- 1Department of Psychiatry, Icahn School of Medicine at Mount Sinai, New York, NY, USA
- 2Nathan S. Kline Institute for Psychiatric Research, Orangeburg, NY, USA
Major depressive disorder (MDD) during adolescence is a common and disabling psychiatric condition; yet, little is known about its neurobiological underpinning. Evidence indicates that MDD in adults involves alterations in white and gray matter; however, sparse research has focused on adolescent MDD. Similarly, little research has accounted for the wide variability of symptom severity among depressed teens. Here, we aimed to investigate white matter (WM) microstructure between 17 adolescents with MDD and 16 matched healthy controls (HC) using diffusion tensor imaging. We further assessed within the MDD group relationships between WM integrity and depression severity, as well as anhedonia and irritability – two core symptoms of adolescent MDD. As expected, adolescents with MDD manifested decreased WM integrity compared to HC in the anterior cingulum and anterior corona radiata. Within the MDD group, greater depression severity was correlated with reduced WM integrity in the genu of corpus callosum, anterior thalamic radiation, anterior cingulum, and sagittal stratum. However, anhedonia and irritability were associated with alterations in distinct WM tracts. Specifically, anhedonia was associated with disturbances in tracts related to reward processing, including the anterior limb of the internal capsule and projection fibers to the orbitofrontal cortex. Irritability was associated with decreased integrity in the sagittal stratum, anterior corona radiata, and tracts leading to prefrontal and temporal cortices. Overall, these preliminary findings provide further support for the hypotheses that there is a disconnect between prefrontal and limbic emotional regions in depression, and that specific clinical symptoms involve distinct alterations in WM tracts.
Introduction
Major depressive disorder (MDD) in adolescence is a prevalent and disabling psychiatric illness associated with serious consequences including academic failure, social withdrawal, substance abuse, and most critically, suicide (1–5). Converging evidence derived from neuroimaging studies suggests that adolescent MDD entails morphological, functional, and neurochemical alterations (6–10). Importantly, since adolescence represents a critical period of rapid neuroplasticity [e.g., increased myelination, synaptic pruning; (11–13)], white matter (WM) alterations can contribute to the neurobiology of adolescent MDD. Indeed, in our prior investigation of gamma-Aminobutyric acid in the anterior cingulate cortex (ACC), we incidentally found reduced WM volume in adolescents with MDD compared to healthy controls [HC; (9)]. However, there has been sparse research focusing on WM alterations in this age group.
Diffusion tensor imaging (DTI) enables the non-invasive examination of in vivo structural connectivity by providing measures of WM microstructure and integrity based on the extent of water diffusion (14). Several DTI measures are typically quantified, with fractional anisotropy (FA) being the most commonly used to reflect WM integrity. Higher FA values suggest greater diffusion in the direction of the axon, and thus greater WM integrity. Other measures, including mean diffusivity (MD), radial diffusivity (RD), and axial diffusivity (AD), can also be determined to investigate different aspects of WM microstructure.
To date, most DTI research in MDD has investigated adults and consistently reported decreased FA in tracts connected to the prefrontal cortex (PFC) or tracts connecting the two hemispheres within the PFC (15, 16). Only one DTI study was carried out in adolescents with depression, demonstrating a similar pattern to adult MDD of reduced FA in tracts connected to the subgenual ACC and the PFC [i.e., uncinate fasciculus, inferior-fronto-occipital fasciculus, anterior cingulum, superior longitudinal fasciculus; (17)]. However, results may have been impacted by the concurrent use of psychotropic medication and past substance abuse in some subjects. Relatedly, medication-naïve adolescents with a familial risk for unipolar depression also demonstrated reduced FA compared to HC in similar tracts (18).
In this study, we aimed to expand on prior work by examining WM integrity in psychotropic medication-free adolescents with MDD compared to HC. Given the inherent heterogeneity of adolescent MDD, we further sought to identify specific WM alterations in relation to MDD severity as well as anhedonia and irritability – two core symptoms of adolescent MDD. Due to our desire to explore a range in depression severity, we included patients with mild to moderate severity. Based on others’ and our prior findings (8, 15–17), we hypothesized that adolescents with MDD would manifest less restricted diffusion (i.e., decreased WM integrity) compared to HC in tracts connecting frontal, striatal, and limbic regions. We also predicted that similar tracts would be associated with depression severity. For anhedonia, we expected reduced WM integrity in tracts that have been implicated in reward-related processing in the ventral striatum [i.e., subgenual cingulate, forceps minor, inferior-fronto-occipital fasciculus, anterior thalamic radiation (ATR), anterior limb of the internal capsule; (19)], and that these would be distinct from those associated with irritability. Finally, only one study has examined irritability in a clinical population [i.e., Huntington’s disease; (20)] and found involvement of the amygdala. As such, we predicted the impairment of tracts connecting to the amygdala (e.g., uncinate fasciculus, inferior-fronto-occipital fasciculus, inferior longitudinal fasciculus).
Materials and Methods
Participants
The sample population partially overlapped with that used for our previously published resting state functional magnetic resonance imaging research (8), and was comprised of 17 adolescents with MDD (ages 13–20 years, M = 16.8, SD = 2.2, 8 female) and 16 HC (ages 13–19 years, M = 16.4, SD = 1.4, 10 female) group matched for age, and all were right-handed. Depressed adolescents were recruited through the New York University (NYU) Child Study Center, the Bellevue Hospital Center Department of Psychiatry, and local advertisements in the NY metropolitan area. HC were recruited from the greater NY metropolitan area through local advertisements and from the families of NYU staff. The study was approved by the NYU School of Medicine and the Icahn School of Medicine at Mount Sinai institutional review boards. Prior to enrollment, study procedures were explained to the subjects and parents. Written informed consent was provided by participants age 18 and older; those under age 18 provided signed assent and a parent/guardian provided signed informed consent.
Inclusion and exclusion criteria
All subjects were ≤20 years old and did not present with any significant medical or neurological disorders. Other exclusionary criteria consisted of an IQ < 80, MRI contraindications as assessed by a standard screening form, a positive urine toxicology test or a positive urine pregnancy test in females.
All MDD subjects met the DSM-IV-TR diagnosis of MDD with a current episode ≥8 weeks duration, and raw severity score ≥40 (i.e., T score ≥ 63) during the initial evaluation on the Children’s Depression Rating Scale-Revised (CDRS-R), and were psychotropic medication-free for at least 7 half-lives of the medication. To explore a wider range of depression severity we included patients presenting with mild to severe depression on the date of the scan. Exclusionary criteria for the MDD group included current or past DSM-IV-TR diagnoses of bipolar disorder, schizophrenia, pervasive developmental disorder, panic disorder, obsessive-compulsive disorder, conduct disorder, Tourette’s disorder, or a substance-related disorder in the past 12 months. Current diagnoses of post-traumatic stress disorder or an eating disorder were also exclusionary. In addition, acute suicidality requiring immediate inpatient admission was exclusionary. HC subjects did not meet the criteria for any major current or past DSM-IV-TR diagnoses and had never received psychotropic medication.
Clinical Assessments
All subjects were assessed by a board-certified child and adolescent psychiatrist or a clinical psychologist at the NYU Child Study Center. Clinical diagnoses were established using the Schedule for Affective Disorders and Schizophrenia for School-Age Children-Present and Lifetime Version [KSADS-PL; (21)], a semi-structured interview performed with both the subjects and their parents. Depression severity was assessed by the CDRS-R and the Beck Depression Inventory, second edition [BDI-II; (22)]. Additionally, suicidality and anxiety were assessed using the Beck Scale for Suicidal Ideation [BSSI; (23)] and the Multidimensional Anxiety Scale for Children [MASC; (24)], respectively. The Kaufman Brief Intelligence Test (25) or the Wechsler Abbreviated Scale of Intelligence (26) were used to estimate IQ. Urine toxicology and pregnancy tests were administered on the day of the scan.
Anhedonia
Our approach to quantifying anhedonia allows for clinician- and self-rated assessments to contribute equally to the anhedonia score (range 1–13). As in our previous studies (8, 9, 27), the score for each subject was computed by summing the responses to three items associated with anhedonia from the clinician-rated CDRS-R (item 2: “difficulty having fun;” scale of 1–7) and the self-rated BDI-II (items 4: “loss of pleasure” and 12: “loss of interest;” scales of 0–3).
Irritability
Our approach again combined both clinician- and self-rated assessments to contribute to the irritability score (range 1–10). The score for each subject was computed by summing the responses to the items associated with irritability from the CDRS-R (item 8: “irritability;” scale of 1–7) and the BDI-II (item 17: “irritability;” scale of 0–3).
Data Acquisition and Analysis
Diffusion data were acquired on a Siemens Allegra 3.0 T scanner at the NYU Center for Brain Imaging using a single-channel head coil. Diffusion-weighted echo-planar images (EPI) were acquired along 12 diffusion gradient directions for acquisition of 35 slices through the whole brain (TR = 6000 ms, TE = 82 ms, flip angle = 90°, b value = 1000 s/mm2, FOV = 192 mm, 128 × 128 matrix, slice thickness = 2.5 mm, with four averages). High-resolution T1-weighted anatomical images were acquired using a magnetization-prepared gradient echo sequence (TR = 2530 ms; TE = 3.25 ms; TI = 1100 ms; flip angle = 7°; 128 slices; FOV = 256 mm; acquisition voxel size = 1.3 mm × 1 mm × 1.3 mm).
All preprocessing was performed using FMRIB’s Software Library (FSL; Oxford, UK): FMRIB’s Diffusion Toolbox (FDT). Preprocessing began with eddy current correction to correct for gradient-coil distortions and small head motions, using affine registration to a b0 reference volume. A diffusion tensor model was fitted to each voxel along the principal λ1, λ2, λ3 directions to generate FA, MD, RD, and AD. We then implemented FSL’s tract-based spatial statistics pipeline [TBSS; (28)], in which a non-linear registration aligned each subject to the FMRIB58_FA template in 1 mm × 1 mm × 1 mm standard space, and then warped each subject into standard Montreal Neurological Institute space (MNI152). A WM “skeleton” was then generated representing a single line running down the centers of all of the common WM fibers by using an FA cut-off of 0.2, and relevant diffusivity measures (i.e., FA, MD, RD, AD) were projected onto the skeleton. Group statistical analysis was then conducted only on voxels within the WM skeleton mask, therefore restricting the voxel-wise analysis only to voxels with high confidence of lying within equivalent major WM pathways in each individual.
In order to assess differences in FA, MD, RD, and AD between the MDD and HC groups, we used FSL’s Randomise with 5000 permutations to perform voxel-wise independent samples t-tests using voxel-based thresholding while controlling for age and sex. Group comparisons did not withstand stringent correction for multiple comparisons using family-wise error correction (FWE; implemented by Randomise) or FDR correction (implemented by FSL’s FDR program). As such, we performed a second, more exploratory analysis in which we accepted clusters of at least 10 contiguous voxels at p < 0.001.
To investigate relationships with depression severity, anhedonia, and irritability, Randomise was again used to perform a series of one-sample t-tests using the score for each category, respectively, while controlling for age and gender. Due to the low variability in scores in the HC group, as well as the nature of the study topic, the analysis was limited to the MDD group whose scores were normally distributed. Once again, tests did not withstand correction for multiple comparisons and we used the more exploratory approach of accepting clusters of at least 10 contiguous voxels at p < 0.001. We used FSL’s cluster program to extract all clusters across the brain, and anatomical localization of each cluster was determined using the FSLView atlas toolbox and the relevant gray matter (Harvard-Oxford Cortical/Subcortical) and WM (Johns Hopkins University WM tractography) atlases. Brain imaging results were prepared for display using FSL’s tbss_fill script, which displays results superimposed upon the WM skeleton from the group TBSS analysis.
Results
Participants
Demographic and clinical characteristics are summarized in Table 1. One subject with MDD had been treated with Lexapro and Ambien for 7 months, but was medication-free for approximately 14 months prior to scanning. A second subject with MDD had a brief trial with Prozac which was self-discontinued prior to participation in this study. All other subjects were psychotropic medication-naïve. Fifteen subjects with MDD had experienced only one episode of depression, with length of episode ranging from 4 to 48 months, and two patients reported having two distinct episodes. Two Shapiro–Wilk tests revealed that anhedonia and irritability were both normally distributed within the MDD group, ps = 0.81, 0.48, respectively. Depression severity (excluding the anhedonia- or irritability-related items) was significantly correlated with both severity of anhedonia (r = 0.66, p < 0.005) and irritability (r = 0.66, p < 0.005) within our MDD sample. Anhedonia and irritability were not correlated (r = 0.37, p = 0.15).
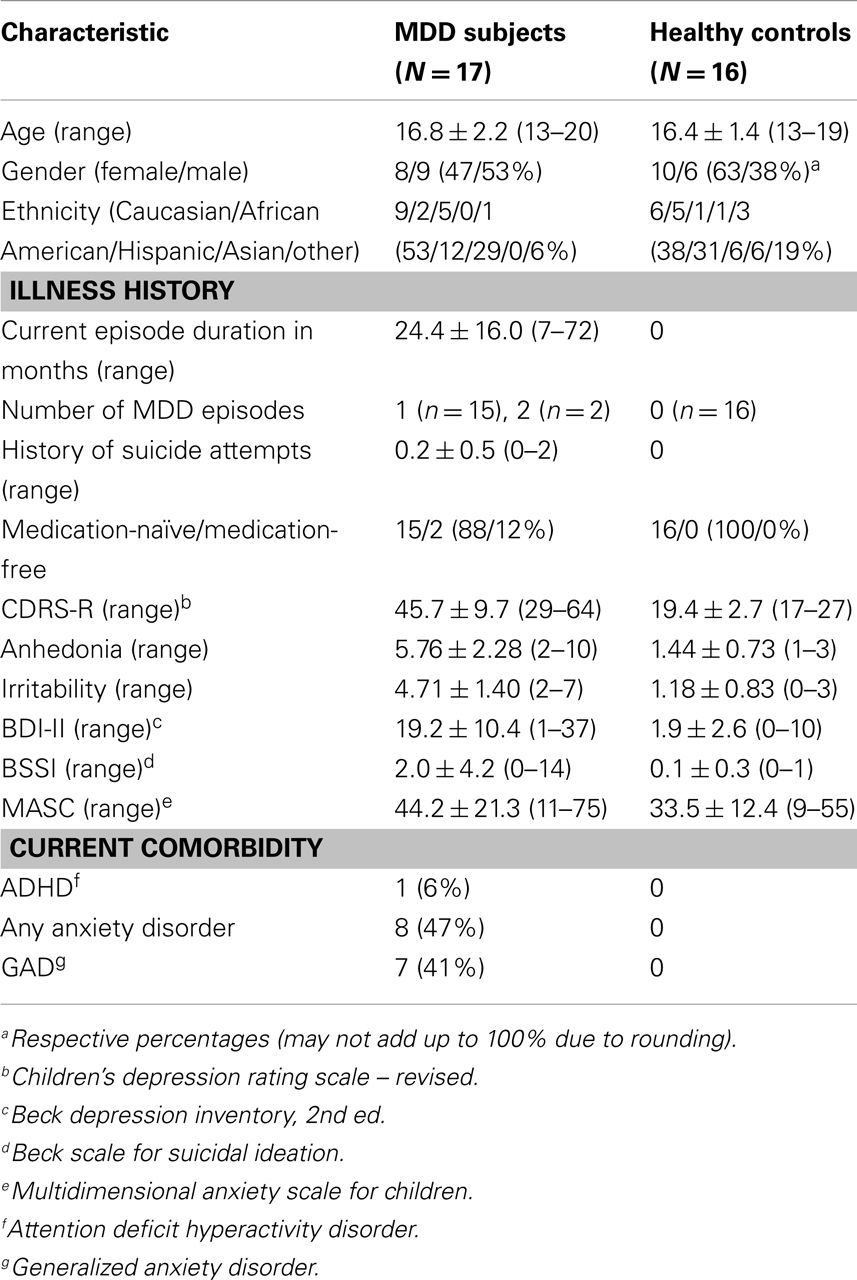
Table 1. Demographic and clinical characteristics of adolescents with major depressive disorder (MDD) and healthy controls.
Whole-Brain Group Comparison
No voxel-wise group comparisons for FA, MD, RD, or AD withstood correction for multiple comparisons. An exploratory analysis using a threshold of p < 0.001, uncorrected with clusters exceeding 10 contiguous voxels, revealed 4 significant clusters (Table 2, Figure 1). Compared with HC, the MDD group had lower FA in the anterior cingulum, and lower AD in the anterior corona radiata (ACR). However, the MDD group also had greater FA and lower RD in the posterior cingulum compared to HC.
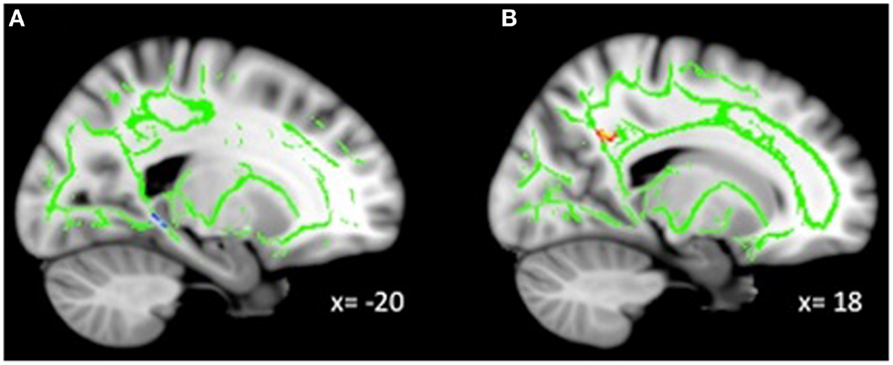
Figure 1. (A) Increased WM integrity in the MDD group vs. HC in the posterior cingulum near the hippocampus; (B) decreased WM integrity in the MDD group vs. HC in the anterior cingulum near the precuneus.
Whole-Brain Correlations with Depression Severity in MDD
Exploratory analyses revealed a total of 16 uncorrected clusters, with 5 overlapping clusters between the 4 diffusivity measures (Table 3, Figure 2). As depression severity increased, FA decreased in the genu of the corpus callosum, the sagittal stratum, the ATR, and the anterior cingulum. Additionally, a positive correlation between MD and depression severity was found in the same sagittal stratum cluster as the FA analysis, as well as in clusters in the ATR and corticospinal tract. Similarly, illness severity was positively correlated with RD in the same sagittal stratum cluster as the FA and MD analyses, the same genu of the corpus callosum and ATR clusters as the FA analysis, the same ATR cluster as the MD analysis, and a cluster in the superior longitudinal fasciculus (SLF). Finally, increased illness severity was associated with increased AD in the same corticospinal cluster as the MD analysis as well as in clusters in the inferior-fronto-occipital fasciculus (IFOF), the SLF, and fibers projecting to the orbitofrontal cortex (OFC).
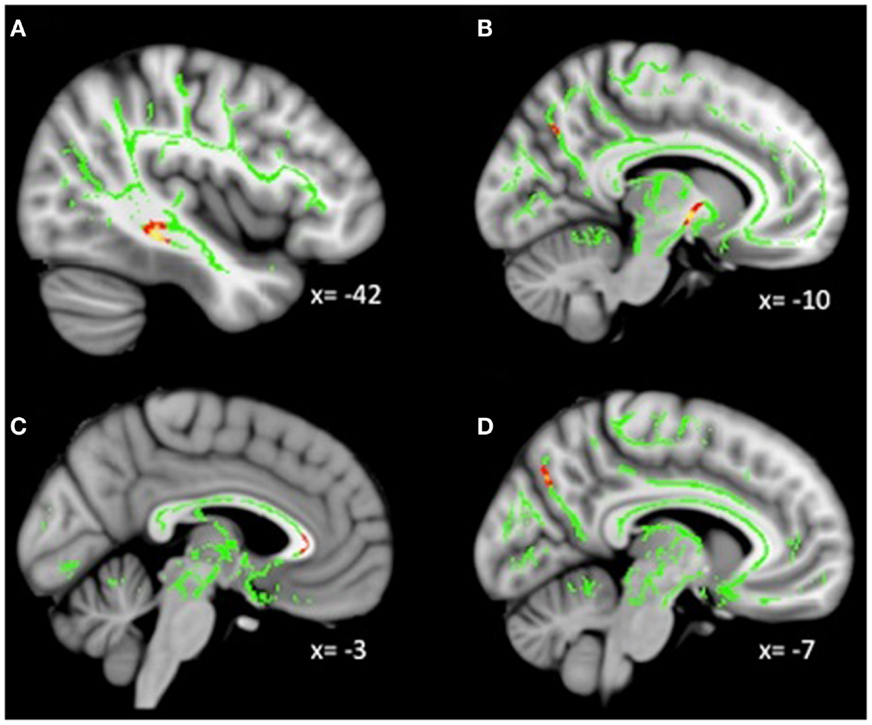
Figure 2. Decreased WM integrity as depression severity increased in the (A) sagittal stratum near the PHG; (B) ATR near the pallidum; (C) genu of the corpus callosum and anterior cingulate; (D) anterior cingulate near the precuneus. Key: PHG, parahippocampal gyrus; ATR, anterior thalamic radiation.
Whole-Brain Correlations with Anhedonia in MDD
Analyses revealed a total of 14 uncorrected clusters, with 2 overlapping clusters between FA and RD (Table 4, Figure 3). As anhedonia increased, FA increased in a cluster in the posterior cingulum near the hippocampus – similar to a cluster from the group comparison analysis – and decreased in the anterior limb of the internal capsule, OFC projection fibers, and the posterior cingulum near the precuneus. Furthermore, anhedonia was positively correlated with MD in OFC projection fibers, the external capsule, and the sagittal stratum. Additionally, increased anhedonia severity was associated with greater RD in the same posterior limb of the internal capsule and posterior cingulum clusters as the FA analysis, the same OFC projection fibers as the MD analysis, and clusters in the ATR and corticospinal tract. Finally, there were positive correlations between anhedonia and AD in the corticospinal tract and projection fibers into the occipital cortex.
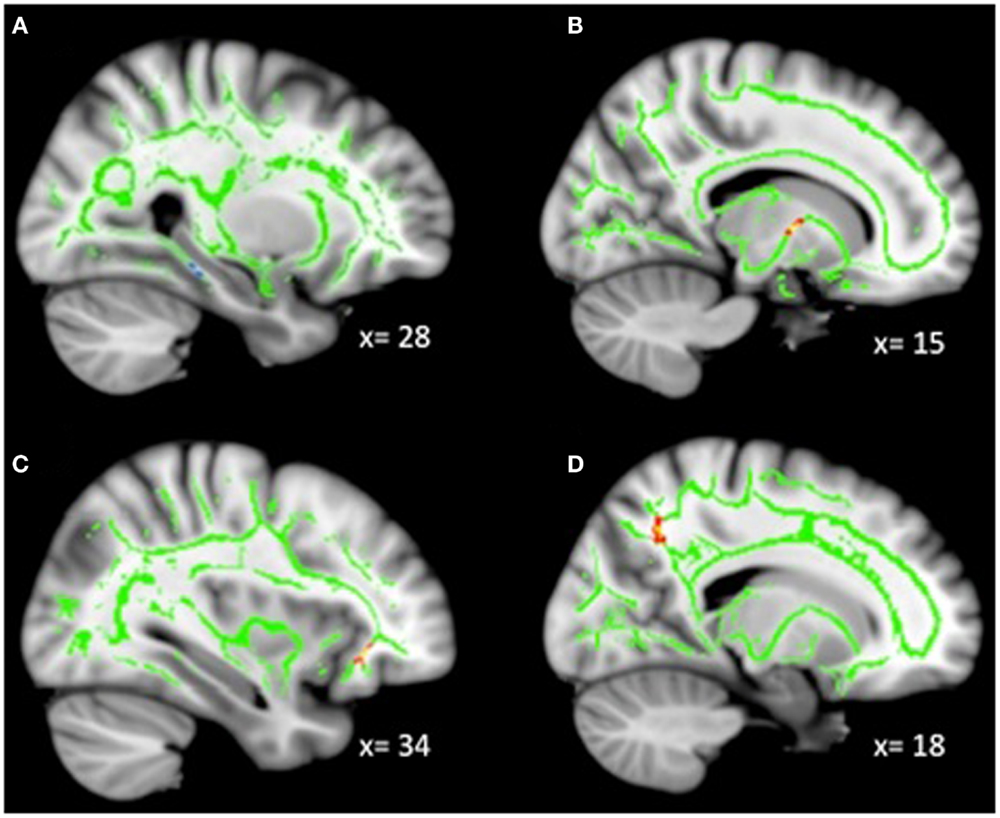
Figure 3. (A) Increased WM integrity as anhedonia increased in the posterior cingulum near the hippocampus; decreased WM integrity as anhedonia increased in the (B) anterior limb of the internal capsule; (C) IFOF near the OFC; (D) posterior cingulum near the precuneus. Key: IFOF, inferior-fronto-occipital fasciculus; OFC, orbitofrontal cortex.
Whole-Brain Correlations with Irritability in MDD
Analyses revealed a total of 14 uncorrected clusters, with 2 overlapping clusters (Table 5, Figure 4). As irritability increased, FA decreased in clusters in the sagittal stratum and IFOF, while MD increased in the same sagittal stratum cluster as well as in clusters in the ACR, SLF, and IFOF. For RD, positive correlations with irritability were evident in the same sagittal stratum cluster, the anterior limb of the internal capsule, and the SLF. Positive correlations were also found between AD and irritability in the same ACR cluster as the MD analysis as well as in the IFOF, the corticospinal tract, and the SLF.
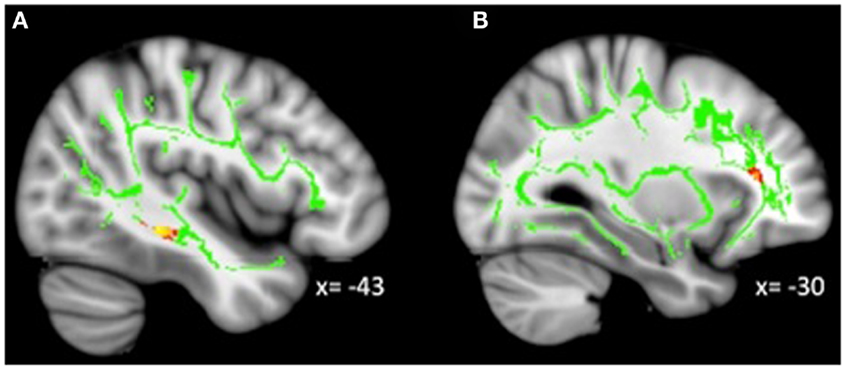
Figure 4. Decreased WM integrity as irritability increased in the (A) sagittal stratum in the IT; (B) IFOF near the MFG. Key: IT, inferior temporal cortex; IFOF, inferior-fronto-occipital fasciculus; MFG, medial frontal gyrus.
Discussion
Consistent with our hypotheses, analyses revealed reduced WM integrity (i.e., decreased FA, and increased MD, RD, AD) in the MDD group compared to HC as well as in the more severely depressed, anhedonic, and irritable patients. Furthermore, despite significant correlations between the two dimensional measures and depression severity, we found distinct WM alterations for both anhedonia and irritability that differed from those for depression severity. Reduced integrity was found in fronto-striatal and thalamic tracts, the corpus callosum, and tracts connected to the inferior temporal (IT) cortex. Additionally, reduced integrity in the sagittal stratum was consistently found in our analyses to be correlated with increasing depression severity, anhedonia, and irritability. Unexpectedly, analyses also revealed a cluster in the posterior cingulum near the hippocampus which demonstrated more anisotropic diffusion, both as anhedonia increased and in the MDD group vs. HC. Finally, it is interesting to note that in many of our analyses there was overlap in the clusters demonstrating a relationship with FA and RD, potentially suggesting that structural issues related to RD are driving the observed relationships with FA in this and other studies.
Group Differences in WM Integrity
Group differences were observed in both the posterior and anterior cingulum. Specifically, depressed adolescents demonstrated decreased WM integrity in the anterior cingulum near the precuneus, and increased integrity in the posterior cingulum near the hippocampus, compared to HC. The cingulum connects the cingulate and entorhinal cortices and is broadly involved in attention, memory, and emotions (29, 30). The anterior portion of the cingulate has been implicated in emotional processing and depression (31). Altered functioning, connectivity, and diffusion around the precuneus are frequently reported in MDD (32, 33). Given the role of the precuneus in self-related processes, and that self-processing is typically altered in depression (34), this potentially suggests that reduced WM integrity contributes to altered functioning in this region early in the course of the disease.
The MDD group also demonstrated more coherent diffusion in the posterior cingulum near the hippocampus. The posterior cingulate is involved in cognitive functions including attention and memory (35). Functional hyperactivity in the hippocampus (36–38), as well as decreased hippocampal volume (39), are consistent findings in adult MDD. Given the role of the hippocampus in learning and memory (40), but also in the regulation of motivation and emotion (41, 42), this region is critical to carrying out normal behaviors that may be altered in depression. Furthermore, greater WM integrity in tracts leading to the hippocampus would be consistent with the literature demonstrating hyperactivity of this region in non-medicated MDD patients. Overall, the categorical comparison between depressed adolescents and HC revealed differences in an important tract connecting prefrontal and limbic regions.
Depression Severity and WM Integrity
Our use of an approach that accounts for a range of depression severity in our sample revealed a pattern of reduced WM integrity as depression severity increased. Specifically, we found reduced integrity in the genu of the corpus callosum, a region that connects prefrontal and orbitofrontal cortices. Many studies have documented altered diffusivity in the genu (16) as well as reduced volume (6, 43–45). Given that the prefrontal and orbitofrontal cortices are involved in critical processes, including decision-making, attention, reward processing, and the evaluation and regulation of emotion (46–48), an interruption in communication between these areas has implications for depression and mood disorders.
Additionally, we found decreased integrity in the sagittal stratum with increasing severity, not only in this analysis, but also in the dimensional analyses within the MDD population. The sagittal stratum is a complex fiber bundle connecting the occipital cortex to the rest of the brain, and includes fibers from many major tracts including the ILF and IFOF (49). The ILF and IFOF both connect the occipital cortex to temporal limbic structures (i.e., amygdala, hippocampus) and the PFC, although the IFOF connects directly to the OFC and the ILF does so indirectly through the uncinate fasciculus (50). Therefore, both tracts are involved in connecting visual information with areas involved in emotional memories, judgments, and behaviors. A meta-analysis of diffusion studies of patients with MDD found WM alterations in both the ILF and IFOF (16). Additionally, alterations in WM have been found in the IFOF for depressed adolescents (17), adolescents with a familial risk for depression (18), and adults with MDD (51).
We also observed reduced integrity with increasing severity in bilateral clusters in the ATR near the pallidum. The ATR connects thalamic nuclei with the PFC through the anterior limb of the internal capsule. Reduced WM integrity has been reported in the ATR in several studies of depressed adults (16). Furthermore, given the role of the thalamus in motivation and goal pursuit (52, 53), altered connectivity within this circuit could contribute to the motivational deficits associated with depression.
Additionally, increased illness severity was associated with reduced integrity in the corticospinal tract near the postcentral gyrus. The corticospinal tract transmits motor impulses from the motor and premotor cortices to the spinal cord. Although this was an unexpected finding, motor disturbances and retardation are a relevant clinical symptom of depression (54). In this way, altered diffusivity may be related to the observed slowing and impairment of motor functions. Finally, we again found decreased integrity with increasing severity in the previously described anterior cingulum near the precuneus, which is consistent with the findings from our group analysis. Overall, our analysis with varied levels of depression severity was more robust than the categorical comparison and revealed a more extensive network of reduced WM integrity.
Anhedonia and WM Integrity
The dimensional analysis with anhedonia revealed an association between increased anhedonia and reduced integrity in the anterior limb of the internal capsule near the thalamus, a tract implicated in reward processing (19). The anterior limb of the internal capsule connects the thalamus with cingulate and prefrontal cortices, which are heavily involved in motivation, decision-making, and evaluating the saliency of emotional and rewarding stimuli. Additionally, increased anhedonia was correlated with reduced integrity in tracts (i.e., IFOF, projection fibers) connected to the posterior lateral OFC (BA 47), an area involved in many functions including emotional and reward processing, complex learning, and the inhibition of responses (46, 47, 55). Depressed patients have demonstrated reduced gray matter volume in the posterior lateral OFC as well as altered functional responses to emotional stimuli, reward processing, and reversal learning (56).
We also found reduced integrity in the external capsule as anhedonia increased. The external capsule contains cholinergic fibers projecting from the basal forebrain to the cerebral cortex. Reduced integrity in the external capsule has been found previously in adult MDD (57, 58). Furthermore, we once again found reduced integrity in the previously discussed sagittal stratum and posterior cingulum near the precuneus with greater symptom severity. Finally, the analysis revealed increased integrity with increased anhedonia in the posterior cingulum near the hippocampus, in an area fairly close to the cluster that showed increased integrity in the MDD group in our categorical comparison. In this way, it is possible that anhedonic symptoms are related to the group differences we observed. Given the previously discussed role of the hippocampus and limbic system in the regulation of motivation and emotion, the relationship between hippocampal functioning and anhedonia represents an important area for future research.
Irritability and WM Integrity
As predicted, we found decreased integrity as irritability increased in a tract near the amygdala (i.e., sagittal stratum including the ILF and IFOF). However, increased irritability was correlated with decreased integrity in tracts primarily connecting to prefrontal and occipital cortices. We also found clusters in the previously discussed IFOF, although one was in the lingual gyrus while the other was in the middle frontal gyrus. The lingual gyrus has been implicated in processing emotional faces (59), which is typically altered in MDD (60). Altered cerebral blood flow and resting state connectivity have been demonstrated in the lingual gyrus in adults with MDD (61, 62). Additionally, decreased integrity in WM has previously been found around the middle frontal gyrus (63), an area broadly involved in a variety of higher-level cognitive processes (64) which are often compromised in MDD.
Reduced integrity related to elevated irritability was also found in the ACR, which connects the striatum to the ACC. Reduced integrity has previously been demonstrated in the ACR in pediatric bipolar patients (65), and dysfunctional activity in the ACC is typically considered a hallmark of depression (37, 42, 66–70). Furthermore, altered intrinsic functional connectivity (i.e., resting state) between the striatum and ACC has been documented for depressed adolescents (8). Finally, a cluster in the SLF in the IT gyrus was found. The SLF is a major bidirectional association tract connecting large parts of the frontal cortex with the parietal, temporal, and occipital lobes. Less restricted diffusion in the SLF has been previously demonstrated for depressed adolescents (17), adolescents with a genetic risk for depression (18), and adults with MDD (71).
Measures of WM Integrity
Although a complete discussion of RD and AD goes beyond the scope of this paper, it is interesting to note that for both the categorical and dimensional analyses we found overlap in clusters with reduced FA and increased RD, but no overlapping relationships with AD. Increased RD may be caused by disturbances in myelin, whereas decreased AD has been suggested to reflect disrupted axonal integrity (72–74). As such, our findings and those from previous research may reflect that alterations in FA for MDD are being driven more by issues of myelination than axonal integrity. However, further research is needed to replicate and expand upon a possible mechanism.
Limitations and Future Directions
Although our findings are consistent with other clinical studies investigating altered WM in depressed adolescents, it should be noted that very liberal thresholds were used for the analyses and the inability to correct for multiple comparisons is an issue of concern. Although our statistical methodology and sample size were comparable to those of other studies of clinical populations using DTI (17, 63, 75), it is possible that the sample sizes used in many clinical studies are not large enough to produce adequate statistical power. In this way, it is difficult to adequately balance the concerns of committing a Type I error by not correcting while also avoiding a Type II error due to small sample sizes and reduced statistical power. Therefore, our findings are considered preliminary. Furthermore, the inclusion of patients with milder symptomatology may have weakened our ability to detect group differences.
Although small sample sizes may be a possible explanation for the relatively weak results in our and other clinical studies of adolescent depression, another possibility is that the adolescent brain is still malleable and the alterations in WM structure may not fully take hold until adulthood (11). Therefore, it is even more pressing to understand a neuroimmunological model of depression and the factors that may contribute to changes in WM before chronicity begins to take effect. For example, given past findings that depressed adolescents exhibit higher levels of circulating inflammatory cytokines (76), one possible explanation for the observed reduction in FA in adult MDD is that it may reflect effects of chronic low grade inflammation. Additionally, given our previous research on fronto-striatal functional connectivity in MDD, future studies should investigate altered WM microstructure using a targeted tractography approach. Finally, further research is needed to investigate this hypothesis and other models of the systemic consequences of depression. To this end, a better understanding of what FA, MD, AD, and RD illustrate in an adolescent population, as well as the factors that contribute to these diffusivity measures, is needed in the field.
Conclusion
Our investigation of altered WM microstructure in medication-free adolescents with MDD revealed a general pattern of impaired WM integrity in the depressed adolescents, and as depression severity, anhedonia, and irritability increased. Our findings are consistent with an overall hypothesis that depression, even in adolescence, involves a disconnection of prefrontal, striatal, and limbic emotional areas (16). Although this represents a good step toward understanding depression during this critical period, more research is needed to understand the factors that ultimately contribute to altered WM microstructure in order to develop potential interventions.
Conflict of Interest Statement
The authors declare that the research was conducted in the absence of any commercial or financial relationships that could be construed as a potential conflict of interest.
Acknowledgments
We would like to thank Chao-Gan Yan of the Child Mind Institute for his help with this project. This study was supported by grants from the NIH (AT004576, MH095807), the Brain & Behavior Research Foundation (formerly NARSAD), the Hope for Depression Research Foundation, and generous gifts from the Leon Levy Foundation.
References
1. Bukstein OG, Brent DA, Kaminer Y. Comorbidity of substance abuse and other psychiatric disorders in adolescents. Am J Psychiatry (1989) 146(9):1131–41.
2. McCarty CA, Mason WA, Kosterman R, Hawkins JD, Lengua LJ, McCauley E. Adolescent school failure predicts later depression among girls. J Adolesc Health (2008) 43(2):180–7. doi:10.1016/j.jadohealth.2008.01.023
3. Seroczynski AD, Cole DA, Maxwell SE. Cumulative and compensatory effects of competence and incompetence on depressive symptoms in children. J Abnorm Psychol (1997) 106(4):586–97. doi:10.1037/0021-843X.106.4.586
4. Levy JC, Deykin EY. Suicidality, depression, and substance abuse in adolescence. Am J Psychiatry (1989) 146(11):1462–7.
5. Centers for Disease Control and Prevention. Mental Health Surveillance Among Children – United States, 2005-2011. Atlanta, GA: U.S. Department of Health and Human Services, Centers for Disease Control and Prevention (2013).
6. Macmaster FP, Carrey N, Marie Langevin L. Corpus callosal morphology in early onset adolescent depression. J Affect Disord (2013) 145(2):256–9. doi:10.1016/j.jad.2012.04.047
7. Yang TT, Simmons AN, Matthews SC, Tapert SF, Frank GK, Max JE, et al. Adolescents with major depression demonstrate increased amygdala activation. J Am Acad Child Adolesc Psychiatry (2010) 49(1):42–51. doi:10.1016/j.jaac.2009.09.004
8. Gabbay V, Ely BA, Li Q, Bangaru SD, Panzer AM, Alonso CM, et al. Striatum-Based circuitry of adolescent depression and anhedonia. J Am Acad Child Adolesc Psychiatry (2013) 52(6):14. doi:10.1016/j.jaac.2013.04.003
9. Gabbay V, Mao X, Klein RG, Ely BA, Babb JS, Panzer AM, et al. Anterior cingulate cortex γ-aminobutyric acid in depressed adolescents: relationship to anhedonia. Arch Gen Psychiatry (2012) 69(2):139–49. doi:10.1001/archgenpsychiatry.2011.131
10. Forbes EE. fMRI studies of reward processing in adolescent depression. Neuropsychopharmacology (2011) 36(1):372–3. doi:10.1038/npp.2010.164
11. Giorgio A, Watkins KE, Chadwick M, James S, Winmill L, Douaud G, et al. Longitudinal changes in grey and white matter during adolescence. Neuroimage (2010) 49(1):94–103. doi:10.1016/j.neuroimage.2009.08.003
12. Paus T. Mapping brain maturation and cognitive development during adolescence. Trends Cogn Sci (2005) 9(2):60–8. doi:10.1016/j.tics.2004.12.008
13. Paus T, Keshavan M, Giedd JN. Why do many psychiatric disorders emerge during adolescence? Nat Rev Neurosci (2008) 9(12):947–57. doi:10.1038/Nrn2513
14. Alexander AL, Lee JE, Lazar M, Field AS. Diffusion tensor imaging of the brain. Neurotherapeutics (2007) 4(3):316–29. doi:10.1016/j.nurt.2007.05.011
15. Sexton CE, Mackay CE, Ebmeier KP. A systematic review of diffusion tensor imaging studies in affective disorders. Biol Psychiatry (2009) 66(9):814–23. doi:10.1016/j.biopsych.2009.05.024
16. Liao Y, Huang X, Wu Q, Yang C, Kuang W, Du M, et al. Is depression a disconnection syndrome? Meta-analysis of diffusion tensor imaging studies in patients with MDD. J Psychiatry Neurosci (2013) 38(1):49–56. doi:10.1503/jpn.110180
17. Cullen KR, Klimes-Dougan B, Muetzel R, Mueller BA, Camchong J, Houri A, et al. Altered white matter microstructure in adolescents with major depression: a preliminary study. J Am Acad Child Adolesc Psychiatry (2010) 49(2):173–83.e1. doi:10.1016/j.jaac.2009.11.005
18. Huang H, Fan X, Williamson DE, Rao U. White matter changes in healthy adolescents at familial risk for unipolar depression: a diffusion tensor imaging study. Neuropsychopharmacology (2011) 36(3):684–91. doi:10.1038/npp.2010.199
19. Koch K, Wagner G, Schachtzabel C, Schultz CC, Gullmar D, Reichenbach JR, et al. Association between white matter fiber structure and reward-related reactivity of the ventral striatum. Hum Brain Mapp (2013). doi:10.1002/hbm.22284. [Epub ahead of print].
20. Kloppel S, Stonnington CM, Petrovic P, Mobbs D, Tuscher O, Craufurd D, et al. Irritability in pre-clinical Huntington’s disease. Neuropsychologia (2010) 48(2):549–57. doi:10.1016/j.neuropsychologia.2009.10.016
21. Kaufman J, Birmaher B, Brent D, Rao U, Flynn C, Moreci P, et al. Schedule for affective disorders and schizophrenia for school-age children-present and lifetime version (K-SADS-PL): initial reliability and validity data. J Am Acad Child Adolesc Psychiatry (1997) 36(7):980–8. doi:10.1097/00004583-199707000-00021
22. Beck AT, Guth D, Steer RA, Ball R. Screening for major depression disorders in medical inpatients with the Beck depression inventory for primary care. Behav Res Ther (1997) 35(8):785–91. doi:10.1016/S0005-7967(97)00025-9
23. Beck AT, Kovacs M, Weissman A. Assessment of suicidal intention: the scale for suicide ideation. J Consult Clin Psychol (1979) 47(2):343–52. doi:10.1037/0022-006X.47.2.343
24. March JS, Parker JD, Sullivan K, Stallings P, Conners CK. The multidimensional anxiety scale for children (MASC): factor structure, reliability, and validity. J Am Acad Child Adolesc Psychiatry (1997) 36(4):554–65. doi:10.1097/00004583-199704000-00019
25. Kaufman AS, Kaufman NL. Manual for the Kaufman Brief Intelligence Test. Circle Pines, MN: American Guidance Service (1990).
26. Wechsler D. Wechsler Abbreviated Scale of Intelligence. San Antonio, TX: The Psychological Corporation (1999).
27. Gabbay V, Ely BA, Babb J, Liebes L. The possible role of the kynurenine pathway in anhedonia in adolescents. J Neural Transm (2012) 119(2):253–60. doi:10.1007/s00702-011-0685-7
28. Smith SM, Jenkinson M, Johansen-Berg H, Rueckert D, Nichols TE, Mackay CE, et al. Tract-based spatial statistics: voxelwise analysis of multi-subject diffusion data. Neuroimage (2006) 31(4):1487–505. doi:10.1016/j.neuroimage.2006.02.024
29. Rudrauf D, Mehta S, Grabowski TJ. Disconnection’s renaissance takes shape: formal incorporation in group-level lesion studies. Cortex (2008) 44(8):1084–96. doi:10.1016/j.cortex.2008.05.005
30. Catani M. Thiebaut de Schotten M. A diffusion tensor imaging tractography atlas for virtual in vivo dissections. Cortex (2008) 44:1105–32. doi:10.1016/j.cortex.2008.05.004
31. Steele JD, Christmas D, Eljamel MS, Matthews K. Anterior cingulotomy for major depression: clinical outcome and relationship to lesion characteristics. Biol Psychiatry (2008) 63(7):670–7. doi:10.1016/j.biopsych.2007.07.019
32. Messina I, Sambin M, Palmieri A, Viviani R. Neural correlates of psychotherapy in anxiety and depression: a meta-analysis. PLoS One (2013) 8(9):e74657. doi:10.1371/journal.pone.0074657
33. Li C, Sun X, Zou K, Yang H, Huang X, Wang Y, et al. Voxel based analysis of DTI in depression patients. Int J Magn Reson Imaging (2007) 1(1):43–8.
34. Nejad AB, Fossati P, Lemogne C. Self-referential processing, rumination and cortical midline structures in major depression. Front Hum Neurosci (2013) 7:666. doi:10.3389/fnhum.2013.00666
35. Vogt BA, Finch DM, Olson CR. Functional heterogeneity in cingulate cortex: the anterior executive and posterior evaluative regions. Cereb Cortex (1992) 2(6):435–43. doi:10.1093/cercor/2.6.435-a
36. Campbell S, Macqueen G. The role of the hippocampus in the pathophysiology of major depression. J Psychiatry Neurosci (2004) 29(6):417–26.
37. Fitzgerald PB, Laird AR, Maller J, Daskalakis ZJ. A meta-analytic study of changes in brain activation in depression. Hum Brain Mapp (2008) 29(6):683–95. doi:10.1002/hbm.20426
38. Cao X, Liu Z, Xu C, Li J, Gao Q, Sun N, et al. Disrupted resting-state functional connectivity of the hippocampus in medication-naive patients with major depressive disorder. J Affect Disord (2012) 141(2–3):194–203. doi:10.1016/j.jad.2012.03.002
39. Sapolsky RM. Depression, antidepressants, and the shrinking hippocampus. Proc Natl Acad Sci U S A (2001) 98(22):12320–2. doi:10.1073/pnas.231475998
40. Eichenbaum H, Yonelinas AP, Ranganath C. The medial temporal lobe and recognition memory. Annu Rev Neurosci (2007) 30:123–52. doi:10.1146/annurev.neuro.30.051606.094328
41. Phillips ML, Drevets WC, Rauch SL, Lane R. Neurobiology of emotion perception I: the neural basis of normal emotion perception. Biol Psychiatry (2003) 54(5):504–14. doi:10.1016/S0006-3223(03)00168-9
42. Seminowicz DA, Mayberg HS, McIntosh AR, Goldapple K, Kennedy S, Segal Z, et al. Limbic-frontal circuitry in major depression: a path modeling meta-analysis. Neuroimage (2004) 22(1):409–18. doi:10.1016/j.neuroimage.2004.01.015
43. Lyoo IK, Kwon JS, Lee SJ, Han MH, Chang CG, Seo CS, et al. Decrease in genu of the corpus callosum in medication-naive, early-onset dysthymia and depressive personality disorder. Biol Psychiatry (2002) 52(12):1134–43. doi:10.1016/S0006-3223(02)01436-1
44. Lacerda AL, Brambilla P, Sassi RB, Nicoletti MA, Mallinger AG, Frank E, et al. Anatomical MRI study of corpus callosum in unipolar depression. J Psychiatr Res (2005) 39(4):347–54. doi:10.1016/j.jpsychires.2004.10.004
45. Kemp A, Macmaster FP, Jaworska N, Yang XR, Pradhan S, Mahnke D, et al. Age of onset and corpus callosal morphology in major depression. J Affect Disord (2013) 150(2):703–6. doi:10.1016/j.jad.2013.05.009
46. Amodio DM, Frith CD. Meeting of minds: the medial frontal cortex and social cognition. Nat Rev Neurosci (2006) 7(4):268–77. doi:10.1038/nrn1884
47. Elliott R, Dolan RJ, Frith CD. Dissociable functions in the medial and lateral orbitofrontal cortex: evidence from human neuroimaging studies. Cereb Cortex (2000) 10(3):308–17. doi:10.1093/cercor/10.3.308
49. Jellison BJ, Field AS, Medow J, Lazar M, Salamat MS, Alexander AL. Diffusion tensor imaging of cerebral white matter: a pictorial review of physics, fiber tract anatomy, and tumor imaging patterns. AJNR Am J Neuroradiol (2004) 25(3):356–69.
50. Ashtari M. Anatomy and functional role of the inferior longitudinal fasciculus: a search that has just begun. Dev Med Child Neurol (2012) 54(1):6–7. doi:10.1111/j.1469-8749.2011.04122.x
51. Kieseppa T, Eerola M, Mantyla R, Neuvonen T, Poutanen VP, Luoma K, et al. Major depressive disorder and white matter abnormalities: a diffusion tensor imaging study with tract-based spatial statistics. J Affect Disord (2010) 120(1–3):240–4. doi:10.1016/j.jad.2009.04.023
52. Cho YT, Fromm S, Guyer AE, Detloff A, Pine DS, Fudge JL, et al. Nucleus accumbens, thalamus and insula connectivity during incentive anticipation in typical adults and adolescents. Neuroimage (2012) 66C:508–21. doi:10.1016/j.neuroimage.2012.10.013
53. Krebs RM, Boehler CN, Roberts KC, Song AW, Woldorff MG. The involvement of the dopaminergic midbrain and cortico-striatal-thalamic circuits in the integration of reward prospect and attentional task demands. Cereb Cortex (2012) 22(3):607–15. doi:10.1093/cercor/bhr134
54. Mergl R, Pogarell O, Juckel G, Rihl J, Henkel V, Frodl T, et al. Hand-motor dysfunction in depression: characteristics and pharmacological effects. Clin EEG Neurosci (2007) 38(2):82–8. doi:10.1177/155005940703800210
55. Hooker C, Knight R. The role of lateral orbitofrontal cortex in the inhibitory control of emotion. In: Zald DH, Rauch SL editors. The Orbitofrontal Cortex. Oxford: Oxford University Press (2006). p. 307–24.
56. Drevets WC. Orbitofrontal cortex function and structure in depression. Ann N Y Acad Sci (2007) 1121:499–527. doi:10.1196/annals.1401.029
57. Korgaonkar MS, Grieve SM, Koslow SH, Gabrieli JD, Gordon E, Williams LM. Loss of white matter integrity in major depressive disorder: evidence using tract-based spatial statistical analysis of diffusion tensor imaging. Hum Brain Mapp (2011) 32(12):2161–71. doi:10.1002/hbm.21178
58. Guo WB, Liu F, Xue ZM, Gao K, Wu RR, Ma CQ, et al. Altered white matter integrity in young adults with first-episode, treatment-naive, and treatment-responsive depression. Neurosci Lett (2012) 522(2):139–44. doi:10.1016/j.neulet.2012.06.027
59. Kitada R, Johnsrude IS, Kochiyama T, Lederman SJ. Brain networks involved in haptic and visual identification of facial expressions of emotion: an fMRI study. Neuroimage (2010) 49(2):1677–89. doi:10.1016/j.neuroimage.2009.09.014
60. Stuhrmann A, Suslow T, Dannlowski U. Facial emotion processing in major depression: a systematic review of neuroimaging findings. Biol Mood Anxiety Disord (2011) 1(1):10. doi:10.1186/2045-5380-1-10
61. Zhang J, Wang J, Wu Q, Kuang W, Huang X, He Y, et al. Disrupted brain connectivity networks in drug-naive, first-episode major depressive disorder. Biol Psychiatry (2011) 70(4):334–42. doi:10.1016/j.biopsych.2011.05.018
62. Ito H, Kawashima R, Awata S, Ono S, Sato K, Goto R, et al. Hypoperfusion in the limbic system and prefrontal cortex in depression: SPECT with anatomic standardization technique. J Nucl Med (1996) 37(3):410–4.
63. Ma N, Li L, Shu N, Liu J, Gong G, He Z, et al. White matter abnormalities in first-episode, treatment-naive young adults with major depressive disorder. Am J Psychiatry (2007) 164(5):823–6. doi:10.1176/appi.ajp.164.5.823
64. Talati A, Hirsch J. Functional specialization within the medial frontal gyrus for perceptual go/no-go decisions based on “what,” “when,” and “where” related information: an fMRI study. J Cogn Neurosci (2005) 17(7):981–93. doi:10.1162/0898929054475226
65. Pavuluri MN, Yang S, Kamineni K, Passarotti AM, Srinivasan G, Harral EM, et al. Diffusion tensor imaging study of white matter fiber tracts in pediatric bipolar disorder and attention-deficit/hyperactivity disorder. Biol Psychiatry (2009) 65(7):586–93. doi:10.1016/j.biopsych.2008.10.015
66. Drevets WC, Bogers W, Raichle ME. Functional anatomical correlates of antidepressant drug treatment assessed using PET measures of regional glucose metabolism. Eur Neuropsychopharmacol (2002) 12(6):527–44. doi:10.1016/S0924-977X(02)00102-5
67. Davidson RJ, Pizzagalli D, Nitschke JB, Putnam K. Depression: perspectives from affective neuroscience. Annu Rev Psychol (2002) 53:545–74. doi:10.1146/annurev.psych.53.100901.135148
68. de Kwaasteniet B, Ruhe E, Caan M, Rive M, Olabarriaga S, Groefsema M, et al. Relation between structural and functional connectivity in major depressive disorder. Biol Psychiatry (2013) 74(1):40–7. doi:10.1016/j.biopsych.2012.12.024
69. Anand A, Li Y, Wang Y, Wu J, Gao S, Bukhari L, et al. Activity and connectivity of brain mood regulating circuit in depression: a functional magnetic resonance study. Biol Psychiatry (2005) 57(10):1079–88. doi:10.1016/j.biopsych.2005.02.021
70. Mayberg HS, Brannan SK, Tekell JL, Silva JA, Mahurin RK, McGinnis S, et al. Regional metabolic effects of fluoxetine in major depression: serial changes and relationship to clinical response. Biol Psychiatry (2000) 48(8):830–43. doi:10.1016/S0006-3223(00)01036-2
71. Murphy ML, Frodl T. Meta-analysis of diffusion tensor imaging studies shows altered fractional anisotropy occurring in distinct brain areas in association with depression. Biol Mood Anxiety Disord (2011) 1(1):3. doi:10.1186/2045-5380-1-3
72. Della Nave R, Ginestroni A, Tessa C, Giannelli M, Piacentini S, Filippi M, et al. Regional distribution and clinical correlates of white matter structural damage in Huntington disease: a tract-based spatial statistics study. AJNR Am J Neuroradiol (2010) 31(9):1675–81. doi:10.3174/ajnr.A2128
73. Kumar R, Macey PM, Woo MA, Harper RM. Rostral brain axonal injury in congenital central hypoventilation syndrome. J Neurosci Res (2010) 88(10):2146–54. doi:10.1002/jnr.22385
74. Kumar R, Nguyen HD, Macey PM, Woo MA, Harper RM. Regional brain axial and radial diffusivity changes during development. J Neurosci Res (2012) 90(2):346–55. doi:10.1002/jnr.22757
75. Versace A, Almeida JR, Hassel S, Walsh ND, Novelli M, Klein CR, et al. Elevated left and reduced right orbitomedial prefrontal fractional anisotropy in adults with bipolar disorder revealed by tract-based spatial statistics. Arch Gen Psychiatry (2008) 65(9):1041–52. doi:10.1001/archpsyc.65.9.1041
Keywords: depression, adolescent, white matter, diffusion tensor imaging, anhedonia, irritability
Citation: Henderson SE, Johnson AR, Vallejo AI, Katz L, Wong E and Gabbay V (2013) A preliminary study of white matter in adolescent depression: relationships with illness severity, anhedonia, and irritability. Front. Psychiatry 4:152. doi: 10.3389/fpsyt.2013.00152
Received: 04 October 2013; Accepted: 08 November 2013;
Published online: 25 November 2013.
Edited by:
Paul Croarkin, Mayo Clinic, USAReviewed by:
Faisal Al-Otaibi, Alfaisal University, Saudi ArabiaNiels Bergsland, Buffalo Neuroimaging Analysis Center, USA
Kirti Saxena, Baylor College of Medicine, USA
Susannah J. Tye, Mayo Clinic, USA
Copyright: © 2013 Henderson, Johnson, Vallejo, Katz, Wong and Gabbay. This is an open-access article distributed under the terms of the Creative Commons Attribution License (CC BY). The use, distribution or reproduction in other forums is permitted, provided the original author(s) or licensor are credited and that the original publication in this journal is cited, in accordance with accepted academic practice. No use, distribution or reproduction is permitted which does not comply with these terms.
*Correspondence: Vilma Gabbay, Department of Psychiatry, Icahn School of Medicine at Mount Sinai, One Gustave L. Levy Place, New York, NY 10029, USA e-mail: vilma.gabbay@mssm.edu