- 1Centre for Neuroscience Studies, Queen's University, Kingston, ON, Canada
- 2Department of Psychiatry, Queen's University, Kingston, ON, Canada
- 3Institute of Medical Biochemistry Leopoldo De Meis, Federal University of Rio de Janeiro, Rio de Janeiro, Brazil
Neuropsychiatric disorders and type 2 diabetes (T2D) are major public health concerns proposed to be intimately connected. T2D is associated with increased risk of dementia, neuropsychiatric and mood disorders. Evidences of the involvement of insulin signaling on brain mechanisms related to depression indicate that insulin resistance, a hallmark of type 2 diabetes, could develop in the brains of depressive patients. In this article, we briefly review possible molecular mechanisms associating defective brain insulin signaling with reward system, neurogenesis, synaptic plasticity and hypothalamic-pituitary-adrenal (HPA) stress axis in depression. We further discuss the involvement of tumor necrosis factor α (TNFα) promoting defective insulin signaling and depressive-like behavior in rodent models. Finally, due to the high resistant rate of anti-depressants, novel insights into the link between insulin resistance and depression may advance the development of alternative treatments for this disease.
Introduction
Depressive disorders, type 2 diabetes (T2D) and obesity are among the top causes of years lived with disability, a widely accepted measure of disease burden on society (1). Major Depressive Disorder represents the highest burden among mental disorders, with a significant impact on individuals, their families and the community at large. The World Health Organization estimates that more than 300 million people suffer from depressive disorders (2). In parallel, T2D has been estimated to afflict >400 million adults worldwide (2). T2D is characterized by peripheral insulin resistance that culminates in hyperglycemia (3). While T2D has precise diagnostic parameters, that includes fasting serum glucose levels and glycated hemoglobin blood levels; depression diagnoses is based on the persistence of some of the following symptoms for >2 weeks: sad/anxious mood, hopelessness, helplessness, decreased energy, appetite/weight changes, headaches, sleep changes, feelings of guilt, loss of interest, decreased concentration, psychomotor retardation and suicide attempts (4).
T2D is a risk factor not only for the development of cardiovascular diseases, but also to neurological and psychiatric disorders, including Alzheimer's disease (AD) (5, 6). Some clinical reports and meta-analyses indicate a correlation between T2D and depression with a bi-directional increased risk between both conditions (6–9). Albeit evidences correlating T2D and depression, confounders implicated in epidemiological studies hamper the temporal order assessment of those co-morbid cases. As a result, the association between both diseases is still unclear (8, 10, 11). Insulin signaling play a role in neuronal dysfunction and cognitive decline in AD (12, 13) and it emerged as a possible mechanism underlying alterations in the brain and in behavior in mood disorders (14). In children, the severity of depressive symptoms predicted the development of insulin resistance (15) and a recent report demonstrated that worst insulin resistance correlated with more pronounced depressive symptoms and dysfunction of the anterior cingulate cortex-hippocampal motivational network in a cohort of obese depressed youths (16).
In this minireview, we highlight a possible role of decreased insulin signaling in the brain, as a result of metabolic dyshomeostasis, in mechanisms involved with depressive behavior.
Molecular Links Between Depression and Diabetes
Brain Insulin Resistance
Insulin has been implicated with diverse central roles, like modulating feeding behavior and energy maintenance by the hypothalamus, as well as memory-related processes by the hippocampus (13, 17–19). Insulin receptors are expressed throughout the brain, including regions classically involved with mood regulation, such as the nucleus accumbens (NAc), the ventral tegmental area (VTA), the amygdala, and the raphe nuclei (20, 21). The knockdown of insulin receptors in the hypothalamus of rats triggered depressive and anxiety-like behaviors in mice (22). Anxiety and depressive-like behaviors were further reported in mice with neuronal-specific knockout of insulin receptors (NIRKO). NIRKO mice also exhibited mitochondrial dysfunction, oxidative stress and increased monoamine oxidase expression and dopamine turnover in the mesolimbic system (23). Interestingly, altered behavior was detected in 17-month-old NIRKO mice, but not in younger animals (23). It is important to note that by this age, these animals display increased white adipose tissue and plasma leptin concentration (17), raising the possibility of the behavior response being a secondary effect to the absence of insulin signaling in neurons.
A recent study demonstrated that the knockdown of insulin receptors in astrocytes also generates anxiety and depressive-like behavior in mice, via decreased purinergic signaling and altered dopamine release (24). A recent post-mortem analysis in the brain of patients diagnosed with mental illness has observed a correlation between gene expression of proteins involved with both the dopaminergic system and the insulin signaling (25), supporting the idea that insulin could regulate the dopaminergic response. Oppositely, another report observed that deleting insulin receptors from dopaminergic neurons had no impact on anxiety or depressive-like behavior in young adult mice (26). The absence of altered behavior in this model counteracts the idea of insulin regulating the dopaminergic system. Other possibilities to explain this phenotype are the development of compensatory mechanisms, or that, similar to what is observed in the NIRKO mice, altered behavior would be detected in older animals.
Defective brain insulin signaling in T2D patients has been associated with impaired transport of the hormone across the blood-brain barrier (27). Markers of impaired insulin signaling are present in the brain of db/db mice, a transgenic model for T2D that lacks the long isoform of the leptin receptor (28). These mice also exhibit increased immobility time in the forced swim test as early as 5 weeks of age, coinciding with an initial metabolic dysregulation, including hyperglycemia, increased food and water intake and body weight (29–31). This animal model also presents with progressive anxious and psychosis-like behavior that progress with age (30). Interestingly, since most metabolic parameters are also aggravated with aging in the db/db mice, it hinders an accurate determination of the major player influencing the behavior. High-fat diet (HFD) promotes T2D symptoms, as well as anxiety and depressive-like behavior in wild-type mice associated with impaired brain insulin signaling (32). Parallelly, HFD disrupts brain reward system of mice, by altering dopamine-related proteins in the VTA, NAc and dorsolateral striatum (32). Overall, further studies designed to investigate a direct correlation between brain insulin dysfunction and depressive-like behavior are needed in the field.
Neurogenesis and Synaptic Plasticity
Hippocampal neurogenesis, a process in which neural progenitors from the subgranular zone differentiate into new neurons at the dentate gyrus, is proposed to be involved with depression and to be impaired in diabetes (33, 34). HFD impairs cell proliferation, insulin signaling and the Akt/glycogen synthase kinase 3β (GSK3β) activation promoted by serotonin in the dentate gyrus of the hippocampus. Interestingly, replacing HFD by chow diet recovered depressive symptoms and Akt/GSK3β response to insulin, even without a complete recovery of body weight. Neurogenesis was partially recovered by a chow diet replacement, suggesting that it was not the only mechanism implicated with the beneficial effect promoted by the regular diet (35). Other hormones like Insulin-like growth factor I (IGF-I) and leptin activate Akt and GSK3β pathway and mediate hippocampal neurogenesis (36–39). Interestingly, downregulation of those hormones are observed in the hippocampus of rodent models of T2D, being other possible targets to the link between T2D and depression (40, 41). Neurogenesis is also proposed to be impaired in T2D due to mitochondrial dysfunction (42). Peroxisome proliferator-activated receptor gamma (PPARγ) agonists increase central insulin sensitivity, mitochondrial biogenesis and prevent depressive-like behavior in rats through facilitation of hippocampal neurogenesis (43, 44).
Defective synaptic plasticity may lead to impairment of stress adaptation, prompting the onset of depression (45). In the food reward circuitry, insulin actions modulate synaptic plasticity in a concentration, time and brain region -dependent manner [for a review see (46)]. For instance, insulin promotes long-term depression of glutamatergic afferent connections into the VTA (47), but increases the activity of striatal cholinergic interneurons, elevating dopamine release into the NAc (48). Downregulation of insulin receptors in the hippocampus of rats impaired proper long-term potentiation response mediated by high frequency stimulation and decreased glutamate receptors levels (19). This approach also worsened learning behavior in a similar fashion to what is observed in T2D rodent models (19). Altogether, data indicate that brain insulin resistance can impair physiological mechanisms of reward and learning that would ultimately elicit depressive symptoms.
Hypothalamic-Pituitary-Adrenal (HPA) Axis
Chronic psychological stress is associated with neuropsychiatric diseases, including depression and also with T2D (49–51). A well-supported theory of depression and T2D pathophysiology involves allostatic load on the hypothalamic-pituitary-adrenal (HPA) axis, a key mediator of the stress response regulating the secretion of glucocorticoids by the adrenal gland (52, 53). In an allostatic model, constant input throughout the life course of an individual will generate learning and adaptive responses, but it may promote ablation of the HPA axis and the emergence of diseases (14). Supporting this idea, variation of cortisol level is observed in the blood of depressive and patients with T2D compared to healthy control participants (54, 55).
Physiologically, insulin elevates adrenocorticotropin and corticosterone hormone levels, promoting HPA axis activation in rats (56). Also, insulin receptor knockdown at the arcuate nucleus of the hypothalamus led to reduced vasopressin response to restraint stress, suggesting that brain insulin resistance could cause disturbances in the HPA axis (14, 56). The hippocampus is proposed to exert negative feedback regulation on the HPA axis (57). Chronic unpredictable stress modulates glucocorticoid and serotonin receptors in the hippocampus of rats, similar to what was observed in the hippocampus of suicidal victims with medical history of depression (58). Interestingly, diabetic rats show decreased expression of hippocampal glucose-dependent type 1 glucocorticoid receptor (59) and lower HPA axis regulation by insulin with decreased response of corticosteroid receptor expression by the hippocampus (60). Collectively, results suggest that brain insulin signaling dysfunction could impair the HPA axis normal response to stress, possibly facilitating the development of depression.
Tumor Necrosis Factor α (TNFα)
T2D patients have elevated circulating levels of pro-inflammatory markers, in particular of the cytokine tumor necrosis factor α (TNFα) (61). Clinical studies indicate that blood concentrations of the pro-inflammatory cytokine tumor necrosis factor α (TNFα) correlates with depression and impaired performance on memory tests (62, 63). In a Bavarian cohort with history of depression elevated blood levels of TNFα, two isoforms of the soluble TNFα receptor and diabetes were commonly observed (64). In mice, intracerebroventricular administration of TNFα induced depressive-like behavior in the forced swim and tail suspension tests, effects that were counteracted by the ablation of the TNFα receptor 1 (TNFR1) (65). On the other hand, a recent study demonstrated that TNFR1 was involved with anxiety-like behavior analyzed by the open-field test, but not with more related depression tests like the forced swim test (66).
TNFα was shown to contribute to depressive states by modifying the serotonin system. For instance, this cytokine can activate the enzyme indoleamine 2,3-dioxygenase degrading the precursor molecule tryptophan and indirectly decreasing brain serotonin levels (67, 68). This cytokine also promotes blood-brain barrier (BBB) disruption in patients with T2D and in a mouse model of depression (69, 70), which could ultimately lead to loss of the BBB transport regulation of other inflammatory signals, and exacerbate allostatic load to the HPA axis, leading to its dysregulation (71–74).
TNFα promotes insulin resistance by the phosphorylation of insulin receptor substrate 1 (IRS1) on serine residues, via the activation of cellular stress-response kinases, including IκB kinase β (IKKβ), c-Jun N-terminal kinase (JNK), and protein kinase RNA-activated (PKR) (12, 75–78). Increased levels of IRS1 phosphorylated at serine residues are observed in the hippocampus and in the hypothalamus of AD mouse models. In the AD context, activation of stress-response kinases was shown to regulate brain insulin signaling impairment and memory behavior tests (12, 13, 75, 78). TNFα further activates the nuclear factor κ B (NFκB), a transcriptional factor that regulates neuronal survival and the transcription rate of other cytokines, that will convey on insulin signaling impairment (79, 80). The NAc of HFD mice have reduced insulin signaling and increased expression of TNFα. Interestingly, both measures plus depressive-like behavior were counteracted by the administration of probiotics (81).
Depressive-like behavior was also reported in these models and it was dependent of TNFα signaling and activation of microglial Toll-like receptor 4 (TLR4) (82). Interestingly, TLR4 expression correlates with depression in humans (83, 84) and followed anxiety and depressive-like behavior in mice fed a high-cholesterol diet (85). Since this receptor can be activated by saturated fatty acids (86),which are associated with higher risk of developing T2D (87), a pathway involving TLR4, TNFα, and insulin resistance could be a mechanistic link between T2D and depression yet to be explored (Figure 1).
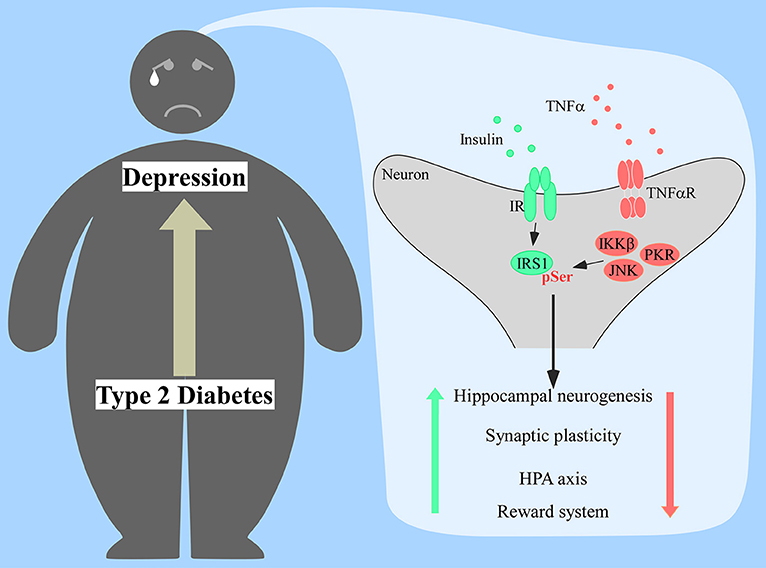
Figure 1. Proposed mechanism of insulin resistance in the brain of diabetic patients prompting the onset of depression. Increased production of tumor necrosis factor α (TNFα) would activate several stress kinases in the brain, including IκB kinase β (IKKβ), c-Jun N-terminal kinase (JNK), and protein kinase RNA-activated (PKR). Activation of those stress pathways leads to the phosphorylation of insulin receptor substrate 1 (IRS1) at serine residues, impacting proper insulin signaling response. Lack of proper central insulin signaling would affect hippocampal neurogenesis, synaptic plasticity, hypothalamic-pituitary-adrenal (HPA) axis response, and the reward system.
Antidepressant Aspects of Antidiabetic Drugs
Due to the heterogeneity of depression and the lack of specific biomarkers, the management of this condition in the primary care setting remains challenging (88, 89) and treatment frequently involves trial and error experimentation. Different types of antidepressants are applied in clinical practices, usually directed to neurotransmitters reuptake and monoamine oxidase inhibition. However, response rates to treatments remain low, with more than 30% of patients with depressive disorders failing to respond to four different antidepressant therapies (90). It is not uncommon to observe cases of treatment-resistant depression despite adequate dosing and duration of antidepressants (91). More effective treatments are required, and some overlapping mechanisms between T2D and depression, suggested above, opens up new avenues for the identification of novel pharmacological targets for the treatment of those comorbid disorders. Regarding this topic, anti-depressant effects of anti-diabetic and anti-inflammatory medications are currently being explored in the field.
Insulin based medications can be considered first-line treatments in T2D in cases of severe basal hyperglycemia or elevated serum glycated hemoglobin. Clinical studies administering intranasal insulin in healthy human subjects have reported improvements in mood and memory (92), as well as better HPA axis response to a social stress test, assessed by decreased saliva and plasma cortisol levels (93). But when evaluated in a cohort of depressive patients, intranasal insulin had no improvements on the depressive scores and neurocognition indexes applied compared to the placebo group (94). Lack of effective response to insulin amongst patients could be related to an intracellular insulin resistance (Figure 1), suggesting that approaches that bypass the first steps of the insulin signaling might show better results.
Liraglutide, another anti-diabetic medication, is an incretin analog that binds to the Glucagon-like peptide 1 receptor and ameliorates insulin signaling. This injectable anti-diabetic medication is capable of crossing the blood-brain barrier (95) and shows positive effects on brain insulin signaling and memory performance on animal models of AD (78, 96, 97). When tested in animal models of depression, liraglutide also had beneficial effects (98, 99). Clinical trials using this drug as a treatment for neurodegenerative diseases, like Alzheimer's disease are ongoing (ClinicalTrials.gov Identifiers: NCT01843075; NCT01469351; NCT02140983). A 4-week pilot study adding liraglutide as a treatment for patients with mood disorders observed better scores on measures of cognitive function compared to baseline (100). But more robust trials involving liraglutide and a placebo group in depressive patients are still warranted.
Metformin is a commonly used treatment for T2D with mechanisms of action not fully understood, but it involves key regulators of cellular energy status, including mitochondrial proteins and the AMP kinase (AMPK) (101, 102). In a cohort of patients with comorbid depression and T2D, metformin was reported to ameliorate depressive behavior when compared to baseline (103). Nonetheless, in a study involving overweight participants with impaired glucose tolerance, metformin had no effect on the Beck Depression Inventory score when compared to the placebo group (104). The effects of metformin in the antidepressant response to sertraline in a group of obese people is currently being evaluated in a phase 4 clinical trial (ClinicalTrials.gov Identifier: NCT00834652).
PPARγ receptor agonists such as thiazolidinediones enhances insulin sensitivity and are used as anti-diabetic drugs (105). Rosiglitazone administration provides antidepressant-like effects in mouse models of depression and T2D (31, 106). Pioglitazone has been evaluated in several clinical trials involving depressed patients and analysis indicated that the drug was more effective in patients with insulin resistance (107–110). Interestingly, pioglitazone effectiveness was age-dependent, being more efficacious in younger subjects (107).
Recent studies have also shown that immunosuppressive agents can improve outcomes in depression. Etanercept and infliximab neutralize TNFα and are currently being used in the treatment of auto-immune disorders (111). In regard to brain diseases, pilot studies administering TNFα inhibitors via intrathecal and perispinal routes had beneficial effects on cognitive measures in AD patients (112–114). But, when delivery subcuteously, etanercept had no beneficial effects on cognition (115), possibly due to restricted transport across the blood-brain barrier (116). A randomized double-blind placebo-controlled trial using an indwelling catheter to deliver infliximab in depressed patients showed no significant changes in Hamilton-Depression (HAM-D) score when compared with the placebo group. However, in patients with a higher inflammatory state, indicated by serum C-Reactive protein (CRP) concentration higher than 5 mg/L, infliximab improved HAM-D scores compared to placebo (117). Since the treatment decreased circulating CRP levels within the responder vs. the non-responder group, and the limitation of infliximab to reach the brain, the beneficial effect of the drug might have been driven by an overall decrease of peripheral inflammatory markers.
Finally, changes in the gut microbiota are associated with stress disorders (118). Probiotics can modulate the HPA axis (119), neurotransmitters (120), inflammatory markers and, as previously mentioned, insulin signaling in the brain (81). Probiotics are emerging as promising treatments for depression, showing positive results in different clinical studies [for a systematic review see (121)].
Conclusion
The association between depression and diabetes is supported by several evidences, but the mechanistic links between both diseases are still emerging. The development of brain insulin resistance is a possible candidate connecting both diseases, but further studies focusing on this issue are warranted in the field. Unraveling this connection is a matter of a great value in order to pursue alternative treatments or to optimize anti-depressants response.
Author Contributions
NL: development of the subject matter, drafting of the article, conception, and design of the figure, critical revision of the article, final approval of the version to be published; ML: development of the subject matter, drafting of the article, critical revision of the article, final approval of the version to be published; CS and DM: critical revision of the article, final approval of the version to be published; RM and FD: development of the subject matter, drafting of the article, critical revision of the article, final approval of the version to be published.
Funding
Work in the authors' laboratory is funded by grants from Conselho Nacional de Desenvolvimento Científico e Tecnológico (CNPq), Fundação de Amparo à Pesquisa do Estado do Rio de Janeiro (FAPERJ), Alzheimer Society of Canada, National Institute for Translational Neuroscience (to FD), Canada Research Chair Program, and Canadian Institutes of Health Research (to DM).
Conflict of Interest Statement
The authors declare that the research was conducted in the absence of any commercial or financial relationships that could be construed as a potential conflict of interest.
References
1. Global Burden of Disease Study C. Global, regional, and national incidence, prevalence, and years lived with disability for 301 acute and chronic diseases and injuries in 188 countries, 1990-2013: a systematic analysis for the Global Burden of Disease Study 2013. Lancet (2015) 386:743–800. doi: 10.1016/S0140-6736(15)60692-4
3. Shepherd PR, Kahn BB. Glucose transporters and insulin action–implications for insulin resistance and diabetes mellitus. N Engl J Med. (1999) 341:248–57. doi: 10.1056/NEJM199907223410406
4. National Institute of Mental Health. Depression Basics. (2016). Available online at: https://www.nimh.nih.gov/health/publications/depression/index.shtml. (Accessed February 01, 2019).
5. Ott A, Stolk RP, van Harskamp F, Pols HA, Hofman A, Breteler MM. Diabetes mellitus and the risk of dementia: the rotterdam study. Neurology (1999) 53:1937–42.
6. Svenningsson I, Bjorkelund C, Marklund B, Gedda B. Anxiety and depression in obese and normal-weight individuals with diabetes type 2: a gender perspective. Scand J Caring Sci. (2012) 26:349–54. doi: 10.1111/j.1471-6712.2011.00940.x
7. Ali S, Stone MA, Peters JL, Davies MJ, Khunti K. The prevalence of co-morbid depression in adults with Type 2 diabetes: a systematic review and meta-analysis. Diabetic Med. (2006) 23:1165–73. doi: 10.1111/j.1464-5491.2006.01943.x
8. Eaton WW, Armenian H, Gallo J, Pratt L, Ford DE. Depression and risk for onset of type II diabetes. A prospective population-based study. Diabetes Care (1996) 19:1097–102.
9. Simon GE, Von Korff M, Saunders K, Miglioretti DL, Crane PK, van Belle G, et al. Association between obesity and psychiatric disorders in the US adult population. Arch General Psychiatry (2006) 63:824–30. doi: 10.1001/archpsyc.63.7.824
10. Mezuk B, Eaton WW, Albrecht S, Golden SH. Depression and type 2 diabetes over the lifespan: a meta-analysis. Diabetes Care (2008) 31:2383–90. doi: 10.2337/dc08-0985
11. Nouwen A, Lloyd CE, Pouwer F. Depression and type 2 diabetes over the lifespan: a meta-analysis. Response to Mezuk et al. Diabetes Care (2009) 32:e56 doi: 10.2337/dc09-0027
12. Bomfim TR, Forny-Germano L, Sathler LB, Brito-Moreira J, Houzel JC, Decker H, et al. An anti-diabetes agent protects the mouse brain from defective insulin signaling caused by Alzheimer's disease- associated Abeta oligomers. J Clin Invest. (2012) 122:1339–53. doi: 10.1172/JCI57256
13. Talbot K, Wang HY, Kazi H, Han LY, Bakshi KP, Stucky A, et al. Demonstrated brain insulin resistance in Alzheimer's disease patients is associated with IGF-1 resistance, IRS-1 dysregulation, and cognitive decline. J Clin Invest. (2012) 122:1316–38. doi: 10.1172/JCI59903
14. Rasgon NL, McEwen BS. Insulin resistance-a missing link no more. Mol Psychiatry (2016) 21:1648–52. doi: 10.1038/mp.2016.162
15. Shomaker LB, Tanofsky-Kraff M, Stern EA, Miller R, Zocca JM, Field SE, et al. Longitudinal study of depressive symptoms and progression of insulin resistance in youth at risk for adult obesity. Diabetes Care (2011) 34:2458–63. doi: 10.2337/dc11-1131
16. Singh MK, Leslie SM, Packer MM, Zaiko YV, Phillips OR, Weisman EF, et al. Brain and behavioral correlates of insulin resistance in youth with depression and obesity. Hormones Behav. (2018) 17:30501–9. doi: 10.1016/j.yhbeh.2018.03.009
17. Bruning JC, Gautam D, Burks DJ, Gillette J, Schubert M, Orban PC, et al. Role of brain insulin receptor in control of body weight and reproduction. Science (2000) 289:2122–5. doi: 10.1126/science.289.5487.2122
18. De Felice FG, Benedict C. A key role of insulin receptors in memory. Diabetes (2015) 64:3653–5. doi: 10.2337/dbi15-0011
19. Grillo CA, Piroli GG, Lawrence RC, Wrighten SA, Green AJ, Wilson SP, et al. Hippocampal insulin resistance impairs spatial learning and synaptic plasticity. Diabetes (2015) 64:3927–36. doi: 10.2337/db15-0596
20. Figlewicz DP, Evans SB, Murphy J, Hoen M, Baskin DG. Expression of receptors for insulin and leptin in the ventral tegmental area/substantia nigra (VTA/SN) of the rat. Brain Res. (2003) 964:107–15. doi: 10.1016/S0006-8993(02)04087-8
21. Woods CA, Guttman ZR, Huang D, Kolaric RA, Rabinowitsch AI, Jones KT, et al. Insulin receptor activation in the nucleus accumbens reflects nutritive value of a recently ingested meal. Physiol Behav. (2016) 159:52–63. doi: 10.1016/j.physbeh.2016.03.013
22. Grillo CA, Piroli GG, Kaigler KF, Wilson SP, Wilson MA, Reagan LP. Downregulation of hypothalamic insulin receptor expression elicits depressive-like behaviors in rats. Behav Brain Res. (2011) 222:230–5. doi: 10.1016/j.bbr.2011.03.052
23. Kleinridders A, Cai W, Cappellucci L, Ghazarian A, Collins WR, Vienberg SG, et al. Insulin resistance in brain alters dopamine turnover and causes behavioral disorders. Proc Natl Acad Sci USA. (2015) 112:3463–8. doi: 10.1073/pnas.1500877112
24. Cai W, Xue C, Sakaguchi M, Konishi M, Shirazian A, Ferris HA, et al. Insulin regulates astrocyte gliotransmission and modulates behavior. J Clin Invest. (2018) 128:2914–26. doi: 10.1172/JCI99366
25. Mansur RB, Fries GR, Subramaniapillai M, Frangou S, De Felice FG, Rasgon N, et al. Expression of dopamine signaling genes in the post-mortem brain of individuals with mental illnesses is moderated by body mass index and mediated by insulin signaling genes. J Psychiatr Res. (2018) 107:128–35. doi: 10.1016/j.jpsychires.2018.10.020
26. Evans MC, Kumar NS, Inglis MA, Anderson GM. Leptin and insulin do not exert redundant control of metabolic or emotive function via dopamine neurons. Hormones Behav. (2018) 106:93–104. doi: 10.1016/j.yhbeh.2018.10.001
27. Gray SM, Aylor KW, Barrett EJ. Unravelling the regulation of insulin transport across the brain endothelial cell. Diabetologia (2017) 60:1512–21. doi: 10.1007/s00125-017-4285-4
28. Son SM, Song H, Byun J, Park KS, Jang HC, Park YJ, et al. Altered APP processing in insulin-resistant conditions is mediated by autophagosome accumulation via the inhibition of mammalian target of rapamycin pathway. Diabetes (2012) 61:3126–38. doi: 10.2337/db11-1735
29. Senador D, Kanakamedala K, Irigoyen MC, Morris M, Elased KM. Cardiovascular and autonomic phenotype of db/db diabetic mice. Exp Physiol. (2009) 94:648–58. doi: 10.1113/expphysiol.2008.046474
30. Sharma AN, Elased KM, Garrett TL, Lucot JB. Neurobehavioral deficits in db/db diabetic mice. Physiol Behav. (2010) 101:381–8. doi: 10.1016/j.physbeh.2010.07.002
31. Sharma AN, Elased KM, Lucot JB. Rosiglitazone treatment reversed depression- but not psychosis-like behavior of db/db diabetic mice. J Psychopharmacol. (2012) 26:724–32. doi: 10.1177/0269881111434620
32. Sharma S, Fulton S. Diet-induced obesity promotes depressive-like behaviour that is associated with neural adaptations in brain reward circuitry. Int J Obes. (2013) 37:382–9. doi: 10.1038/ijo.2012.48
33. Hill AS, Sahay A, Hen R. Increasing adult hippocampal neurogenesis is sufficient to reduce anxiety and depression-like behaviors. Neuropsychopharmacology (2015) 40:2368–78. doi: 10.1038/npp.2015.85
34. Lindqvist A, Mohapel P, Bouter B, Frielingsdorf H, Pizzo D, Brundin P, et al. High-fat diet impairs hippocampal neurogenesis in male rats. Eur J Neurol. (2006) 13:1385–8. doi: 10.1111/j.1468-1331.2006.01500.x
35. Papazoglou IK, Jean A, Gertler A, Taouis M, Vacher CM. Hippocampal GSK3beta as a molecular link between obesity and depression. Mol Neurobiol. (2015) 52:363–74. doi: 10.1007/s12035-014-8863-x
36. Garza JC, Guo M, Zhang W, Lu XY. Leptin increases adult hippocampal neurogenesis in vivo and in vitro. J Biol Chem. (2008) 283:18238–47. doi: 10.1074/jbc.M800053200
37. Garza JC, Guo M, Zhang W, Lu XY. Leptin restores adult hippocampal neurogenesis in a chronic unpredictable stress model of depression and reverses glucocorticoid-induced inhibition of GSK-3beta/beta-catenin signaling. Mol Psychiatry (2012) 17:790–808. doi: 10.1038/mp.2011.161
38. Trejo JL, Carro E, Torres-Aleman I. Circulating insulin-like growth factor I mediates exercise-induced increases in the number of new neurons in the adult hippocampus. J Neurosci. (2001) 21:1628–34. doi: 10.1523/JNEUROSCI.21-05-01628.2001
39. Zheng WH, Quirion R. Insulin-like growth factor-1 (IGF-1) induces the activation/phosphorylation of Akt kinase and cAMP response element-binding protein (CREB) by activating different signaling pathways in PC12 cells. BMC Neurosci. (2006) 7:51. doi: 10.1186/1471-2202-7-51
40. Gomes RJ, de Oliveira CA, Ribeiro C, Mota CS, Moura LP, Tognoli LM, et al. Effects of exercise training on hippocampus concentrations of insulin and IGF-1 in diabetic rats. Hippocampus (2009) 19:981–7. doi: 10.1002/hipo.20636
41. Mori H, Hanada R, Hanada T, Aki D, Mashima R, Nishinakamura H, et al. Socs3 deficiency in the brain elevates leptin sensitivity and confers resistance to diet-induced obesity. Nat Med. (2004) 10:739–43. doi: 10.1038/nm1071
42. Fujimaki S, Kuwabara T. Diabetes-induced dysfunction of mitochondria and stem cells in skeletal muscle and the nervous system. Int J Mol Sci. (2017) 18:E2147. doi: 10.3390/ijms18102147
43. Bonato JM, Bassani TB, Milani H, Vital M, de Oliveira RMW. Pioglitazone reduces mortality, prevents depressive-like behavior, and impacts hippocampal neurogenesis in the 6-OHDA model of Parkinson's disease in rats. Exp Neurol. (2018) 300:188–200. doi: 10.1016/j.expneurol.2017.11.009
44. Pipatpiboon N, Pratchayasakul W, Chattipakorn N, Chattipakorn SC. PPARgamma agonist improves neuronal insulin receptor function in hippocampus and brain mitochondria function in rats with insulin resistance induced by long term high-fat diets. Endocrinology (2012) 153:329–38. doi: 10.1210/en.2011-1502
45. Detka J, Kurek A, Basta-Kaim A, Kubera M, Lason W, Budziszewska B. Neuroendocrine link between stress, depression and diabetes. Pharmacol Reports (2013) 65:1591–600. doi: 10.1016/S1734-1140(13)71520-2
46. Ferrario CR, Reagan LP. Insulin-mediated synaptic plasticity in the CNS: Anatomical, functional and temporal contexts. Neuropharmacology (2018) 136(Pt B):182–91. doi: 10.1016/j.neuropharm.2017.12.001
47. Labouebe G, Liu S, Dias C, Zou H, Wong JC, Karunakaran S, et al. Insulin induces long-term depression of ventral tegmental area dopamine neurons via endocannabinoids. Nat Neurosci. (2013) 16:300–8. doi: 10.1038/nn.3321
48. Stouffer MA, Woods CA, Patel JC, Lee CR, Witkovsky P, Bao L, et al. Insulin enhances striatal dopamine release by activating cholinergic interneurons and thereby signals reward. Nat Commun. (2015) 6:8543. doi: 10.1038/ncomms9543
49. Faulenbach M, Uthoff H, Schwegler K, Spinas GA, Schmid C, Wiesli P. Effect of psychological stress on glucose control in patients with Type 2 diabetes. Diabetic Med. (2012) 29: 128-31. doi: 10.1111/j.1464-5491.2011.03431.x
50. Heraclides A, Chandola T, Witte DR, Brunner EJ. Psychosocial stress at work doubles the risk of type 2 diabetes in middle-aged women: evidence from the Whitehall II study. Diabetes Care (2009) 32:2230–5. doi: 10.2337/dc09-0132
51. Viseu J, Leal R, de Jesus SN, Pinto P, Pechorro P, Greenglass E. Relationship between economic stress factors and stress, anxiety, and depression: moderating role of social support. Psychiatry Res. (2018) 268:102–7. doi: 10.1016/j.psychres.2018.07.008
52. Aguilera G. HPA axis responsiveness to stress: implications for healthy aging. Exp Gerontol. (2011) 46:90–5. doi: 10.1016/j.exger.2010.08.023
53. Smith SM, Vale WW. The role of the hypothalamic-pituitary-adrenal axis in neuroendocrine responses to stress. Dialog Clin Neurosci. (2006) 8:383–95.
54. Carroll BJ, Cassidy F, Naftolowitz D, Tatham NE, Wilson WH, Iranmanesh A, et al. Pathophysiology of hypercortisolism in depression. Acta Psychiatr Scand Suppl. (2007) (433):90–103. doi: 10.1111/j.1600-0447.2007.00967.x
55. Oltmanns KM, Dodt B, Schultes B, Raspe HH, Schweiger U, Born J, et al. Cortisol correlates with metabolic disturbances in a population study of type 2 diabetic patients. Eur J Endocrinol. (2006) 154:325–31. doi: 10.1530/eje.1.02074
56. Chong AC, Vogt MC, Hill AS, Bruning JC, Zeltser LM. Central insulin signaling modulates hypothalamus-pituitary-adrenal axis responsiveness. Mol Metabol. (2015) 4:83–92. doi: 10.1016/j.molmet.2014.12.001
57. Jacobson L, Sapolsky R. The role of the hippocampus in feedback regulation of the hypothalamic-pituitary-adrenocortical axis. Endocr Rev. (1991) 12:118–34. doi: 10.1210/edrv-12-2-118
58. Lopez JF, Chalmers DT, Little KY, Watson SJ. A.E. Bennett Research Award. Regulation of serotonin1A, glucocorticoid, and mineralocorticoid receptor in rat and human hippocampus: implications for the neurobiology of depression. Biol Psychiatry (1998) 43:547–73.
59. Chan O, Chan S, Inouye K, Shum K, Matthews SG, Vranic M. Diabetes impairs hypothalamo-pituitary-adrenal (HPA) responses to hypoglycemia, and insulin treatment normalizes HPA but not epinephrine responses. Diabetes (2002) 51:1681–9. doi: 10.2337/diabetes.51.6.1681
60. Chan O, Inouye K, Akirav E, Park E, Riddell MC, Vranic M, et al. Insulin alone increases hypothalamo-pituitary-adrenal activity, and diabetes lowers peak stress responses. Endocrinology (2005) 146:1382–90. doi: 10.1210/en.2004-0607
61. Mirza S, Hossain M, Mathews C, Martinez P, Pino P, Gay JL, et al. Type 2-diabetes is associated with elevated levels of TNF-alpha, IL-6 and adiponectin and low levels of leptin in a population of Mexican Americans: a cross-sectional study. Cytokine (2012) 57:136–42. doi: 10.1016/j.cyto.2011.09.029
62. Johnson LA, Edwards M, Gamboa A, Hall J, Robinson M, O'Bryant SE. Depression, inflammation, and memory loss among Mexican Americans: analysis of the HABLE cohort. Int Psychogeriatr. (2017) 29:1693–9. doi: 10.1017/S1041610217001016
63. Postal M, Lapa AT, Sinicato NA, de Oliveira Pelicari K, Peres FA, Costallat LT, et al. Depressive symptoms are associated with tumor necrosis factor alpha in systemic lupus erythematosus. J Neuroinflamm. (2016) 13:5. doi: 10.1186/s12974-015-0471-9
64. Himmerich H, Fulda S, Linseisen J, Seiler H, Wolfram G, Himmerich S, et al. Depression, comorbidities and the TNF-alpha system. Eur Psychiatry (2008) 23:421–9. doi: 10.1016/j.eurpsy.2008.03.013
65. Kaster MP, Gadotti VM, Calixto JB, Santos AR, Rodrigues AL. Depressive-like behavior induced by tumor necrosis factor-alpha in mice. Neuropharmacology (2012) 62:419–26. doi: 10.1016/j.neuropharm.2011.08.018
66. Morgan JA, Singhal G, Corrigan F, Jaehne EJ, Jawahar MC, Baune BT. Exercise related anxiety-like behaviours are mediated by TNF receptor signaling, but not depression-like behaviours. Brain Res. (2018) 1695:10–7. doi: 10.1016/j.brainres.2018.05.032
67. Popov A, Abdullah Z, Wickenhauser C, Saric T, Driesen J, Hanisch FG, et al. Indoleamine 2,3-dioxygenase-expressing dendritic cells form suppurative granulomas following Listeria monocytogenes infection. J Clin Invest. (2006) 116: 3160–70. doi: 10.1172/JCI28996
68. Zhu CB, Blakely RD, Hewlett WA. The proinflammatory cytokines interleukin-1beta and tumor necrosis factor-alpha activate serotonin transporters. Neuropsychopharmacology (2006) 31:2121–31. doi: 10.1038/sj.npp.1301029
69. Cheng Y, Desse S, Martinez A, Worthen RJ, Jope RS, Beurel E. TNFalpha disrupts blood brain barrier integrity to maintain prolonged depressive-like behavior in mice. Brain Behav Immun. (2018) 69:556–67. doi: 10.1016/j.bbi.2018.02.003
70. Starr JM, Wardlaw J, Ferguson K, MacLullich A, Deary IJ, Marshall I. Increased blood-brain barrier permeability in type II diabetes demonstrated by gadolinium magnetic resonance imaging. J Neurol Neurosurg Psychiatry (2003) 74:70–6. doi: 10.1136/jnnp.74.1.70
71. Felger JC, Haroon E, Woolwine BJ, Raison CL, Miller AH. Interferon-alpha-induced inflammation is associated with reduced glucocorticoid negative feedback sensitivity and depression in patients with hepatitis C virus. Physiol Behav. (2016) 166:14–21. doi: 10.1016/j.physbeh.2015.12.013
72. Pham GS, Mathis KW. Lipopolysaccharide challenge reveals hypothalamic-pituitary-adrenal axis dysfunction in murine systemic lupus erythematosus. Brain Sci. (2018) 8: E184. doi: 10.3390/brainsci8100184
73. Bugajski J, Gadek-Michalska A, Borycz J, Glod R, Olowska A. The role of prostaglandins and the hypothalamic and hippocampal histamine in the clonidine-induced pituitary-adrenocortical response. J Physiol Pharmacol. (1996) 47:487–95.
74. Chan PC, Wu TN, Chen YC, Lu CH, Wabitsch M, Tian YF, et al. Targeted inhibition of CD74 attenuates adipose COX-2-MIF-mediated M1 macrophage polarization and retards obesity-related adipose tissue inflammation and insulin resistance. Clin Sci. (2018) 132:1581–96. doi: 10.1042/CS20180041
75. Clarke JR, Lyra ESNM, Figueiredo CP, Frozza RL, Ledo JH, Beckman D, et al. Alzheimer-associated Abeta oligomers impact the central nervous system to induce peripheral metabolic deregulation. EMBO Mol Med. (2015) 7:190–210. doi: 10.15252/emmm.201404183
76. De Felice FG, Ferreira ST. Inflammation, defective insulin signaling, and mitochondrial dysfunction as common molecular denominators connecting type 2 diabetes to Alzheimer disease. Diabetes (2014) 63:2262–72. doi: 10.2337/db13-1954
77. Hotamisligil GS, Peraldi P, Budavari A, Ellis R, White MF, Spiegelman BM. IRS-1-mediated inhibition of insulin receptor tyrosine kinase activity in TNF-alpha- and obesity-induced insulin resistance. Science (1996) 271:665–8.
78. Lourenco MV, Clarke JR, Frozza RL, Bomfim TR, Forny-Germano L, Batista AF, et al. TNF-alpha mediates PKR-dependent memory impairment and brain IRS-1 inhibition induced by Alzheimer's beta-amyloid oligomers in mice and monkeys. Cell Metabol. (2013) 18: 831–43. doi: 10.1016/j.cmet.2013.11.002
79. Dong J, Jimi E, Zeiss C, Hayden MS, Ghosh S. Constitutively active NF-kappaB triggers systemic TNFalpha-dependent inflammation and localized TNFalpha-independent inflammatory disease. Genes Dev. (2010) 24:1709–17. doi: 10.1101/gad.1958410
80. Gupta S, Bi R, Kim C, Chiplunkar S, Yel L, Gollapudi S. Role of NF-kappaB signaling pathway in increased tumor necrosis factor-alpha-induced apoptosis of lymphocytes in aged humans. Cell Death Differ. (2005) 12:177–83. doi: 10.1038/sj.cdd.4401557
81. Soto M, Herzog C, Pacheco JA, Fujisaka S, Bullock K, Clish CB, et al. Gut microbiota modulate neurobehavior through changes in brain insulin sensitivity and metabolism. Mol Psychiatry (2018) 23:2287–301. doi: 10.1038/s41380-018-0086-5
82. Ledo JH, Azevedo EP, Beckman D, Ribeiro FC, Santos LE, Razolli D, et al. Crosstalk between brain innate immunity and serotonin signaling underlies depressive-like behavior induced by Alzheimer's amyloid-β oligomers in mice. J Neurosci. (2016) 36:12106–16. doi: 10.1523/JNEUROSCI.1269-16.2016
83. Hung YY, Kang HY, Huang KW, Huang TL. Association between toll-like receptors expression and major depressive disorder. Psychiatry Res. (2014) 220:283–6. doi: 10.1016/j.psychres.2014.07.074
84. Wu MK, Huang TL, Huang KW, Huang YL, Hung YY. Association between toll-like receptor 4 expression and symptoms of major depressive disorder. Neuropsychiatr Dis Treat. (2015) 11:1853–7. doi: 10.2147/NDT.S88430
85. Strekalova T, Evans M, Costa-Nunes J, Bachurin S, Yeritsyan N, Couch Y, et al. Tlr4 upregulation in the brain accompanies depression- and anxiety-like behaviors induced by a high-cholesterol diet. Brain Behav Immun. (2015) 48:42–7. doi: 10.1016/j.bbi.2015.02.015
86. Milanski M, Degasperi G, Coope A, Morari J, Denis R, Cintra DE, et al. Saturated fatty acids produce an inflammatory response predominantly through the activation of TLR4 signaling in hypothalamus: implications for the pathogenesis of obesity. J Neurosci. (2009) 29:359–70. doi: 10.1523/JNEUROSCI.2760-08.2009
87. Luukkonen PK, Sadevirta S, Zhou Y, Kayser B, Ali A, Ahonen L, et al. Saturated fat is more metabolically harmful for the human liver than unsaturated fat or simple sugars. Diabetes Care (2018) 41:1732–9. doi: 10.2337/dc18-0071
88. Coyne JC, Schwenk TL, Fechner-Bates S. Nondetection of depression by primary care physicians reconsidered. General Hosp Psychiatry (1995) 17:3–12.
89. Greer J, Halgin R, Harvey E. Global versus specific symptom attributions: predicting the recognition and treatment of psychological distress in primary care. J Psychosomat Res. (2004) 57:521–7. doi: 10.1016/j.jpsychores.2004.02.020
90. Rush AJ, Trivedi MH, Wisniewski SR, Nierenberg AA, Stewart JW, Warden D, et al. Acute and longer-term outcomes in depressed outpatients requiring one or several treatment steps: a STAR*D report. Am J Psychiatry (2006) 163:1905–17. doi: 10.1176/ajp.2006.163.11.1905
91. Thomas SJ, Shin M, McInnis MG, Bostwick JR. Combination therapy with monoamine oxidase inhibitors and other antidepressants or stimulants: strategies for the management of treatment-resistant depression. Pharmacotherapy (2015) 35:433–49. doi: 10.1002/phar.1576
92. Benedict C, Hallschmid M, Hatke A, Schultes B, Fehm HL, Born J, et al. Intranasal insulin improves memory in humans. Psychoneuroendocrinology (2004) 29:1326–34. doi: 10.1016/j.psyneuen.2004.04.003
93. Bohringer A, Schwabe L, Richter S, Schachinger H. Intranasal insulin attenuates the hypothalamic-pituitary-adrenal axis response to psychosocial stress. Psychoneuroendocrinology (2008) 33:1394–400. doi: 10.1016/j.psyneuen.2008.08.002
94. Cha DS, Best MW, Bowie CR, Gallaugher LA, Woldeyohannes HO, Soczynska JK, et al. A randomized, double-blind, placebo-controlled, crossover trial evaluating the effect of intranasal insulin on cognition and mood in individuals with treatment-resistant major depressive disorder. J Affect Dis. (2017) 210:57–65. doi: 10.1016/j.jad.2016.12.006
95. Duarte AI, Candeias E, Correia SC, Santos RX, Carvalho C, Cardoso S, et al. Crosstalk between diabetes and brain: glucagon-like peptide-1 mimetics as a promising therapy against neurodegeneration. Biochim Biophys Acta (2013) 1832:527–41. doi: 10.1016/j.bbadis.2013.01.008
96. Batista AF, Forny-Germano L, Clarke JR, Lyra ESNM, Brito-Moreira J, Boehnke SE, et al. The diabetes drug liraglutide reverses cognitive impairment in mice and attenuates insulin receptor and synaptic pathology in a non-human primate model of Alzheimer's disease. J Pathol. (2018) 245:85–100. doi: 10.1002/path.5056
97. McClean PL, Parthsarathy V, Faivre E, Holscher C. The diabetes drug liraglutide prevents degenerative processes in a mouse model of Alzheimer's disease. J Neurosci. (2011) 31:6587–94. doi: 10.1523/JNEUROSCI.0529-11.2011
98. Sharma AN, Ligade SS, Sharma JN, Shukla P, Elased KM, Lucot JB. GLP-1 receptor agonist liraglutide reverses long-term atypical antipsychotic treatment associated behavioral depression and metabolic abnormalities in rats. Metabol Brain Dis. (2015) 30:519–27. doi: 10.1007/s11011-014-9591-7
99. Weina H, Yuhu N, Christian H, Birong L, Feiyu S, Le W. Liraglutide attenuates the depressive- and anxiety-like behaviour in the corticosterone induced depression model via improving hippocampal neural plasticity. Brain Res. (2018) 1694:55–62. doi: 10.1016/j.brainres.2018.04.031
100. Mansur RB, Ahmed J, Cha DS, Woldeyohannes HO, Subramaniapillai M, Lovshin J, et al. Liraglutide promotes improvements in objective measures of cognitive dysfunction in individuals with mood disorders: a pilot, open-label study. J Affect Disorder. (2017) 207:114–20. doi: 10.1016/j.jad.2016.09.056
101. Madiraju AK, Erion DM, Rahimi Y, Zhang XM, Braddock DT, Albright RA, et al. Metformin suppresses gluconeogenesis by inhibiting mitochondrial glycerophosphate dehydrogenase. Nature (2014) 510:542–6. doi: 10.1038/nature13270
102. Zhou G, Myers R, Li Y, Chen Y, Shen X, Fenyk-Melody J, et al. Role of AMP-activated protein kinase in mechanism of metformin action. J Clin Invest. (2001) 108:1167–74. doi: 10.1172/JCI13505
103. Guo M, Mi J, Jiang QM, Xu JM, Tang YY, Tian G, et al. Metformin may produce antidepressant effects through improvement of cognitive function among depressed patients with diabetes mellitus. Clin Exp Pharmacol Physiol. (2014) 41:650–6. doi: 10.1111/1440-1681.12265
104. Ackermann RT, Edelstein SL, Narayan KM, Zhang P, Engelgau MM, Herman WH, et al. Changes in health state utilities with changes in body mass in the diabetes prevention program. Obesity (2009) 17:2176–81. doi: 10.1038/oby.2009.114
105. Nam JS, Nam JY, Yoo JS, Cho M, Park JS, Ahn CW, et al. The effect of rosiglitazone on insulin sensitivity and mid-thigh low-density muscle in patients with Type 2 diabetes. Diabetic Med. (2010) 27:30–6. doi: 10.1111/j.1464-5491.2009.02897.x
106. Cheng Y, Rodriguiz RM, Murthy SR, Senatorov V, Thouennon E, Cawley NX, et al. Neurotrophic factor-alpha1 prevents stress-induced depression through enhancement of neurogenesis and is activated by rosiglitazone. Mol Psychiatry (2015) 20:744–54. doi: 10.1038/mp.2014.136
107. Lin KW, Wroolie TE, Robakis T, Rasgon NL. Adjuvant pioglitazone for unremitted depression: clinical correlates of treatment response. Psychiatry Res. (2015) 230:846–52. doi: 10.1016/j.psychres.2015.10.013
108. Roohafza H, Shokouh P, Sadeghi M, Alikhassy Z, Sarrafzadegan N. A possible role for pioglitazone in the management of depressive symptoms in metabolic syndrome patients (EPICAMP Study): a double blind, randomized clinical trial. Int Scholar Res Notices (2014) 2014:697617. doi: 10.1155/2014/697617
109. Sepanjnia K, Modabbernia A, Ashrafi M, Modabbernia MJ, Akhondzadeh S. Pioglitazone adjunctive therapy for moderate-to-severe major depressive disorder: randomized double-blind placebo-controlled trial. Neuropsychopharmacology (2012) 37:2093–100. doi: 10.1038/npp.2012.58
110. Zeinoddini A, Sorayani M, Hassanzadeh E, Arbabi M, Farokhnia M, Salimi S, et al. Pioglitazone adjunctive therapy for depressive episode of bipolar disorder: a randomized, double-blind, placebo-controlled trial. Depress Anxiety (2015) 32:167–73. doi: 10.1002/da.22340
111. Lai Y, Dong C. Therapeutic antibodies that target inflammatory cytokines in autoimmune diseases. Int Immunol. (2016) 28:181–8. doi: 10.1093/intimm/dxv063
112. Shi JQ, Wang BR, Jiang WW, Chen J, Zhu YW, Zhong LL, et al. Cognitive improvement with intrathecal administration of infliximab in a woman with Alzheimer's disease. J Am Geriatr Soc. (2011) 59:1142–4. doi: 10.1111/j.1532-5415.2011.03445.x
113. Tobinick E, Gross H, Weinberger A, Cohen H. TNF-alpha modulation for treatment of Alzheimer's disease: a 6-month pilot study. Med Gen Med. (2006) 8:25.
114. Tobinick EL, Gross H. Rapid cognitive improvement in Alzheimer's disease following perispinal etanercept administration. J Neuroinflamm. (2008) 5:2. doi: 10.1186/1742-2094-5-2
115. Butchart J, Brook L, Hopkins V, Teeling J, Puntener U, Culliford D, et al. Etanercept in Alzheimer disease: A randomized, placebo-controlled, double-blind, phase 2 trial. Neurology (2015) 84:2161–8. doi: 10.1212/WNL.0000000000001617
116. Boado RJ, Hui EK, Lu JZ, Zhou QH, Pardridge WM. Selective targeting of a TNFR decoy receptor pharmaceutical to the primate brain as a receptor-specific IgG fusion protein. J Biotechnol. (2010) 146:84–91. doi: 10.1016/j.jbiotec.2010.01.011
117. Raison CL, Rutherford RE, Woolwine BJ, Shuo C, Schettler P, Drake DF, et al. A randomized controlled trial of the tumor necrosis factor antagonist infliximab for treatment-resistant depression: the role of baseline inflammatory biomarkers. JAMA Psychiatry (2013) 70:31–41. doi: 10.1001/2013.jamapsychiatry.4
118. O'Mahony SM, Marchesi JR, Scully P, Codling C, Ceolho AM, Quigley EM, et al. Early life stress alters behavior, immunity, and microbiota in rats: implications for irritable bowel syndrome and psychiatric illnesses. Biol Psychiatry (2009) 65:263–7. doi: 10.1016/j.biopsych.2008.06.026
119. Ait-Belgnaoui A, Durand H, Cartier C, Chaumaz G, Eutamene H, Ferrier L, et al. Prevention of gut leakiness by a probiotic treatment leads to attenuated HPA response to an acute psychological stress in rats. Psychoneuroendocrinology (2012) 37:1885–95. doi: 10.1016/j.psyneuen.2012.03.024
120. Pandey S, Singh A, Chaudhari N, Nampoothiri LP, Kumar GN. Protection against 1,2-di-methylhydrazine-induced systemic oxidative stress and altered brain neurotransmitter status by probiotic Escherichia coli CFR 16 secreting pyrroloquinoline quinone. Curr Microbiol. (2015) 70: 690–7. doi: 10.1007/s00284-014-0763-9
Keywords: depression, type 2 diabetes, insulin resistance, inflammation, synaptic plasticity, hippocampus, HPA axis, dopamine
Citation: Lyra e Silva NM, Lam MP, Soares CN, Munoz DP, Milev R and De Felice FG (2019) Insulin Resistance as a Shared Pathogenic Mechanism Between Depression and Type 2 Diabetes. Front. Psychiatry 10:57. doi: 10.3389/fpsyt.2019.00057
Received: 21 August 2018; Accepted: 25 January 2019;
Published: 14 February 2019.
Edited by:
Tomas Hajek, Dalhousie University, CanadaReviewed by:
Jess Gerard Fiedorowicz, The University of Iowa, United StatesTamas Kozicz, Mayo Clinic, United States
Copyright © 2019 Lyra e Silva, Lam, Soares, Munoz, Milev and De Felice. This is an open-access article distributed under the terms of the Creative Commons Attribution License (CC BY). The use, distribution or reproduction in other forums is permitted, provided the original author(s) and the copyright owner(s) are credited and that the original publication in this journal is cited, in accordance with accepted academic practice. No use, distribution or reproduction is permitted which does not comply with these terms.
*Correspondence: Fernanda G. De Felice, ZmVsaWNlQGJpb3FtZWQudWZyai5icg==; ZmVybmFuZGEuZGVmZWxpY2VAcXVlZW5zdS5jYQ==
†These authors have contributed equally to this work