- 1Department of Behavioural Neuroscience, Institute of Normal and Pathological Physiology, Centre of Experimental Medicine, Slovak Academy of Sciences, Bratislava, Slovakia
- 2Social, Cognitive and Affective Neuroscience Unit, Department of Cognition, Emotion, and Methods in Psychology, Faculty of Psychology, University of Vienna, Vienna, Austria
Deficits in the gating of sensory stimuli, i.e., the ability to suppress the processing of irrelevant sensory input, are considered to play an important role in the pathogenesis of several neuropsychiatric disorders, in particular schizophrenia. Gating is disrupted both in schizophrenia patients and their unaffected relatives, suggesting that gating deficit may represent a biomarker associated with a genetic liability to the disorder. To assess the strength of the evidence for the etiopathogenetic links between genetic variation, gating efficiency, and schizophrenia, we carried out a systematic review of human genetic association studies of sensory gating (suppression of the P50 component of the auditory event-related brain potential) and sensorimotor gating (prepulse inhibition of the acoustic startle response). Sixty-three full-text articles met the eligibility criteria for inclusion in the review. In total, 117 genetic variants were reported to be associated with gating functions: 33 variants for sensory gating, 80 variants for sensorimotor gating, and four variants for both sensory and sensorimotor gating. However, only five of these associations (four for prepulse inhibition—CHRNA3 rs1317286, COMT rs4680, HTR2A rs6311, and TCF4 rs9960767, and one for P50 suppression—CHRNA7 rs67158670) were consistently replicated in independent samples. Although these variants and genes were all implicated in schizophrenia in research studies, only two polymorphisms (HTR2A rs6311 and TCF4 rs9960767) were also reported to be associated with schizophrenia at a meta-analytic or genome-wide level of evidence. Thus, although gating is widely considered as an important endophenotype of schizophrenia, these findings demonstrate that evidence for a common genetic etiology of impaired gating functions and schizophrenia is yet unsatisfactory, warranting further studies in this field.
Introduction
Sensory and sensorimotor gating are conceptualized as basic cognitive processes that regulate the processing of sensory input by the brain. It has been suggested that gating represents a filtering mechanism, preventing distraction and sensory overload, or a protective mechanism, securing uninterrupted processing of stimuli (1–4). Importantly, it has been further postulated that disrupted gating may contribute to information processing deficits, cognitive fragmentation, and thought disorder, the hallmark feature of schizophrenia psychosis (5–8).
Sensory gating is routinely examined by measuring the electroencephalographic event-related potentials (ERPs) during a paired-pulse paradigm (7). The paradigm comprises trials with two identical auditory stimuli of the same intensity, a conditioning stimulus (S1) and a testing stimulus (S2), that are presented successively with an interstimulus interval of 500 ms (9, 10). Auditory stimuli elicit an ERP, which is characterized by a positive peak ~40–90 ms after stimulus onset, known as P50 wave. It has been suggested that response to S1 triggers an inhibitory mechanism that results in a reduced amplitude of the P50 wave after the presentation of S2. The diminution of the P50 wave to S2 relative to that elicited by S1, called P50 suppression or P50 gating, is the operational definition of sensory gating (7, 10, 11). Other well-established, but less commonly assessed, measures of sensory gating include the suppression of the N100 and P200 ERP waves (12, 13). The most widely used measure of sensorimotor gating, on the other hand, is prepulse inhibition (PPI) of the acoustic startle reflex. During the PPI paradigm, the presentation of a sudden and intense auditory startling stimulus (pulse) is preceded (usually 30–120 ms) by a weaker non-startling stimulus (prepulse). This leads to a reduction in the startle reflex also known as PPI. In humans, PPI is commonly quantified by measuring the eye-blink component of the startle reflex using electromyography of the periocular muscles (14–16).
Both PPI and P50 gating are robustly reduced in schizophrenia spectrum disorders [e.g., (17–20)], but also several other psychiatric conditions, in particular, bipolar disorder and obsessive–compulsive disorder [e.g., (21–28), for review see e.g., (29, 30)]. Deficits in PPI and P50 gating were reported not only in psychiatric patients but also in their unaffected first-degree relatives [(19, 31–33), for a recent review of PPI studies see ref. (34)]. Several studies have demonstrated a significant heritability of these measures, ranging 29–58% for PPI and 10–68% for P50 gating (31, 35–42). Given these attributes, including a high test–retest reliability, PPI and P50 suppression deficits are considered as important endophenotypes of neuropsychiatric disorders (20, 37), i.e., intermediate phenotypes (or markers) that are associated with disorders but are simpler in terms of the genetic and neurobiological architecture (43–45). Endophenotypes represent an important approach to deal with the complexity and polygenic nature of mental disorders such as schizophrenia. It is supposed that studying the genetic architecture of endophenotypes and their relationship with biological processes impaired in neuropsychiatric disorders may contribute to a better understanding of the underlying pathophysiology [e.g., (46)]. The genetic basis of gating in humans has been intensively studied over the last decades, and it has become apparent that a significant genetic component is involved in both PPI and P50 suppression. Despite an extensive and rapidly growing body of literature on the relationship between genotype and gating in humans, the underlying genetic architecture of these endophenotypes remains elusive due to fragmentary evidence and lack of verification. Recently, Quednow et al. (47) carried out a systematic review (and a meta-analysis) of human association studies of PPI (sensorimotor gating). However, a similar assessment of sensory gating studies is lacking, as is an integrative review of genetic determinants of both sensory and sensorimotor gating functions. The aim of this work was thus to evaluate current knowledge regarding the etiopathogenetic links between genetic variation, gating efficiency, and schizophrenia. For this purpose, we carried out a systematic review of published genetic association studies assessing the relationship between genetic variation and the efficiency of sensory and sensorimotor gating in humans. Furthermore, we critically assessed the reliability of these findings by examining the quality of the studies, the number of replications, and the relative number of positive and negative results. Finally, we evaluated the evidence for genetic mechanisms shared between gating and schizophrenia.
Materials and Methods
Study Design
The review process followed the Preferred Reporting Items for Systematic Reviews and Meta-Analyses (PRISMA) guidelines (48). The Covidence online software (Covidence systematic review software, Veritas Health Innovation, Melbourne, Australia; available at www.covidence.org) was used to facilitate the process of screening, paper selection, and data extraction.
Search Strategy
To identify eligible studies, we performed a systematic, comprehensive search of the published literature using Pubmed and Scopus until October 2019. The electronic databases were searched using the combination of Boolean operators and the following key words: sensorimotor gating, sensory gating, prepulse inhibition, P50, startle, polymorphism, gene and human, among others (for exact search phrases utilized, see Supplementary Data 1). Also, a secondary search of relevant articles was performed by screening the references of included full-text papers.
Inclusion Criteria and Study Selection
To be included in the review a study had to meet the following inclusion criteria: (1) study design of a candidate gene association study (CGAS), genome-wide association study (GWAS), or included these studies as a part of more complex study design (e.g., pharmacogenetic study), (2) study enrolled human subjects (healthy participants or psychiatric patients), (3) study outcomes included sensorimotor gating as indexed by PPI or sensory gating indices such as P50, N100, or P200 suppression, and (4) the report was written in English. Only original research papers were included; other article types, such as reviews, meta-analyses, case reports, editorials, and commentaries, were excluded.
The search results were imported to the Covidence, and after removing duplicates, titles and abstracts of identified studies were independently screened by the first and the second author (RR and DB). At this stage, only irrelevant studies that obviously did not meet the inclusion criteria were excluded from the review. Next, for the remaining potentially eligible papers, the same two authors independently assessed full texts to select only those articles that meet all of the abovementioned inclusion criteria. Any disagreement between the two reviewers was discussed and resolved by consensus. If needed, the third author (IR) was involved to reach a decision.
Data Extraction
The following information was extracted from the selected studies: name of the first author, affiliation of the first author, year of publication, country, study design, sample characteristics (sample size, mean age, sex ratio, race/ethnicity, inclusion/exclusion criteria and population—healthy vs. psychiatric patients), parameters of the auditory stimulation (duration and intensity of acoustic stimuli, background sound intensity, and sound frequency), electrode placement, statistical test used, description of assessed polymorphisms (polymorphism type, reference number/label, chromosomal position, closest gene, reference and minor allele frequency, functional consequence, and association with disorders), and study outcomes of interest (genotype effects on PPI and sensory gating across all samples including p-value, effect size, direction of the effect, mean values, and standard deviations). The data extraction was carried out by DB and RR, working independently and in duplicate, using the Covidence data extraction tool. All data extraction forms from both reviewers were inspected for potential errors and compiled by RR.
Quality Assessment
The quality of genetic studies (Q-Genie) 11-item tool was used to evaluate the quality of all included studies. We opted for this tool since it was specifically developed and validated to facilitate the assessment of the global quality of genetic association studies, and it proved to be valid and reliable for both expert and non-expert raters (49). The quality assessment was conducted by DB and RR, working independently and in duplicate. The final quality score for each study was calculated by averaging the respective scores from the two reviewers. Following the Q-Genie scoring system, scores below 33 indicate poor-quality studies, scores between 33 and 40 indicate studies of moderate quality, and scores above 40 indicate good-quality studies. The degree of interobserver agreement was tested using the Cohen's kappa coefficient (κ), with values categorized as poor (≤0.20), fair (0.21–0.40), moderate (0.41–0.60), substantial (0.61–0.80), and almost perfect agreement (>0.80) (50). The Cohen's kappa coefficient was computed by using the FREQ procedure in SAS Studio software (SAS University Edition, release 3.8, SAS Institute, Cary, NC, USA).
Results
Identification of Relevant Studies
The systematic search yielded 1,820 potentially relevant references. After removing 369 duplicates and 1,326 irrelevant articles identified by screening abstracts, the full texts of the remaining 125 papers were assessed for eligibility. Of them, excluded were 39 studies that did not meet the inclusion criteria and 16 duplicates not captured by Covidence, leaving 70 papers. Based on the Q-Genie scoring system, 41 out of the 70 relevant studies (58.6%) were rated high quality, 22 (31.4%) moderate, and 7 (10.0%) poor. A weighted kappa value of 0.55, 95% CI (0.50–0.60) indicates a moderate agreement between the two raters (DB and RR). Poor-quality studies were excluded from the systematic review due to concerns about the validity of results, leaving 63 eligible papers. No additional papers were identified by screening the references of the included articles. The process of study selection is depicted in the PRISMA flow diagram below (Figure 1).
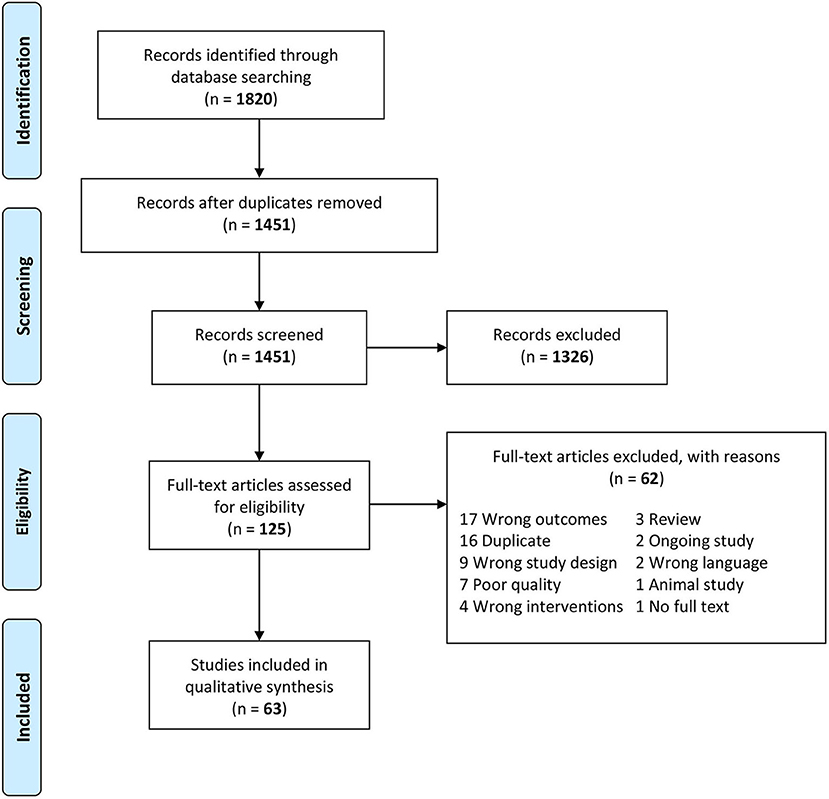
Figure 1. The Preferred Reporting Items for Systematic Reviews and Meta-Analyses (PRISMA) flow diagram of the study selection process (51).
Basic Description of the Included Studies
The final selection included 53 CGAS, 3 GWAS, and seven pharmacogenetic studies (25, 52–113). These studies investigated in total 63 independent sample groups: 36 samples of healthy individuals, 20 patient samples (16 with schizophrenia), and seven samples involving both patients and healthy individuals. Sensorimotor gating (PPI) was assessed in 41 studies, sensory gating (P50 or N100 suppression, for simplicity thereafter referred to as P50 gating) in 18 studies, and four studies assessed both measures. A short summary of the basic characteristics of the studies is provided in Table 1; details for each study included in this review are provided in Supplementary Table 1.
Identification and Description of Genetic Polymorphisms
Data extraction from the eligible studies resulted in the identification of 201 polymorphisms located within or close to 77 genes. Association with PPI was tested for 125 polymorphisms. Among them, 84 variants, within or close to 37 genes, were reported as significantly (p < 0.05) associated with PPI in at least one sample. Association with P50 gating was investigated for 109 polymorphisms, of which 37, located within or close to 13 genes, were significantly associated with this measure in at least one sample. Association with both PPI and P50 gating was investigated in 54 variants and a significant association with both measures was reported for four polymorphisms (COMT rs4680, rs165599, ANKK1 rs1800497, and TCF4 rs9960767). A vast majority of the variants were single nucleotide polymorphisms (SNPs, Table 2; for a detailed summary see Supplementary Tables 2–5).
To provide insight into the involved biological mechanism, we conducted an enrichment analysis using the Gene Ontology Resource (114–116). The associated variants were annotated by dbSNP and clustered based on the overrepresentation of the corresponding genes in the Gene Ontology classification section Biological Processes. The results of this analysis are provided in Table 3. Associations were considered as consistent (reliable) if a significant association with PPI or P50 gating was reported in at least two independent samples, and the number of reported significant associations was higher than the number of null findings. For both PPI and P50 gating, the reported positive associations included several genes involved in neurodevelopmental processes and/or cellular signaling (in particular glutamatergic, dopaminergic, serotoninergic, and cholinergic neurotransmission). However, most of the polymorphisms for which positive associations were reported were explored in only one published study (PPI: 64.3%, P50: 81.1%). Applying our criterion of reliability, only four associations with PPI (CHRNA3 rs1317286, COMT rs4680, HTR2A rs6311, and TCF4 rs9960767) and one with P50 gating (CHRNA7 rs67158670) can be considered as consistent.
Among the polymorphisms positively associated with PPI, 22 (26.2%) are functional variants, i.e., related to the level of gene expression or the biological function of the protein products (as reported in the reviewed studies). For the remaining 62 (73.8%) variants, no direct functional consequences were reported. For P50 gating, 4 (10.8%) polymorphisms positively associated with this measure are functional and 33 (89.2%) are without known functional consequences. To examine the potential functional role of the positively associated variants, we carried out an in silico analysis using the HaploReg resource (117). The results of this analysis showed that a substantial proportion of polymorphisms that were associated with PPI (41 SNPs) and P50 gating (14 SNPs) overlap with regulatory motifs such as promoter/enhancer histone marks or DNase I hypersensitive sites (for a detailed description see Supplementary Tables 2–5).
Discussion
In this paper, we reviewed the available data on the relationship between genetic variability and sensory information filtering in humans. More specifically, we summarized, in a systematic manner, findings from genetic association studies published in peer-reviewed journals, examining the effect of common genetic variants on two well-established parameters of gating functions, PPI and P50/N100 ERP suppression (jointly referred to as P50 gating), deficits of which are considered as schizophrenia endophenotypes. We found that association with PPI or P50 gating was reported for variants located within or near 37 and 13 genes, respectively, which are involved in a variety of biological processes, mostly related to neurotransmission and neurodevelopment. However, most of the polymorphisms positively associated with PPI and/or P50 gating were examined in only one study or were not consistently replicated in other studies. According to our criteria for reliability (i.e., association confirmed in at least two independent samples and positive outcomes outnumbering negative results), only four polymorphisms within four genes for PPI (CHRNA3 rs1317286, COMT rs4680, HTR2A rs6311, and TCF4 rs9960767) and one polymorphism for P50 gating (CHRNA7 rs67158670) can be considered as reliable or consistent across the studies. Two of them (COMT rs4680 and TCF4 rs9960767) were identified as significantly associated with PPI also by Quednow et al. (47), who included 16 independent samples into a meta-analysis. Although a large number of reported associations were with non-coding polymorphisms, our analysis shows that a substantial proportion of them may play a role in gene expression by affecting the binding of transcription factors or chromatin remodeling. However, since enhancers may activate transcription of their target genes over considerable distances, up to hundreds or even thousands of kilobases, caution should be taken when making inferences about the functional connection between non-coding variants in these regions and target genes (118).
Replication of Association Results
From the 35 associations that were tested in more than one study, only 10 polymorphisms were reported to be significantly associated with gating in two or more studies. Low statistical power in some studies could increase the probability of false-negative results and unsuccessful replications. Although we excluded studies whose quality was evaluated as poor according to the Q-Genie scoring system, yet in 12 of 63 studies that fulfilled the criteria to be included in this review, sample size was lower than 50. Furthermore, a considerable number of negative replication results (14 of 30) come from samples that differed in ethnicity compared to the initial studies reporting positive results. Notably, in addition to genetic diversity, difference in startle response and PPI across ethnic groups (119) could decrease the number of successful replications. On the other hand, the non-replications seem not to be due to diversity in stimulation parameters since these did not substantially differ between almost all studies that had yielded discrepant outcomes. In the light of considerable heritability of gating functions (31, 35–42), the low number of reliably assessed genetic associations clearly indicates that, despite the relatively large number of genetic studies, current knowledge on the genetic architecture of gating functions remains very limited. Next, we will focus our discussion on the variants/genes consistently associated with sensory and/or sensorimotor gating functions.
Catechol-O-Methyltransferase
Catechol-o-methyltransferase (COMT) is an enzyme degrading catecholamines. A single nucleotide G-A substitution at codon 158 results in a change from valine to methionine (Val158Met) causing a missense mutation with a lower metabolic activity of the enzyme. This polymorphism significantly affects dopamine turnover in the prefrontal cortex (PFC, Val allele associated with reduced PFC dopamine levels), PFC activity, and executive functions in healthy humans [for review see e.g., (120, 121)]. Numerous studies reported COMT Val158Met polymorphism to be related with liability to schizophrenia and several other mental disorders, but a recent meta-analysis did not confirm a significant association with schizophrenia (122). The Val allele was associated with weaker PPI in six of seven studies included in our review. In agreement with these reports, a study by Giakoumaki et al. (63) has shown that administration of a COMT inhibitor tolcapone increased PPI in Val allele carriers. As highlighted by the meta-analysis by Quednow et al. (47), the association of PPI with COMT Val158Met polymorphism is stronger in men than in women. Interestingly, a similar pattern of sex-dependent effects of this variation was also reported for response inhibition and linked with the activity of the PFC [(123), see also (124)]. Given the putative role of the PFC in the modulation of sensorimotor gating (125–128), it could be speculated that the prefrontal circuitry is also involved in the sex-specific effects of COMT genotype on PPI, which remains to be established in future studies. The evidence for the association of COMT Val158Met with P50 gating is less consistent, as a significant association was reported in seven and non-significant in nine studied samples.
Another COMT polymorphism, rs165599, has not fulfilled our reliability criteria but was reported to be significantly associated with both PPI and P50 gating (only in one study each). Functional consequences of this variation are less clear, although there is evidence indicating its relationship with COMT mRNA levels in the brain of healthy humans, and IQ and the presence of psychotic symptoms in patients with 22q11 deletion syndrome (129, 130). A large case-control study reported its association with schizophrenia in women but not in men, suggesting that this SNP confers a sex-specific genetic component in schizophrenia (131). Notably, rs165599 and rs4680 are both part of a three-marker haplotype (together with rs2075507) that has been implicated in COMT protein level, PFC function in obsessive–compulsive disorder and attention-deficit hyperactivity disorder (129, 132, 133). This haplotype was significantly associated with P50 in a sample of patients with bipolar disorder (but not in healthy controls) (25). Its relationship with PPI has not been studied yet, as far as we are informed.
To sum up, there is considerable evidence that genetic variability of COMT affects gating functions, which fits with the proposed role of dopamine in the PFC (134). Interestingly, however, disruption of PPI following administration of dopamine agonists in rodents has been attributed to modulation of striatal rather than cortical circuitry (135, 136). In humans, on the other hand, the effects of dopamine agonists on PPI are less evident and reliable (137). Given the importance of PPI to study the neurobiology of schizophrenia in animal models, it would be desirable in future studies to shed more light on the specific roles of dopamine in cortical and striatal processing related to gating in humans and rodents.
Serotonin 2A Receptor
The HTR2A gene encodes a G-protein-coupled serotonin 2A receptor (5-HT2AR). In humans, 5-HT2AR is widely expressed throughout the brain with particularly high density in the neocortex (138). 5-HT2AR has been implicated in multiple brain functions such as learning, memory, and cognition [for review see (139)]. Importantly, several lines of evidence implicate 5-HT2AR in the pathophysiology of psychiatric disorders. First, genetic variants in the HTR2A gene and functional abnormalities of 5-HT2AR are associated with many psychiatric disorders including schizophrenia [for review see (140)]. Second, 5-HT2AR antagonists produce antipsychotic and antidepressant-like effects, whereas agonists have psychotomimetic properties including PPI-disruptive effects (140, 141). HTR2A rs6311 (also known as −1438A/G) is a functional SNP, which lies upstream of the HTR2A promoter region and alters its activity (142). Meta-analyses confirmed the association of this polymorphism with schizophrenia and obsessive–compulsive disorder (143–145). Given the involvement of serotonin in multiple neurobiological processes, warranted are further studies of the role of 5-HT2AR in gating and its relationship with schizophrenia.
Nicotinic Acetylcholine Receptor
A lot of research implicates signaling via nicotinic acetylcholine receptor (nAChR) in gating, schizophrenia, and nicotine dependence [for review see (146)]. It is well established in rodents and humans that the agonist of nAChR nicotine enhances PPI and P50 gating [for review see (147)]. In humans, sensorimotor gating efficiency was found to be inversely related to nicotine dependence (148). Smoking and nicotine dependence are highly prevalent in schizophrenia, and it has been proposed that tobacco is used by the patients as self-medication to alleviate the symptoms, in particular the impairment of cognitive functions (149). Moreover, recent research indicates that nicotine dependence and schizophrenia may share a part of their genetic liability [for review see (150)]. Across the reviewed studies, consistent associations were reported between PPI and variation in CHRNA3 gene as well as between P50 gating and CHRFAM7A gene.
CHRNA3 is a part of a CHRNA5–CHRNA3–CHRNB4 gene cluster on chromosome 15 (15q25 region), encoding α5, α3, and β4 subunits of the nAChR, linked in previous studies to nicotine dependence as well as schizophrenia (151–153). Our search specifically points to CHRNA3 rs1317286, which was reported to be associated with nicotine dependence in a GWAS (154). The analysis using HaploReg indicates that this SNP overlaps with enhancer histone marks and may thus play a role in CHRNA3 transcription. However, due to high linkage disequilibrium, it is difficult to determine causative variants in the CHRNA5–CHRNA3–CHRNB4 cluster, which is under complex and coordinated regulatory control (155). Interestingly, TCF4 (see below) has been identified as one of the regulators of gene expression at this locus (156).
CHRFAM7A is a partial duplication of a gene encoding α7 nAChR, CHRNA7. Translation of CHRFAM7A is low, but it seems to negatively regulate α7 nAChR function [for review see (157)]. The P50 gating-associated polymorphism of CHRFAM7A denoted as rs67158670 (or CHRFAM7AΔ2bp) is a 2-bp deletion in exon 6. This mutation causes a frameshift in translation, resulting in a truncated protein, which is even a more potent inhibitor of α7 nAChR (157). Reduced expression of CHRNA7 was found in the frontal cortex of schizophrenia patients post-mortem (158) and smoking counteracts this deficit (159). In addition to the association with P50 gating, studies reported association of CHRFAM7AΔ2bp with schizophrenia, bipolar disorder, and episodic memory [for review see (157)]. The impact of CHRFAM7AΔ2bp on brain development is debated, and research in this direction could bring new discoveries of the pathomechanistic links between gating deficits and schizophrenia.
Transcription Factor 4
The TCF4 gene codes for a basic helix–loop–helix protein, transcription factor 4, which belongs to a subclass of transcriptional regulators termed E-proteins. E-proteins bind to a specific promoter element known as the Ephrussi-box (E-box) to regulate transcription of target genes in various tissues including the brain [for review see (160)]. Although the precise physiological function of TCF4 is not yet fully understood, a recent study demonstrated that binding sites for TCF4 are present in a large number of genes involved in nervous system development, ion transport, and signal transduction (156). Moreover, this study also showed that TCF4 binding sites are found in many susceptibility genes implicated in common neurodevelopmental disorders including schizophrenia and autism spectrum disorders. Notably, several SNPs in TCF4 itself have been directly linked to schizophrenia, underscoring the possible role of this gene in schizophrenia pathogenesis (161). Our analysis points to a reliable association of TCF4 rs9960767 with PPI. Notably, Quednow et al. (90) reported that the effect of this polymorphism on PPI is moderated by smoking behavior, which fits with the regulatory role of TCF4 on the CHRNA5–CHRNA3–CHRNB4 cluster (156). The association of this variation with schizophrenia was confirmed at a meta-analytic and genome-wide level [for review see (162)]. TCF4 rs9960767 is located within intron 3 of the TCF4 gene and has no direct obvious functional consequences. Neither is there evidence of its linkage disequilibrium with other common non-synonymous polymorphisms or causal variants, which alter TCF4 mRNA expression in adult human brain (163). Williams et al. (163) suggested that rs9960767 may exert effects on TCF4 expression in a developmental context. Our findings support this notion as the HaploReg analysis indicates that rs9960767 may affect putative binding sites of transcription factors Foxa and STAT in the brain germinal matrix, which plays a critical role during brain development. All these findings indicate that sensorimotor gating deficit is a constituent of the neurodevelopmental insult, which is assumed to play a crucial role in the pathogenesis of schizophrenia (164).
Common Genetic Factors of Gating Functions
Four polymorphisms out of 45 variants studied so far were reported to be significantly associated with both PPI and P50 gating. Given our criteria of reliability, however, none of these associations was reliable for both measures. Evidence for common genetic mechanisms underlying both sensory and sensorimotor gating thus remains elusive. Although sensory and sensorimotor gating represent related concepts, the hallmark of which is inhibition, the relationship between PPI and P50 suppression is not fully understood. Correlation between the magnitude of PPI and P50 suppression seems weak since most studies found no significant relationship between the two measures (165–171). Furthermore, PPI primarily relies on the processing in the brainstem and the basal ganglia, which is modulated by the cerebral cortex (125–128, 135, 172–176), while the sources of P50 ERP and P50 suppression are thought to be localized predominantly in the hippocampus, the temporal and the frontal lobes (167, 177–179). Given the importance of PPI and P50 gating in psychiatry, further research is warranted to clarify the relationship between these two phenomena in more detail at both the cognitive/psychological and neurobiological levels.
Conclusion
Our review identified a considerable number of genetic variants associated with PPI or P50 gating in previous studies. However, a critical evaluation of the reports shows associations of only five polymorphisms (four for PPI and one for P50 gating) as consistently replicated across the studies. From these, only two variants (HTR2A rs6311 and TCF4 rs9960767, both associated with PPI) also show a reliable association with schizophrenia (meta-analytic or genome-wide evidence). Although deficits in sensory and sensorimotor gating are widely considered as important endophenotypes of schizophrenia, the evidence for the common genetic etiology of the impaired gating functions and schizophrenia thus remains limited, and further large-scale studies are warranted to advance our understanding of this complex problem.
Data Availability Statement
The original contributions presented in the study are included in the article/Supplementary Materials. Further inquiries can be directed to the corresponding author.
Author Contributions
IR provided the concept and design of the study. DB and RR collected and analyzed the data. RR, IR, and DB wrote and revised the manuscript. All authors contributed to the article and approved the submitted version.
Conflict of Interest
The authors declare that the research was conducted in the absence of any commercial or financial relationships that could be construed as a potential conflict of interest.
Acknowledgments
We thank Bronislava Brejová for assistance with enrichment analysis.
Supplementary Material
The Supplementary Material for this article can be found online at: https://www.frontiersin.org/articles/10.3389/fpsyt.2020.550225/full#supplementary-material
References
1. Freedman R, Adler LE, Gerhardt GA, Waldo M, Baker N, Rose GM, et al. Neurobiological studies of sensory gating in schizophrenia. Schizophr Bull. (1987) 13:669–78. doi: 10.1093/schbul/13.4.669
2. McGhie A, Chapman J. Disorders of attention and perception in early schizophrenia. Br J Med Psychol. (1961) 34:103–16. doi: 10.1111/j.2044-8341.1961.tb00936.x
3. Graham FK. The more or less startling effects of weak prestimulation. Psychophysiology. (1975) 12:238–48. doi: 10.1111/j.1469-8986.1975.tb01284.x
4. Blumenthal TD. Presidential address 2014: the more-or-less interrupting effects of the startle response. Psychophysiology. (2015) 52:1417–31. doi: 10.1111/psyp.12506
5. Geyer MA, Braff DL. Startle habituation and sensorimotor gating in schizophrenia and related animal models. Schizophr Bull. (1987) 13:643–68. doi: 10.1093/schbul/13.4.643
6. Braff DL. Gating and habituation of the startle reflex in schizophrenic patients. Arch Gen Psychiatry. (1992) 49:206. doi: 10.1001/archpsyc.1992.01820030038005
7. Braff DL, Light GA. The use of neurophysiological endophenotypes to understand the genetic basis of schizophrenia. Dialogues Clin Neurosci. (2005) 7:125−35. doi: 10.31887/DCNS.2005.7.2/dlbraff
8. Braff DL, Geyer MA. Sensorimotor gating and schizophrenia. Arch Gen Psychiatry. (1990) 47:181. doi: 10.1001/archpsyc.1990.01810140081011
9. Arnfred SM, Chen ACN. Exploration of somatosensory P50 gating in schizophrenia spectrum patients: reduced P50 amplitude correlates to social anhedonia. Psychiatry Res. (2004) 125:147–60. doi: 10.1016/j.psychres.2003.12.008
10. Dolu N, Süer C, Özesmi Ç. A comparison of the different interpair intervals in the conditioning-testing P50 paradigms. Int J Psychophysiol. (2001) 41:265–70. doi: 10.1016/S0167-8760(01)00134-9
11. Jones LA, Hills PJ, Dick KM, Jones SP, Bright P. Cognitive mechanisms associated with auditory sensory gating. Brain Cogn. (2016) 102:33–45. doi: 10.1016/j.bandc.2015.12.005
12. Lijffijt M, Lane SD, Meier SL, Boutros NN, Burroughs S, Steinberg JL, et al. P50, N100, and P200 sensory gating: relationships with behavioral inhibition, attention, and working memory. Psychophysiology. (2009) 46:1059–68. doi: 10.1111/j.1469-8986.2009.00845.x
13. Brockhaus-Dumke A, Schultze-Lutter F, Mueller R, Tendolkar I, Bechdolf A, Pukrop R, et al. Sensory gating in schizophrenia: P50 and N100 gating in antipsychotic-free subjects at risk, first-episode, and chronic patients. Biol Psychiatry. (2008) 64:376–84. doi: 10.1016/j.biopsych.2008.02.006
14. Powell SB, Weber M, Geyer MA. Genetic models of sensorimotor gating: relevance to neuropsychiatric disorders. Curr Top Behav Neurosci. (2012) 12:251–318. doi: 10.1007/7854_2011_195
15. Blumenthal TD, Cuthbert BN, Filion DL, Hackley S, Lipp O V., Van Boxtel A. Committee report: Guidelines for human startle eyeblink electromyographic studies. Psychophysiology. (2005) 42:1–15. doi: 10.1111/j.1469-8986.2005.00271.x
16. Fridlund AJ, Cacioppo JT. Guidelines for human electromyographic research. Psychophysiology. (1986) 23:567–89. doi: 10.1111/j.1469-8986.1986.tb00676.x
17. Bramon E, Rabe-Hesketh S, Sham P, Murray RM, Frangou S. Meta-analysis of the P300 and P50 waveforms in schizophrenia. Schizophr Res. (2004) 70:315–29. doi: 10.1016/j.schres.2004.01.004
18. Cadenhead KS, Geyer MA, Braff DL. Impaired startle prepulse inhibition and habituation in patients with schizotypal personality disorder. Am J Psychiatry. (1993) 150:1862–7. doi: 10.1176/ajp.150.12.1862
19. Clementz BA, Geyer MA, Braff DL. Poor P50 suppression among schizophrenia patients and their first- degree biological relatives. Am J Psychiatry. (1998) 155:1691–4. doi: 10.1176/ajp.155.12.1691
20. Swerdlow NR, Light GA, Thomas ML, Sprock J, Calkins ME, Green MF, et al. Deficient prepulse inhibition in schizophrenia in a multi-site cohort: internal replication and extension. Schizophr Res. (2018) 198:6–15. doi: 10.1016/j.schres.2017.05.013
21. Hashimoto T, Shimizu E, Koike K, Orita Y, Suzuki T, Kanahara N, et al. Deficits in auditory P50 inhibition in obsessive–compulsive disorder. Prog Neuro-Psychopharmacol Biol Psychiatry. (2008) 32:288–96. doi: 10.1016/j.pnpbp.2007.08.021
22. Hoenig K, Hochrein A, Quednow BB, Maier W, Wagner M. Impaired prepulse inhibition of acoustic startle in obsessive-compulsive disorder. Biol Psychiatry. (2005) 57:1153–8. doi: 10.1016/j.biopsych.2005.01.040
23. Swerdlow NR, Karban B, Ploum Y, Sharp R, Geyer MA, Eastvold A. Tactile prepuff inhibition of startle in children with Tourette's syndrome: in search of an “fMRI-friendly” startle paradigm. Biol Psychiatry. (2001) 50:578–85. doi: 10.1016/S0006-3223(01)01164-7
24. Patterson J V, Sandman CA, Ring A, Jin Y, Bunney Jr. WE. An initial report of a new biological marker for bipolar disorder: P85 evoked brain potential. Bipolar Disord. (2009) 11:596–609. doi: 10.1111/j.1399-5618.2009.00734.x
25. Ancín I, Cabranes JA, Vázquez-Álvarez B, Santos JL, Sánchez-Morla E, García-Jiménez MÁ, Fernández C, Barabash A. Sensory gating deficit is associated with catechol-O-methyltransferase polymorphisms in bipolar disorder. World J Biol Psychiatry. (2011) 12:376–84. doi: 10.3109/15622975.2011.552192
26. Sánchez-Morla EM, Mateo J, Aparicio A, García-Jiménez MÁ, Jiménez E, Santos JL. Prepulse inhibition in euthymic bipolar disorder patients in comparison with control subjects. Acta Psychiatr Scand. (2016) 134:350–9. doi: 10.1111/acps.12604
27. Mao Z, Bo Q, Li W, Wang Z, Ma X, Wang C. Prepulse inhibition in patients with bipolar disorder: a systematic review and meta-analysis. BMC Psychiatry. (2019) 19:282. doi: 10.1186/s12888-019-2271-8
28. Lijffijt M, Moeller FG, Boutros NN, Steinberg JL, Meier SL, Lane SD, et al. Diminished P50, N100 and P200 auditory sensory gating in bipolar I disorder. Psychiatry Res. (2009) 167:191–201. doi: 10.1016/j.psychres.2008.04.001
29. Braff DL, Geyer MA, Swerdlow NR. Human studies of prepulse inhibition of startle: normal subjects, patient groups, and pharmacological studies. Psychopharmacology. (2001) 156:234–58. doi: 10.1007/s002130100810
30. Geyer MA. The family of sensorimotor gating disorders: comorbidities or diagnostic overlaps? Neurotox Res. (2006) 10:211–20. doi: 10.1007/BF03033358
31. Greenwood TA, Light GA, Swerdlow NR, Calkins ME, Green MF, Gur RE, et al. Gating deficit heritability and correlation with increased clinical severity in schizophrenia patients with positive family history. Am J Psychiatry. (2016) 173:385–91. doi: 10.1176/appi.ajp.2015.15050605
32. Schulze KK, Hall M-H, McDonald C, Marshall N, Walshe M, Murray RM, et al. P50 auditory evoked potential suppression in bipolar disorder patients with psychotic features and their unaffected relatives. Biol Psychiatry. (2007) 62:121–8. doi: 10.1016/j.biopsych.2006.08.006
33. Cadenhead KS, Swerdlow NR, Shafer KM, Diaz M, Braff DL. Modulation of the startle response and startle laterality in relatives of schizophrenic patients and in subjects with schizotypal personality disorder: evidence of inhibitory deficits. Am J Psychiatry. (2000) 157:1660–8. doi: 10.1176/appi.ajp.157.10.1660
34. Li W, Mao Z, Bo Q, Sun Y, Wang Z, Wang C. Prepulse inhibition in first-degree relatives of schizophrenia patients: a systematic review. Early Interv Psychiatry. (2020). doi: 10.1111/eip.13003. [Epub ahead of print].
35. Greenwood TA, Braff DL, Light GA, Cadenhead KS, Calkins ME, Dobie DJ, et al. Initial heritability analyses of endophenotypic measures for schizophrenia: the consortium on the genetics of schizophrenia. Arch Gen Psychiatry. (2007) 64:1242–50. doi: 10.1001/archpsyc.64.11.1242
36. Young DA, Waldo M, Rutledge JH, Freedman R. Heritability of inhibitory gating of the P50 auditory-evoked potential in monozygotic and dizygotic twins. Neuropsychobiology. (1996) 33:113–7. doi: 10.1159/000119260
37. Hall MH, Schulze K, Rijsdijk F, Picchioni M, Ettinger U, Bramon E, et al. Heritability and reliability of P300, P50 and duration mismatch negativity. Behav Genet. (2006) 36:845–57. doi: 10.1007/s10519-006-9091-6
38. Hasenkamp W, Epstein MP, Green A, Wilcox L, Boshoven W, Lewison B, et al. Heritability of acoustic startle magnitude, prepulse inhibition, and startle latency in schizophrenia and control families. Psychiatry Res. (2010) 178:236–43. doi: 10.1016/j.psychres.2009.11.012
39. Seidman LJ, Hellemann G, Nuechterlein KH, Greenwood TA, Braff DL, Cadenhead KS, et al. Factor structure and heritability of endophenotypes in schizophrenia: findings from the Consortium on the Genetics of Schizophrenia (COGS-1). Schizophr Res. (2015) 163:73–9. doi: 10.1016/j.schres.2015.01.027
40. Anokhin AP, Heath AC, Myers E, Ralano A, Wood S. Genetic influences on prepulse inhibition of startle reflex in humans. Neurosci Lett. (2003) 353:45–8. doi: 10.1016/j.neulet.2003.09.014
41. Aukes MF, Alizadeh BZ, Sitskoorn MM, Selten J-P, Sinke RJ, Kemner C, et al. Finding suitable phenotypes for genetic studies of schizophrenia: heritability and segregation analysis. Biol Psychiatry. (2008) 64:128–36. doi: 10.1016/j.biopsych.2007.12.013
42. Anokhin AP, Vedeniapin AB, Heath AC, Korzyukov O, Boutros NN. Genetic and environmental influences on sensory gating of mid-latency auditory evoked responses: a twin study. Schizophr Res. (2007) 89:312–9. doi: 10.1016/j.schres.2006.08.009
43. Flint J, Munafò MR. The endophenotype concept in psychiatric genetics. Psychol Med. (2007) 37:163–80. doi: 10.1017/S0033291706008750
44. Braff DL, Freedman R. Endophenotypes in studies of the genetics of schizophrenia. In: Davis KL, Charney D, Coyle J, Nemeroff C, editors. Neuropsychopharmacology: The Fifth Generation of Progress. Philadelphia, PA: Lippincott Williams & Wilkins (2002). p. 703–16.
45. Gottesman II, Gould TD. The endophenotype concept in psychiatry: etymology and strategic intentions. Am J Psychiatry. (2003) 160:636–45. doi: 10.1176/appi.ajp.160.4.636
46. Braff DL. The importance of endophenotypes in schizophrenia research. Schizophr Res. (2015) 163:1–8. doi: 10.1016/j.schres.2015.02.007
47. Quednow BB, Ejebe K, Wagner M, Giakoumaki SG, Bitsios P, Kumari V, et al. Meta-analysis on the association between genetic polymorphisms and prepulse inhibition of the acoustic startle response. Schizophr Res. (2018) 198:52–9. doi: 10.1016/j.schres.2017.12.011
48. Shamseer L, Moher D, Clarke M, Ghersi D, Liberati A, Petticrew M, et al. Preferred reporting items for systematic review and meta-analysis protocols (PRISMA-P) 2015: elaboration and explanation. BMJ. (2015) 350:g7647. doi: 10.1136/bmj.g7647
49. Sohani ZN, Meyre D, de Souza RJ, Joseph PG, Gandhi M, Dennis BB, et al. Assessing the quality of published genetic association studies in meta-analyses: the quality of genetic studies (Q-Genie) tool. BMC Genet. (2015) 16:50. doi: 10.1186/s12863-015-0211-2
50. Tang W, Hu J, Zhang H, Wu P, He H. Kappa coefficient: a popular measure of rater agreement. Shanghai Arch psychiatry. (2015) 27:62–7. doi: 10.11919/j.issn.1002-0829.215010
51. Moher D, Liberati A, Tetzlaff J, Altman DG, The PRISMA Group. Preferred reporting items for systematic reviews and meta-analyses: the PRISMA statement. PLoS Med. (2009) 6:e1000097. doi: 10.1371/journal.pmed1000097
52. Bertelsen B, Oranje B, Melchior L, Fagerlund B, Werge TM, Mikkelsen JD, et al. Association Study of CHRNA7 promoter variants with sensory and sensorimotor gating in schizophrenia patients and healthy controls: a Danish Case-Control Study. NeuroMolecular Med. (2015) 17:423–30. doi: 10.1007/s12017-015-8371-9
53. Bräuer D, Strobel A, Hensch T, Diers K, Lesch K-P, Brocke B. Genetic variation of serotonin receptor function affects prepulse inhibition of the startle. J Neural Transm. (2009) 116:607–13. doi: 10.1007/s00702-009-0222-0
54. Cabranes JA, Ancín I, Santos JL, SÁnchez-Morla E, García-JimÉnez MÁ, López-Ibor JJ, et al. No effect of polymorphisms in the non-duplicated region of the CHRNA7 gene on sensory gating P50 ratios in patients with schizophrenia and bipolar disorder. Psychiatry Res. (2013) 205:276–8. doi: 10.1016/j.psychres.2012.08.015
55. Comasco E, Gulinello M, Hellgren C, Skalkidou A, Sylven S, Sundström-Poromaa I. Sleep duration, depression, and oxytocinergic genotype influence prepulse inhibition of the startle reflex in postpartum women. Eur Neuropsychopharmacol. (2016) 26:767–76. doi: 10.1016/J.EURONEURO.2016.01.002
56. Comasco E, Hellgren C, Olivier J, Skalkidou A, Sundström Poromaa I. Supraphysiological hormonal status, anxiety disorders, and COMT Val/Val genotype are associated with reduced sensorimotor gating in women. Psychoneuroendocrinology. (2015) 60:217–23. doi: 10.1016/j.psyneuen.2015.06.019
57. de Koning MB, Boot E, Bloemen OJN, van Duin EDA, Abel KM, de Haan L, et al. Startle reactivity and prepulse inhibition of the acoustic startle response are modulated by catechol-O-methyl-transferase Val(158) Met polymorphism in adults with 22q11 deletion syndrome. J Psychopharmacol. (2012) 26:1548–60. doi: 10.1177/0269881112456610
58. de Koning MB, van Duin EDA, Boot E, Bloemen OJN, Bakker JA, Abel KM, et al. PRODH rs450046 and proline x COMT Val158Met interaction effects on intelligence and startle in adults with 22q11 deletion syndrome. Psychopharmacology. (2015) 232:3111–22. doi: 10.1007/s00213-015-3971-5
59. de la Salle S, Smith D, Choueiry J, Impey D, Philippe T, Dort H, et al. Effects of COMT genotype on sensory gating and its modulation by nicotine: differences in low and high P50 suppressors. Neuroscience. (2013) 241:147–56. doi: 10.1016/j.neuroscience.2013.03.029
60. Demily C, Louchart-de-la-Chapelle S, Nkam I, Ramoz N, Denise P, Nicolas A, et al. Does COMT val158met polymorphism influence P50 sensory gating, eye tracking or saccadic inhibition dysfunctions in schizophrenia? Psychiatry Res. (2016) 246:738–44. doi: 10.1016/j.psychres.2016.07.066
61. Flomen RH, Shaikh M, Walshe M, Schulze K, Hall M-H, Picchioni M, et al. Association between the 2-bp deletion polymorphism in the duplicated version of the alpha7 nicotinic receptor gene and P50 sensory gating. Eur J Hum Genet. (2013) 21:76–81. doi: 10.1038/ejhg.2012.81
62. Gajewska A, Blumenthal TD, Winter B, Herrmann MJ, Conzelmann A, Mühlberger A, et al. Effects of ADORA2A gene variation and caffeine on prepulse inhibition: a multi-level risk model of anxiety. Prog Neuro Psychopharmacol Biol Psychiatry. (2013) 40:115–21. doi: 10.1016/j.pnpbp.2012.08.008
63. Giakoumaki SG, Roussos P, Bitsios P. Improvement of prepulse inhibition and executive function by the COMT inhibitor tolcapone depends on COMT Val158Met polymorphism. Neuropsychopharmacology. (2008) 33:3058–68. doi: 10.1038/npp.2008.82
64. Greenbaum L, Levin R, Lerer E, Alkelai A, Kohn Y, Heresco-Levy U, et al. Association of Reelin (RELN) single nucleotide polymorphism rs7341475 with prepulse inhibition in the Jewish Israeli population. Biol Psychiatry. (2011) 69:e17–8. doi: 10.1016/j.biopsych.2010.09.059
65. Greenwood TA, Lazzeroni LC, Maihofer AX, Swerdlow NR, Calkins ME, Freedman R, et al. Genome-wide Association of Endophenotypes for Schizophrenia From the Consortium on the Genetics of Schizophrenia (COGS) Study. JAMA Psychiatry. (2019) 76:1274. doi: 10.1001/jamapsychiatry.2019.2850
66. Greenwood TA, Lazzeroni LC, Murray SS, Cadenhead KS, Calkins ME, Dobie DJ, et al. Analysis of 94 candidate genes and 12 endophenotypes for schizophrenia from the Consortium on the Genetics of Schizophrenia. Am J Psychiatry. (2011) 168:930–46. doi: 10.1176/appi.ajp.2011.10050723
67. Greenwood TA, Light GA, Swerdlow NR, Radant AD, Braff DL. Association analysis of 94 candidate genes and schizophrenia-related endophenotypes. PLoS ONE. (2012) 7:e29630. doi: 10.1371/journal.pone.0029630
68. Hall M-H, Chen C-Y, Cohen BM, Spencer KM, Levy DL, Öngür D, et al. Genomewide association analyses of electrophysiological endophenotypes for schizophrenia and psychotic bipolar disorders: a preliminary report. Am J Med Genet Part B Neuropsychiatr Genet. (2015) 168:151–61. doi: 10.1002/ajmg.b.32298
69. Hall M-H, Levy DL, Salisbury DF, Haddad S, Gallagher P, Lohan M, et al. Neurophysiologic effect of GWAS derived schizophrenia and bipolar risk variants. Am J Med Genet Part B Neuropsychiatr Genet. (2014) 165:9–18. doi: 10.1002/ajmg.b.32212
70. Hashimoto R, Ohi K, Yasuda Y, Fukumoto M, Yamamori H, Takahashi H, et al. Variants of the RELA gene are associated with schizophrenia and their startle responses. Neuropsychopharmacology. (2011) 36:1921–31. doi: 10.1038/npp.2011.78
71. Hessl D, Berry-Kravis E, Cordeiro L, Yuhas J, Ornitz EM, Campbell A, et al. Prepulse inhibition in fragile X syndrome: feasibility, reliability, and implications for treatment. Am J Med Genet B Neuropsychiatr Genet. (2009) 150B:545–53. doi: 10.1002/ajmg.b.30858
72. Hokyo A, Kanazawa T, Uenishi H, Tsutsumi A, Kawashige S, Kikuyama H, et al. Habituation in prepulse inhibition is affected by a polymorphism on the NMDA receptor 2B subunit gene (GRIN2B). Psychiatr Genet. (2010) 20:191–8. doi: 10.1097/YPG.0b013e32833a201d
73. Hong LE, Wonodi I, Stine OC, Mitchell BD, Thaker GK. Evidence of missense mutations on the neuregulin 1 gene affecting function of prepulse inhibition. Biol Psychiatry. (2008) 63:17–23. doi: 10.1016/j.biopsych.2007.05.011
74. Kirenskaya AV, Storozheva ZI, Kolobov VV, Sherstnev VV. The acoustic startle response and polymorphism of the gene for catechol-O-methyltransferase in the norm and in schizophrenia. Neurochem J. (2015) 9:76–83. doi: 10.1134/S1819712415010031
75. Knott V, Millar A, Fisher D, Albert P. Effects of nicotine on the amplitude and gating of the auditory P50 and its influence by dopamine D2 receptor gene polymorphism. Neuroscience. (2010) 166:145–56. doi: 10.1016/j.neuroscience.2009.11.053
76. Lennertz L, Quednow BB, Schuhmacher A, Petrovsky N, Frommann I, Schulze-Rauschenbach S, et al. The functional coding variant Asn107Ile of the neuropeptide S receptor gene (NPSR1) is associated with schizophrenia and modulates verbal memory and the acoustic startle response. Int J Neuropsychopharmacol. (2012) 15:1205–15. doi: 10.1017/S1461145711001623
77. Leonard S, Gault J, Hopkins J, Logel J, Vianzon R, Short M, et al. Association of promoter variants in the α7 nicotinic acetylcholine receptor subunit gene with an inhibitory deficit found in schizophrenia. Arch Gen Psychiatry. (2002) 59:1085–96. doi: 10.1001/archpsyc.59.12.1085
78. Levin R, Heresco-Levy U, Bachner-Melman R, Israel S, Shalev I, Ebstein RP. Association between arginine vasopressin 1a receptor (AVPR1a) promoter region polymorphisms and prepulse inhibition. Psychoneuroendocrinology. (2009) 34:901–8. doi: 10.1016/j.psyneuen.2008.12.014
79. Liu X, Hong X, Chan RCK, Kong F, Peng Z, Wan X, et al. Association study of polymorphisms in the alpha 7 nicotinic acetylcholine receptor subunit and catechol-o-methyl transferase genes with sensory gating in first-episode schizophrenia. Psychiatry Res. (2013) 209:431–8. doi: 10.1016/j.psychres.2013.03.027
80. Lu BY, Martin KE, Edgar JC, Smith AK, Lewis SF, Escamilla MA, et al. Effect of catechol O-methyltransferase val(158)met polymorphism on the p50 gating endophenotype in schizophrenia. Biol Psychiatry. (2007) 62:822–5. doi: 10.1016/j.biopsych.2006.11.030
81. Majic T, Rentzsch J, Gudlowski Y, Ehrlich S, Juckel G, Sander T, et al. COMT Val108/158Met genotype modulates human sensory gating. Neuroimage. (2011) 55:818–24. doi: 10.1016/j.neuroimage.2010.12.031
82. Mao Q, Tan Y-L, Luo X-G, Tian L, Wang Z-R, Tan S-P, Chen S, Yang G-G, An H-M, Yang F-D, et al. Association of catechol-O-methyltransferase Val 108/158 Met genetic polymorphism with schizophrenia, P50 sensory gating, and negative symptoms in a Chinese population. Psychiatry Res. (2016) 242:271–6. doi: 10.1016/j.psychres.2016.04.029
83. Matsuno H, Ohi K, Hashimoto R, Yamamori H, Yasuda Y, Fujimoto M, et al. A Naturally occurring null variant of the NMDA type glutamate receptor NR3B subunit is a risk factor of schizophrenia. PLoS ONE. (2015) 10:e0116319. doi: 10.1371/journal.pone.0116319
84. Millar A, Smith D, Choueiry J, Fisher D, Albert P, Knott V. The moderating role of the dopamine transporter 1 gene on P50 sensory gating and its modulation by nicotine. Neuroscience. (2011) 180:148–56. doi: 10.1016/j.neuroscience.2011.02.008
85. Montag C, Hartmann P, Merz M, Burk C, Reuter M. D2 receptor density and prepulse inhibition in humans: negative findings from a molecular genetic approach. Behav Brain Res. (2008) 187:428–32. doi: 10.1016/j.bbr.2007.10.006
86. Notzon S, Vennewald N, Gajewska A, Klahn AL, Diemer J, Winter B, et al. Is prepulse modification altered by continuous theta burst stimulation? DAT1 genotype and motor threshold interact on prepulse modification following brain stimulation. Eur Arch Psychiatry Clin Neurosci. (2017) 267:767–79. doi: 10.1007/s00406-017-0786-x
87. Perkins KA, Lerman C, Coddington S, Jetton C, Karelitz JL, Wilson A, et al. Gene and gene by sex associations with initial sensitivity to nicotine in nonsmokers. Behav Pharmacol. (2008) 19:630–40. doi: 10.1097/FBP.0b013e32830c3621
88. Petrovsky N, Ettinger U, Kessler H, Mössner R, Wolfsgruber S, Dahmen N, et al. The effect of nicotine on sensorimotor gating is modulated by a CHRNA3 polymorphism. Psychopharmacology. (2013) 229:31–40. doi: 10.1007/s00213-013-3081-1
89. Petrovsky N, Quednow BB, Ettinger U, Schmechtig A, Mössner R, Collier DA, et al. Sensorimotor gating is associated with CHRNA3 polymorphisms in schizophrenia and healthy volunteers. Neuropsychopharmacology. (2010) 35:1429–39. doi: 10.1038/npp.2010.12
90. Quednow BB, Brinkmeyer J, Mobascher A, Nothnagel M, Musso F, Gründer G, et al. Schizophrenia risk polymorphisms in the TCF4 gene interact with smoking in the modulation of auditory sensory gating. Proc Natl Acad Sci USA. (2012) 109:6271–6. doi: 10.1073/pnas.1118051109
91. Quednow BB, Ettinger U, Mössner R, Rujescu D, Giegling I, Collier DA, et al. The schizophrenia risk allele C of the TCF4 rs9960767 polymorphism disrupts sensorimotor gating in schizophrenia spectrum and healthy volunteers. J Neurosci. (2011) 31:6684–91. doi: 10.1523/JNEUROSCI.0526-11.2011
92. Quednow BB, Kühn K-U, Mössner R, Schwab SG, Schuhmacher A, Maier W, et al. Sensorimotor gating of schizophrenia patients is influenced by 5-HT2A receptor polymorphisms. Biol Psychiatry. (2008) 64:434–7. doi: 10.1016/j.biopsych.2008.02.019
93. Quednow BB, Schmechtig A, Ettinger U, Petrovsky N, Collier DA, Vollenweider FX, et al. Sensorimotor gating depends on polymorphisms of the serotonin-2A receptor and catechol-O-methyltransferase, but Not on Neuregulin-1 Arg38Gln genotype: a replication study. Biol Psychiatry. (2009) 66:614–20. doi: 10.1016/j.biopsych.2009.05.007
94. Quednow BB, Wagner M, Mössner R, Maier W, Kühn K-UU. Sensorimotor gating of schizophrenia patients depends on catechol o-methyltransferase val158met polymorphism. Schizophr Bull. (2010) 36:341–6. doi: 10.1093/schbul/sbn088
95. Raux G, Bonnet-Brilhault F, Louchart S, Houy E, Gantier R, Levillain D, et al. The -2 bp deletion in exon 6 of the “alpha 7-like” nicotinic receptor subunit gene is a risk factor for the P50 sensory gating deficit. Mol Psychiatry. (2002) 7:1006–11. doi: 10.1038/sj.mp.4001140
96. Roussos P, Giakoumaki SG, Adamaki E, Bitsios P. The influence of schizophrenia-related neuregulin-1 polymorphisms on sensorimotor gating in healthy males. Biol Psychiatry. (2011) 69:479–86. doi: 10.1016/j.biopsych.2010.09.009
97. Roussos P, Giakoumaki SG, Adamaki E, Georgakopoulos A, Anastasios G, Robakis NK, et al. The association of schizophrenia risk D-amino acid oxidase polymorphisms with sensorimotor gating, working memory and personality in healthy males. Neuropsychopharmacology. (2011) 36:1677–88. doi: 10.1038/npp.2011.49
98. Roussos P, Giakoumaki SG, Bitsios P. A risk PRODH haplotype affects sensorimotor gating, memory, schizotypy, and anxiety in healthy male subjects. Biol Psychiatry. (2009) 65:1063–70. doi: 10.1016/j.biopsych.2009.01.003
99. Roussos P, Giakoumaki SG, Bitsios P. The dopamine D3 receptor Ser9Gly polymorphism modulates prepulse inhibition of the acoustic startle reflex. Biol Psychiatry. (2008) 64:235–40. doi: 10.1016/j.biopsych.2008.01.020
100. Roussos P, Giakoumaki SG, Bitsios P. Tolcapone effects on gating, working memory, and mood interact with the synonymous catechol-O-methyltransferase rs4818c/g polymorphism. Biol Psychiatry. (2009) 66:997–1004. doi: 10.1016/j.biopsych.2009.07.008
101. Roussos P, Giakoumaki SG, Georgakopoulos A, Robakis NK, Bitsios P. The CACNA1C and ANK3 risk alleles impact on affective personality traits and startle reactivity but not on cognition or gating in healthy males. Bipolar Disord. (2011) 13:250–9. doi: 10.1111/j.1399-5618.2011.00924.x
102. Roussos P, Giakoumaki SG, Rogdaki M, Pavlakis S, Frangou S, Bitsios P. Prepulse inhibition of the startle reflex depends on the catechol O-methyltransferase Val158Met gene polymorphism. Psychol Med. (2008) 38:1651–8. doi: 10.1017/S0033291708002912
103. Roussos P, Giakoumaki SG, Zouraraki C, Fullard JF, Karagiorga VE, Tsapakis EM, et al. The relationship of common risk variants and polygenic risk for schizophrenia to sensorimotor gating. Biol Psychiatry. (2016) 79:988–96. doi: 10.1016/j.biopsych.2015.06.019
104. Roussos P, Katsel P, Davis KL, Giakoumaki SG, Lencz T, Malhotra AK, et al. Convergent findings for abnormalities of the NF-κB signaling pathway in schizophrenia. Neuropsychopharmacology. (2013) 38:533–9. doi: 10.1038/npp.2012.215
105. Rovný R, Marko M, Katina S, Murínová J, Roháriková V, Cimrová B, et al. Association between genetic variability of neuronal nitric oxide synthase and sensorimotor gating in humans. Nitric Oxide. (2018) 80:32–6. doi: 10.1016/J.NIOX.2018.08.002
106. Schuhmacher A, Becker T, Rujescu D, Quednow BB, Lennertz L, Wagner M, et al. Investigation of tryptophan hydroxylase 2 (TPH2) in schizophrenia and in the response to antipsychotics. J Psychiatr Res. (2012) 46:1073–80. doi: 10.1016/j.jpsychires.2012.04.021
107. Shaikh M, Hall M-H, Schulze K, Dutt A, Walshe M, Williams I, et al. Do COMT, BDNF and NRG1 polymorphisms influence P50 sensory gating in psychosis? Psychol Med. (2011) 41:263–76. doi: 10.1017/S003329170999239X
108. Shi J, Wang Z, Tan Y, Fan H, An H, Zuo L, et al. CHRNA4 was associated with prepulse inhibition of schizophrenia in Chinese: a pilot study. Cogn Neuropsychiatry. (2016) 21:156–67. doi: 10.1080/13546805.2016.1155437
109. Vogt J, Yang J, Mobascher A, Cheng J, Li Y, Liu X, et al. Molecular cause and functional impact of altered synaptic lipid signaling due to a prg-1 gene SNP. EMBO Mol Med. (2016) 8:25–38. doi: 10.15252/emmm.201505677
110. Völter C, Riedel M, Wöstmann N, Aichert DS, Lobo S, Costa A, et al. Sensorimotor gating and D2 receptor signalling: evidence from a molecular genetic approach. Int J Neuropsychopharmacol. (2012) 15:1427–40. doi: 10.1017/S1461145711001787
111. Vorstman JAS, Turetsky BI, Sijmens-Morcus MEJ, de Sain MG, Dorland B, Sprong M, et al. Proline affects brain function in 22q11DS children with the low activity COMT158 allele. Neuropsychopharmacology. (2009) 34:739–46. doi: 10.1038/npp.2008.132
112. Wonodi I, McMahon RP, Krishna N, Mitchell BD, Liu J, Glassman M, et al. Influence of kynurenine 3-monooxygenase (KMO) gene polymorphism on cognitive function in schizophrenia. Schizophr Res. (2014) 160:80–7. doi: 10.1016/j.schres.2014.10.026
113. Zarchi O, Carmel M, Avni C, Attias J, Frisch A, Michaelovsky E, et al. Schizophrenia-like neurophysiological abnormalities in 22q11.2 deletion syndrome and their association to COMT and PRODH genotypes. J Psychiatr Res. (2013) 47:1623–9. doi: 10.1016/j.jpsychires.2013.07.004
114. Mi H, Muruganujan A, Ebert D, Huang X, Thomas PD. PANTHER version 14: more genomes, a new PANTHER GO-slim and improvements in enrichment analysis tools. Nucleic Acids Res. (2019) 47:D419–26. doi: 10.1093/nar/gky1038
115. The Gene Ontology Consortium. The gene ontology resource: 20 years and still GOing strong. Nucleic Acids Res. (2019) 47:D330–8. doi: 10.1093/nar/gky1055
116. Ashburner M, Ball CA, Blake JA, Botstein D, Butler H, Cherry JM, et al. Gene ontology: tool for the unification of biology. the gene ontology consortium. Nat Genet. (2000) 25:25–9. doi: 10.1038/75556
117. Ward LD, Kellis M. HaploReg: a resource for exploring chromatin states, conservation, and regulatory motif alterations within sets of genetically linked variants. Nucleic Acids Res. (2012) 40:D930–4. doi: 10.1093/nar/gkr917
118. Bulger M, Groudine M. Functional and mechanistic diversity of distal transcription enhancers. Cell. (2011) 144:327–39. doi: 10.1016/j.cell.2011.01.024
119. Hasenkamp W, Norrholm SD, Green A, Lewison B, Boshoven W, Keyes M, et al. Differences in startle reflex and prepulse inhibition in European-Americans and African-Americans. Psychophysiology. (2008) 45:876–82. doi: 10.1111/j.1469-8986.2008.00680.x
120. Tunbridge EM, Harrison PJ, Weinberger DR. Catechol-o-methyltransferase, cognition, and psychosis: Val158Met and beyond. Biol Psychiatry. (2006) 60:141–51. doi: 10.1016/j.biopsych.2005.10.024
121. Witte AV, Flöel A. Effects of COMT polymorphisms on brain function and behavior in health and disease. Brain Res Bull. (2012) 88:418–28. doi: 10.1016/j.brainresbull.2011.11.012
122. Taylor S. Association between COMT Val158Met and psychiatric disorders: a comprehensive meta-analysis. Am J Med Genet B Neuropsychiatr Genet. (2018) 177:199–210. doi: 10.1002/ajmg.b.32556
123. White TP, Loth E, Rubia K, Krabbendam L, Whelan R, Banaschewski T, et al. Sex differences in COMT polymorphism effects on prefrontal inhibitory control in adolescence. Neuropsychopharmacology. (2014) 39:2560–9. doi: 10.1038/npp.2014.107
124. Tunbridge EM, Harrison PJ. Importance of the COMT gene for sex differences in brain function and predisposition to psychiatric disorders. Curr Top Behav Neurosci. (2011) 8:119–40. doi: 10.1007/7854_2010_97
125. Kumari V, Antonova E, Zachariah E, Galea A, Aasen I, Ettinger U, et al. Structural brain correlates of prepulse inhibition of the acoustic startle response in healthy humans. Neuroimage. (2005) 26:1052–8. doi: 10.1016/j.neuroimage.2005.03.002
126. Kumari V, Gray JA, Geyer MA, ffytche D, Soni W, Mitterschiffthaler MT, et al. Neural correlates of tactile prepulse inhibition: a functional MRI study in normal and schizophrenic subjects. Psychiatry Res Neuroimaging. (2003) 122:99–113. doi: 10.1016/S0925-4927(02)00123-3
127. Hazlett EA, Buchsbaum MS, Haznedar MM, Singer MB, Germans MK, Schnur DB, et al. Prefrontal cortex glucose metabolism and startle eyeblink modification abnormalities in unmedicated schizophrenia patients. Psychophysiology. (1998) 35:186–98. doi: 10.1111/1469-8986.3520186
128. Swerdlow NR, Koob GF. Dopamine, schizophrenia, mania, and depression: toward a unified hypothesis of cortico-striatopallido-thalamic function. Behav Brain Sci. (1987) 10:197–208. doi: 10.1017/S0140525X00047488
129. Gothelf D, Law AJ, Frisch A, Chen J, Zarchi O, Michaelovsky E, et al. Biological effects of COMT haplotypes and psychosis risk in 22q11.2 deletion syndrome. Biol Psychiatry. (2014) 75:406–13. doi: 10.1016/j.biopsych.2013.07.021
130. Bray NJ, Buckland PR, Williams NM, Williams HJ, Norton N, Owen MJ, et al. A haplotype implicated in schizophrenia susceptibility is associated with reduced COMT expression in human brain. Am J Hum Genet. (2003) 73:152–61. doi: 10.1086/376578
131. Shifman S, Bronstein M, Sternfeld M, Pisanté-Shalom A, Lev-Lehman E, Weizman A, et al. A highly significant association between a COMT haplotype and schizophrenia. Am J Hum Genet. (2002) 71:1296–302. doi: 10.1086/344514
132. Meyer-Lindenberg A, Nichols T, Callicott JH, Ding J, Kolachana B, Buckholtz J, et al. Impact of complex genetic variation in COMT on human brain function. Mol Psychiatry. (2006) 11:867–77. doi: 10.1038/sj.mp.4001860
133. Michaelovsky E, Gothelf D, Korostishevsky M, Frisch A, Burg M, Carmel M, et al. Association between a common haplotype in the COMT gene region and psychiatric disorders in individuals with 22q11.2DS. Int J Neuropsychopharmacol. (2008) 11:351–63. doi: 10.1017/S1461145707008085
134. Ott T, Nieder A. Dopamine and cognitive control in prefrontal cortex. Trends Cogn Sci. (2019) 23:213–34. doi: 10.1016/j.tics.2018.12.006
135. Swerdlow NR, Geyer MA, Braff DL. Neural circuit regulation of prepulse inhibition of startle in the rat: current knowledge and future challenges. Psychopharmacology. (2001) 156:194–215. doi: 10.1007/s002130100799
136. Swerdlow NR, Mansbach RS, Geyer MA, Pulvirenti L, Koob GF, Braff DL. Amphetamine disruption of prepulse inhibition of acoustic startle is reversed by depletion of mesolimbic dopamine. Psychopharmacology. (1990) 100:413–6. doi: 10.1007/BF02244616
137. Swerdlow N, Eastvold A, Karban B, Ploum Y, Stephany N, Geyer M, et al. Dopamine agonist effects on startle and sensorimotor gating in normal male subjects: time course studies. Psychopharmacology. (2002) 161:189–201. doi: 10.1007/s00213-002-1040-3
138. Burnet PW, Eastwood SL, Lacey K, Harrison PJ. The distribution of 5-HT1A and 5-HT2A receptor mRNA in human brain. Brain Res. (1995) 676:157–68. doi: 10.1016/0006-8993(95)00104-X
139. Zhang G, Stackman RW, Jr. The role of serotonin 5-HT2A receptors in memory and cognition. Front Pharmacol. (2015) 6:225. doi: 10.3389/fphar.2015.00225
140. Quednow BB, Geyer MA, Halberstadt AL. Serotonin and schizophrenia. Handb Behav Neurosci. (2020) 31:711–43. doi: 10.1016/B978-0-444-64125-0.00039-6
141. Moravcíková L, Csatlósová K, Durišová B, Ondácová K, Pavlovicová M, Lacinová L, Dremencov E. Role of serotonin-2A receptors in pathophysiology and treatment of depression. In: Guiard B, Di Giovanni G, editors. 5-HT2A Receptors in the Central Nervous System. Cham: Springer International Publishing (2018). p. 205–30.
142. Parsons MJ, D'Souza UM, Arranz M-J, Kerwin RW, Makoff AJ. The−1438A/G polymorphism in the 5-hydroxytryptamine type 2A receptor gene affects promoter activity. Biol Psychiatry. (2004) 56:406–10. doi: 10.1016/j.biopsych.2004.06.020
143. Gu L, Long J, Yan Y, Chen Q, Pan R, Xie X, et al. HTR2A −1438A/G polymorphism influences the risk of schizophrenia but not bipolar disorder or major depressive disorder: a meta-analysis. J Neurosci Res. (2013) 91:623–33. doi: 10.1002/jnr.23180
144. Farrell MS, Werge T, Sklar P, Owen MJ, Ophoff RA, O'Donovan MC, et al. Evaluating historical candidate genes for schizophrenia. Mol Psychiatry. (2015) 20:555–62. doi: 10.1038/mp.2015.16
145. Taylor S. Disorder-specific genetic factors in obsessive-compulsive disorder: a comprehensive meta-analysis. Am J Med Genet B Neuropsychiatr Genet. (2016) 171:325–32. doi: 10.1002/ajmg.b.32407
146. Mackowick KM, Barr MS, Wing VC, Rabin RA, Ouellet-Plamondon C, George TP. Neurocognitive endophenotypes in schizophrenia: modulation by nicotinic receptor systems. Prog Neuropsychopharmacol Biol Psychiatry. (2014) 52:79–85. doi: 10.1016/j.pnpbp.2013.07.010
147. Kumari V, Postma P. Nicotine use in schizophrenia: the self medication hypotheses. Neurosci Biobehav Rev. (2005) 29:1021–34. doi: 10.1016/j.neubiorev.2005.02.006
148. Kumari V, Gray JA. Smoking withdrawal, nicotine dependence and prepulse inhibition of the acoustic startle reflex. Psychopharmacology. (1999) 141:11–5. doi: 10.1007/s002130050800
149. Dalack GW, Healy DJ, Meador-Woodruff JH. Nicotine dependence in schizophrenia: clinical phenomena and laboratory findings. Am J Psychiatry. (1998) 155:1490–501. doi: 10.1176/ajp.155.11.1490
150. Quigley H, MacCabe JH. The relationship between nicotine and psychosis. Ther Adv Psychopharmacol. (2019) 9:204512531985996. doi: 10.1177/2045125319859969
151. Brunzell DH, Stafford AM, Dixon CI. Nicotinic receptor contributions to smoking: insights from human studies and animal models. Curr Addict Reports. (2015) 2:33–46. doi: 10.1007/s40429-015-0042-2
152. Jackson KJ, Fanous AH, Chen J, Kendler KS, Chen X. Variants in the 15q25 gene cluster are associated with risk for schizophrenia and bipolar disorder. Psychiatr Genet. (2013) 23:20. doi: 10.1097/YPG.0b013e32835bd5f1
153. Ware JJ, Munafò MR. Genetics of smoking behaviour. Curr Top Behav Neurosci. (2015) 23:19–36. doi: 10.1007/978-3-319-13665-3_2
154. Berrettini W, Yuan X, Tozzi F, Song K, Francks C, Chilcoat H, et al. Alpha-5/alpha-3 nicotinic receptor subunit alleles increase risk for heavy smoking. Mol Psychiatry. (2008) 13:368–73. doi: 10.1038/sj.mp.4002154
155. Barrie ES, Hartmann K, Lee S-H, Frater JT, Seweryn M, Wang D, et al. The CHRNA5/CHRNA3/CHRNB4 nicotinic receptor regulome: genomic architecture, regulatory variants, and clinical associations. Hum Mutat. (2017) 38:112–9. doi: 10.1002/humu.23135
156. Forrest MP, Hill MJ, Kavanagh DH, Tansey KE, Waite AJ, Blake DJ. The psychiatric risk gene transcription factor 4 (TCF4) regulates neurodevelopmental pathways associated with schizophrenia, autism, and intellectual disability. Schizophr Bull. (2018) 44:1100–10. doi: 10.1093/schbul/sbx164
157. Sinkus ML, Graw S, Freedman R, Ross RG, Lester HA, Leonard S. The human CHRNA7 and CHRFAM7A genes: a review of the genetics, regulation, and function. Neuropharmacology. (2015) 96:274–88. doi: 10.1016/j.neuropharm.2015.02.006
158. Guan ZZ, Zhang X, Blennow K, Nordberg A. Decreased protein level of nicotinic receptor alpha7 subunit in the frontal cortex from schizophrenic brain. Neuroreport. (1999) 10:1779–82. doi: 10.1097/00001756-199906030-00028
159. Mexal S, Berger R, Logel J, Ross RG, Freedman R, Leonard S. Differential regulation of alpha7 nicotinic receptor gene (CHRNA7) expression in schizophrenic smokers. J Mol Neurosci. (2010) 40:185–95. doi: 10.1007/s12031-009-9233-4
160. Forrest MP, Hill MJ, Quantock AJ, Martin-Rendon E, Blake DJ. The emerging roles of TCF4 in disease and development. Trends Mol Med. (2014) 20:322–31. doi: 10.1016/j.molmed.2014.01.010
161. Ripke S, Neale BM, Corvin A, Walters JTR, Farh K-H, Holmans PA, et al. Biological insights from 108 schizophrenia-associated genetic loci. Nature. (2014) 511:421–7. doi: 10.1038/nature13595
162. Quednow BB, Brzózka MM, Rossner MJ. Transcription factor 4 (TCF4) and schizophrenia: integrating the animal and the human perspective. Cell Mol Life Sci. (2014) 71:2815–35. doi: 10.1007/s00018-013-1553-4
163. Williams HJ, Moskvina V, Smith RL, Dwyer S, Russo G, Owen MJ, et al. Association between TCF4 and schizophrenia does not exert its effect by common nonsynonymous variation or by influencing cis-acting regulation of mRNA expression in adult human brain. Am J Med Genet B Neuropsychiatr Genet. (2011) 156B:781–4. doi: 10.1002/ajmg.b.31219
164. Birnbaum R, Weinberger DR. Genetic insights into the neurodevelopmental origins of schizophrenia. Nat Rev Neurosci. (2017) 18:727–40. doi: 10.1038/nrn.2017.125
165. Brenner CA, Edwards CR, Carroll CA, Kieffaber PD, Hetrick WP. P50 and acoustic startle gating are not related in healthy participants. Psychophysiology. (2004) 41:702–8. doi: 10.1111/j.1469-8986.2004.00206.x
166. Braff DL, Light GA, Swerdlow NR. Prepulse inhibition and P50 suppression are both deficient but not correlated in schizophrenia patients. Biol Psychiatry. (2007) 61:1204–7. doi: 10.1016/j.biopsych.2006.08.015
167. Oranje B, Geyer MA, Bocker KBE, Leon Kenemans J, Verbaten MN. Prepulse inhibition and P50 suppression: commonalities and dissociations. Psychiatry Res. (2006) 143:147–58. doi: 10.1016/j.psychres.2005.11.002
168. Cadenhead KS, Light GA, Geyer MA, McDowell JE, Braff DL. Neurobiological measures of schizotypal personality disorder: defining an inhibitory endophenotype? Am J Psychiatry. (2002) 159:869–71. doi: 10.1176/appi.ajp.159.5.869
169. Schwarzkopf SB, Lamberti JS, Smith DA. Concurrent assessment of acoustic startle and auditory P50 evoked potential measures of sensory inhibition. Biol Psychiatry. (1993) 33:815–28. doi: 10.1016/0006-3223(93)90023-7
170. Ellenbroek BA, van Luijtelaar G, Frenken M, Cools AR. Sensory gating in rats: lack of correlation between auditory evoked potential gating and prepulse inhibition. Schizophr Bull. (1999) 25:777–88. doi: 10.1093/oxfordjournals.schbul.a033418
171. Oranje B, van Berckel BN, Kemner C, van Ree JM, Kahn RS, Verbaten MN. P50 suppression and prepulse inhibition of the startle reflex in humans: a correlational study. Biol Psychiatry. (1999) 45:883–90. doi: 10.1016/S0006-3223(98)00128-0
172. Koch M. The neurobiology of startle. Prog Neurobiol. (1999) 59:107–28. doi: 10.1016/S0301-0082(98)00098-7
173. Koch M, Kungel M, Herbert H. Cholinergic neurons in the pedunculopontine tegmental nucleus are involved in the mediation of prepulse inhibition of the acoustic startle response in the rat. Exp Brain Res. (1993) 97:71–82. doi: 10.1007/BF00228818
174. Swerdlow NR, Light GA. Sensorimotor gating deficits in schizophrenia: advancing our understanding of the phenotype, its neural circuitry and genetic substrates. Schizophr Res. (2018) 198:1–5. doi: 10.1016/j.schres.2018.02.042
175. Schwabe K, Krauss JK. What rodent models of deep brain stimulation can teach us about the neural circuit regulation of prepulse inhibition in neuropsychiatric disorders. Schizophr Res. (2018) 198:45–51. doi: 10.1016/j.schres.2017.06.033
176. Swerdlow NR, Braff DL, Geyer MA. Animal models of deficient sensorimotor gating: what we know, what we think we know, and what we hope to know soon. Behav Pharmacol. (2000) 11:185–204. doi: 10.1097/00008877-200006000-00002
177. Grunwald T, Boutros NN, Pezer N, von Oertzen J, Fernández G, Schaller C, et al. Neuronal substrates of sensory gating within the human brain. Biol Psychiatry. (2003) 53:511–9. doi: 10.1016/S0006-3223(02)01673-6
178. Mayer A, Hanlon F, Franco A, Teshiba T, Thoma R, Clark V, et al. The neural networks underlying auditory sensory gating. Neuroimage. (2009) 44:182–9. doi: 10.1016/j.neuroimage.2008.08.025
Keywords: schizophrenia, endophenotypes, intermediate phenotype, prepulse inhibition, P50, sensory gating, sensorimotor gating, startle reflex
Citation: Rovný R, Besterciová D and Riečanský I (2020) Genetic Determinants of Gating Functions: Do We Get Closer to Understanding Schizophrenia Etiopathogenesis? Front. Psychiatry 11:550225. doi: 10.3389/fpsyt.2020.550225
Received: 08 April 2020; Accepted: 12 October 2020;
Published: 25 November 2020.
Edited by:
Xiancang Ma, First Affiliated Hospital of Xi'an Jiaotong University, ChinaReviewed by:
Boris B. Quednow, University of Zurich, SwitzerlandAlex Hatzimanolis, National and Kapodistrian University of Athens, Greece
Copyright © 2020 Rovný, Besterciová and Riečanský. This is an open-access article distributed under the terms of the Creative Commons Attribution License (CC BY). The use, distribution or reproduction in other forums is permitted, provided the original author(s) and the copyright owner(s) are credited and that the original publication in this journal is cited, in accordance with accepted academic practice. No use, distribution or reproduction is permitted which does not comply with these terms.
*Correspondence: Igor Riečanský, aWdvci5yaWVjYW5za3lAdW5pdmllLmFjLmF0