (2019) 24:952–64. doi: 10.1038/s41380-018-0252-9
23. Stevens FL, Hurley RA, Taber KH. Anterior cingulate cortex: unique role in cognition and emotion. J Neuropsychiatry Clin Neurosci. (2011) 23:121–5. doi: 10.1176/jnp.23.2.jnp121
24. Gabbay V, Mao X, Klein RG, et al. Anterior cingulate cortexγ-aminobutyric acid in depressed adolescents: relationship to anhedonia. Arch Gen Psychiatry. (2012) 69:139–49. doi: 10.1001/archgenpsychiatry.2011.131
25. Fair DA, Dosenbach NU, Church JA, Cohen AL, Brahmbhatt S, Miezin FM, et al. Development of distinct control networks through segregation and integration. Proc Natl Acad Sci USA. (2007) 104:13507–12. doi: 10.1073/pnas.0705843104
26. Power JD, Fair DA, Schlaggar BL, Petersen SE. The development of human functional brain networks. Neuron. (2010) 67:735–48. doi: 10.1016/j.neuron.2010.08.017
27. Lichenstein SD, Verstynen T, Forbes EE. Adolescent brain development and depression: a case for the importance of connectivity of the anterior cingulate cortex. Neurosci Biobehav Rev. (2016) 70:271–87. doi: 10.1016/j.neubiorev.2016.07.024
28. Ho TC, Sacchet MD, Connolly CG, Margulies DS, Tymofiyeva O, Paulus MP, et al. Inflexible functional connectivity of the dorsal anterior cingulate cortex in adolescent major depressive disorder. Neuropsychopharmacology. (2017) 42:2434–45. doi: 10.1038/npp.2017.103
29. Ballaz S, Morales I, Rodríguez M, Obeso JA. Ascorbate prevents cell death from prolonged exposure to glutamate in an in vitro model of human dopaminergic neurons. J Neurosci Res. (2013) 91:1609–17. doi: 10.1002/jnr.23276
30. Majewska MD, Bell JA. Ascorbic acid protects neurons from injury induced by glutamate and NMDA. Neuroreport. (1990) 1:194–6. doi: 10.1097/00001756-199011000-00004
31. Sandstrom MI, Rebec GV. Extracellular ascorbate modulates glutamate dynamics: role of behavioral activation. BMC Neurosci. (2007) 8:1–6. doi: 10.1186/1471-2202-8-32
32. Kocot J, Luchowska-Kocot D, Kiełczykowska M, Musik I, Kurzepa J. Does vitamin C influence neurodegenerative diseases and psychiatric disorders? Nutrients. (2017) 9:659. doi: 10.3390/nu9070659
33. Kaufman J, Birmaher B, Brent D, Rao U, Flynn C, Moreci P, et al. Schedule for affective disorders and schizophrenia for school-age children-present and lifetime version (K-SADS-PL): initial reliability and validity data. J Am Acad Child Adolesc Psychiatry. (1997) 36:980–8. doi: 10.1097/00004583-199707000-00021
34. Kaufman J, Birmaher B, Brent DA, Ryan ND, Rao U. K-SADS-PL. J Am Acad Child Adolesc Psychiatry. (2000) 39:1208. doi: 10.1097/00004583-200010000-00002
35. Poznanski EO, Grossman JA, Buchsbaum Y, Banegas M, Freeman L, Gibbons R. Preliminary studies of the reliability and validity of the children's depression rating scale. J Am Acad Child Psychiatry. (1984) 23:191–7. doi: 10.1097/00004583-198403000-00011
36. Maxwell ME. Family Interview for Genetic Studies (FIGS): A Manual for FIGS. Bethesda, MD: Clinical Neurogenetics Branch, Intramural Research Program, National Institute of Mental Health (1992).
37. Mayes TL, Bernstein IH, Haley CL, Kennard BD, Emslie GJ. Psychometric properties of the children's depression rating scale-revised in adolescents. J Child Adolesc Psychopharmacol. (2010) 20:513–6. doi: 10.1089/cap.2010.0063
38. Breen EJ, Tan W, Khan A. The statistical value of raw fluorescence signal in luminex xMAP based multiplex immunoassays. Sci Rep. (2016) 6:26996. doi: 10.1038/srep26996
39. Maecker HT, Rosenberg-Hasson Y, Kolstad KD, Steen VD, Chung LS. A novel utility to correct for plate/batch/lot and nonspecific binding artifacts in luminex data. J Immunol. (2020) 204:3425–33. doi: 10.4049/jimmunol.2000017
40. Howren MB, Lamkin DM, Suls J. Associations of depression with c-reactive protein, IL-1, and IL-6: a meta-analysis. Psychosomat Med. (2009) 71:171–86. doi: 10.1097/PSY.0b013e3181907c1b
41. Liu Y, Ho RCM, Mak A. Interleukin (IL)-6, tumour necrosis factor alpha (TNF-α) and soluble interleukin-2 receptors (sIL-2R) are elevated in patients with major depressive disorder: a meta-analysis and meta-regression. J Affect Dis. (2012) 139:230–9. doi: 10.1016/j.jad.2011.08.003
42. Raison CL, Rutherford RE, Woolwine BJ, Shuo C, Schettler P, Drake DF, et al. A randomized controlled trial of the tumor necrosis factor antagonist infliximab for treatment-resistant depression: The role of baseline inflammatory biomarkers. Archiv Gen Psychiatry. (2013) 70:31–41. doi: 10.1001/2013.jamapsychiatry.4
43. Valkanova V, Ebmeier KP, Allan CL. CRP, IL-6 and depression: a systematic review and meta-analysis of longitudinal studies. J Affect Dis. (2013) 150:736–44. doi: 10.1016/j.jad.2013.06.004
44. Miller EM, Mcdade TW. A highly sensitive immunoassay for interleukin-6 in dried blood spots. Am J Hum Biol. (2012) 24:863–5. doi: 10.1002/ajhb.22324
45. Skogstrand K, Thysen AH, Jørgensen CS, Rasmussen EM, Andersen ÅB, Lillebaek T, et al. Antigen-induced cytokine and chemokine release test for tuberculosis infection using adsorption of stimulated whole blood on filter paper and multiplex analysis. Scand J Clin Lab Invest. (2012) 72:204–11. doi: 10.3109/00365513.2011.649014
46. Bodenhausen G, Freeman R, Turner DL. Suppression of artifacts in two-dimensional J spectroscopy. J Magnet Res. (1977) 27:511–4. doi: 10.1016/0022-2364(77)90016-6
47. Gu M, Hurd R, Noeske R, Baltusis L, Hancock R, Sacchet MD, et al. GABA editing with macromolecule suppression using an improved MEGA-SPECIAL sequence. Magnet Res Med. (2018) 79:41–7. doi: 10.1002/mrm.26691
48. Webb PG, Sailasuta N, Kohler SJ, Raidy T, Moats RA, Hurd R. Automated single-voxel proton MRS: technical development and multisite verification. Magnet Res Med. (1994) 31:365–73. doi: 10.1002/mrm.1910310404
49. Provencher SW. Automatic quantitation of localized in vivo 1H spectra with LCModel. NMR Biomed. (2001) 14:260–4. doi: 10.1002/nbm.698
50. Shih Y, Buchert M, Chung HW, Jurgen H, Von Elverfeldt D. Vitamin C estimation with standard 1H spectroscopy using a clinical 3T MR system: detectability and reliability within the human brain. J Magnet Res Imaging. (2008) 28:351–8. doi: 10.1002/jmri.21466
51. Gu M, Hurd RE, Spielman DM. Quantification of Glutathione and Asocrbate in the human Brain Using Short-TE PRESS. International Society of Magnetic Resonance in Medication (2019). Available online at: https://index.mirasmart.com/ISMRM2019/PDFfiles/2230.html (accessed February 01, 2021).
52. Fischl B, Salat DH, Busa E, Albert M, Dieterich M, Haselgrove C, et al. Whole brain segmentation: automated labeling of neuroanatomical structures in the human brain. Neuron. (2002) 33:341–55. doi: 10.1016/S0896-6273(02)00569-X
53. Lewis CP, Port JD, Blacker CJ, Sonme AI, Seewoo BJ, Leffler JM, et al. Altered anterior cingulate glutamatergic metabolism in depressed adolescents with current suicidal ideation. Transl Psychiatry. (2020). 10, 1–12. doi: 10.1038/s41398-020-0792-z
54. Croarkin PE, Nakonezny PA, Wall CA, Murphy LL, Sampson SM, Frye MA, et al. Transcranial magnetic stimulation potentiates glutamatergic neurotransmission in depressed adolescents. Psychiatr Res Neuroimaging. (2016) 247:25–33. doi: 10.1016/j.pscychresns.2015.11.005
55. Shah SA, Yoon GH, Kim HO, Kim MO. Vitamin C neuroprotection against dose-dependent glutamate-induced neurodegeneration in the postnatal brain. Neurochem Res. (2015) 40:875–84. doi: 10.1007/s11064-015-1540-2
56. Moritz B, Schmitz AE, Rodrigues ALS, Dafre AL, Cunha MP. The role of vitamin C in stress-related disorders. J Nutr Biochem. (2020) 85(Suppl. 1):108459. doi: 10.1016/j.jnutbio.2020.108459
57. Cray C, Zaias J, Altman NH. Acute phase response in animals: a review. Comp Med. (2009) 59:517–2.
58. Nelson TE, Ur CL, Gruol DL. Chronic interleukin-6 exposure alters electrophysiological properties and calcium signaling in developing cerebellar purkinje neurons in culture. J Neurophysiol. (2002) 88:475–86. doi: 10.1152/jn.2002.88.1.475
59. Liao SL, Chen CJ. Differential effects of cytokines and redox potential on glutamate uptake in rat cortical glial cultures. Neurosci Lett. (2001) 299:113–6. doi: 10.1016/S0304-3940(01)01499-9
60. Rizzo SS, Neal SJ, Hughes ZA, Beyna M, Rosenzweig-Lipson S, Moss SJ, et al. Evidence for sustained elevation of IL-6 in the CNS as a key contributor of depressive-like phenotypes. Transl Psychiatry. (2012) 2:e199. doi: 10.1038/tp.2012.120
61. Kautz MM, Coe CL, McArthur BA, Mac Giollabhui N, Ellman LM, Abramson LY, et al. Longitudinal changes of inflammatory biomarkers moderate the relationship between recent stressful life events and prospective symptoms of depression in a diverse sample of urban adolescents. Brain Behav Immun. (2020) 86:43–52. doi: 10.1016/j.bbi.2019.02.029
Keywords: glutamate, depression, magnetic resonance spectroscopy, interleukin-6, ascorbate
Citation: Ho TC, Teresi GI, Segarra JR, Ojha A, Walker JC, Gu M, Spielman DM, Sacchet MD, Jiang F, Rosenberg-Hasson Y, Maecker H and Gotlib IH (2021) Higher Levels of Pro-inflammatory Cytokines Are Associated With Higher Levels of Glutamate in the Anterior Cingulate Cortex in Depressed Adolescents. Front. Psychiatry 12:642976. doi: 10.3389/fpsyt.2021.642976
Received: 18 December 2020; Accepted: 26 February 2021;
Published: 15 April 2021.
Edited by:
Marcio Gerhardt Soeiro-de-Souza, University of São Paulo, BrazilReviewed by:
Gabriele Ende, University of Heidelberg, GermanySophie E. Holmes, Yale University, United States
Copyright © 2021 Ho, Teresi, Segarra, Ojha, Walker, Gu, Spielman, Sacchet, Jiang, Rosenberg-Hasson, Maecker and Gotlib. This is an open-access article distributed under the terms of the Creative Commons Attribution License (CC BY). The use, distribution or reproduction in other forums is permitted, provided the original author(s) and the copyright owner(s) are credited and that the original publication in this journal is cited, in accordance with accepted academic practice. No use, distribution or reproduction is permitted which does not comply with these terms.
*Correspondence: Tiffany C. Ho, tiffany.ho@ucsf.edu