- 1Department of MRI, Shaanxi Provincial People’s Hospital, Xi’an, China
- 2Department of Radiology, The First Affiliated Hospital of Xi’an Jiaotong University, Xi’an, China
- 3Department of Psychiatry, The First Affiliated Hospital of Xi’an Jiaotong University, Xi’an, China
Purpose: Auditory verbal hallucinations (AVH) are among the most common and prominent symptoms of schizophrenia. Although abnormal functional connectivity associated with AVH has been reported in multiple regions, the changes in information flow remain unclear. In this study, we aimed to elucidate causal influences related to AVH in key regions of auditory, language, and memory networks, by using Granger causality analysis (GCA).
Patients and Methods: Eighteen patients with schizophrenia with AVH and eighteen matched patients without AVH who received resting-state fMRI scans were enrolled in the study. The bilateral superior temporal gyrus (STG), Broca’s area, Wernicke’s area, putamen, and hippocampus were selected as regions of interest.
Results: Granger causality (GC) increased from Broca’s area to the left STG, and decreased from the right homolog of Wernicke’s area to the right homolog of Broca’s area, and from the right STG to the right hippocampus in the AVH group compared with the non-AVH group. Correlation analysis showed that the normalized GC ratios from the left STG to Broca’s area, from the left STG to the right homolog of Broca’s area, and from the right STG to the right homolog of Broca’s area were negatively correlated with severity of AVH, and the normalized GC ratios from Broca’s area to the left hippocampus and from Broca’s area to the right STG were positively correlated with severity of AVH.
Conclusion: Our findings indicate a causal influence of pivotal regions involving the auditory, language, and memory networks in schizophrenia with AVH, which provide a deeper understanding of the neural mechanisms underlying AVH.
Introduction
Auditory verbal hallucinations (AVH) are the most prominent and burdensome symptoms of schizophrenia, and comprise sensory experiences wherein voices are heard without a causative external stimulus. The prevalence of AVH exceeds 60% in patients with schizophrenia worldwide (1, 2). In 30% of patients with AVH, the hallucinations are not affected by clinical treatments (3), and cause functional disability and therefore lower quality of life. Clinical options to treat such patients are currently limited. Since the neurobiological mechanisms involved in AVH remain unclear; insights into the pathophysiology of AVH could facilitate the development of novel strategies to treat patients who do not respond to currently used medications.
The mechanism by which AVH occurs spontaneously from the brain’s intrinsic activity is of great clinical interest. Resting-state functional magnetic resonance imaging (fMRI) can show spontaneous brain activity in vivo, and can therefore provide invaluable insights into the psychopathology of AVH. Based on time series derived from resting state fMRI data, functional connectivity (FC) analysis can be used to calculate the temporal correlations between a blood-oxygenation-level dependent in any two regions, offering an effective method to study alterations in brain connectivity within multiple brain networks associated with AVH in schizophrenia.
In previous models including the “memory intrusion”, “self-monitoring”, “two hit bottom-up and top-down”, and hybrid models, language, auditory, and memory brain networks were closely associated with AVH in patients with schizophrenia (4). The superior temporal gyrus (STG) (auditory cortex), Broca’s area (speech production), Wernicke’s area (language comprehension), hippocampus (memory retrieval), and putamen (initiating language representations) are the key areas responsible for AVH in schizophrenia, and showed functional alterations in previous brain imaging studies (5–18). Although aberrant FC associated with AVH has been reported in these regions in many resting-state fMRI studies (6, 7, 15, 16, 19–25), the interactions between these regions have not been described completely. Furthermore, the information flow within these networks has not been completely characterized.
Effective connectivity (EC) analyses using data on time-lagged relationships between cerebral regions, offers additional information on the directionality of information flow within brain networks (26, 27). Granger causality analysis (GCA) is a special method used to study EC, and could yield deductive networks based on hypothetical seed regions without prior knowledge. This model is simple, has low computational complexity, and is not limited by the number of brain regions studied. It is therefore an efficient and convenient method for analyzing fMRI data on resting-state functional organization in various healthy and diseased brain networks (28–30). To our knowledge, this method has not been used to study the information flow within the aforementioned regions in resting-state fMRI studies.
In this study, we used the GCA method on resting-state fMRI data to determine the patterns of effective connections between the bilateral STG, Broca’s area, Wernicke’s area, hippocampus, and putamen in schizophrenia patients with and without AVH, in order to obtain novel imaging evidence for the elucidation of the neural mechanisms underlying AVH. We first used GCA to study the differences in effective connections between schizophrenia patients with and without AVH to determine the direction of connection changes. We then performed correlation analysis between changes in causal influence and severity of hallucination symptoms to identify the functional disturbances caused by AVH.
Materials and Methods
Subjects
This study was conducted in accordance with the Declaration of Helsinki. The experimental protocol was approved by the Ethics Committee of Shaanxi Provincial People’s Hospital, and written parental consent was obtained from all participants.
Study participants were recruited from the in-patient ward, and met the following criteria: 1) diagnosed with schizophrenia/schizoaffective disorder by using the Structured Clinical Interview of the DSM-IV (SCID); 2) with the Positive and Negative Syndrome Scale (PANSS) assessment; 3) Han Chinese in origin; 4) right-handed; 5) between 18 and 40 years old; 6) received conventional MRI and resting-state fMRI scans with available images. Exclusion criteria were as follows: 1) history of other psychotic disorders; 2) substance abuse or dependence; 3) severe medical disorders; 4) traumatic brain injury; 5) electroconvulsive therapy within the past 6 months; 6) intellectual disability or neurological impairment. Patients who presented with AVHs at least once a day for the past four weeks, with a PANSS P3 score ≥4 were assigned to the AVH group, and patients who did not experience hallucinations or had a P3 score of ≤3 belonged to the non-AVH group (31, 32).
All clinical data were double reviewed by a senior researcher, who verified the credibility of the patient statements. Information of usage of antipsychotics (name, dosage, and duration of drugs used before MRI scanning) was also collected, and the converted antipsychotic doses to chlorpromazine (CPZ) milligram equivalent units were calculated. No significant correlation was found between granger causality (GC) and illness duration or CPZ equivalent in either AVH group or non-AVH group; thus, they were not taken as covariates in further statistics.
MRI Acquisition
All MRI data were performed within 3 days after the PANSS. Conventional MRI and resting-state fMRI were acquired on a 3.0T scanner (Signa HDxt, General Electric Medical System, Milwaukee, WI, USA) with an eight-channel phase array radio-frequency head coil. Head motion was minimized by positioned with restraining foam pads. Earplugs were used to decrease the effects of scanner noise. The total scan time was <20 min. Three-dimensional fast-spoiled gradient-recalled echo T1-weighted images (repetition time/echo time, 10/4.6–14 ms) and fast-spin echo T2-weighted images (repetition time/echo time, 4,200/116.4–124.0 ms) were obtained. Before fMRI scanning, the patients were instructed to stay awake, relax, keep their eyes closed, and refrain from moving. Blood oxygen level-dependent (BOLD) fMRI was acquired using the following parameters: 185 volumes, echo time = 30 ms, repetition time = 2000 ms, flip angle = 90°, 40 axial slices, slice thickness = 4 mm; field of view, 240 × 240 mm2; matrix = 64×64. The acquisition time was 6 min and 30 s.
Data Preprocessing
Preprocessing of fMRI data was performed using SPM12 software (http://www.fil.ion.ucl.ac.uk/spm). Functional images were subjected to slice-timing correction and were then realigned to the first volume to correct for head motions. The data with head movement > 1.5 mm and/or rotation angle >1.5° were excluded. The realigned images were then spatially normalized to the MNI EPI template and resampled to a voxel size of 3 × 3 × 3 mm3. A band-pass filter between 0.01 and 0.08 Hz was subsequently applied to the data to remove the effects of very-low-frequency drift and high-frequency noise. Finally, spatial smoothing was applied using a 6-mm full-width-at-half-maximum Gaussian kernel. The head-motion parameters, white-matter signals, and cerebrospinal-fluid-signals were regressed out of the BOLD signals.
Regions of Interest (ROIs) Selection
The bilateral STG, Broca’s area, Wernicke’s area, putamen, and hippocampus were selected as the ROIs. Masks representing each of the ROIs were created by using the Wake Forest University PickAtlas tool with TD-ICBM Human Atlas (TD Brodmann) (33). The fMRI time courses of all the voxels located within the masks for each ROI were extracted.
Granger Causality Analysis
GCA was performed using REST-GCA software (http://www.restfmri.net). By applying an order 2 vector auto-regression model, for any two ROI time series x(t) and y(t), the time domain pairwise GCA components from x(t) to y(t) (Fx→y) and from y(t) to x(t) (Fy→x) were calculated respectively. Pairwise Granger causal connectivity indicates that neuronal activity of one region is predictive of activity occurring in another region. Causal influence was normalized using the following computing method (34):
Rx→y is the ratio describing the relative strength and directionality of the causal influences between x and y. A positive Rx→y with a larger absolute value indicates stronger causal influence from x to y, while a negative Rx→y with a larger absolute value denotes stronger causal influence from y to x. Changes in Rx→y were calculated by analyzing the differences between the AVH and non-AVH groups.
Correlation Analysis
The association between changes in causal influence and hallucination were assessed using correlation analyses performed between the normalized Rx→y ratios of the pairwise ROIs and the PANSS P3 score. The Bonferroni correction was applied to correct for multiple comparisons, and P < 0.005 (0.05/10) was considered statistically significant.
Statistical Analysis
Statistical analysis was performed on SPSS 17.0 (SPSS, Chicago, IL, USA). The measurement data of normal distribution were presented as means ± standard deviations, and categorical data as frequencies and percentages. The two sample t-test or χ2 test, were used to compare demographic and clinical data between the AVH and non-AVH groups. All statistical tests were two-tailed, and P ≤ 0.05 was considered significant.
Results
Subjects
This study included 18 patients with AVH and 18 without AVH. The AVH and non-AVH groups did not show significant differences in age, gender, education, smoking, drinking, illness duration, proportion of first episode patients, CPZ equivalent dose, total, negative and general symptom severity PANSS score. Positive PANSS score and PANSS P3 score were significantly higher in the AVH group than in the non-AVH group (Table 1).
GCA Results
Compared with the non-AVH group, AVH group showed an increase in GC from the right homolog of Broca’s area to the left STG, and a decrease from the right STG to the right hippocampus, from the left putamen to the right hippocampus, from the right putamen to the right hippocampus, and from the right putamen to Broca’s area (Tables 2 and 3).
The normalized ratios of GC from the left STG to Broca’s area and its right homolog, from the right STG to the right homolog of Broca’s area, and from the right STG to the right hippocampus were significantly lower in the AVH group than in the non-AVH group. The bi-directional causal values (Fx→y and Fy→x) explained the results caused by increased GC from Broca’s area and its right homolog to the left STG and from the right homolog of Broca’s area to the right STG, and the decreased GC from the right STG to the right hippocampus (Table 4 and Figure 1).
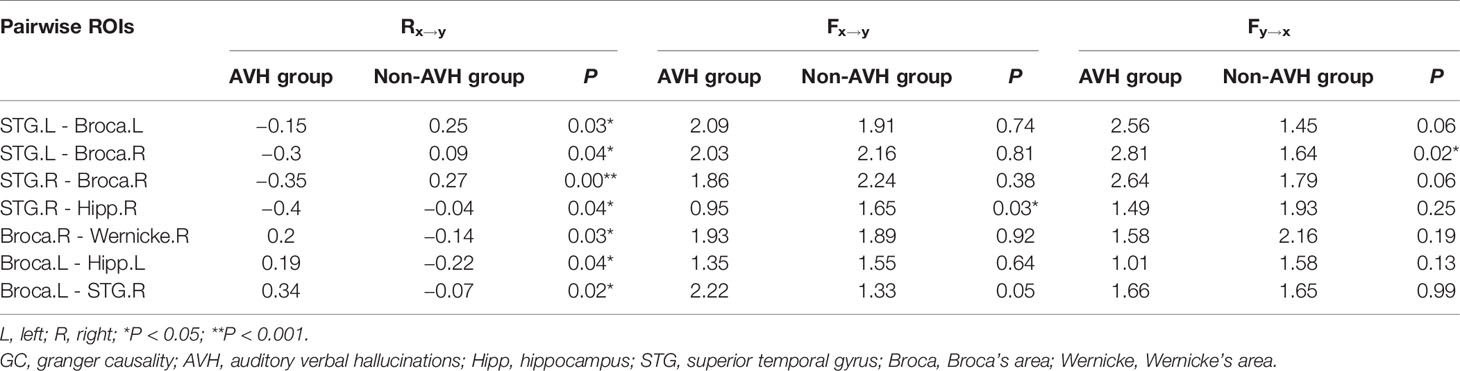
Table 4 Comparisons of the pairwise normalized ratios of GC values (Rx→y) between AVH and non-AVH groups.
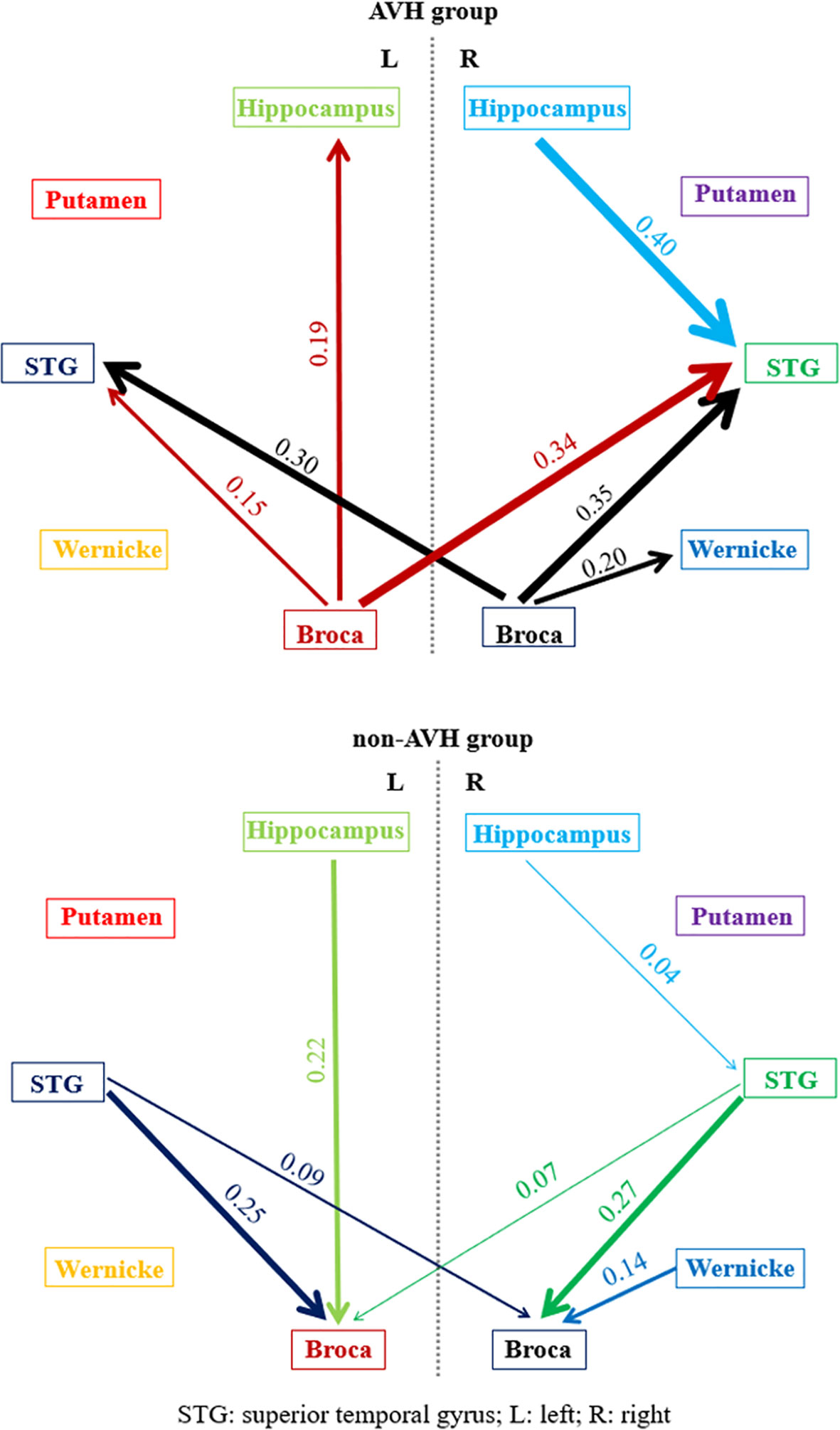
Figure 1 The pairwise normalized ratios of GC values (Rx→y) in AVH and non-AVH groups. The thickness of lines with marked numbers indicated the strength of granger causality. The different color of lines corresponded to different brain regions.
The normalized ratios of Granger causality from the right homolog of Broca’s area to the right homolog of Wernicke’s area, from Broca’s area to the left hippocampus, and from Broca’s area to the STG were significantly increased in the AVH group (Table 4 and Figure 1). Bi-directional causal values (Fx→y and Fy→x), indicated that these differences were mainly due to the decreased Granger causality from the right homolog of Wernicke’s area to the right homolog of Broca’s area and from the left hippocampus to Broca’s area, and increased Granger causality from Broca’s area to the STG (Table 4 and Figure 1).
Correlation Analysis
The normalized ratios of Granger causality from the left STG to Broca’s area, from the left STG to the right homolog of Broca’s area, and from the right STG to the right homolog of Broca’s area were negatively correlated with PANSS P3 score, and the normalized ratios of Granger causality from Broca’s area to the left hippocampus and from Broca’s area to the right STG were positively correlated with PANSS P3 score (Table 5). However, after the Bonferroni correction, statistically significant correlations were only observed between normalized ratios of Granger causality from the right STG to the right homolog of Broca’s area and from Broca’s area to the right STG and PANSS P3 score.
Discussion
GCA, an effective connection analysis method, is used to examine the directional interaction and influence between brain regions by calculating the information between two time series. In recent years, GCA has been widely applied in the field of neurocognitive science. Studying alterations in interactive causal influences (driving forces) in brain regions involved in schizophrenia-related hallucinations could improve our understanding of the underlying neurobiological substrates. In this study, we found significant differences in effective connections related to auditory, speech, and memory circuits, involving the STG, Wernicke’s area, Broca’s area, and hippocampus, in schizophrenia patients with and without AVH by using GCA. These abnormal functional connections could contribute causally to the onset of auditory hallucinations.
The Auditory Processing Circuit
The STG contains the auditory cortex, which is responsible for the perception and processing of sounds. Broca’s area is closely associated with the auditory cortex. Previous resting state fMRI studies suggested that abnormal connectivity between Broca’s area and the auditory cortex could be responsible for AVH (8, 24, 35). However, evidence of resting connectivity between these two areas is unclear. Sommer et al. (8) reported reduced synchronization between the left STG and inferior frontal gyrus (IFG, close to Broca’s area) in patients with chronic AVH (8). Hoffman et al. (35) studied the time course of AVH and FC at the various stages of hallucinations (35), and found an increased coupling just prior to hallucinations between the left IFG and right temporal areas (including the STG and middle temporal gyrus). Diederen et al. (24) also observed elevated connectivity between the left STG and right IFG (24). Thus, the change in connection strength in the two regions is not clear, and possibly not uniform in all patients with schizophrenia. Furthermore, the directionality of abnormal connections related to auditory and language processing regions remains unclear.
Our results from the GCA provide information on the direction of the effective connection between the STG and Broca’s area. We observed increased Granger causality from Broca’s area and Broca’s homolog to the bilateral STG in the AVH group, which was also associated with severity of hallucination. To date, only Baojuan et al. (36) have studied the interaction between the auditory cortex and Broca’s area in a dynamic causal model. They reported that a positive correlation was observed between the strength of EC from Broca’s area to the auditory cortex and AVH severity, which is consistent with our results. The increased effective connection from the auditory cortex to Broca’s area was likely a compensatory change due to the decreased connection from Wernicke’s area to Broca’s area (7), which was also verified in our study. In addition, we hypothesize that AVHs are derived from auditory cortical activity, and spontaneous activity is very likely caused by the intrusion of internal auditory signals from Broca’s area.
The Speech Processing Circuit
Our results showed decreased Granger causality from the right homolog of Wernicke’s area to the right homolog of Broca’s area. Broca’s area is involved in the production of language, and Wernicke’s area is involved in the comprehension of written and spoken language. Although the left hemisphere is widely considered the site of speech processing, studies in patients who suffered strokes indicate that the right hemisphere is also capable of basic language production (37, 38). Further studies indicated that the right homologs of Broca’s and Wernicke’s areas have prominent roles in the processing of emotional information and spoken language tone (39, 40). Our results also clearly indicated functional disturbance in speech processing circuits in patients with AVHs.
Functional alterations in Broca’s and Wernicke’s areas in patients with schizophrenia who experience AVHs have been widely reported in fMRI studies (4). However, there have been few reports of abnormal resting FC between these areas, and most results have been inconsistent. Vercammen et al. reported reduced FC between the left temporal-parietal junction and the right homolog of Broca’s area in schizophrenia patients with AVH. However, patients without AVH were not studied, and symptom correlations for hallucination severity were not presented (23). Hoffman et al. found greater FC between the bilateral Wernicke’s area and Brodmann area 45/46 of IFG (Broca’s area) in patients with AVH compared to patients without AVH (41); follow-up analysis indicated greater FC along a loop linking Wernicke’s area, the IFG, and the putamen compared with patients without AVH and healthy controls. In addition, structural connectivity in the left arcuate fasciculus, which connects Broca’s and Wernicke’s areas, was lower in patients with schizophrenia with AVH than in patients with non-AVH schizophrenia and healthy controls (42–45). These results also suggested disrupted functional interactions within speech-processing systems.
AVH have been linked to several cognitive mechanisms, including misattribution or impaired self-monitoring during speech generation, which could be due to disrupted connectivity between frontal and temporo-parietal brain regions (46). Misattribution models of AVH posit that auditory hallucination experiences occur because of failure to monitor internal speech and attributing it to an external source (47, 48), and therefore predict abnormal FC between typical speech processing areas, mainly in the left fronto-temporal network (including Broca’s and Wernicke’s areas), possibly extending to right hemisphere language homolog areas (49). The delayed “corollary discharge” theory (50, 51) proposes that the failure of neural signal transmission between temporal speech perception areas and inferior frontal speech production areas cause precognition disability in inner speech generation, and temporal speech perception areas cannot suppress response intensity in auditory perception areas, which subsequently incorrectly identify inner speech as external speech. However, the directional information of the connection between Broca’s and Wernicke’s areas is not described clearly.
Ćurčić-Blake et al. (7) primarily investigated EC in the language circuitry by using dynamic causal modeling in schizophrenia patients with and without AVH in an inner speech task. Their results showed diminished connectivity from Wernicke’s area to Broca’s area and a decreasing trend in connectivity from homologs of Broca’s and Wernicke’s areas to Broca’s area. Our results were obtained in a resting state by using GCA, but also suggested reduced information transmission from temporal to frontal language areas in schizophrenia AVH patients. Thus, neuronal activity of frontal language areas was less restrained by temporal language areas, leading to diminished self-monitoring and subsequent misperception of internal speech. These findings add to the aforementioned theoretical models from the perspective of information flow. However, similar research methods and results have not been reported and the connection changes in language processing mode underlying AVH have not been fully characterized. Further studies to verify these preliminary findings should be conducted.
The Memory Circuit
Increased GC from the left hippocampus to left Broca’s area and decreased GC from the right STG to the right hippocampus were observed in this study. The hippocampus is associated with complex memories in humans, and may be a temporary storage site for memories (52). Hippocampal damage was associated with the occurrence of auditory hallucinations in animal models of schizophrenia (53). Several neuroimaging studies have suggested that the hippocampus is involved in auditory hallucinations. Amad et al. (54) showed that functional connections, white matter connections, and hippocampal volume changes were associated with auditory hallucinations. A meta-analysis based on “activation study” demonstrated increased activity in the left hippocampus/parahippocampal region during AVH in patients with schizophrenia (10). This region also connects widely distributed association cortices, including the language areas responsible for hallucinatory experiences. The hippocampus/parahippocampal region could therefore trigger auditory hallucination-related language brain areas, as our findings also suggest.
Based on a resting-state study, some researchers (8) found reduced FC between the left STG and left hippocampus in patients with chronic schizophrenia with auditory hallucinations compared to patients with non-auditory hallucination, which was negatively correlated with the severity of AVH. Baojuan et al. (36) reported a weakened effective connection from the auditory cortex to the hippocampus in patients with schizophrenia with AVH, by using the dynamic causal model, which was consistent with the results of this study. Although no projection fibers have been found between the auditory cortex and hippocampus anatomically, the results of this study and previous studies all suggest that there might be functional interactions between the two regions. However, there is still no clear understanding of how the reduction of the auditory cortical-hippocampal connection is related with the occurrence of auditory hallucinations. Baojuan et al. (36) speculated that since there are long longitudinal fibers (inferior longitudinal fasciculus) linking the visual region and the hippocampus (55), there might be a similar “auditory cortical-hippocampal projection” participating in the processing of auditory information. We hypothesized that reduced auditory cortical-hippocampal connectivity may lead to aberrant memory retrieval, which is regulated by the hippocampus/parahippocampal gyrus, subsequently triggering memory pieces stored in subcortical regions, especially those related to language, thereby causing the appearance of unconscious auditory hallucinations.
Limitations
There were some limitations associated with this study. First, the sample size was small, and the study only included patients from one hospital, which might limit the scope and statistical power of our findings. Second, ROI selection was mainly based on previous literature researches, which were frequently reported regions associated with auditory hallucinations. However, several other regions associated with auditory hallucination, but with no clear consensus, were not included, which might limit the exploration of alterations in effective connections. Third, GCA ignores the influence of neurohemodynamics, which may cause displacement distortion and therefore false causality. The dynamic causal model can quantify changes of the effective connection at the neuron level, which can make up for the defects of the Granger causality model but is directly affected by the accuracy of selected ROIs. Therefore, combining data-driven and model-driven analysis by using these two effective connection methods could provide more accurate results and is a promising avenue for future research.
Conclusion
In this effective connection study based on GCA, we found abnormal connections in specific directions involving the auditory cortex, auditory language formation regions, and hippocampus in schizophrenia with auditory hallucinations, which may explain the mechanism of auditory hallucination from insights of auditory processing, origin of internal speech and memory. These results also provide new imaging evidences in related neural mechanisms of auditory hallucinations from the direction of the information flow.
Data Availability Statement
The raw data supporting the conclusions of this article will be made available by the authors, without undue reservation.
Ethics Statement
The studies involving human participants were reviewed and approved by the Ethics Committee of Shaanxi Provincial People’s Hospital. The patients/participants provided their written informed consent to participate in this study.
Author Contributions
XiaZ and JY were responsible for the study concept and design. JG, DZ, and LW carried out the literature search and analyzed the data. JG wrote the first draft of the manuscript. YF, YW, and XinZ carried out the image acquisition. WW examined patients with psychopathological scales. JG, DZ, MT, and XL assisted with data analysis and interpretation of findings. All authors contributed to the article and approved the submitted version.
Funding
This work was supported by National Natural Science Foundation of China (No. 81801327).
Conflict of Interest
The authors declare that the research was conducted in the absence of any commercial or financial relationships that could be construed as a potential conflict of interest.
Acknowledgments
We thank all participants and their parents for their loyalty and cooperation.
References
1. Alderson-Day B, McCarthy-Jones S, Fernyhough C. Hearing voices in the resting brain: A review of intrinsic functional connectivity research on auditory verbal hallucinations. Neurosci Biobehav Rev (2015) 55:78–87. doi: 10.1016/j.neubiorev.2015.04.016
2. Llorca PM, Pereira B, Jardri R, Chereau-Boudet I, Brousse G, Misdrahi D, et al. Hallucinations in schizophrenia and Parkinson’s disease: an analysis of sensory modalities involved and the repercussion on patients. Sci Rep (2016) 6:38152. doi: 10.1038/srep38152
3. McCarthy-Jones S, Trauer T, Mackinnon A, Sims E, Thomas N, Copolov DL. A new phenomenological survey of auditory hallucinations: evidence for subtypes and implications for theory and practice. Schizophr Bull (2014) 40(1):231–5. doi: 10.1093/schbul/sbs156
4. Ćurčić-Blake B, Ford JM, Hubl D, Orlov ND, Sommer IE, Waters F, et al. Interaction of language, auditory and memory brain networks in auditory verbal hallucinations. Prog Neurobiol (2017) 148:1–20. doi: 10.1016/j.pneurobio.2016.11.002
5. Kuhn S, Gallinat J. Quantitative meta-analysis on state and trait aspects of auditory verbal hallucinations in schizophrenia. Schizophr Bull (2012) 38(4):779–86. doi: 10.1093/schbul/sbq152
6. Cui LB, Liu K, Li C, Wang LX, Guo F, Tian P, et al. Putamen-related regional and network functional deficits in first-episode schizophrenia with auditory verbal hallucinations. Schizophr Res (2016) 173(1-2):13–22. doi: 10.1016/j.schres.2016.02.039
7. Ćurčić-Blake B, Liemburg E, Vercammen A, Swart M, Knegtering H, Bruggeman R, et al. When Broca goes uninformed: Reduced information flow to Broca’s area in schizophrenia patients with auditory hallucinations. Schizophr Bull (2013) 39(5):1087–95. doi: 10.1093/schbul/sbs107
8. Sommer IE, Clos M, Meijering AL, Diederen KM, Eickhoff SB. Resting state functional connectivity in patients with chronic hallucinations. PloS One (2012) 7(9):e43516. doi: 10.1371/journal.pone.0043516
9. Hare SM, Law AS, Ford JM, Mathalon DH, Ahmadi A, Damaraju E, et al. Disrupted network cross talk, hippocampal dysfunction and hallucinations in schizophrenia. Schizophr Res (2018) 199:226–34. doi: 10.1016/j.schres.2018.03.004
10. Jardri R, Pouchet A, Pins D, Thomas P. Cortical activations during auditory verbal hallucinations in schizophrenia: a coordinate-based meta-analysis. Am J Psychiatry (2011) 168(1):73–81. doi: 10.1176/appi.ajp.2010.09101522
11. Lavigne KM, Woodward TS. Hallucination- and speech-specific hypercoupling in frontotemporal auditory and language networks in schizophrenia using combined task-based fMRI data: An fBIRN study. Hum Brain Mapp (2018) 39(4):1582–95. doi: 10.1002/hbm.23934
12. Cui LB, Liu L, Guo F, Chen YC, Chen G, Xi M, et al. Disturbed Brain Activity in Resting-State Networks of Patients with First-Episode Schizophrenia with Auditory Verbal Hallucinations: A Cross-sectional Functional MR Imaging Study. Radiology (2017) 283(3):810–9. doi: 10.1148/radiol.2016160938
13. Liu L, Cui LB, Xi YB, Wang XR, Liu YC, Xu ZL, et al. Association Between Connectivity of Hippocampal Sub-Regions and Auditory Verbal Hallucinations in Schizophrenia. Front Neurosci (2019) 13:424. doi: 10.3389/fnins.2019.00424
14. Gong J, Wang J, Luo X, Chen G, Huang H, Huang R, et al. Abnormalities of intrinsic regional brain activity in first-episode and chronic schizophrenia: a meta-analysis of resting-state functional MRI. J Psychiatry Neurosci (2020) 45(1):55–68. doi: 10.1503/jpn.180245
15. Scheinost D, Tokoglu F, Hampson M, Hoffman R, Constable RT. Data-Driven Analysis of Functional Connectivity Reveals a Potential Auditory Verbal Hallucination Network. Schizophr Bull (2019) 45(2):415–24. doi: 10.1093/schbul/sby039
16. Zhang W, Li S, Wang X, Gong Y, Yao L, Xiao Y, et al. Abnormal dynamic functional connectivity between speech and auditory areas in schizophrenia patients with auditory hallucinations. NeuroImage Clin (2018) 19:918–24. doi: 10.1016/j.nicl.2018.06.018
17. Orlov ND, Giampietro V, O’Daly O, Lam SL, Barker GJ. Real-time fMRI neurofeedback to down-regulate superior temporal gyrus activity in patients with schizophrenia and auditory hallucinations: a proof-of-concept study. Transl Psychiatry (2018) 8: (1):46. doi: 10.1038/s41398-017-0067-5
18. Alonso-Solís A, Vives-Gilabert Y, Portella MJ, Rabella M, Grasa EM, Roldán A, et al. Altered amplitude of low frequency fluctuations in schizophrenia patients with persistent auditory verbal hallucinations. Schizophr Res (2017) 189:97–103. doi: 10.1016/j.schres.2017.01.042
19. Raij TT, Valkonen-Korhonen M, Holi M, Therman S, Lehtonen J, Hari R. Reality of auditory verbal hallucinations. Brain (2009) 132(Pt 11):2994–3001. doi: 10.1093/brain/awp186
20. Price CJ. The anatomy of language: a review of 100 fMRI studies published in 2009. Ann N Y Acad Sci (2010) 1191(1):62–88. doi: 10.1111/j.1749-6632.2010.05444.x
21. Zhang L, Li B, Wang H, Li L, Liao Q, Liu Y, et al. Decreased middle temporal gyrus connectivity in the language network in schizophrenia patients with auditory verbal hallucinations. Neurosci Lett (2017) 653:177–82. doi: 10.1016/j.neulet.2017.05.042
22. Chang X, Collin G, Xi Y, Cui L, Scholtens LH, Sommer IE, et al. Resting-state functional connectivity in medication-naïve schizophrenia patients with and without auditory verbal hallucinations: A preliminary report. Schizophr Res (2017) 188:75–81. doi: 10.1016/j.schres.2017.01.024
23. Vercammen A, Knegtering H, den Boer JA, Liemburg EJ, Aleman A. Auditory hallucinations in schizophrenia are associated with reduced functional connectivity of the temporo-parietal area. Biol Psychiatry (2010) 67(10):912–8. doi: 10.1016/j.biopsych.2009.11.017
24. Diederen KM, Neggers SF, de Weijer AD, van Lutterveld R, Daalman K, Eickhoff SB, et al. Aberrant resting-state connectivity in non-psychotic individuals with auditory hallucinations. Psychol Med (2013) 43(8):1685–96. doi: 10.1017/S0033291712002541
25. Shinn AK, Baker JT, Cohen BM, Ongür D. Functional connectivity of left Heschl’s gyrus in vulnerability to auditory hallucinations in schizophrenia. Schizophr Res (2013) 143(2-3):260–8. doi: 10.1016/j.schres.2012.11.037
26. Friston KJ. Functional and effective connectivity in neuroimaging: A synthesis. Hum Brain Mapp (1994) 2(1-2):56–78. doi: 10.1002/hbm.460020107
27. Stephan KE, Friston KJ. Analyzing effective connectivity with functional magnetic resonance imaging. Wiley Interdiscip Rev Cognit Sci (2010) 1(3):446–59. doi: 10.1002/wcs.58
28. Zhang Y, Wang J, Zhang G, Zhu Q, Cai W, Tian J, et al. The neurobiological drive for overeating implicated in Prader-Willi syndrome. Brain Res (2015) 1620(1):72–80. doi: 10.1016/j.brainres.2015.05.008
29. Zhang Y, Li Q, Wen X, Cai W, Li G, Tian J, et al. Granger causality reveals a dominant role of memory circuit in chronic opioid dependence. Addict Biol (2017) 22(4):1068–80. doi: 10.1111/adb.12390
30. Wen X, Liu Y, Yao L, Ding M. Top-down regulation of default mode activity in spatial visual attention. J Neurosci (2013) 33(15):6444–53. doi: 10.1523/JNEUROSCI.4939-12.2013
31. Benetti S, Pettersson-Yeo W, Allen P, Catani M, Williams S, Barsaglini A, et al. Auditory verbal hallucinations and brain dysconnectivity in the perisylvian language network: a multimodal investigation. Schizophr Bull (2015) 41(1):192–200. doi: 10.1093/schbul/sbt172
32. Cui Y, Liu B, Song M, Lipnicki DM, Li J, Xie S, et al. Auditory verbal hallucinations are related to cortical thinning in the left middle temporal gyrus of patients with schizophrenia. Psychol Med (2018) 48(1):115–22. doi: 10.1017/S0033291717001520
33. Maldjian JA, Laurienti PJ, Kraft RA, Burdette JH. An automated method for neuroanatomic and cytoarchitectonic atlas-based interrogation of fMRI data sets. Neuroimage (2003) 19(3):1233–9. doi: 10.1016/s1053-8119(03)00169-1
34. Sridharan D, Levitin DJ, Menon V. A critical role for the right fronto-insular cortex in switching between central-executive and default-mode networks. Proc Natl Acad Sci U S A (2008) 105(34):12569–74. doi: 10.1073/pnas.0800005105
35. Hoffman RE, Pittman B, Constable RT, Bhagwagar Z, Hampson M. Time course of regional brain activity accompanying auditory verbalhallucinations in schizophrenia. Br J Psychiatry (2011) 198(4):277–83. doi: 10.1192/bjp.bp.110.086835
36. Baojuan L, Longbiao C, Yibin X, Friston KJ, Guo F, Wang HN, et al. Abnormal effective connectivity in the brain is involved in auditory verbal hallucinations in schizophrenia. Neurosci Bull (2017) 33(3):1–11. doi: 10.1007/s12264-017-0101-x
37. Thiel A, Schumacher B, Wienhard K, Gairing S, Kracht LW, Wagner R, et al. Direct demonstration of transcallosal disinhibition in language networks. J Cereb Blood Flow Metab (2006) 26(9):1122–7. doi: 10.1038/sj.jcbfm.9600350
38. Voets NL, Adcock JE, Flitney DE, Behrens TE, Hart Y, Stacey R, et al. Distinct right frontal lobe activation in language processing following left hemisphere injury. Brain (2006) 129(Pt 3):754–66. doi: 10.1093/brain/awh679
39. Meyer M, Alter K, Friederici AD, Lohmann G, von Cramon DY. FMRI reveals brain regions mediating slow prosodic modulations in spoken sentences. Hum Brain Mapp (2002) 17(2):73–88. doi: 10.1002/hbm.10042
40. Mitchell RL, Elliott R, Barry M, Cruttenden A, Woodruff PW. The neural response to emotional prosody, as revealed by functional magnetic resonance imaging. Neuropsychologia (2003) 41(10):1410–21. doi: 10.1016/s0028-3932(03)00017-4
41. Hoffman RE, Fernandez T, Pittman B, Hampson M. Elevated functional connectivity along a corticostriatal loop and the mechanism of auditory/verbal hallucinations in patients with schizophrenia. Biol Psychiatry (2011) 69(5):407–14. doi: 10.1016/j.biopsych.2010.09.050
42. Ćurčić-Blake B, Nanetti L, Van de Meer L, Cerliani L, Renken R, Pijnenborg GH, et al. Not on speaking terms: Hallucinations and structural network disconnectivity in schizophrenia. Brain Struct Funct (2015) 220(1):407–18. doi: 10.1007/s00429-013-0663-y
43. Psomiades M, Fonteneau C, Mondino M, Luck D, Haesebaert F, Suaud-Chagny MF, et al. Integrity of the arcuate fasciculus in patients with schizophrenia with auditory verbal hallucinations: A DTI-tractography study. NeuroImage Clin (2016) 12(C):970–5. doi: 10.1016/j.nicl.2016.04.013
44. McCarthy-Jones S, Oestreich LK, Whitford TJ. Reduced integrity of the left arcuate fasciculus is specifically associated with auditory verbal hallucinations in schizophrenia. Schizophr Res (2015) 162(1-3):1–6. doi: 10.1016/j.schres.2014.12.041
45. Geoffroy PA, Houenou J, Duhamel A, Amad A, De Weijer AD, Curcic-Blake B, et al. The Arcuate Fasciculus in auditory-verbal hallucinations: a meta-analysis of diffusion-tensor-imaging studies. Schizophr Res (2014) 159(1):234–7. doi: 10.1016/j.schres.2014.07.014
46. Allen P, Modinos G, Hubl D, Shields G, Cachia A, Jardri R, et al. Neuroimaging auditory hallucinations in schizophrenia: from neuroanatomy to neurochemistry and beyond. Schizophr Bull (2012) 38(4):695–703. doi: 10.1093/schbul/sbs066
47. Woodward TS, Menon M. Misattribution models (II): Source monitoring in hallucinating schizophrenia subjects. New York;: Springer (2013).
48. Price CJ. A review and synthesis of the first 20 years of PET and fMRI studies of heard speech, spoken language and reading. Neuroimage (2012) 62(2):816–47. doi: 10.1016/j.neuroimage.2012.04.062
49. Sommer IE, Diederen KM, Blom JD, Willems A, Kushan L, Slotema K, et al. Auditory verbal hallucinations predominantly activate the right inferior frontal area. Brain (2008) 131(Pt 12):3169–77. doi: 10.1093/brain/awn251
50. Whitford TJ, Mathalon DH, Shenton ME, Roach BJ, Bammer R, Adcock RA, et al. Electrophysiological and diffusion tensor imaging evidence of delayed corollary discharges in patients with schizophrenia. Psychol Med (2011) 41(5):959–69. doi: 10.1017/S0033291710001376
51. Bose A, Nawani H, Agarwal SM, Shivakumar V, Kalmady SV, Shenoy S, et al. Effect of fronto-temporal transcranial direct current stimulation on corollary discharge in schizophrenia: A randomized, double-blind, sham-controlled mediation analysis study. Schizophr Res (2019) 204:411–2. doi: 10.1016/j.schres.2018.07.040
52. Chai XJ, Ofen N, Jacobs LF, Gabrieli JD. Scene complexity: influence on perception, memory, and development in the medial temporal lobe. Front Hum Neurosci (2010) 4(1):21. doi: 10.3389/fnhum.2010.00021
53. Diederen KM, Neggers SF, Daalman K, Blom JD, Goekoop R, Kahn RS, et al. Deactivation of the parahippocampal gyrus preceding auditory hallucinations in schizophrenia. Am J Psychiatry (2010) 167(4):427–35. doi: 10.1176/appi.ajp.2009.09040456
54. Amad A, Cachia A, Gorwood P, Pins D, Delmaire C, Rolland B, et al. The multimodal connectivity of the hippocampal complex in auditory and visual hallucinations. Mol Psychiatry (2014) 19(2):184–91. doi: 10.1038/mp.2012.181
Keywords: schizophrenia, auditory verbal hallucination, magnetic resonance imaging, resting state, effective connectivity
Citation: Gao J, Zhang D, Wang L, Wang W, Fan Y, Tang M, Zhang X, Lei X, Wang Y, Yang J and Zhang X (2020) Altered Effective Connectivity in Schizophrenic Patients With Auditory Verbal Hallucinations: A Resting-State fMRI Study With Granger Causality Analysis. Front. Psychiatry 11:575. doi: 10.3389/fpsyt.2020.00575
Received: 31 March 2020; Accepted: 05 June 2020;
Published: 24 June 2020.
Edited by:
Shaohua Hu, Zhejiang University, ChinaReviewed by:
Lin Liu, Peking University Health Science Centre, ChinaNing Hua Wang, Xijing Hospital, Fourth Military Medical University, China
Manli Huang, Zhejiang University, China
Copyright © 2020 Gao, Zhang, Wang, Wang, Fan, Tang, Zhang, Lei, Wang, Yang and Zhang. This is an open-access article distributed under the terms of the Creative Commons Attribution License (CC BY). The use, distribution or reproduction in other forums is permitted, provided the original author(s) and the copyright owner(s) are credited and that the original publication in this journal is cited, in accordance with accepted academic practice. No use, distribution or reproduction is permitted which does not comply with these terms.
*Correspondence: Xiaoling Zhang, enhsLjgyMkAxNjMuY29t; Jian Yang, eWoxMTE4QG1haWwueGp0dS5lZHUuY24=
†These authors have contributed equally to this work