- Faculty of Psychology, Southwest University, Chongqing, China
Substance addiction and food addiction are significant social problems worldwide. In previous studies of substance addiction, transcranial direct current stimulation (tDCS) has been used to influence craving of substance or food. However, the reported effects are not always consistent due to inconsistent experimental settings. The way modulators influence the effect of tDCS on substance addiction is worth exploring. This meta-analysis was conducted to estimate the effect size of tDCS on substance and food craving and to investigate the influence of potential modulators. We systemically identified and reviewed studies on substance/food craving using tDCS that were published between January 2008 to January 2020. A total of 32 eligible studies were identified. Hedges' g was computed as an indicator of the effect of tDCS and some potential moderators (substance type, stimulation sites, current intensities, number of sessions, duration of stimulation, and study design) were examined using subgroup analysis. Random effects analysis revealed a total medium effect size [Hedges' g = 0.536, 95% confidence interval (CI): 0.389–0.683, after adjusting Hedges' g = 0.416, 95% CI: 0.262–0.570] preferring active over sham stimulation to reduce craving. A significant difference was observed between the number of sessions (repeated stimulation was better than single stimulation). The duration of stimulation may have a positive influence on the effects of tDCS. No other significant differences were found in other subgroups analysis. In conclusion, our results provided evidence that tDCS can be an effective way to reduce craving of substance or food, and longer multiple stimulus durations in all can more effectively reduce craving; however, the influences of modulators still need be to be examined in depth in future.
Introduction
Substance dependence (or substance addiction) is a chronic relapsing brain disorder leading patients to use a substance continuously despite the negative consequences that result from doing it (1). Many types of substances can cause addiction, such as addictive drugs including alcohol and tobacco. According to statistics released by the United Nations Office of Drug and Crimes, the percentage of individuals using cannabis, cocaine, and opioid worldwide were 3.8, 0.37, and 1.08%, respectively, in 2017 (2). Meanwhile, the harmful use of alcohol was estimated to cause 2.5 million deaths each year (3) and tobacco use causes more than five million deaths worldwide each year (4).
In the meantime, food addiction can be described as the dependence on refined foods that meets the Diagnostic and Statistical Manual of Mental Disorders (DSM-IV) substance use disorder criteria (5). Food addiction is significantly linked to obesity (6). Considering around 93.3 million US adults (7) and about 86 million people in China (8) could be classified as obese in 2016, food addiction is similar to substance addiction because food regulates body process by acting as the source of required energy and has a hedonic component that makes it an effective natural reward. Food-related rewards may promote increased intake and trigger withdrawal-related symptoms (e.g., overeating), suggesting that the behaviors parallel substance abuse (9). One possible explanation for food addiction (or overeating) is that sugar, fats, salt, caffeine, refined sweeteners, and refined carbohydrates in processed foods are addictive substances (5).
With substance addiction or food addiction, craving is dependent upon the past experience of an urge or desire to use substances (10). Reducing craving is an important aspect of treating substance dependence or food addiction. A direct method to reduce craving is pharmacotherapy (11–14), which is a long-term therapy focused on substitution or withdrawal. Because the use of medication carries a risk of damaging cardiopulmonary function (15), its application requires care. Cognitive treatments such as cognitive-behavior coping skills treatment (CBT) (16) and psychological counseling (17) are other mainstream treatments for helping patients recognize, avoid, and cope with the substance. It is often used with medications that interact with the type of psychotherapy provided (18, 19). Additionally, with the development of neural science, non-invasive neurostimulation techniques, including transcranial direct current stimulation (tDCS) and transcranial magnetic stimulation (TMS), have been widely used to reduce substance cravings (20, 21). Electromagnetic brain stimulation could regulate activity in specific brain regions. Recent studies have explored the application of non-invasive neurostimulation for the treatment of substance dependence (22–25).
Among them, tDCS, which uses a weak safe current of 1–2 mA for 3–20 min to increase (anodal tDCS) or decrease (cathodal tDCS) cortical excitability (26, 27), has a significant effect on reducing cravings (28, 29). However, the results of the effect of tDCS on drug craving are mixed. Salling et al. (30) reviewed research on brain stimulation in addiction and found that tDCS has an acute effect on drug and alcohol cravings without consistent results. Coles et al. (31) found that tDCS reduced craving and consumption for alcohol and drugs while the results for tobacco were unclear because of different stimulation methods and parameters. Some studies indicated that a single stimulation of the left dorsolateral prefrontal cortex (DLPFC) with anodal tDCS significantly reduced cravings (32–35), but other findings did not support this conclusion (36–38). This kind of inconsistency may come from different study designs, stimulation parameters, and characteristics of participants in different studies.
Considering the inconsistent findings of empirical investigations and that only a medium effect of tDCS was found in a meta-analysis, it is essential to explore potential modulators separately. In 2013, Jansen et al. (39) performed the first meta-analysis in this field (both TMS and tDCS studies included). After comparing the effects on alcohol/nicotine users and people with a high craving for food, the results indicated that craving levels were decreased in substance dependence following non-invasive neurostimulation of the prefrontal cortex and further revealed a significant medium effect size of neurostimulation, but no difference between substances, stimulation technology, or side of stimulation. Recently, another meta-analysis from Song et al. (40) reported a medium effect size of TMS and tDCS treatment on craving and consumption, making a comparison between substance type/stimulation sessions/stimulated regions and found that multi-session stimulation had larger effects. Different from existing research, this meta-analysis evaluates tDCS based on separate stimulation parameters (e.g., the stimulation site, current intensity, number of sessions, duration of single-stimulation, and total-stimulation); the duration of stimulation, in particular, has seen little prior analysis. Substance type and study design may be important modulators that need to be investigated in depth. We aimed to conduct a meta-analysis focusing on the effects of tDCS in decreasing substance/food craving, and exploring the influence of potential modulators systematically. This should help find the optimal stimulation parameters in clinical settings for drug dependence and food addiction.
Methods
An online search was conducted in the Web of Science, PubMed, and Google Scholar databases for articles published from January 2008 to January 2020. This search was implemented following the PICO-method (Patient/Population, Intervention, Comparison, Outcome) building a framework where “substance or food addiction” represented “P,” “tDCS stimulation” represented “I,” “active and sham stimulation” represented “C,” and “craving” represented “O”. The PRISMA (Preferred Reporting Items for Systematic Reviews and Meta-Analyses) guidelines were followed in this meta-analysis.
Inclusion and Exclusion Criteria for the Selection of Studies
We searched for the combination of two sets of pre-defined terms in the title or abstracts. The following terms were set as the search terms: (transcranial direct current stimulation OR tDCS) AND (substance dependence OR substance abuse OR alcohol OR drug OR tobacco OR nicotine OR eating disorder OR food addiction).
All studies included in the meta-analysis met the following inclusion criteria: 1) tDCS was used as the stimulation tool; 2) substance craving/food craving changes were measured; 3) sham stimulation as a control condition; and 4) means, standard deviations, t, F, or p statistics and the number of participants in each intervention group were provided completely as basic data in order to calculate effect size.
Studies were excluded if: 1) they were meta-analyses, reviews, meeting abstracts, or case studies; 2) the subjects had other mental disorders other than substance addiction/food addiction; 3) tDCS was used combined with other intervention strategies (e.g., cognitive training or psychotherapy); or 4) the subjects were animals.
Data Extraction
Stimulation Parameters
The most important stimulation parameters were stimulation site (left DLPFC, right DLPFC, or other area), current intensity (2 mA or 1 mA), number of stimulation sessions (single-session or multi-session), duration of single-stimulation (10–30 min), and total-stimulation (10–200 min).
Substance Type
Four types of substance dependence were analyzed: drugs (e.g., cocaine, marijuana, methamphetamine, heroin, and opium), tobacco, alcohol, and food.
Study Design
Studies included in this meta-analysis were divided into double-blind or single-blind experiments, and into parallel experiments (i.e., more than two groups of participants and each group receives different treatments) and crossover experiments (all participants receive the same treatments but in a random order).
Craving Measures
The main outcome measures included in this meta-analysis were state craving scores from different questionnaires. For each study, craving scores pre-tDCS and post-tDCS of all participants (both active and sham groups) were obtained. Effect sizes on craving levels were standardized for the effect of stimulation.
Data Analysis
The Cochrane Collaboration's risk of bias tool was used to judge the risk of bias within individual studies (41). The publications identified through this search strategy were then examined by the three researchers (JQ, JC, and ZZ) individually to confirm eligibility. If there was any disagreement, it was resolved by discussion.
General Meta-Analysis
The Comprehensive Meta-Analysis 2.0 (CMA 2.0) computer program was used to process and analyze the data. Main analyses were implemented to examine the effects of tDCS on craving. To measure the difference in craving levels between active and sham stimulation, effect sizes (Hedges' g) were calculated. According to Borenstein et al. (42), Hedges' g is regarded as a conservative estimate, which could be applied to studies regardless of sample sizes. Basing on the value of g, the results may be defined as reflecting a small (g=0.2–0.5), medium (g=0.5–0.8), or large (g>0.8) effect.
Moderator Analyses
A random-effects model was used to analyze the difference between subgroups. Compared with the fixed effects model, the random effects model interval provides an unadventurous estimate of accuracy allowing for the existence of heterogeneity, which is more suitable for generalization (42). All comparisons used an alpha of 0.05.
The meta-regression was used to identify whether the duration of stimulation might influence the effect size estimates. Since every study was weighted by the precision of their respective effect estimates, the influence on the relationship depended on the size of study. This allows for residual heterogeneity among intervention effects rather than being modeled by explanatory variables (43).
Publication Bias
Publication bias analyzes whether the decision to publish or distribute a study was influenced by its findings (44). Publication bias was assessed using Egger's regression test (45). If publication bias was identified, a trim-and-fill procedure was applied to modify the effect size caused by publication bias (46).
Results
Studies Included in the Meta-Analysis
The literature search identified 32 eligible studies (see Table 1). Figure 1 shows the flow diagram of the inclusion and exclusion process. Of the included studies, 8 for nicotine, 10 for alcohol, 7 for food, and 7 for other drug cravings. There were four studies in which subjects received two types of active anodal stimulation at different sites in independent sessions (33, 34, 57, 68). The four studies were divided into eight “units of analysis.” We adjusted for the interdependence of these data in the analyses by taking study as a unit of design instead of the unit for analyses because in this case it was difficult to distinguish differences in both design and stimulation locations (39). Therefore, there were 36 units of analysis included in total. The assessment of the risk of bias for all studies included is summarized in Supplementary Table 1.
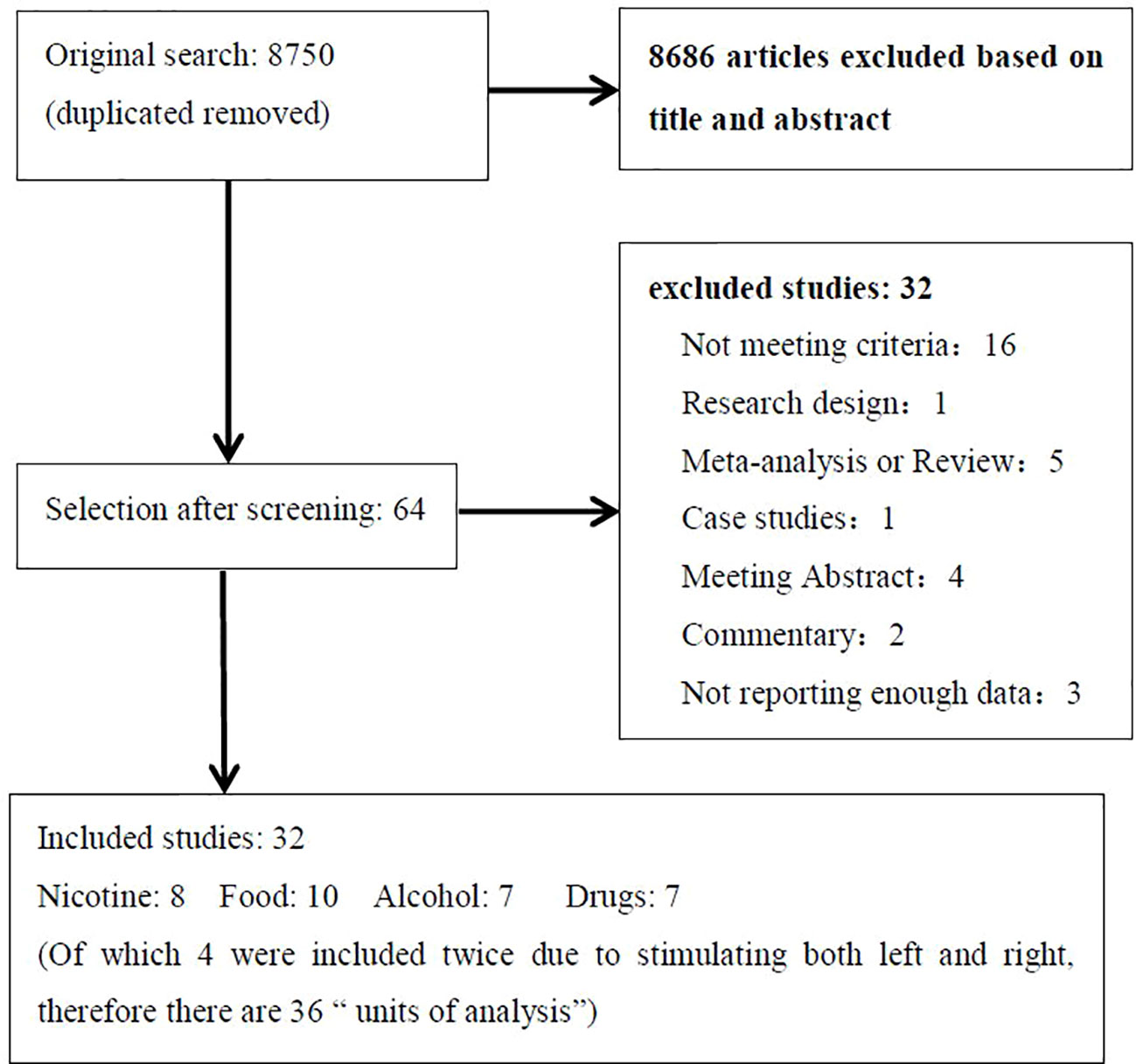
Figure 1 Flow diagram showing the search and selection procedure that was used for this meta-analysis. It showed the reasons for exclusion in “not meeting criteria” and final numbers of study included in the meta-analysis.
General Effect Size (Hedges' g) on Craving
The test for heterogeneity was significant (Q=55.44, df=31, p=0.004, I2=44.08%), showing that there was heterogeneity between the study findings. In order to address the heterogeneity problem, we used a random effects analysis for the meta-analysis because of its conservative estimate and appropriate nature for generalization (42). As shown in Figure 2, this analysis revealed a standardized effect size (Hedges' g) of 0.536 [95% confidence interval (CI): 0.389–0.683], indicating a medium effect size favoring active stimulation over sham stimulation (z=7.153, p<0.001). The variation caused by the true difference in the effect accounts for 44.08% of the total variation with medium heterogeneity in this study, which implies that there may be important potential modulating variables and subgroup analysis was needed to test for the moderating effects (74, 75).
Influence of Moderators
Stimulation Parameters (Stimulation Site, Current Intensity, Number of Sessions)
The left or right DLPFC was the anodal stimulation site in all studies except two (66, 70), which were excluded from this subgroup analysis, leaving 13 units for the left DLPFC and 21 units for the right DLPFC. Comparisons were made between the left and right DLPFC regardless of the cathodal site. The results showed that the difference between the left DLPFC and right DLPFC was not significant (Q=2.673, p=0.102) although Hedges' g for the left DLPFC was 0.402, while the Hedges' g for the right DLPFC was 0.636.
As far as current intensity was concerned, a comparison was made between 1 mA and 2 mA [six studies with 1 mA, 24 studies with 2 mA, two studies was not included because the stimulation current was 1.5 mA (66, 70)]. There was no significant difference in effect size between 1 and 2 mA (Q=1.635, p=0.201); the Hedges' g was 0.381 for 1 mA and 0.592 for 2 mA (Table 2).
A comparison was also made between the number of sessions (single-session vs. multi-session) (21 studies with one session, and 11 studies with multiple sessions). The Hedges' g for single-session stimulation was 0.443, while the Hedges' g for multi-session stimulation was 0.751 (see Figure 3). The results revealed a significant difference in effect size between the two subgroups (Q=4.261, p=0.039) (see Table 2). We found multiple session of tDCS intervention may have a better effect on craving.
Duration of Single Stimulation and All Stimulation
We performed a meta-regression analysis based on the duration of single stimulation and Hedges' g. Regarding the duration of single stimulation, we found a marginally significant result that the longer the stimulus lasts, the more the craving decreases (Q=2.832, β=0.023, p=0.092) (see Figure 4). Due to only the presence of only five values and the marginally significant result, we extended the analysis to include total stimulation time (duration of single stimulation × number of sessions) to obtain a more credible result. Regarding total stimulation duration, we found that longer stimulation was related to greater effect size (Q=8.505, β=0.003, p=0.004) (see Figure 5).
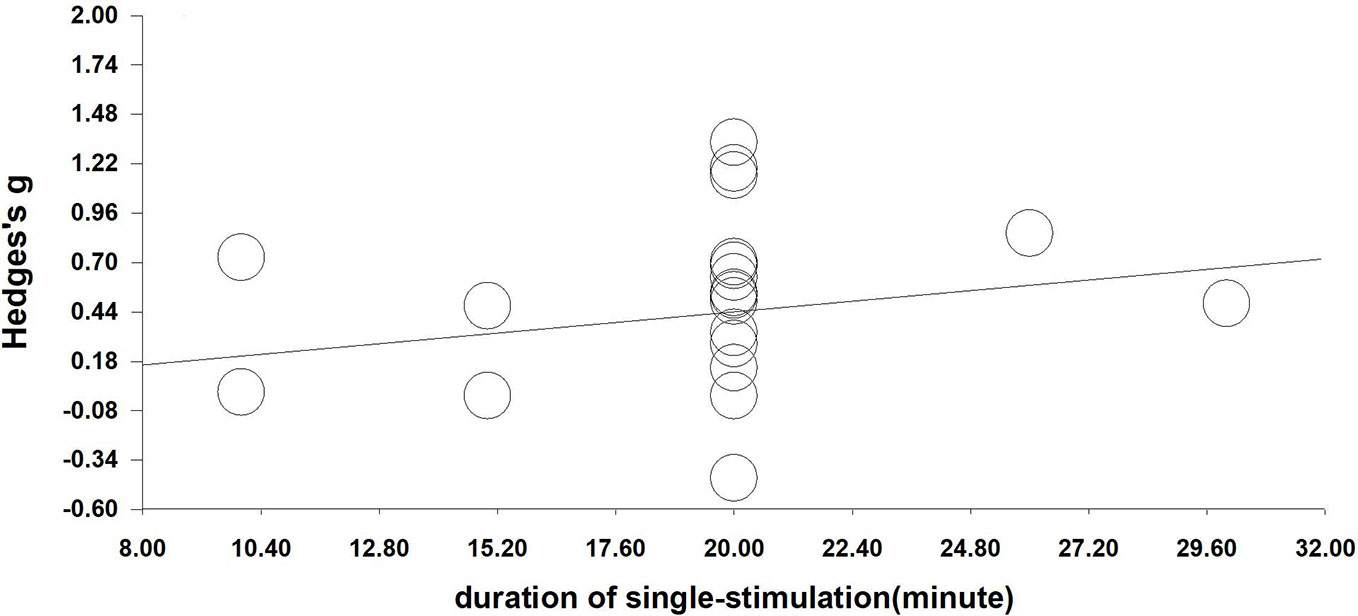
Figure 4 Regression of the duration of single-stimulation on the effect size of neuromodulation of craving. (Q=2.832, β=0.023, p=0.092).
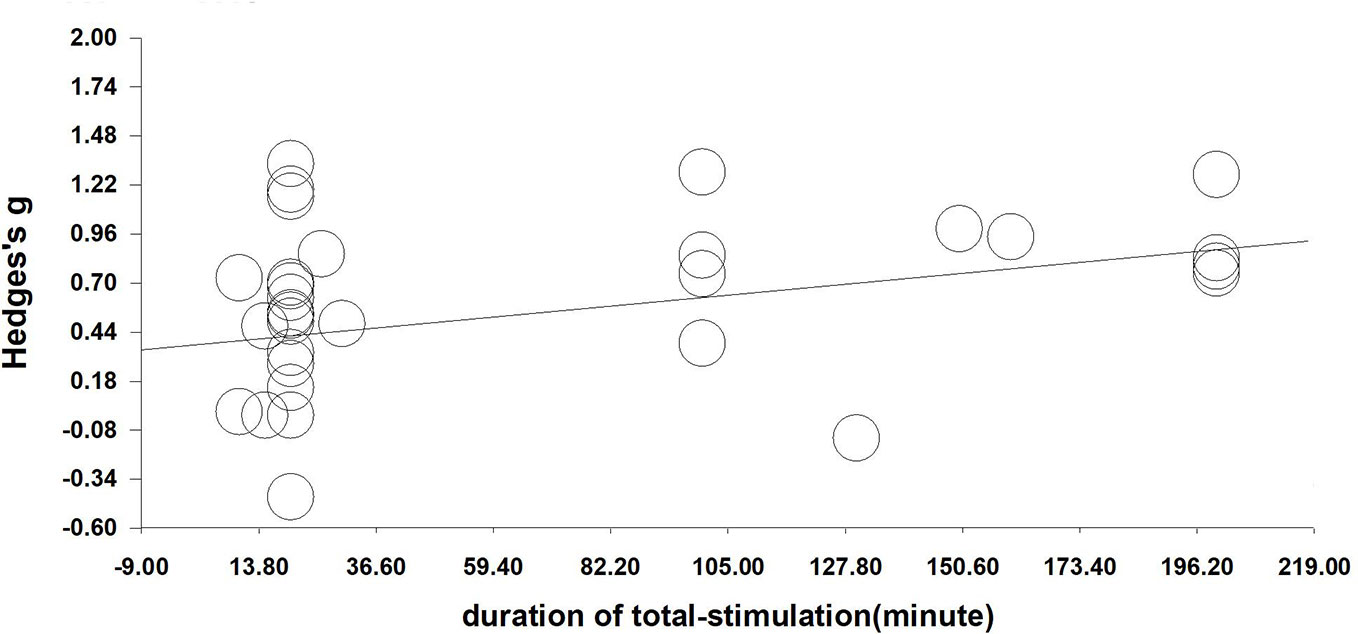
Figure 5 Regression of sessions on the effect size of neuromodulation of craving. (Q=8.505, β=0.003, p=0.004).
Substance Type
We compared the results for alcohol users, nicotine users, drug users, and food bingers. The results did not show a significant difference in effect size between substance types [Q (3)=4.121, p=0.249] (see Table 2).
When substance type was divided into two categories, substance and food, where alcohol, nicotine, and drugs were combined into substance, the Hedges' g for substance was 0.616, while the Hedges' g for food was 0.371. However, there was no significant difference between substance and food (Q=2.474, p=0.116).We also compared food with other substance types separately, and found that there was an obvious difference between drugs and food (Q=4.094, p=0.043), but no difference between alcohol and food nor nicotine and food.
Study Design (Crossover-Design or Parallel-Design; Double-Blind or Single-Blind)
A comparison was made between study designs. No significant difference was found between crossover and parallel designs (Q=0.913, p=0.339) or between double and single blind designs (Q=0.405, p=0.524) (see Table 2). However, we found that the number of sessions may influence the results because most (8/11) multiple stimulation studies were parallel designs. Therefore, we compared the effects of the crossover design and parallel design by using the number of sessions as a covariate. The result confirmed no significant difference in effect size between crossover and parallel designs (Q=1.77, p=0.183).
Evaluation of Publication Bias
Egger's regression test was performed to empirically examine the presence of any publication bias. A publication bias was observed (p=0.031), as shown in Figure 6. A funnel plot was created (76) in which the measure of precision (standard error) of the effect size (Hedges' g) was shown with a trim-and-fill procedure applied (46). The results of the trim and fill method highlighted that there are seven “missing” effect sizes on the left side of the funnel plot. After adjustment, the analysis indicated an average effect size of 0.416 (95% CI: 0.262–0.570), which was comparable to the original result (Hedges' g=0.536), suggesting that the publication bias influenced our results lightly.
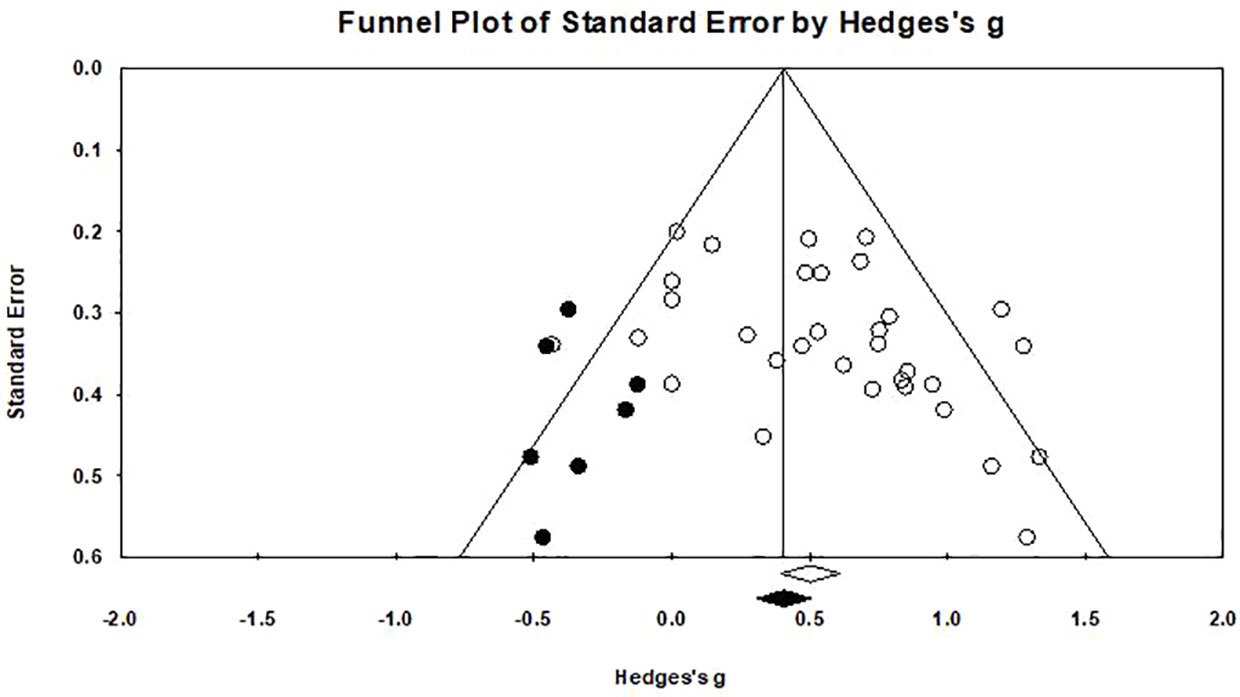
Figure 6 Funnel plot for a model without moderators (random-effects model), the solid circles in the funnel plot represents the seven studies that were trimmed to the left. The solid diamond on the abscissa indicate that the correction effect size is 0.416, and the hollow diamond on the right represents the original effect size is 0.536.
Discussion
Based on 32 empirical studies, we performed a meta-analysis to review the effect of tDCS on substance craving and the influence of potential moderators. The results revealed a significant medium effect size (Hedges' g=0.536 or 0.416 after adjusting for publication bias) favoring real tDCS stimulation over sham stimulation in the reduction of craving. Furthermore, the number of sessions could significantly influence the effect of tDCS, favoring multi-session over single-session treatment. Other modulators appear to have no influence on craving reduction, such as stimulation site, current intensity, substances type, or study design.
Effect of Transcranial Direct Current Stimulation on Reducing Craving and Its Possible Mechanism
Our findings confirmed that there was significant decrease in craving after tDCS stimulation of the DLPFC, which supports previous findings (39, 40, 77, 78).
One possible mechanism by which DLPFC stimulation decreases craving is an increase in “cognitive control.” As we know, the executive control network (ECN, including the DLPFC, orbitofrontal cortex, and anterior cingulate cortex) plays an important role in human executive control (79–81), including craving control (82, 83). tDCS can induce and regulate neural plasticity (84). Bilateral tDCS with anodal stimulation of the right DLPFC increases the intra-network functional connectivity (71). Cavaliere et al. (85) also showed that anodal tDCS over the DLPFC increases intra-network co-activation of the ECN. Thus, increased ECN activity and functional connectivity of the ECN could help individuals to decline or reduce craving—in other words, after changing the activity of the DLPFC subjects were able to better suppress their urges through its connections to the ECN.
Stimulation of the prefrontal cortex-stimulated dopaminergic pathways is another explanation. Addicted subjects were hardly roused by nondrug-related stimuli other than the substance they were addicted to, with decreased dopamine function disrupting frontal inhibition (86). Researchers have found that DLPFC stimulation alters the activation and functional connectivity of the cortical and subcortical reward systems in healthy individuals (87). Simultaneously, the membrane potential of pyramidal cells are regulated by anodal and cathodal tDCS, which alter the glutamate tone in the cortex, which is considered to correlate with GABA release, to restore the best excitation/inhibition balance to achieve the best steady-state plasticity in learning and cognition (88). Thus, tDCS stimulation of the DLPFC may improve the behavior of substance-dependent individuals by modulating the activities of brain regions such as the anterior cingulate cortex (ACC), the orbitofrontal cortex (OFC), and caudate nucleus.
However, it is not clear how tDCS affects substance and food craving. Further studies are needed to explore the psychological process and neural mechanisms underlying tDCS stimulation at the same time. A promising method can be seen in studies that use functional magnetic resonance imaging (fMRI) to explore the changes caused by tDCS stimulation (87, 89).
Influence of Moderators
Among all the stimulation parameters, our subgroup analyses showed that multi-session tDCS had a significant greater effect on reducing craving than single-session tDCS, which is in agreement with Song et al.'s (40) and Kim et al.'s (77) findings. This suggests that the effects of tDCS on craving reduction can be cumulative. However, it is worth noting that the multiple stimulations included in the meta-analysis were all performed separately and not on the same day, because one study reported there was no benefit of twice-daily stimulation over once-daily stimulation in increasing cortical excitability (90).
Although not significant, we found a trending positive influence of the duration of single stimulations (p=0.092). The non-significant results may be because there were sufficient data in the analysis. After calculating the total stimulation duration, there was a trend that longer durations were related to larger Hedges' g (p=0.004). The result is consistent with the finding that the duration of stimulation may enhance its efficacy in given applications (91). However, more evidence of the effect of stimulation duration is needed in future.
According to the substance type subgroup analyses, the effects on drug users were the biggest (Hedges' g=0.742), followed by alcohol and nicotine (Hedges' g=0.587 and Hedges' g=0.555), and then food (Hedges' g=0.371), suggesting that the process of food addiction may differ from substance addiction. In some study, food addiction has a bearing on stress exposure with damage to the hippocampus (92) and patients with food addiction were easily affected by a sad mood (93). Stress, cognition, and emotion recognition may play a more important role in food addiction than other addictions meaning the treatment of food addiction may be more complex.
As for other factors, since the beneficial effects of tDCS vary with the pathology (94), we speculate that tDCS may be more effective for severely addictive substances than lightly addictive substances. That is to say that the severity of addiction may be a factor influencing the effect size of tDCS. However, the severity of addiction cannot be determined in the current study because there is no consistent standard for severity across various addictions and we have difficulty extracting effective data to distinguish the severity of addiction in the current literature.
Although we tried to explore various regulatory variables, there are still some possible influencing variables, which are not discussed in this paper, such as tDCS combined with other treatments (cognitive bias modification, emotional regulation). tDCS combined with cognitive bias modification has shown limited effect on treatment outcomes (95–97). Moreover, emotional regulation can help the curative effects of tDCS (98–101); after emotion regulation training, using tDCS has better results (100). Thus, different substance addictions may affect the participants' emotional management, in turn altering the effects of tDCS. Due to the limited number of studies and the great heterogeneity among the literature, they were not included in the current meta-analysis. However, these works are of great significance and may be a new trend for tDCS research that deserves further research.
Regarding stimulation site, we found that there was no difference between right/anodal, left/cathodal and left/anodal, right/cathodal protocols for substance, and food dependence, supporting the conclusion of Jansen et al. (39) that putting the anode on the left or right would not affect the results of treating addictive disorders. However, although not significant, we could not ignore that the right DLPFC may be a better choice for most individuals because of the higher Hedges' g (0.613) than that of the left DLPFC (0.402). Boggio et al. (68) showed that only the right anode + left cathode montage was significantly associated with a reduction of craving for marijuana. It seems there may have the possibility that the effect of stimulation site will depend on the health status of the subject and the type of substance dependence. Other parameters, including current intensity (1 vs. 2 mA), study design (crossover or parallel, and double blind or single blind) had no significant difference.
Limitations and Future Directions
In this meta-analysis, some limitations should be considered. First, the number of libraries and articles available was limited. Although we searched for accessible published articles, we still could not be sure that all relevant research was included. Second, the main effect of tDCS is only a medium effect size, suggesting more empirical studies are needed to explore the modulators of tDCS treatment of craving control, e.g., the substance type, addiction severity, and abstinence duration. Unfortunately, the majority of included studies did not report the abstinence duration or addiction severity; therefore, we could not examine its moderating effect in tDCS performed for craving control. Thus, an in-detail report of dependence-related data would be very important in the future. Third, the tools for measuring cravings need to be improved. In most studies, craving was measured using self-report questionnaires or visual analogue scales, which are subject to socially desirable answers. Some objective methods to measure drug craving (e.g., physiological measurement) would be very helpful in investigating the effect size of craving reduction.
Conclusion
The current meta-analysis provided evidence that tDCS protocols improved the symptoms of substance and food dependence as indicated by reduced craving, but some modulators does influence the effect of tDCS treatment on addiction. In general, our findings suggested that 2 mA stimulation may be better than 1 mA; multi-session interventions may be better than single-session. We also suggested that tDCS may be not ideal for the treatment of food addiction, implicating that compared with substance addiction, food addiction may be different in nature and needs complex treatment such as tDCS combined with psychological interventions. In clinic, the optimal treatment plan for any specific type of addiction still needs be to be examined in depth in future.
Data Availability Statement
All datasets generated for this study are included in the article/Supplementary Material.
Author Contributions
Conceiving of the design framework: JC and ZZ. Writing the manuscript: JC and JQ. Revising the manuscript: JC, JQ, QH and ZZ.
Funding
This work was supported by the Fundamental Research Funds for Central Universities (#SWU1809006).
Conflict of Interest
The authors declare that the research was conducted in the absence of any commercial or financial relationships that could be construed as a potential conflict of interest.
Supplementary Material
The Supplementary Material for this article can be found online at: https://www.frontiersin.org/articles/10.3389/fpsyt.2020.00598/full#supplementary-material
References
1. Kalivas PW, Volkow ND. The neural basis of addiction: a pathology of motivation and choice. Am J Psychiatry (2005) 162, no. 8:1403–13. doi: 10.1176/appi.ajp.162.8.1403
2. United Nations Office on Drugs and Crime (UNODC). (2017). Annual prevalence of the use of cannabis, cocaine, opioids, opiates, amphetamine-type stimulants and “ecstasy” by region and globally. UNODC. https://dataunodc.un.org/drugs/prevalence_regional-2017.
3. World Health Organization. (2009). Global strategy to reduce harmful use of alcohol: report on the WHO regional technical consultation, 24-26 February 2009, Nonthaburi, Thailand. No. SEA-Ment-161. WHO Regional Office for South-East Asia. , https://apps.who.int/iris/handle/10665/205143.
4. ITC Project, World Health Organization, World Heart Federation. Cardiovascular harms from tobacco use and secondhand smoke: Global gaps in awareness and implications for action. Waterloo, Ontario, Canada and Geneva, Switzerland (2012).
5. Ifland JR, Preuss HG, Marcus MT, Rourke KM, Taylor WC, Burau K, et al. Refined food addiction: a classic substance use disorder. Med Hypotheses (2009) 72(5):518–26. doi: 10.1016/j.mehy.2008.11.035
6. Pedram P, Wadden D, Amini P, Gulliver W, Randell E, Cahill F, et al. Food addiction: its prevalence and significant association with obesity in the general population. PloS One (2013) 8(9):e74832. doi: 10.1371/journal.pone.0074832
7. Hales CM, Carroll MD, Fryar CD, Ogden CL. Prevalence of obesity among adults and youth: United States, 2015–2016. Centers for Disease Control and Prevention (2017). Available at: https://www.cdc.gov/nchs/data/databriefs/db288.pdf.
8. World Health Organization (WHO). Prevalence of obesity among adults, BMI >= 30 (age-standardized estimate) (%) https://www.who.int/data/gho/data/indicators/indicator-details/GHO/prevalence-of-obesity-among-adults-bmi-=-30-(age-standardized-estimate)-(-).
9. Hone-Blanchet A, Fecteau S. Overlap of food addiction and substance use disorders definitions: analysis of animal and human studies. Neuropharmacology (2014) 85:81–90. doi: 10.1016/j.neuropharm.2014.05.019
10. Witkiewitz K, Bowen S, Douglas H, Sharon HH. Mindfulness-based relapse prevention for substance craving. Addictive Behav (2013) 38(2):1563–71. doi: 10.1016/j.addbeh.2012.04.001
11. Dole VP, Nyswander M. A medical treatment for diacetylmorphine (heroin) addiction: a clinical trial with methadone hydrochloride. Jama (1965) 193(8):646–50. doi: 10.1001/jama.1965.03090080008002
12. D'Onofrio G, O'Connor PG, Pantalon MV, Chawarski MC, Busch SH, Owens PH, et al. Emergency department–initiated buprenorphine/naloxone treatment for opioid dependence: a randomized clinical trial. Jama (2015) 313(16):1636–44. doi: 10.1001/jama.2015.3474
13. Molyneux A, Lewis S, Leivers U, Anderton A, Antoniak M, Brackenridge A, et al. Clinical trial comparing nicotine replacement therapy (NRT) plus brief counselling, brief counselling alone, and minimal intervention on smoking cessation in hospital inpatients. Thorax (2003) 58(6):484–8. doi: 10.1136/thorax.58.6.484
14. Miller NS, Gold MS. Management of withdrawal syndromes and relapse prevention in drug and alcohol abuse. Am Family Physician (1998) 58(1):139.
15. van Dorp EL, Yassen A, Dahan A. Naloxone treatment in opioid addiction: the risks and benefits. Expert Opin Drug Safety (2007) 6(2):125–32. doi: 10.1517/14740338.6.2.125
16. Carroll KM. A cognitive-behavioral approach: Treating cocaine addiction. Bethesda, MD: National Institute on Drug Abuse, Department of Health and Human Services (1998). NIH Publication Number 98–4308.
17. Stanton MD, Todd TC eds. The family therapy of drug abuse and addiction. New York: Guilford (1982).
18. Prochaska JJ, Benowitz NL. The past, present, and future of nicotine addiction therapy. Annu Rev Med (2016) 67:467–86. doi: 10.1146/annurev-med-111314-033712
19. Swift RM. Drug therapy for alcohol dependence. New Engl J Med (1999) 340(19):1482–90. doi: 10.1146/annurev-med-111314-033712
20. Xuejiao Li, Zou Z. The application of transcranial direct current stimulation (tDCS) in the treatment of substance dependence. Adv Psychol Sci (2016) 24(9):1398–408. doi: 10.3724/SP.J.1042.2016.01398
21. Valero-Cabré A, Amengual JL, Stengel C, Pascual-Leone A, Coubard OA. Transcranial magnetic stimulation in basic and clinical neuroscience: A comprehensive review of fundamental principles and novel insights. Neurosci Biobehav Rev (2017) 83:381–404. doi: 10.1016/j.neubiorev.2017.10.006
22. Kuhn J, Möller M, Lenartz D, Bührle CP, Visser-Vandewalle V. “Neuromodulation for addiction.” In: Textbook of neuromodulation. New York, NY: Springer (2015). p. 247–55. doi: 10.1007/978-1-4939-1408-1_17
23. Feil J, Zangen A. Brain stimulation in the study and treatment of addiction. Neurosci Biobehav Rev (2010) 34, no. 4:559–74. doi: 10.1016/j.neubiorev.2009.11.006
24. Gorelick DA, Zangen A, . George MS. Transcranial magnetic stimulation (TMS) in the treatment of substance addiction. Ann New Y Acad Sci (2014) 1327(1):79–93. doi: 10.1111/nyas.12479
25. Hone-Blanchet ,A, Fecteau S. “The use of non-invasive brain stimulation in drug addictions.” In: The stimulated brain. Academic Press (2014). p. 425–52. doi: 10.1016/B978-0-12-404704-4.00015-6
26. Andre Russowsky B, Amadera J, Berbel B, Sarah Volz M, Rizzerio BG, Fregni F. A systematic review on reporting and assessment of adverse effects associated with transcranial direct current stimulation. Int J Neuropsychopharmacol (2011) 14(8):1133–45. doi: 10.1017/S1461145710001690
27. Nitsche MA, Paulus W. Excitability changes induced in the human motor cortex by weak transcranial direct current stimulation. J Physiol (2000) 527(3):633–9. doi: 10.1111/j.1469-7793.2000.t01-1-00633.x
28. Lupi M, Martinotti G, Santacroce R, Cinosi E, Carlucci M, Marini S, et al. Transcranial direct current stimulation in substance use disorders: a systematic review of scientific literature. J ECT (2017) 33(3):203–9. doi: 10.1097/YCT.0000000000000401
29. Wing VC, Barr MS, Wass CE, Lipsman N, Lozano AM, Daskalakis ZJ, et al. Brain stimulation methods to treat tobacco addiction. Brain Stimulation (2013) 6(3):221–30. doi: 10.1016/j.brs.2012.06.008
30. Salling MC, Martinez D. Brain stimulation in addiction. Neuropsychopharmacology (2016) 41(12):2798–809. doi: 10.1038/npp.2016.80
31. Coles AS, Kozak K, George TP. A review of brain stimulation methods to treat substance use disorders. Am J Addict (2018) 27(2):71–91. doi: 10.1111/ajad.12674
32. Falcone M, Bernardo L, Ashare RL, Hamilton R, Faseyitan O, McKee SA, et al. Transcranial direct current brain stimulation increases ability to resist smoking. Brain Stimulation (2016) 9(2):191–6. doi: 10.1016/j.brs.2015.10.004
33. Boggio PS, Sultani N, Fecteau S, Merabet L, Mecca T, Pascual-Leone A, et al. Prefrontal cortex modulation using transcranial DC stimulation reduces alcohol craving: a double-blind, sham-controlled study. Drug Alcohol Depend (2008) 92(1-3):55–60. doi: 10.1016/j.drugalcdep.2007.06.011
34. Fregni F, Liguori P, Fecteau S, Nitsche MA, Pascual-Leone A, Boggio PS. Cortical stimulation of the prefrontal cortex with transcranial direct current stimulation reduces cue-provoked smoking craving: a randomized, sham-controlled study. J Clin Psychiatry (2008) 69(1):32–40. doi: 10.4088/JCP.v69n0105
35. da Silva MC, Conti CL, Klauss J, Alves LG, Mineiro do Nascimento Cavalcante H, Fregni F, et al. Behavioral effects of transcranial direct current stimulation (tDCS) induced dorsolateral prefrontal cortex plasticity in alcohol dependence. J Physiology-Paris (2013) 107(6):493–502. doi: 10.1016/j.jphysparis.2013.07.003
36. Kroczek AM, Häußinger FB, Rohe T, Schneider S, Plewnia C, Batra A, et al. Effects of transcranial direct current stimulation on craving, heart-rate variability and prefrontal hemodynamics during smoking cue exposure. Drug Alcohol Depend (2016) 168:123–7. doi: 10.1016/j.drugalcdep.2016.09.006
37. Nakamura-Palacios EM, de Almeida Benevides MC, da Penha Zago-Gomes M, de Oliveira RW, de Vasconcellos VF, de Castro LN, et al. Auditory event-related potentials (P3) and cognitive changes induced by frontal direct current stimulation in alcoholics according to Lesch alcoholism typology. Int J Neuropsychopharmacol (2012) 15(5):601–16. doi: 10.1017/S1461145711001040
38. Jiansong Xu, Fregni F, Brody AL, Rahman AS. Transcranial direct current stimulation reduces negative affect but not cigarette craving in overnight abstinent smokers. Front Psychiatry (2013) 4:112. doi: 10.3389/fpsyt.2013.00112
39. Jansen JM, Daams JG, Koeter MWJ, Veltman DJ, van den Brink W, Goudriaan AE. Effects of non-invasive neurostimulation on craving: a meta-analysis. Neurosci Biobehav Rev (2013) 37(10):2472–80. doi: 10.1016/j.neubiorev.2013.07.009
40. Song S, Zilverstand A, Gui W, Li H-j, Zhou X. Effects of single-session versus multi-session non-invasive brain stimulation on craving and consumption in individuals with drug addiction, eating disorders or obesity: A meta-analysis. Brain Stimulation (2019) 12(3):606–18. doi: 10.1016/j.brs.2018.12.975
41. Higgins JPT, Altman DG, Gøtzsche PC, Jüni P, Moher D, Oxman AD, et al. The Cochrane Collaboration’s tool for assessing risk of bias in randomised trials. BMJ (2011) 343:d5928. doi: 10.1136/bmj.d5928
42. Borenstein M, Hedges LV, Higgins JPT, Rothstein HR. Introduction to meta-analysis. John Wiley & Sons (2011).
43. Thompson SG, Sharp SJ. Explaining heterogeneity in meta-analysis: a comparison of methods. Stat Med (1999) 18(20):2693–708. doi: 10.1002/(SICI)1097-0258(19991030)18:20<2693::AID-SIM235>3.0.CO;2-V
44. Song F, Parekh S, Hooper L, Loke YK, Ryder J, Sutton AJ, et al. Dissemination and publication of research findings: an updated review of related biases. Health Technol Assess (2010) 14(8):1–193. doi: 10.3310/hta14080
45. Egger M, Smith GD, Schneider M, Minder C. Bias in meta-analysis detected by a simple, graphical test. Bmj (1997) 315(7109):629–34. doi: 10.1136/bmj.315.7109.629
46. Duval S, Richard T. Trim and fill: a simple funnel-plot–based method of testing and adjusting for publication bias in meta-analysis. Biometrics (2000) 56(2):455–63. doi: 10.1111/j.0006-341X.2000.00455.x
47. Boggio PS, Liguori P, Sultani N, Rezende L, Fecteau S, Fregni F Cumulative priming effects of cortical stimulation on smoking cue-induced craving. Neurosci Lett (2009) 463(1):82–6. doi: 10.1016/j.neulet.2009.07.041
48. Fecteau S, Agosta S, Hone-Blanchet A, Fregni F, Boggio P, Ciraulo D, et al. Modulation of smoking and decision-making behaviors with transcranial direct current stimulation in tobacco smokers: a preliminary study. Drug Alcohol Depend (2014) 140:78–84. doi: 10.1016/j.drugalcdep.2014.03.036
49. Yang LZ, Shi B, Li H, Zhang W, Liu Y, Wang H, et al. Electrical stimulation reduces smokers’ craving by modulating the coupling between dorsal lateral prefrontal cortex and parahippocampal gyrus. Soc Cogn Affect Neurosci (2017) 12(8):1296–302. doi: 10.1093/scan/nsx055
50. Mondino M, Luck D, Grot S, Januel D, Suaud-Chagny MF, Poulet E, et al. Effects of repeated transcranial direct current stimulation on smoking, craving and brain reactivity to smoking cues. Sci Rep (2018) 8(1):1–1. doi: 10.1038/s41598-018-27057-1
51. Hajloo N, Pouresmali A, Goradel JA, Mowlaie M. The Effects of Transcranial Direct Current Stimulation of Dorsolateral Prefrontal Cortex on Reduction of Craving in Daily and Social Smokers. Iran J Psychiatry (2019) 14(4):291. doi: 10.18502/ijps.v14i4.1979
52. da Silva MC, Conti CL, Klauss J, Alves LG, do Nascimento Cavalcante HM, Fregni F, et al. Behavioral effects of transcranial direct current stimulation (tDCS) induced dorsolateral prefrontal cortex plasticity in alcohol dependence. J Physiol Paris (2013) 107(6):493–502. doi: 10.1016/j.jphysparis.2013.07.003
53. Klauss J, Penido Pinheiro LC, Silva Merlo BL, Correia Santos GD, Fregni F, Nitsche MA, et al. A randomized controlled trial of targeted prefrontal cortex modulation with tDCS in patients with alcohol dependence. Int J Neuropsychopharmacol (2014) 17(11):1793–803. doi: 10.1017/S1461145714000984
54. den Uyl TE, Gladwin TE, Wiers RW. Transcranial direct current stimulation, implicit alcohol associations and craving. Biol Psychol (2015) 105:37–42. doi: 10.1016/j.biopsycho.2014.12.004
55. Wietschorke K, Lippold J, Jacob C, Polak T, Herrmann MJ. Transcranial direct current stimulation of the prefrontal cortex reduces cue-reactivity in alcohol-dependent patients. J Neural Transm (2016) 123(10):1173–8. doi: 10.1007/s00702-016-1541-6
56. Klauss J, Anders QS, Felippe LV, Nitsche MA, Nakamura-Palacios EM. Multiple sessions of transcranial direct current stimulation (tDCS) reduced craving and relapses for alcohol use: A randomized placebo-controlled trial in alcohol use disorder. Front Pharmacol (2018) 9:716. doi: 10.3389/fphar.2018.00716
57. Fregni F, Orsati F, Pedrosa W, Fecteau S, Tome FAM, Nitsche MA, et al. Transcranial direct current stimulation of the prefrontal cortex modulates the desire for specific foods. Appetite (2008) 51:(1):34–41. doi: 10.1016/j.appet.2007.09.016
58. Goldman RL, Borckardt JJ, Frohman HA, O’Neil PM, Madan A, Campbell LK, et al. Prefrontal cortex transcranial direct current stimulation (tDCS) temporarily reduces food cravings and increases the self-reported ability to resist food in adults with frequent food craving. Appetite (2011) 56(3):741–6. doi: 10.1016/j.appet.2011.02.013
59. Kekic M, McClelland J, Campbell I, Nestler S, Rubia K, David AS, et al. The effects of prefrontal cortex transcranial direct current stimulation (tDCS) on food craving and temporal discounting in women with frequent food cravings. Appetite (2014). 78:55–62. doi: 10.1016/j.appet.2014.03.010
60. Lapenta OM, Di Sierve K, de Macedo EC, Fregni F, Boggio PS. Transcranial direct current stimulation modulates ERP-indexed inhibitory control and reduces food consumption. Appetite (2014) 83:42–8. doi: 10.1016/j.appet.2014.08.005
61. Jauch-Chara K, Kistenmacher A, Herzog N, Schwarz M, Schweiger U, Oltmanns KM. Repetitive electric brain stimulation reduces food intake in humans. Am J Clin Nutr (2014) 100(4):1003–9. doi: 10.3945/ajcn.113.075481
62. Georgii C, Goldhofer P, Meule A, Blechert J. Food craving, food choice and consumption: The role of impulsivity and sham-controlled tDCS stimulation of the right dlPFC. Physiol Behav (2017) 177:20–6. doi: 10.1016/j.physbeh.2017.04.004
63. Montenegro RA, Okano AH, Cunha FA, Gurgel JL, Fontes EB, Farinatti PT. Prefrontal cortex transcranial direct current stimulation associated with aerobic exercise change aspects of appetite sensation in overweight adults. Appetite (2012) 58(1):333–8. doi: 10.1016/j.appet.2011.11.008
64. Ray MK, Sylvester MD, Osborn L, Helms J, Turan B, Burgess EE, et al. The critical role of cognitive-based trait differences in transcranial direct current stimulation (tDCS) suppression of food craving and eating in frank obesity. Appetite (2017) 116:568–74. doi: 10.1016/j.appet.2017.05.046
65. Ray MK, Sylvester MD, Osborn L, Helton A, Pittman BR, Wagstaff LE, McRae III TR, et al. The effect of expectation on transcranial direct current stimulation (tDCS) to suppress food craving and eating in individuals with overweight and obesity. Appetite (2019) 136:1–7. doi: 10.1016/j.appet.2018.12.044
66. Chen S, Jackson T, Dong D, Zhang X, Chen H. Exploring effects of single-session anodal tDCS over the inferior frontal gyrus on responses to food cues and food cravings among highly disinhibited restrained eaters: A preliminary study. Neurosci Lett (2019) 706:211–6. doi: 10.1016/j.neulet.2019.05.035
67. Batista EK, Klauss J, Fregni F, Nitsche MA, Nakamura-Palacios EM. A randomized placebo-controlled trial of targeted prefrontal cortex modulation with bilateral tDCS in patients with crack-cocaine dependence. Int J Neuropsychopharmacol (2015) 18(12):pyv066. doi: 10.1093/ijnp/pyv066
68. Boggio PS, Zaghi S, Villani AB, Fecteau S, Pascual-Leone A, Fregni F. Modulation of risk-taking in marijuana users by transcranial direct current stimulation (tDCS) of the dorsolateral prefrontal cortex (DLPFC). Drug Alcohol Depend (2010) 112(3):220–5. doi: 10.1016/j.drugalcdep.2010.06.019
69. Shahbabaie A, Golesorkhi M, Zamanian B, Ebrahimpoor M, Keshvari F, Nejati V, et al. State dependent effect of transcranial direct current stimulation (tDCS) on methamphetamine craving. Int J Neuropsychopharmacol (2014) 17(10):1591–8. doi: 10.1017/S1461145714000686
70. Wang Y, Shen Y, Cao X, Shan C, Pan J, He H, et al. Transcranial direct current stimulation of the frontal-parietal-temporal area attenuates cue-induced craving for heroin. J Psychiatr Res (2016) 79:1–3. doi: 10.1016/j.jpsychires.2016.04.001
71. Shahbabaie A, Ebrahimpoor M, Hariri A, . Nitsche MA, Hatami J, Fatemizadeh E, et al. Transcranial DC stimulation modifies functional connectivity of large-scale brain networks in abstinent methamphetamine users. Brain Behav (2018) 8(3):e00922. doi: 10.1002/brb3.922
72. Taremian F, Nazari S, Moradveisi L, Moloodi R. Transcranial direct current stimulation on opium craving, depression, and anxiety: a preliminary study. J ECT (2019) 35(3):201–6. doi: 10.1097/YCT.0000000000000568
73. Rohani Anaraki M, Dolatshahi B, Nosratabadi M, Nouri Yalghouzaghaji M, Rezaei Mashhadi S. Repeated Transcranial Direct Current Stimulation (tDCS) on Methamphetamine Craving: A Randomized, Sham-controlled Study. Iran Rehabil J (2019) 17(4):385–94. doi: 10.32598/irj.17.4.385
74. Cooper HM. Integrating research: A guide for literature reviews. Sage Publications, Inc. (1989).
75. Higgins JPT, Thompson SG, Deeks JJ, Altman DG. Measuring inconsistency in meta-analyses. Bmj (2003) 327(7414):557–60. doi: 10.1136/bmj.327.7414.557
76. Sterne JAC, Egger M. Funnel plots for detecting bias in meta-analysis: guidelines on choice of axis. J Clin Epidemiol (2001) 54(10):1046–55. doi: 10.1016/S0895-4356(01)00377-8
77. Kim J, Iwata Y, Plitman E, Caravaggio F, Ku Chung J, Shah P, et al. A meta-analysis of transcranial direct current stimulation for schizophrenia:Is more better? J Psychiatr Res (2019) 110:117–26. doi: 10.1016/j.jpsychires.2018.12.009
78. Mostafavi S-A, Khaleghi A, Mohammadi MR, Akhondzadeh S. Is transcranial direct current stimulation an effective modality in reducing food craving? A systematic review and meta-analysis. Nutr Neurosci (2020) 23(1):55–67. doi: 10.1080/1028415X.2018.1470371
79. Miller EK, Cohen JD. An integrative theory of prefrontal cortex function. Annu Rev Neurosci (2001) 24(1):167–202. doi: 10.1146/annurev.neuro.24.1.167
80. Boettiger CA, Chanon VW, Kelm MK. Brain mechanisms of addiction treatment effects. Biol Res Addict (2013) 2:431–9. doi: 10.1016/B978-0-12-398335-0.00043-1
81. Wu L, Soder RB, Schoemaker Dorothée, Carbonnell F, Sziklas V, Rowley J, et al. Resting state executive control network adaptations in amnestic mild cognitive impairment. J Alzheimer's Dis (2014) 40(4):993–1004. doi: 10.3233/JAD-131574
82. Naqvi NH, Bechara A. The hidden island of addiction: the insula. Trends Neurosci (2009) 32(1):56–67. doi: 10.1016/j.tins.2008.09.009
83. Fedota JR, Stein EA. Resting-state functional connectivity and nicotine addiction: prospects for biomarker development. Ann New Y. Acad Sci (2015) 1349(1):64–82. doi: 10.1111/nyas.12882
84. Kuo M-F, Paulus W, Nitsche MA. Therapeutic effects of non-invasive brain stimulation with direct currents (tDCS) in neuropsychiatric diseases. Neuroimage (2014) 85:948–60. doi: 10.1016/j.neuroimage.2013.05.117
85. Cavaliere C, Aiello M, Di Perri C, Amico E, Martial C, Thibaut A, et al. “Functional connectivity substrates for tDCS response in minimally conscious state patients. Front Cell Neurosci (2016) 10:257. doi: 10.3389/fncel.2016.00257
86. Volkow ND, Fowler JS, Wang GJ, Swanson JM. Dopamine in drug abuse and addiction: results from imaging studies and treatment implications. Mol Psychiatry (2004) 9(6):557–69. doi: 10.1038/sj.mp.4001507
87. Weber MJ, Messing SB, Rao H, Detre JA, L. Thompson-Schill S. Prefrontal transcranial direct current stimulation alters activation and connectivity in cortical and subcortical reward systems: A tDCS-fMRI study. Hum Brain Mapp (2014) 35(8):3673–86. doi: 10.1002/hbm.22429
88. Krause ,B, Márquez-Ruiz J, Kadosh RC. The effect of transcranial direct current stimulation: a role for cortical excitation/inhibition balance? Front Hum Neurosci (2013) 7:602. doi: 10.3389/fnhum.2013.00602
89. Bin S, Yang L-Z, Liu Y, Zhang X. Neural mechanism by which transcranial direct current stimulation reduces cigarette cravings: study protocol for a randomized controlled crossover trial. Asia Pac J Clin Trials: Nerv Syst Dis (2018) 3(1):17. doi: 10.4103/2542-3932.226188
90. Alonzo A, Brassil J, Taylor JL, Martin D, Loo CK. Daily transcranial direct current stimulation (tDCS) leads to greater increases in cortical excitability than second daily transcranial direct current stimulation. Brain Stimulation (2012) 5(3):208–13. doi: 10.1016/j.brs.2011.04.006
91. Shekhawat GS, Sundram F, Bikson M, Truong D, De Ridder D, Stinear CM, et al. Intensity, duration, and location of high-definition transcranial direct current stimulation for tinnitus relief. Neurorehabil Neural Repair (2016) 30(4):349–59. doi: 10.1177/1545968315595286
92. Morris MJ, Beilharz JE, Maniam J, Reichelt AC, Westbrook RF. Why is obesity such a problem in the 21st century? The intersection of palatable food, cues and reward pathways, stress, and cognition. Neurosci Biobehav Rev (2015) 58:36–45. doi: 10.1016/j.neubiorev.2014.12.002
93. Frayn M, Sears CR, von Ranson KM. A sad mood increases attention to unhealthy food images in women with food addiction. Appetite (2016) 100:55–63. doi: 10.1016/j.appet.2016.02.008
94. Lefaucheur JP, Antal A, Ayache SS, Benninger DH, Brunelin J, Cogiamanian F, et al. Evidence-based guidelines on the therapeutic use of transcranial direct current stimulation (tDCS). Clin Neurophysiol (2017) 128(1):56–92. doi: 10.1016/j.clinph.2016.10.087
95. Claus ED, Klimaj SD, Chavez R, Martinez AD, Clark VP. A randomized trial of combined tDCS over right inferior frontal cortex and cognitive bias modification: Null effects on drinking and alcohol approach bias. Alcohol: Clin Exp Res (2019) 43(7):1591–9. doi: 10.1111/acer.14111
96. den Uyl TE, Gladwin TE, Wiers RW. Electrophysiological and behavioral effects of combined transcranial direct current stimulation and alcohol approach bias retraining in hazardous drinkers. Alcohol: Clin Exp Res (2016) 40(10):2124–33. doi: 10.1111/acer.13171
97. den Uyl TE, Gladwin TE, Rinck M, Lindenmeyer J, Wiers RW. A clinical trial with combined transcranial direct current stimulation and alcohol approach bias retraining. Addict Biol (2017) 22(6):1632–40. doi: 10.1111/adb.12463
98. Burgess EE, Sylvester MD, Morse KE, Amthor FR, Mrug S, Lokken KL. Effects of transcranial direct current stimulation (tDCS) on binge-eating disorder. Int J Eat Disord (2016) 49.10:930–6. doi: 10.1002/eat.22554
99. Feeser M, Prehn K, Kazzer P, Amthor FR, Mungee A, Bajbouj M, et al. Transcranial direct current stimulation enhances cognitive control during emotion regulation. Brain Stimulation (2014) 7.1:105–12. doi: 10.1016/j.brs.2013.08.006
100. Kooteh BR, Dolatshahi B, Nosratabadi M, Bakhshani NM, Mahdavi A, Hakami MC, et al. Combination Therapy and Opioids: Effectiveness of Transcranial Direct-Current Stimulation (tDCS) and Emotion Regulation Training in Reducing Current Drug Craving. Maedica A J Clin Med (2020) 15(1). doi: 10.26574/maedica.2020.15.1.53
101. Rigi Kooteh B, Bakhshani NM, Nosratabadi M, Dolatshahi B. Effectiveness of Transcranial Direct-Current Stimulation (tDCS) and Emotion Regulation Training in Reducing Current Drug Craving and Drug-Use Thoughts and Fantasies in Opioid-Dependent Patients: The Issue of Precedence. Int J High Risk Behav Addict (2019) 8 (2). doi: 10.5812/ijhrba.94499
Keywords: transcranial direct current stimulation, tDCS, craving, substance dependence, food addiction, dorsolateral prefrontal cortex
Citation: Chen J, Qin J, He Q and Zou Z (2020) A Meta-Analysis of Transcranial Direct Current Stimulation on Substance and Food Craving: What Effect Do Modulators Have? Front. Psychiatry 11:598. doi: 10.3389/fpsyt.2020.00598
Received: 16 March 2020; Accepted: 09 June 2020;
Published: 26 June 2020.
Edited by:
Yanhui Liao, Sir Run Run Shaw Hospital, ChinaCopyright © 2020 Chen, Qin, He and Zou. This is an open-access article distributed under the terms of the Creative Commons Attribution License (CC BY). The use, distribution or reproduction in other forums is permitted, provided the original author(s) and the copyright owner(s) are credited and that the original publication in this journal is cited, in accordance with accepted academic practice. No use, distribution or reproduction is permitted which does not comply with these terms.
*Correspondence: Zhiling Zou, em91emxAc3d1LmVkdS5jbg==
†These authors have contributed equally to this work and share first authorship