- 1Department of Psychiatry, Psychotherapy and Psychosomatics, Faculty of Medicine, RWTH Aachen University, Aachen, Germany
- 2JARA - Translational Brain Medicine, Jülich-Aachen Research Alliance, Jülich, Germany
- 3Department of High-field Magnetic Resonance, Max-Planck-Institute for Biological Cybernetics, Tübingen, Germany
- 4Institute of Neuroscience and Medicine: JARA-Institute Brain Structure Function Relationship (INM 10), Research Center Jülich, Jülich, Germany
- 5Carver College of Medicine, Iowa Neuroscience Institute, University of Iowa, Iowa City, IA, United States
- 6Department of Psychiatry, Carver College of Medicine, University of Iowa, Iowa City, IA, United States
Brain derived neurotrophic factor (BDNF) has been implicated in the pathophysiology of schizophrenia. As BDNF regulates axonal and dendritic growth, altered BDNF levels in schizophrenia patients might underlie changes in structural connectivity that have been identified by magnetic resonance imaging (MRI). We investigated a possible correlation between BDNF serum levels, fiber tract architecture, and regional grey matter volumes in 19 schizophrenia patients and a gender- and age-matched control group. Two patients had to be excluded due to abnormalities in their MRI scans. Serum samples were obtained to determine BDNF levels, and T1- as well as diffusion-weighted sequences were acquired. We, then, investigated correlations between BDNF serum levels with neuroimaging parameters, using Voxel-based Morphometry (VBM) and Tract-based Spatial Statistics (TBSS). We found a significant negative correlation between BDNF serum levels and FA values in the right inferior fronto-occipital fasciculus and the right superior longitudinal fasciculus. These regions also showed a decrease in AD values in schizophrenia patients. Grey matter volumes were reduced in patients but there was no correlation between regional grey matter volumes and BDNF. The right superior longitudinal fasciculus has been repeatedly identified to exhibit microstructural changes in schizophrenia patients. Our findings of a negative correlation between BDNF and FA values in patients might indicate that BDNF is upregulated to compensate decreased structural connectivity as it induces neural plasticity and shows increased levels in damaged tissue. These findings of our pilot study are encouraging leads for future research in larger samples.
Introduction
Schizophrenia is a severe chronic neuropsychiatric disorder (1–3) that shows a heritability of about 0.8 (4). More than 100 common variants associated with schizophrenia have been identified so far. These variants map to a large range of diverse genes. Given this diversity of the genes involved, a comprehensive understanding of common pathophysiological mechanisms is still lacking. However, recent studies have highlighted an association with synaptic plasticity for many of these susceptibility genes (5). Consequently, signaling pathways involved in the formation and maintenance of synaptic connections might constitute a common hub, over which all these diverse gene variants exert identical or related pathophysiological effects.
The neurotrophins are a protein family that might play a key role in this regard, as its members exert core functions in synapse formation, axonal and dendritic outgrowth, and other neuroplastic processes (6, 7). One of the members of the neurotrophin family, brain-derived growth factor (BDNF), is involved in the differentiation and growth of neurons (8). It maintains neurons and induces plasticity of neurons in the central nervous system, as well as in the peripheral nervous system (8–11). It has various functions in the brain, for example, the maintenance of cortical dendrites (12). BDNF is also involved in neuronal processes associated with learning and memory (8, 13). Mechanistically, BDNF influences neuronal plasticity by activating intracellular signaling cascades via TrkB receptors. These signalling cascades activate the transcription of genes that induce cell differentiation and survival of neurons (8), as well as axonal and dendritic branching and growth (8, 9). In addition, TrkB receptor-associated pathways affect glutamergic neurotransmission (11, 14), which is hypothesized to be hypofunctional in schizophrenia (15).
Altered serum levels of BDNF in patients compared to healthy volunteers were shown for many different neuropsychiatric diseases (e.g. Alzheimers, Epilepsy, Autism, Depression or Bipolar Disorder) (7, 16–19). In particular, meta-analyses showed a reduction of BDNF serum levels in schizophrenia with moderate effect sizes and heterogeneity (20, 21). Most original studies reported decreased BDNF levels (22–25) as well, while others, however, showed increases (26, 27) or no significant differences in BDNF serum levels (28). One potential explanation for these seemingly contradictory findings is the circadian rhythm of BDNF secretion (29). As not all studies determined BDNF serum levels at a standardized time of the day, this might have influenced the results. In addition, age, BMI, and duration of disease and medication might influence the results (21, 30) as well. Furthermore, there might be influencing factors not yet identified. Consequently, BDNF might mediate its effects via more intricate mechanisms than a mere reduction of overall levels.
These molecular mechanisms might—at least in part—underlie distinct alterations in brain structure in schizophrenia. Grey matter changes with mainly a fronto-temporo-thalamo-basal ganglionary pattern have been robustly reported across neuroimaging studies (31, 32). Improved imaging techniques, namely diffusion tensor imaging (DTI), in the last decade have also found changes in fiber tract architecture in schizophrenia patients. As schizophrenia has long been regarded as a disease of altered neural connectivity (33), DTI opened a possibility to study structural connectivity in vivo. Fiber tracts commonly reported to be altered are located in the frontal and temporal deep white matter, mainly within the dominant hemisphere (34–36) but also bihemispheric (37–39). In addition, long fiber tracts connecting regions of the frontal lobe, thalamus and cingulate gyrus as well as hippocampus and amygdala, and occipital lobe have been implicated to exhibit structural changes in schizophrenia (35, 36). These fiber tracts are identified to play a role in language and working memory, functions that are altered in schizophrenia (40, 41)
In this pilot study, we wanted to explore whether there was an association between micro- and mesostructural grey matter/fiber tract changes and BDNF serum levels in schizophrenia.
Although studies on a possible association of BDNF and grey matter have been previously published (42, 43), there are none comparing BDNF levels and white matter (fiber tracts) in vivo. Correlating BDNF levels with white matter fiber tracts and grey matter could provide initial clues if there was a relation between molecular parameters and the anatomical changes that are observed in patients.
Therefore, we a) obtained serum samples to determine BDNF concentrations; b) conducted MRI scans, including diffusion-weighed sequences, to analyze changes in grey matter and structural connectivity; and c) finally correlated BDNF serum levels with imaging parameters to gather further information about the impact of BDNF on the long fiber tracts.
Materials and Methods
Subjects
The study protocol was approved by the institutional review board of RWTH Aachen University Hospital, Aachen, Germany. A total of 20 healthy volunteers (12 male and 8 female) recruited from the local community and 19 patients with schizophrenia (11 male and 8 female) recruited at the Department of Psychiatry, Psychotherapy, and Psychosomatics, RWTH Aachen University Hospital, were enrolled in this study, as, in part, previously reported by Neugebauer and colleagues (44). Two of the patients had to be excluded due to abnormalities in their MRI data sets (one because of grossly enlarged lateral ventricles, most likely due to infantile hypoxia, and another one due to technical artifacts).Written informed consent was given by all participants prior to inclusion. The participants were matched for age, gender, and BMI as those criteria have been reported to exert an impact on BDNF levels as well as all participants were right handed due to the effects of handedness on neuroimaging parameters (30, 45–47). Inclusion criteria for patients and healthy controls were as follows: 1. Age between 18 and 55, 2. no history of a psychiatric disease for the healthy volunteers; for the patients exclusively, diagnosis of schizophrenia according to ICD-10 (F20.X) by an experienced clinician at RWTH Aachen University Hospital, 3. Right handedness, 4. German as mother language, and 5. Central European origin. Exclusion criteria were as follows for the patients and healthy controls: 1. general exclusion criteria for MRI, 2. known gross morphological cerebral abnormalities, 3. gravidity, 4. drug use, 5. infectious or chronical illness.
For all patients on treatment, the equivalent dose of olanzapine was estimated (Table 1) (48). The duration of disease was recorded for each patient, and schizophrenia symptoms were assessed with the Positive and Negative Syndrome Scale (PANSS).
Demographical and characteristic data as age, BMI, and gender was analyzed using SPSS 25 (SPSS, Inc., Chicago, IL, USA). We used t-tests to compare age and BMI and a X2 test to compare gender ratios between groups as seen in Table 2.
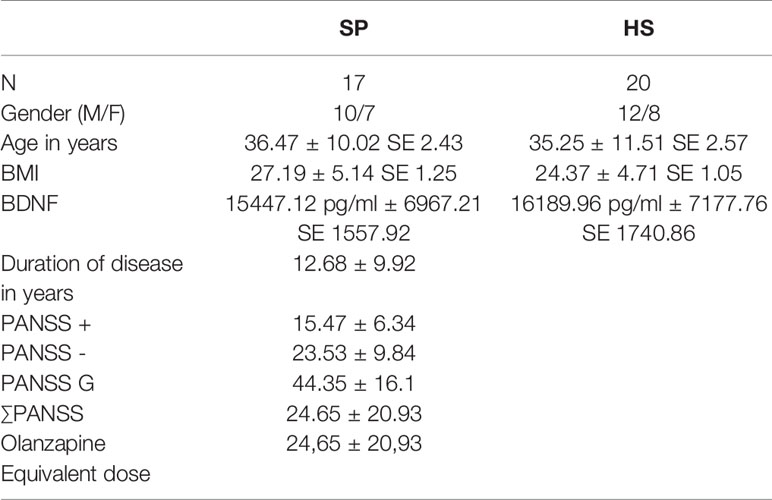
Table 2 Statistical analysis of the participants (HS, healthy subjects; SP, schizophrenia patients; N, number of subjects per group; BMI, Body Mass Index; BDNF, brain derived neurotrophic factor; ± Standard deviation; SE, standard error of the mean; PANSS - PANSS negative score, PANSS + - PANSS positive score; PANSS G - PANSS general score; ∑PANSS - PANSS total score).
BDNF Serum Level Assessment
Blood samples were drawn from all participants at the same time (8 am) in the morning to account for circadian patterns of peripheral BDNF levels (29, 49). Two serum gel tubes were drawn from each participant and centrifuged at 2000 rpm for 10 minutes. The serum was pipetted into tubes and stored at –80°Celsius at the Department of Psychiatry, Psychotherapy, and Psychosomatics, RWTH Aachen University Hospital until further analysis. Standard enzyme linked immunosorbent assays (ELISAs) (Quantikine ELISA, Human BDNF Immunoassay, R&D Systems) (see Supplementary Material) were used to detect the actual serum concentration following the protocol provided by the manufacturer. All samples were analyzed in duplicate in one parallel session. To check for a normal distribution in the dataset, we used the Kolmogorov-Smirnov Test. As this test indeed indicated a normal distribution, we decided to use a two-sample t-test with an uncorrected p-value < 0.05 between BDNF and the two groups.
MRI Data Acquisition
All MRI images were collected using a Siemens Trio 3T MRI scanner (Siemens Medical Systems, Erlangen, Germany) at the Department of Psychiatry, Psychotherapy, and Psychosomatics, RWTH Aachen University Hospital, immediately after the blood draw. A 32–channel coil was used to obtain the images. The head was immobilized using cushions to minimize head movement. In a session lasting 30 to 45 minutes, diffusion-weighted data, resting-state fMRI, and T1 anatomical sequences were acquired from all participants.
The T1 protocol for the Magnetization Prepared Rapid Acquisition Gradient Echo (MP-RAGE) sequence was as follows: sagittal slices 176, slice thickness = 1mm, field of view (FoV) = 250 mm, resolution matrix size 256 × 256 × 176, isotropic resolution of 1 mm, repetition time (TR)/echo time (TE)/inversion time (TI) = 1900 ms/2.52 ms/900 ms, flip angle (FP) = 9°, voxel size = 0.976 x 0.976 x 1 mm, duration = 7:49 min.
Diffusion-weighed sequences were acquired with 2.5 mm isotropic resolution, b-value of 1500 and 64 directions, and one non-diffusion image in each subject.
MRI Analysis
The MRI images were inspected manually by an experienced clinician to exclude inadequate data sets, e.g., due to gross morphological structural abnormalities, technical and motion artefacts. We had to exclude two data sets of schizophrenia patients (one because of grossly enlarged lateral ventricles, most likely due to infantile hypoxia, and another due to technical artifacts). These two data sets were also excluded from the study regarding BDNF correlation.
Voxel-Based Morphometry (VBM)
In brief, a VBM analysis was carried out as follows:
We used the Diffeomorphic Anatomical Registration Through Exponentiated Lie Algebra (DARTEL) segmentation algorithm of SPM 12 (50) for whole brain voxel-based morphometry (VBM) analysis on the NIFTI files of both schizophrenia patients and healthy controls. First, we conducted a group comparison between patients and controls. In a subsequent step, BDNF median values from two quantitative measurements were calculated and potential correlations with brain structural changes were investigated. All analyses were thresholded at a p-value of 0.05.
A detailed protocol of this approach can be found in Neugebauer et al. (44).
Tract-Based Spatial Statistics (TBSS)
The analysis of the diffusion-weighed data sets was carried out using the standard FSL tool package (http://www.fmrib.ox.ac.uk/fsl/) for TBSS according to the standard protocol (51, 52). At first, all Siemens default output DICOM data sets were converted into NIFTI files with the dcm2nii tool, and the DICOM headers were used to determine the b-value and b-vector. “Then, voxel-wise statistical analysis of the Fractional Anisotropy (FA) data was carried out using TBSS [Tract-Based Spatial Statistics (51, 53), part of FSL (52, 53)]. First, FA images were created by fitting a tensor model to the raw diffusion data using FDT, and then brain-extraction using BET (54). All of the subjects' FA data were then aligned into a common space using the nonlinear registration tool, FNIRT (55, 56), which uses a b-spline representation of the registration warp field (57). Thereafter, the mean FA image was created and thinned to create a mean FA skeleton (we used a threshold for FA ≥ 0.2), which represents the centers of all tracts common to the group. Each subject's aligned FA data was then projected onto this skeleton and the resulting data fed into voxelwise cross-subject statistics”. We used a threshold for FA ≥ 0.2. Each step of this protocol was reviewed visually. We performed Voxel-wise group comparisons (healthy vs patients). BDNF regressions were performed for FA maps of both groups separately and one analysis for both groups combined using FSL randomise (58). The same protocol was carried out for Axial Diffusivity (AD), Mean Diffusivity (MD), and Radial Diffusivity (RD). All analyses were carried out using TFCE (Threshold-Free Cluster Enhancement) at a p-value < 0.05, corrected for family-wise error (FWE).
Results
BDNF Serum Levels
There was no significant difference between the BDNF serum levels of the healthy volunteers and the schizophrenia patients (p-value of 0.752). The mean BDNF level of the controls was 15447.12 pg/ml (SD 6967.21, SD mean 1557.92), and the mean BDNF level of the patients was 16189.96 pg/ml (SD 7177.76, SD mean 1740.86) (Table 2). There was one outliner in the patient group, but even with this outliner removed, differences between the two groups did not reach statistical significance.
Regional Grey Matter Volumes and Their Correlations With BDNF Serum Levels
There were no significant correlations between regional grey matter volumes and BDNF serum levels.
As described previously, we found significant reductions of grey matter volume in schizophrenia patients in a widespread fronto-temporo-parietal network (44).
White Matter Changes and Their Correlations With BDNF Serum Levels
There were no significant differences between the healthy volunteers and schizophrenia patients regarding the group comparison of FA maps as well as MD and RD maps with a threshold of ≥ 0.2 and a p-value of 0.05 TFCE corrected. However, patients showed lower AD values in the right inferior fronto-occipital fasciculus and right superior longitudinal fasciculus, the same region where FA values correlated negatively with BDNF serum levels in patients (Figure 1).
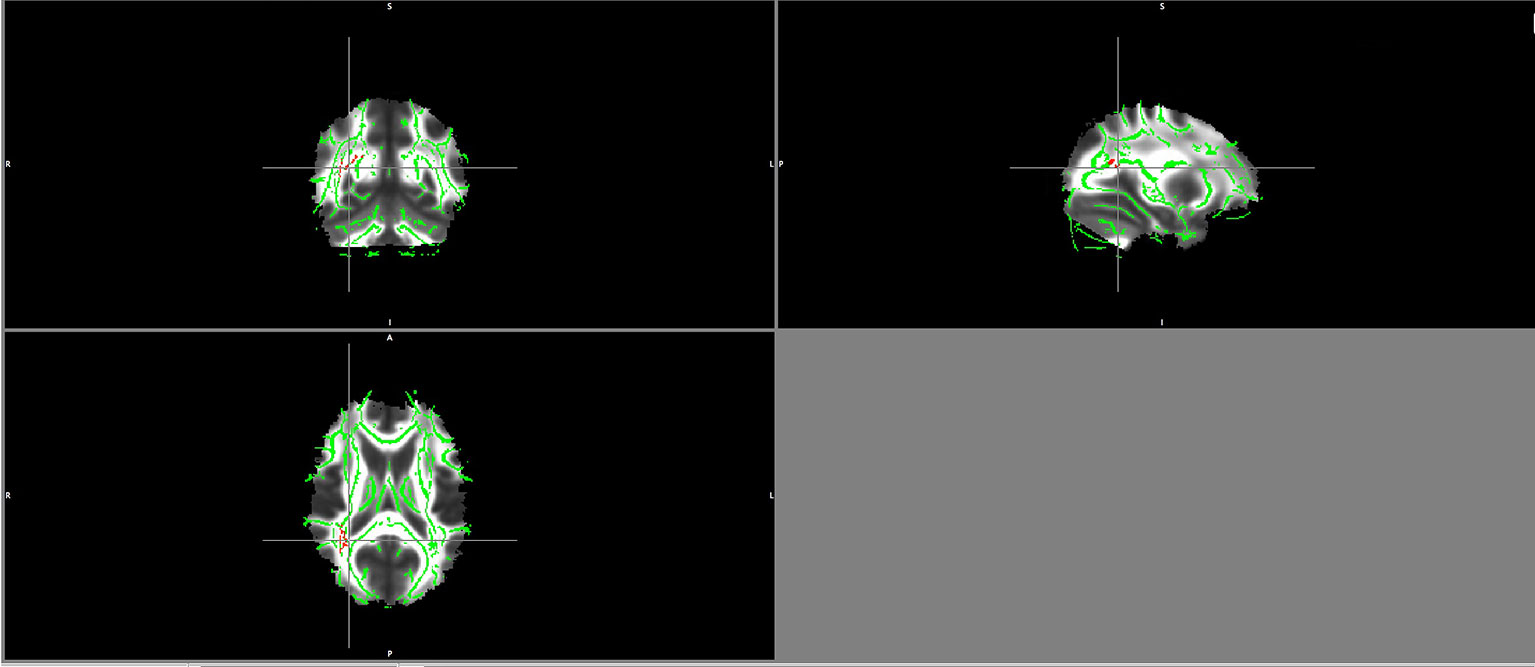
Figure 1 Lower AD values in patients maps in the right superior longitudinal fasciculus and right inferior fronto-occipital fasciculus (in red).
Significant negative correlations between BDNF serum levels and FA values in schizophrenia patients were detected in the right inferior fronto-occipital fasciculus and the right superior longitudinal fasciculus (Figure 2).
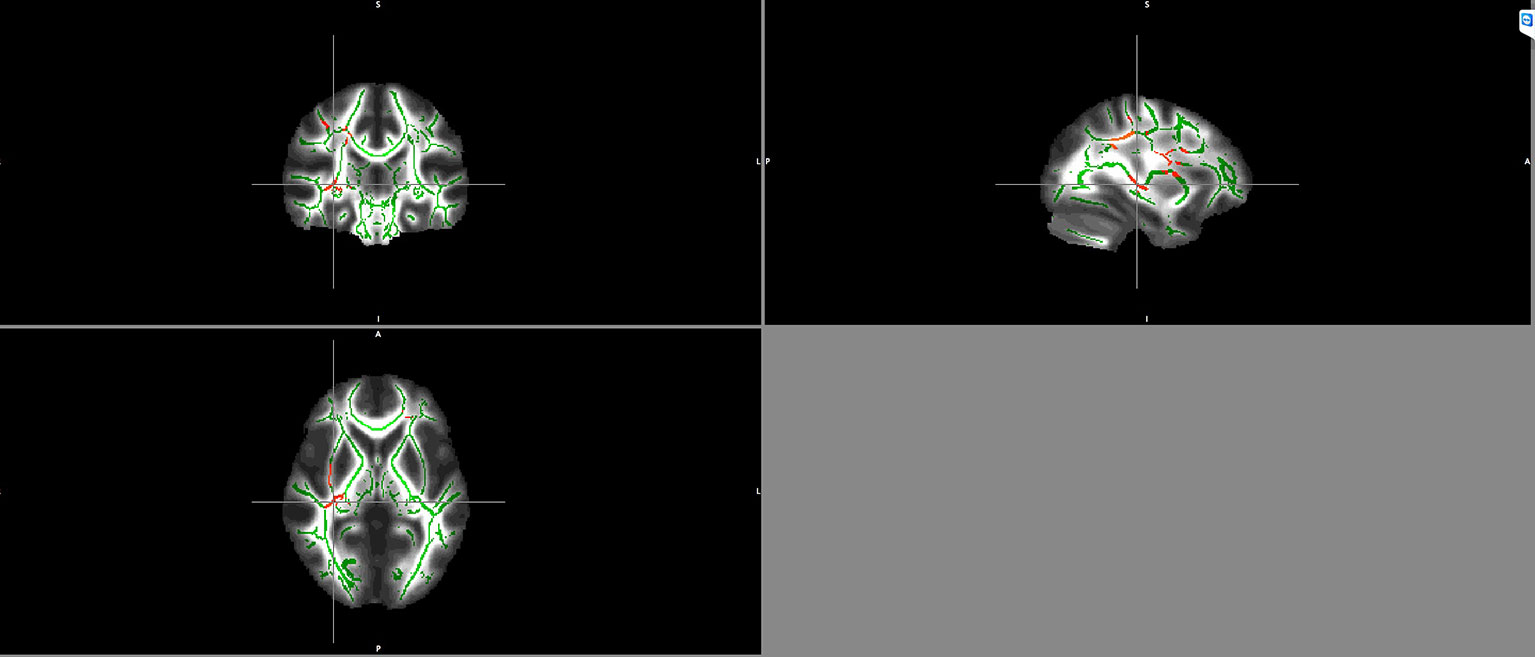
Figure 2 Negative correlation between BDNF serum levels and FA maps of patients in the right inferior fronto-occipital fasciculus and the right superior longitudinal fasciculus. Clusters identifying Voxels with significant negative correlations between FA and BDNF are highlighted in red.
Discussion
Our pilot study was one of the first to explore potential correlations between BDNF serum levels and meso- and macroscopic imaging parameters in schizophrenia patients. Our findings could provide first hints at potential molecular mechanisms underlying these brain structural changes in patients.
Neuroanatomical Changes in Patients Compared to Healthy Controls
We detected a decrease in AD in the right superior longitudinal fasciculus and right inferior fronto-occipital fasciculus in our patients, whereas RD did not change significantly. In general, AD is seen as a maker for axonal integrity (59, 60), whereas RD is seen as a marker for myelin integrity (61, 62). It is certainly challenging to infer from changes in DTI parameters on microstructural changes, especially in a small sample like ours. Nevertheless, it is tempting to speculate on the neurobiological underpinnings, as our study showed a decrease of AD in patients in the superior longitudinal fasciculus (SLF) and right inferior fronto-occipital fasciculus. We would interpret these reduced AD values as suggestive of a possible axonal pathology in schizophrenia patients. In particular, the SLF and the inferior fronto-occipital fasciculus are two areas that were previously shown to be altered in patients with schizophrenia (37, 63). Most studies found a decrease in FA values in these fiber tracts in schizophrenia patients (37, 63). However, only few studies (64–69) have investigated changes of AD and RD in schizophrenia so far. Their results were heterogenous. Some showed no difference in AD values compared to healthy controls, while others showed an increase of AD values accompanied by reduced FA values and increased RD values, which were interpreted as an indication for decreased myelinization of axons (70, 71).
In contrast to these prior studies, we found a decrease of AD values in schizophrenia patients. We interpreted it as possible alterations in axonal integrity. As schizophrenia is hypothesized to be a disease of impaired neural connectivity (72–75), changes in AD, hence, could indicate alterations in structural connectivity. Studies on the underlying histology (76, 77) showed that there is indeed decreased axonal tropism in brains of schizophrenia patients (78). In summary, we hypothesize that our results of reduced AD values in schizophrenia patients hint towards axonal changes as the “smallest common denominator” for schizophrenia patients.
The GMV in schizophrenia patients was reduced compared to healthy controls. These changes have repeatedly been interpreted as an indicator of perikaryal atrophy as a result of inactivity due to impaired synaptic plasticity (79, 80).
A Potential Role for BDNF in Fiber Tract Changes of Schizophrenia Patients
Our finding of a negative correlation between BDNF serum levels and the FA values of patients in the right inferior fronto-occipital fasciculus and the superior longitudinal fasciculus (SLF) might seem contrary to the current understanding of BDNF values in schizophrenia. One might expect a positive correlation of BDNF and FA values, as BDNF is known to induce physiological differentiation and growth of neurons (8), especially in axonal and dendritic outgrowth as well as the maintenance of these cellular structures (6, 7, 12). The function of BDNF in a pathophysiological framework such as schizophrenia though should be met with caution, as for example, genome wide association studies in schizophrenia have shown variants in multiple genes regulating neuronal and synaptic plasticity and dendritic growth. As a result, it is commonly expected that these biological functions are mediated by dys-/hypofunctional signaling pathways in schizophrenia (5). Hence, seeing increased dendritic growth and synaptic plasticity as a result of upregulated BDNF in schizophrenia patients and therefore resulting in a positive correlation with FA seems unlikely. We would hypothesize that a negative correlation of BDNF and FA values in the SLF is an indicator for an ongoing response mechanism due to alterations in synaptic, dendritic, and axonal plasticity but that this response mechanism is not able to accomplish a physiological response as BDNF downstream signaling is impaired and therefore BDNF is incapable of inducing neuronal plasticity and further dendritic growth. Our own findings of a negative correlation between BDNF serum levels and FA values in the SLF of schizophrenia patients might reflect this dysfunctional neurotrophic mechanism. Due to its neurotrophic effects mentioned above, increased BDNF concentrations have been reported in brain regions with tissue damage, indicating a role for BDNF in neuronal repair mechanisms (9, 12, 81). Consequently, structural impairments of the SLF could lead to a compensatory increase of BDNF levels, however, potentially due to a disruption of neurotrophic downstream signaling cascades (5), this obviously does not lead to a restitution of this tissue. These findings would indicate that dysregulated BDNF levels in schizophrenia patients might point to impaired repair mechanisms with elevated serum concentrations as a response to altered structural connectivity, rather than an early step in the causal chain that leads to these alterations in connectivity. In addition, the SLF has been repeatedly indicated as altered in schizophrenia patients by various studies (63, 82); Studies found that FA values were decreased in the SLF (83–85) in patient populations. This was interpreted by the authors as a potential mesostructural correlation of altered neural plasticity.
Decreased AD values were found in the same region as the negative correlation between FA values and BDNF levels. We, thus, hypothesize that changes in AD are the smallest common dominator in schizophrenia patients, whereas our findings of a negative correlation of BDNF and FA values are a result of increased BDNF levels due to more global fiber tract changes, as FA is a summative parameter for microstructural integrity (e.g., axonal diameter and packing density, myelination, etc.) (86, 87).
In summary, we hypothesize that BDNF levels increase as response to altered white matter possible axonal damage. The upregulated BDNF then tries to induce plasticity by activating its TrkB pathway, but as mentioned in literature, this signaling cascade is believed to be altered in patients with schizophrenia (26, 88, 89) so that BDNF cannot induce its effects of plasticity anymore (Figure 3).
There is also another important mechanism related to the pathophysiology of schizophrenia in which BDNF is involved in—glutamatergic neurotransmission. BDNF exerts its effects via two different pathways: TrkB and p57. Through TrkB activation, glutamate secretion and NMDA receptor function is modulated (14, 90). As stated in the disconnection hypothesis, modulation of synaptic plasticity through NMDA receptors is abnormal, so the growth of dendritic spines and axonal myelination is disrupted (33, 91). These dysfunctions might be associated with altered BDNF levels, as BDNF influences and—with higher levels—increases NMDA receptor-dependent myelination of axons (91). As we found a negative correlation between BDNF levels and FA maps in schizophrenia patients, it could be, as mentioned above, consecutive to altered structural connectivity in the SLF as a kind of feedback mechanism. The SLF is a central fiber tract connecting the frontal, temporal, occipital, and parietal lobe and has an impact in memory and spatial information processing (41), functions that are impaired in schizophrenia (84) and are also connected to BDNF. Through the TrkB receptor cascade, BDNF stimulates long time potentiation (LTP) in hippocampal neurona, which, in turn, is well known to play a key role in learning and memory (92). In addition, modification of glutamate levels influences characteristic positive and negative symptoms as well as disturbances in working memory (93).
Comparison of AD, MD, and RD With BDNF Levels
AD, MD, and RD values did not significantly correlate with BDNF values of patients and healthy subjects. This can be interpreted in different ways. FA is a summative parameter for microstructural integrity (e.g., axonal diameter and packing density, myelination, etc.) (86, 87), in general, that is sensitive to a variety of microstructural changes. Neuroimaging findings, in turn, have been reported to exhibit higher variance in schizophrenia patients than in healthy control groups (36, 94). This heterogeneity within the patient group, in turn, might obscure changes in other DTI parameters, especially since the sample enrolled in our pilot study was comparatively small. It will be an important issue for future studies with larger samples to achieve a better characterization of the microstructural changes that correlated with BDNF serum levels.
No Correlation of Grey Matter and BDNF Values
In addition, we did not find any correlation between BDNF and grey matter changes as suggested by earlier publications (42, 43). Our findings suggest that changes in BDNF serum levels in schizophrenia are mainly associated with pathologies in axons, dendrites, and/or myelination (9). Consequently, our findings would support a role for dysfunctional BDNF signaling as a failed repair mechanism in schizophrenia with consequences for neural connectivity (33, 95).
Limitations
One major limitation of our study is certainly the comparatively small sample size. The small number also does not allow any sub-analyses that might yield important insights into the exact relationship between BDNF serum levels and brain structural changes. However, it deserves to be pointed out that our project was intended as a pilot study and is, to the best of our knowledge, the first to examine potential correlations between BDNF serum levels and white matter changes in schizophrenia. Consequently, little is known about a relationship between BDNF and white matter microstructure in actual schizophrenia patients. More studies with larger sample sizes are needed to corroborate our findings further.
Our study is based on a one-time assessment and did not realize a longitudinal approach. It might be that medication (20, 84) has an impact on brain volume and white matter integrity, as well as BDNF levels. Another contributing factor exerting an effect on brain anatomy, and most likely, BDNF levels, certainly is duration of the disease (96). There are a number of studies investigating a possible association of brain volume loss and antipsychotic medication (32, 97–99). One of the largest longitudinal datasets suggests a correlation between cumulative antipsychotic medication and progressive brain volume loss (97) as does a follow-up study on the same cohort (32). This has led to the idea of a causal relationship between antipsychotic medication and neuroanatomical changes in schizophrenia. However, other publications have discussed that notion critically (99). Studies regarding a correlation of white matter integrity and antipsychotic treatment Xiao et al. (100), Huang et al. (101) Cho et al. (102), Zeng et al. (84), McNabb et al. (66) described heterogenous results. Most report an effect of antipsychotic treatment on white matter, but have yielded different findings regarding the directionality of these changes (84, 100, 102). One study, for example, found improved white matter integrity after antipsychotic treatment (84), while another described an opposite association (102). Thus, it seems unclear at this point what effects antipsychotic treatment might have on white matter in schizophrenia patients. Nevertheless, we certainly cannot rule out a possible influence of antipsychotic medication on our findings. Also, the broad distribution in age (30, 103) and BMI in this small sample size might have influenced our results as well as there might be factors we are not yet aware of that might influence BDNF serum levels. It also remains opaque if the relationship between BDNF serum levels and white matter changes is present at the onset of schizophrenia or if such a relationship manifests throughout the course of the disease (104).
Conclusion
To the best of our knowledge, this is the first study to investigate potential correlations between brain structural changes and BDNF serum levels in schizophrenia. We found a negative correlation between BDNF levels and FA values in the SLF, a fiber tract that connects frontal with temporal and also parietal and occipital regions and has been repeatedly implicated in schizophrenia. This negative correlation might reflect impaired repair mechanisms in schizophrenia patients. The lack of significant correlations between BDNF serum levels and grey matter changes highlights the importance of BDNF for synaptic plasticity, while it does not seem to have a significant pathophysiological effect for cell migration of perikaryal structure. Future studies enrolling larger collectives will have to corroborate these findings.
Data Availability Statement
The datasets for this manuscript are not publicly available due to local IRB regulations. Requests to access the datasets should be directed to Christine Hammans, christine.hammans@rwth-aachen.de.
Ethics Statement
The studies involving human participants were reviewed and approved by Ethics committee University Hospital Aachen. The patients/participants provided their written informed consent to participate in this study.
Author Contributions
TN-J designed the study, supervised data acquisition, analysis, and interpretation and corrected the manuscript. LM, MS, and AN performed informed consent. CH and VK analyzed the MRI data. KN helped with data acquisition and analysis. CH wrote the manuscript, assisted with data acquisition, analyzed, and interpreted the data. TW, TA, and UH corrected the manuscript.
Funding
This work was sponsored by the foundation program “START” of the Ministry of Science and Research of the state of North Rhine-Westphalia, Germany.
Conflict of Interest
The authors declare that the research was conducted in the absence of any commercial or financial relationships that could be construed as a potential conflict of interest.
Acknowledgments
We thank J. Weis for his contribution to the application for the START foundation.
Supplementary Material
The Supplementary Material for this article can be found online at: https://www.frontiersin.org/articles/10.3389/fpsyt.2020.00031/full#supplementary-material
References
1. Andreasen NC. Negative symptoms in schizophrenia. definition and reliability. Arch Gen Psychiatry (1982) 39(7):784–8. doi: 10.1001/archpsyc.1982.04290070020005
2. Andreasen NC. Methods for assessing positive and negative symptoms. Mod Probl Pharmacopsychiatry (1990) 24:73–88. doi: 10.1159/000418013
3. Andreasen NC, Carpenter WT. Diagnosis and classification of schizophrenia. Schizophr Bull (1993) 19(2):199–214. doi: 10.1093/schbul/19.2.199
4. Sullivan PF, Kendler KS, Neale MC. Schizophrenia as a complex trait: evidence from a meta-analysis of twin studies. Arch Gen Psychiatry (2003) 60(12):1187–92. doi: 10.1001/archpsyc.60.12.1187
5. Schizophrenia Working Group of the Psychiatric Genomics Consortium. Biological insights from 108 schizophrenia-associated genetic loci. Nature (2014) 511(7510):421–7. doi: 10.1038/nature13595
6. Avital A, Goshen I, Kamsler A, Segal M, Iverfeldt K, Richter-Levin G, et al. Impaired interleukin-1 signaling is associated with deficits in hippocampal memory processes and neural plasticity. Hippocampus (2003) 13(7):826–34. doi: 10.1002/hipo.10135
7. Nickl-Jockschat T, Michel TM. The role of neurotrophic factors in autism. Mol Psychiatry (2011) 16(5):478–90. doi: 10.1038/mp.2010.103
8. Reichardt LF. Neurotrophin-regulated signalling pathways. Philos Trans R Soc B: Biol Sci (2006) 361(1473):1545–64. doi: 10.1098/rstb.2006.1894
9. Numakawa T, Suzuki S, Kumamaru E, Adachi N, Richards M, Kunugi H. BDNF function and intracellular signaling in neurons. Histol Histopathol (2010) 25(2):237–58. doi: 10.14670/HH-25.237
10. Gomez-Pinilla F. Voluntary exercise induces a BDNF-mediated mechanism that promotes neuroplasticity. J Neurophysiol (2002) 88(5):2187–95. doi: 10.1152/jn.00152.2002
11. Thoenen H. Neurotrophins and neuronal plasticity. Science (1995) 270(5236):593–8. doi: 10.1126/science.270.5236.593
12. Gorski JA, Zeiler SR, Tamowski S, Jones KR. Brain-derived neurotrophic factor is required for the maintenance of cortical dendrites. J Neurosci (2003) 23(17):6856–65. doi: 10.1523/JNEUROSCI.23-17-06856.2003
13. Bekinschtein P, Cammarota M, Katche C, Slipczuk L, Rossato JI, Goldin A, et al. BDNF is essential to promote persistence of long-term memory storage. Proc Natl Acad Sci (2008) 105(7):2711–6. doi: 10.1073/pnas.0711863105
14. Carvalho AL, Caldeira MV, Santos SD, Duarte CB. Role of the brain-derived neurotrophic factor at glutamatergic synapses. Br J Pharmacol (2008) 153 Suppl 1:S310–24. doi: 10.1038/sj.bjp.0707509
15. Howes O, McCutcheon R, Stone J. Glutamate and dopamine in schizophrenia: An update for the 21st century. J Psychopharmacol (Oxford) (2015) 29(2):97–115. doi: 10.1177/0269881114563634
16. Song J-H, Yu J-T, Tan L. Brain-derived neurotrophic factor in alzheimer's disease: risk, mechanisms, and therapy. Mol Neurobiol (2015) 52(3):1477–93. doi: 10.1007/s12035-014-8958-4
17. Autry AE, Monteggia LM. Brain-derived neurotrophic factor and neuropsychiatric disorders. Pharmacol Rev (2012) 64(2):238–58. doi: 10.1124/pr.111.005108
18. Castrén E, Kojima M. Brain-derived neurotrophic factor in mood disorders and antidepressant treatments. Neurobiol Dis (2017) 97(Pt B):119–26. doi: 10.1016/j.nbd.2016.07.010
19. Iughetti L, Lucaccioni L, Fugetto F, Predieri B, Berardi A, Ferrari F. Brain-derived neurotrophic factor and epilepsy: a systematic review. Neuropeptides (2018) 72:23–9. doi: 10.1016/j.npep.2018.09.005
20. Green MJ, Matheson SL, Shepherd A, Weickert CS, Carr VJ. Brain-derived neurotrophic factor levels in schizophrenia: a systematic review with meta-analysis. Mol Psychiatry (2011) 16(9):960–72. doi: 10.1038/mp.2010.88
21. Fernandes BS, Steiner J, Berk M, Molendijk ML, Gonzalez-Pinto A, Turck CW, et al. Peripheral brain-derived neurotrophic factor in schizophrenia and the role of antipsychotics: meta-analysis and implications. Mol Psychiatry (2015) 20(9):1108–19. doi: 10.1038/mp.2014.117
22. Buckley PF, Pillai A, Evans D, Stirewalt E, Mahadik S. Brain derived neurotropic factor in first-episode psychosis. Schizophr Res (2007) 91(1-3):1–5. doi: 10.1016/j.schres.2006.12.026
23. Chen DC, Wang J, Wang B, Yang SC, Zhang CX, Zheng YL, et al. Decreased levels of serum brain-derived neurotrophic factor in drug-naïve first-episode schizophrenia: relationship to clinical phenotypes. Psychopharmacology (2009) 207(3):375–80. doi: 10.1007/s00213-009-1665-6
24. Ikeda Y, Yahata N, Ito I, Nagano M, Toyota T, Yoshikawa T, et al. Low serum levels of brain-derived neurotrophic factor and epidermal growth factor in patients with chronic schizophrenia. Schizophr Res (2008) 101(1-3):58–66. doi: 10.1016/j.schres.2008.01.017
25. Jindal RD, Pillai AK, Mahadik SP, Eklund K, Montrose DM, Keshavan MS. Decreased BDNF in patients with antipsychotic naïve first episode schizophrenia. Schizophr Res (2010) 119(1-3):47–51. doi: 10.1016/j.schres.2009.12.035
26. Mackin P, Gallagher P, Watson S, Young AH, Ferrier IN. Changes in brain-derived neurotrophic factor following treatment with mifepristone in bipolar disorder and schizophrenia. Aust N Z J Psychiatry (2007) 41(4):321–6. doi: 10.1080/00048670701213211
27. Reis HJ, Nicolato R, Barbosa IG, Teixeira do Prado PH, Romano-Silva MA, Teixeira AL. Increased serum levels of brain-derived neurotrophic factor in chronic institutionalized patients with schizophrenia. Neurosci Lett (2008) 439(2):157–9. doi: 10.1016/j.neulet.2008.05.022
28. Huang T-L, Lee C-T. Associations between serum brain-derived neurotrophic factor levels and clinical phenotypes in schizophrenia patients. J Psychiatr Res (2006) 40(7):664–8. doi: 10.1016/j.jpsychires.2005.11.004
29. Pluchino N, Cubeddu A, Begliuomini S, Merlini S, Giannini A, Bucci F, et al. Daily variation of brain-derived neurotrophic factor and cortisol in women with normal menstrual cycles, undergoing oral contraception and in postmenopause. Hum Reprod (2009) 24(9):2303–9. doi: 10.1093/humrep/dep119
30. Pillai A, Bruno D, Sarreal AS, Hernando RT, Saint-Louis LA, Nierenberg J, et al. Plasma BDNF levels vary in relation to body weight in females. PloS One (2012) 7(7):e39358. doi: 10.1371/journal.pone.0039358
31. Nickl-Jockschat T, Schneider F, Pagel AD, Laird AR, Fox PT, Eickhoff SB. Progressive pathology is functionally linked to the domains of language and emotion: meta-analysis of brain structure changes in schizophrenia patients. Eur Arch Psychiatry Clin Neurosci (2011) 261 Suppl 2:S166–71. doi: 10.1007/s00406-011-0249-8
32. Andreasen NC, Liu D, Ziebell S, Vora A, Ho B-C. Relapse duration, treatment intensity, and brain tissue loss in schizophrenia: a prospective longitudinal MRI study. Am J Psychiatry (2013) 170(6):609–15. doi: 10.1176/appi.ajp.2013.12050674
33. Friston K, Brown HR, Siemerkus J, Stephan KE. The dysconnection hypothesis (2016). Schizophr Res (2016) 176(2-3):83–94. doi: 10.1016/j.schres.2016.07.014
34. Ellison-Wright I, Bullmore E. Meta-analysis of diffusion tensor imaging studies in schizophrenia. Schizophr Res (2009) 108(1-3):3–10. doi: 10.1016/j.schres.2008.11.021
35. Kubicki M, McCarley R, Westin C-F, Park H-J, Maier S, Kikinis R, et al. A review of diffusion tensor imaging studies in schizophrenia. J Psychiatr Res (2007) 41(1-2):15–30. doi: 10.1016/j.jpsychires.2005.05.005
36. Vitolo E, Tatu MK, Pignolo C, Cauda F, Costa T, Ando' A, et al. White matter and schizophrenia: a meta-analysis of voxel-based morphometry and diffusion tensor imaging studies. Psychiatry Res Neuroimaging (2017) 270:8–21. doi: 10.1016/j.pscychresns.2017.09.014
37. Ellison-Wright I, Nathan PJ, Bullmore ET, Zaman R, Dudas RB, Agius M, et al. Distribution of tract deficits in schizophrenia. BMC Psychiatry (2014) 14:99. doi: 10.1186/1471-244X-14-99
38. Nakamura K, Kawasaki Y, Takahashi T, Furuichi A, Noguchi K, Seto H, et al. Reduced white matter fractional anisotropy and clinical symptoms in schizophrenia: a voxel-based diffusion tensor imaging study. Psychiatry Res (2012) 202(3):233–8. doi: 10.1016/j.pscychresns.2011.09.006
39. Pasternak O, Westin C-F, Dahlben B, Bouix S, Kubicki M. The Extent of Diffusion MRI Markers of Neuroinflammation and White Matter Deterioration in Chronic Schizophrenia. Schizophr Res (2014) 161(1):113–8. doi: 10.1016/j.schres.2014.07.031
40. Madhavan KM, McQueeny T, Howe SR, Shear P, Szaflarski J. Superior longitudinal fasciculus and language functioning in healthy aging. Brain Res (2014) 1562:11–22. doi: 10.1016/j.brainres.2014.03.012
41. Rizio AA, Diaz MT. Language, aging, and cognition: Frontal aslant tract and superior longitudinal fasciculus contribute toward working memory performance in older adults. Neuroreport (2016) 27(9):689–93. doi: 10.1097/WNR.0000000000000597
42. Song X, Quan M, Lv L, Li X, Pang L, Kennedy D, et al. Decreased cortical thickness in drug naïve first episode schizophrenia: in relation to serum levels of BDNF. J Psychiatr Res (2015) 60:22–8. doi: 10.1016/j.jpsychires.2014.09.009
43. Rao J, Chiappelli J, Kochunov P, Regenold WT, Rapoport SI, Hong LE. Is schizophrenia a neurodegenerative disease? Evidence from age-related decline of brain-derived neurotrophic factor in the brains of schizophrenia patients and matched nonpsychiatric controls. Neurodegener Dis (2015) 15(1):38–44. doi: 10.1159/000369214
44. Neugebauer K, Hammans C, Wensing T, Kumar V, Grodd W, Mevissen L, et al. Nerve Growth Factor Serum Levels Are Associated With Regional Gray Matter Volume Differences in Schizophrenia Patients. Front Psychiatry (2019) 10:275. doi: 10.3389/fpsyt.2019.00275
45. Lommatzsch M, Zingler D, Schuhbaeck K, Schloetcke K, Zingler C, Schuff-Werner P, et al. The impact of age, weight and gender on BDNF levels in human platelets and plasma. Neurobiol Aging (2005) 26(1):115–23. doi: 10.1016/j.neurobiolaging.2004.03.002
46. Li M, Chen H, Wang J, Liu F, Long Z, Wang Y, et al. Handedness- and Hemisphere-Related Differences in Small-World Brain Networks: A Diffusion Tensor Imaging Tractography Study. Brain Connect (2014) 4(2):145–56. doi: 10.1089/brain.2013.0211
47. Seizeur R, Magro E, Prima S, Wiest-Daesslé N, Maumet C, Morandi X. Corticospinal tract asymmetry and handedness in right- and left-handers by diffusion tensor tractography. Surg Radiol Anat (2013) 36(2):111–24. doi: 10.1007/s00276-013-1156-7
48. Gardner DM, Murphy AL, O'Donnell H, Centorrino F, Baldessarini RJ. International consensus study of antipsychotic dosing. Am J Psychiatry (2010) 167(6):686–93. doi: 10.1176/appi.ajp.2009.09060802
49. Begliuomini S, Lenzi E, Ninni F, Casarosa E, Merlini S, Pluchino N, et al. Plasma brain-derived neurotrophic factor daily variations in men: correlation with cortisol circadian rhythm. J Endocrinol (2008) 197(2):429–35. doi: 10.1677/JOE-07-0376
50. Ashburner J. A fast diffeomorphic image registration algorithm. NeuroImage (2007) 38(1):95–113. doi: 10.1016/j.neuroimage.2007.07.007
51. Smith SM, Jenkinson M, Johansen-Berg H, Rueckert D, Nichols TE, Mackay CE, et al. Tract-based spatial statistics: voxelwise analysis of multi-subject diffusion data. NeuroImage (2006) 31(4):1487–505. doi: 10.1016/j.neuroimage.2006.02.024
52. Smith SM, Johansen-Berg H, Jenkinson M, Rueckert D, Nichols TE, Miller KL, et al. Acquisition and voxelwise analysis of multi-subject diffusion data with tract-based spatial statistics. Nat Protoc (2007) 2(3):499–503. doi: 10.1038/nprot.2007.45
53. Smith SM, Jenkinson M, Woolrich MW, Beckmann CF, Behrens TEJ, Johansen-Berg H, et al. Advances in functional and structural MR image analysis and implementation as FSL. NeuroImage (2004) 23(S1):208–19.
55. Andersson JLR, Jenkinson M, Smith S. Non-linear optimisation. FMRIB Technical Report TR07JA1 (2007a). Available from www.fmrib.ox.ac.uk/analysis/techrep
56. Andersson JLR, Jenkinson M, Smith S. Non-linear registration, aka Spatial Normalisation FMRIB Technical Report TR07JA2 (2007b). Available from www.fmrib.ox.ac.uk/analysis/techrep
57. Rueckert D, Sonoda LI, Hayes C, Hill DL, Leach MO, Hawkes DJ. Nonrigid registration using free-form deformations: application to breast MR images. IEEE Trans Med Imaging (1999) 18(8):712–21. doi: 10.1109/42.796284
58. Winkler AM, Ridgway GR, Webster MA, Smith SM, Nichols TE. Permutation inference for the general linear model. NeuroImage (2014) 92:381–97. doi: 10.1016/j.neuroimage.2014.01.060
59. Sun S-W, Liang H-F, Trinkaus K, Cross AH, Armstrong RC, Song S-K. Noninvasive detection of cuprizone induced axonal damage and demyelination in the mouse corpus callosum. Magn Reson Med (2006) 55(2):302–8. doi: 10.1002/mrm.20774
60. Sun S-W, Liang H-F, Le TQ, Armstrong RC, Cross AH, Song S-K. Differential sensitivity of in vivo and ex vivo diffusion tensor imaging to evolving optic nerve injury in mice with retinal ischemia. NeuroImage (2006) 32(3):1195–204. doi: 10.1016/j.neuroimage.2006.04.212
61. Song S-K, Sun S-W, Ju W-K, Lin S-J, Cross AH, Neufeld AH. Diffusion tensor imaging detects and differentiates axon and myelin degeneration in mouse optic nerve after retinal ischemia. NeuroImage (2003) 20(3):1714–22. doi: 10.1016/j.neuroimage.2003.07.005
62. Song S-K, Sun S-W, Ramsbottom MJ, Chang C, Russell J, Cross AH. Dysmyelination revealed through MRI as increased radial (but unchanged axial) diffusion of water. NeuroImage (2002) 17(3):1429–36. doi: 10.1006/nimg.2002.1267
63. Oestreich LK, Lyall AE, Pasternak O, Kikinis Z, Newell DT, Savadjiev P, et al. Characterizing white matter changes in chronic schizophrenia: A free-water imaging multi-site study. Schizophr Res (2017) 189:153–61. doi: 10.1016/j.schres.2017.02.006
64. Onay A, Yapıcı Eser H, Ulaşoğlu Yıldız Ç, Aslan S, Talı ET. A combined VBM and DTI study of schizophrenia: bilateral decreased insula volume and cerebral white matter disintegrity corresponding to subinsular white matter projections unlinked to clinical symptomatology. Diagn Interv Radiol (2017) 23(5):390–7. doi: 10.5152/dir.2017.16519
65. Fitzsimmons J, Schneiderman JS, Whitford TJ, Swisher T, Niznikiewicz MA, Pelavin PE, et al. Cingulum bundle diffusivity and delusions of reference in first episode and chronic schizophrenia. Psychiatry Res (2014) 224(2):124–32. doi: 10.1016/j.pscychresns.2014.08.002
66. McNabb CB, Kydd R, Sundram F, Soosay I, Russell BR. Differences in white matter connectivity between treatment-resistant and treatment-responsive subtypes of schizophrenia. Psychiatry Res Neuroimaging (2018) 282:47–54. doi: 10.1016/j.pscychresns.2018.11.002
67. Wigand M, Kubicki M, Clemm von Hohenberg C, Leicht G, Karch S, Eckbo R, et al. Auditory verbal hallucinations and the interhemispheric auditory pathway in chronic schizophrenia. World J Biol Psychiatry (2015) 16(1):31–44. doi: 10.3109/15622975.2014.948063
68. Park JY, Park H-J, Kim D-J, Kim J-J. Positive symptoms and water diffusivity of the prefrontal and temporal cortices in schizophrenia patients: a pilot study. Psychiatry Res (2014) 224(1):49–57. doi: 10.1016/j.pscychresns.2014.07.003
69. Abdul-Rahman MF, Qiu A, Sim K. Regionally specific white matter disruptions of fornix and cingulum in schizophrenia. PloS One (2011) 6(4):e18652. doi: 10.1371/journal.pone.0018652
70. Whitford TJ, Kubicki M, Schneiderman JS, O'Donnell LJ, King R, Alvarado JL, et al. Corpus callosum abnormalities and their association with psychotic symptoms in patients with schizophrenia. Biol Psychiatry (2010) 68(1):70–7. doi: 10.1016/j.biopsych.2010.03.025
71. Levitt JJ, Alvarado JL, Nestor PG, Rosow L, Pelavin PE, McCarley RW, et al. Fractional anisotropy and radial diffusivity: diffusion measures of white matter abnormalities in the anterior limb of the internal capsule in schizophrenia. Schizophr Res (2012) 136(1-3):55–62. doi: 10.1016/j.schres.2011.09.009
72. Marenco S, Stein JL, Savostyanova AA, Sambataro F, Tan H-Y, Goldman AL, et al. Investigation of anatomical thalamo-cortical connectivity and FMRI activation in schizophrenia. Neuropsychopharmacology (2012) 37(2):499–507. doi: 10.1038/npp.2011.215
73. Rapoport JL, Giedd JN, Gogtay N. Neurodevelopmental model of schizophrenia: Update 2012. Mol Psychiatry (2012) 17(12):1228–38. doi: 10.1038/mp.2012.23
74. Nieto R, Kukuljan M, Silva H. BDNF and schizophrenia: from neurodevelopment to neuronal plasticity, learning, and memory. Front Psychiatry (2013) 4:45. doi: 10.3389/fpsyt.2013.00045
75. Kubota M, Miyata J, Sasamoto A, Sugihara G, Yoshida H, Kawada R, et al. Thalamocortical disconnection in the orbitofrontal region associated with cortical thinning in schizophrenia. JAMA Psychiatry (2013) 70(1):12–21. doi: 10.1001/archgenpsychiatry.2012.1023
76. Uranova NA, Vostrikov VM, Vikhreva OV, Zimina IS, Kolomeets NS, Orlovskaya DD. The role of oligodendrocyte pathology in schizophrenia. Int J Neuropsychopharmacol (2007) 10(4):537–45. doi: 10.1017/S1461145707007626
77. Uranova NA, Vikhreva OV, Rachmanova VI, Orlovskaya DD. Ultrastructural alterations of myelinated fibers and oligodendrocytes in the prefrontal cortex in schizophrenia: a postmortem morphometric study. Schizophr Res Treat (2011) 2011:325789. doi: 10.1155/2011/325789
78. Najjar S, Pearlman DM. Neuroinflammation and white matter pathology in schizophrenia: systematic review. Schizophr Res (2015) 161(1):102–12. doi: 10.1016/j.schres.2014.04.041
79. Douaud G, Smith S, Jenkinson M, Behrens T, Johansen-Berg H, Vickers J, et al. Anatomically related grey and white matter abnormalities in adolescent-onset schizophrenia. Brain (2007) 130(Pt 9):2375–86. doi: 10.1093/brain/awm184
80. Sasamoto A, Miyata J, Kubota M, Hirao K, Kawada R, Fujimoto S, et al. Global association between cortical thinning and white matter integrity reduction in schizophrenia. Schizophr Bull (2014) 40(2):420–7. doi: 10.1093/schbul/sbt030
81. Chiang M-C, Barysheva M, Toga AW, Medland SE, Hansell NK, James MR, et al. BDNF gene effects on brain circuitry replicated in 455 twins. NeuroImage (2011) 55(2):448–54. doi: 10.1016/j.neuroimage.2010.12.053
82. Fitzsimmons J, Kubicki M, Shenton ME. Review of functional and anatomical brain connectivity findings in schizophrenia. Curr Opin Psychiatry (2013) 26(2):172–87. doi: 10.1097/YCO.0b013e32835d9e6a
83. Kubicki M, Park H, Westin CF, Nestor PG, Mulkern RV, Maier SE, et al. DTI and MTR abnormalities in schizophrenia: analysis of white matter integrity. NeuroImage (2005) 26(4):1109–18. doi: 10.1016/j.neuroimage.2005.03.026
84. Zeng B, Ardekani BA, Tang Y, Zhang T, Zhao S, Cui H, et al. Abnormal white matter microstructure in drug-naive first episode schizophrenia patients before and after eight weeks of antipsychotic treatment. Schizophr Res (2016) 172(1-3):1–8. doi: 10.1016/j.schres.2016.01.051
85. Zhang X, Gao J, Zhu F, Wang W, Fan Y, Ma Q, et al. Reduced white matter connectivity associated with auditory verbal hallucinations in first-episode and chronic schizophrenia: a diffusion tensor imaging study. Psychiatry Res Neuroimaging (2018) 273:63–70. doi: 10.1016/j.pscychresns.2018.01.002
86. Horsfield MA, Jones DK. Applications of diffusion-weighted and diffusion tensor MRI to white matter diseases - a review. NMR BioMed (2002) 15(7-8):570–7. doi: 10.1002/nbm.787
87. Farrell JA, Landman BA, Jones CK, Smith SA, Prince JL, van Zijl PC, et al. Effects of signal-to-noise ratio on the accuracy and reproducibility of diffusion tensor imaging-derived fractional anisotropy, mean diffusivity, and principal eigenvector measurements at 1.5 T. J Magn Reson Imaging (2007) 26(3):756–67. doi: 10.1002/jmri.21053
88. Mohammadi A, Amooeian VG, Rashidi E. Dysfunction in brain-derived neurotrophic factor signaling pathway and susceptibility to schizophrenia, parkinson's and alzheimer's diseases. Curr Gene Ther (2018) 18(1):45–63. doi: 10.2174/1566523218666180302163029
89. Pillai A. Brain-derived neurotropic factor/TrkB signaling in the pathogenesis and novel pharmacotherapy of schizophrenia. Neurosignals (2008) 16(2-3):183–93. doi: 10.1159/000111562
90. Sasi M, Vignoli B, Canossa M, Blum R. Neurobiology of local and intercellular BDNF signaling. Pflugers Arch (2017) 469(5-6):593–610. doi: 10.1007/s00424-017-1964-4
91. Lundgaard I, Luzhynskaya A, Stockley JH, Wang Z, Evans KA, Swire M, et al. Neuregulin and BDNF induce a switch to NMDA receptor-dependent myelination by oligodendrocytes. PloS Biol (2013) 11(12):e1001743. doi: 10.1371/journal.pbio.1001743
92. Lu B, Nagappan G, Lu Y. BDNF and synaptic plasticity, cognitive function, and dysfunction. Handb Exp Pharmacol (2014) 220:223–50. doi: 10.1007/978-3-642-45106-5_9
93. Gargiulo PÁ, Landa De Gargiulo AI. Glutamate and modeling of schizophrenia symptoms: review of our findings: 1990-2014. Pharmacol Rep (2014) 66(3):343–52. doi: 10.1016/j.pharep.2014.03.010
94. Kelly S, Jahanshad N, Zalesky A, Kochunov P, Agartz I, Alloza C, et al. Widespread white matter microstructural differences in schizophrenia across 4322 individuals: results from the ENIGMA Schizophrenia DTI Working Group. Mol Psychiatry (2018) 23(5):1261–9. doi: 10.1038/mp.2017.170
95. Friston KJ. Schizophrenia and the disconnection hypothesis. Acta Psychiatr Scand Suppl (1999) 395:68–79. doi: 10.1111/j.1600-0447.1999.tb05985.x
96. Wu C-H, Hwang T-J, Chen Y-J, Hsu Y-C, Lo Y-C, Liu C-M, et al. Primary and secondary alterations of white matter connectivity in schizophrenia: a study on first-episode and chronic patients using whole-brain tractography-based analysis. Schizophr Res (2015) 169(1-3):54–61. doi: 10.1016/j.schres.2015.09.023
97. Ho B-C, Andreasen NC, Ziebell S, Pierson R, Magnotta V. Long-term antipsychotic treatment and brain volumes: a longitudinal study of first-episode schizophrenia. Arch Gen Psychiatry (2011) 68(2):128–37. doi: 10.1001/archgenpsychiatry.2010.199
98. Moncrieff J, Leo J. A systematic review of the effects of antipsychotic drugs on brain volume. Psychol Med (2010) 40(9):1409–22. doi: 10.1017/S0033291709992297
99. van Haren N, Kahn R. Letter to the editor: a comment on ‘a systematic review of the effects of antipsychotic drugs on brain volume' by Moncrieff & Leo (2010). Psychol Med (2010) 40(12):2105–6. doi: 10.1017/S0033291710001686
100. Xiao Y, Sun H, Shi S, Jiang D, Tao B, Zhao Y, et al. White matter abnormalities in never-treated patients with long-term schizophrenia. Am J Psychiatry (2018) 175(11):1129–36. doi: 10.1176/appi.ajp.2018.17121402
101. Huang J-Y, Liu C-M, Hwang T-J, Chen Y-J, Hsu Y-C, Hwu H-G, et al. Shared and distinct alterations of white matter tracts in remitted and nonremitted patients with schizophrenia. (2018). Hum Brain Mapp 39(5):2007–19. doi: 10.1016/j.schres.2014.05.020
102. Cho SJ, Kim M-K, Bang SY, Bang M, Lee S-H. White matter integrity associated with severity reductions in positive symptoms after amisulpride treatment in drug-free patients with schizophrenia. Neurosci Lett (2018) 685:131–6. doi: 10.1016/j.neulet.2018.08.036
103. Erickson KI, Prakash RS, Voss MW, Chaddock L, Heo S, McLaren M, et al. Brain-derived neurotrophic factor is associated with age-related decline in hippocampal volume. J Neurosci (2010) 30(15):5368–75. doi: 10.1523/JNEUROSCI.6251-09.2010
Keywords: schizophrenia, brain derived neurotrophic factor, diffusion tensor imaging, Tract-based Spatial Statistics, neuroimaging, superior longitudinal fasciculus, dysconnectivity hypothesis
Citation: Hammans C, Neugebauer K, Kumar V, Mevissen L, Sternkopf MA, Novakovic A, Wensing T, Habel U, Abel T and Nickl-Jockschat T (2020) BDNF Serum Levels are Associated With White Matter Microstructure in Schizophrenia - A Pilot Study. Front. Psychiatry 11:31. doi: 10.3389/fpsyt.2020.00031
Received: 04 October 2019; Accepted: 10 January 2020;
Published: 21 February 2020.
Edited by:
Jerome Brunelin, INSERM U1028 Centre de Recherche en Heurosciences de Lyon, FranceReviewed by:
Sara Poletti, Vita-Salute San Raffaele University, ItalyHikaru Hori, University of Occupational and Environmental Health Japan, Japan
Copyright © 2020 Hammans, Neugebauer, Kumar, Mevissen, Sternkopf, Novakovic, Wensing, Habel, Abel and Nickl-Jockschat. This is an open-access article distributed under the terms of the Creative Commons Attribution License (CC BY). The use, distribution or reproduction in other forums is permitted, provided the original author(s) and the copyright owner(s) are credited and that the original publication in this journal is cited, in accordance with accepted academic practice. No use, distribution or reproduction is permitted which does not comply with these terms.
*Correspondence: Christine Hammans, christine.hammans@rwth-aachen.de