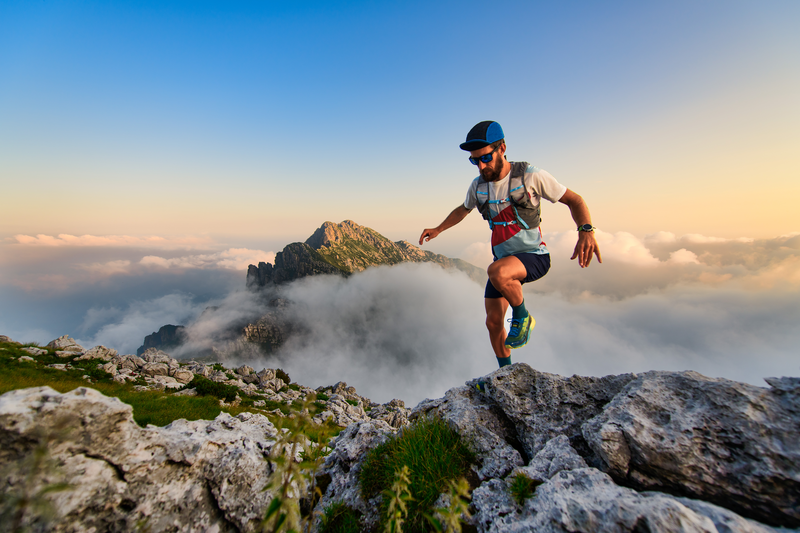
94% of researchers rate our articles as excellent or good
Learn more about the work of our research integrity team to safeguard the quality of each article we publish.
Find out more
ORIGINAL RESEARCH article
Front. Plant Sci.
Sec. Plant Nutrition
Volume 16 - 2025 | doi: 10.3389/fpls.2025.1549801
The final, formatted version of the article will be published soon.
You have multiple emails registered with Frontiers:
Please enter your email address:
If you already have an account, please login
You don't have a Frontiers account ? You can register here
Carbon, nitrogen and phosphorus, as the basic components of plants, determine plant growth and adaptation strategies, while there are certain differences in nutrient allocation among different plant organs. However,little is known about the manner in which resource allocation mediates the plant life history strategy. Here, we collected three census field survey datasets from the Heishiding 50-ha dynamic plot showing functional traits and nutrient allocation among leaves and roots (⍺nutrient) from 92 woody species to determine the relationship between nutrient allocation and the plant life history strategy. Carbon allocation ⍺carbon was mainly determined by intraspecific variation while nitrogen allocation ⍺nitrogen and phosphorus allocation ⍺phosphorus was determined by interspecific variation. Species allocating more nitrogen to leaves showed greater resource acquisition traits, while species allocating more nitrogen to roots showed greater resource conservation traits. We found a trade-off between the plant relative growth rate and conspecific density dependence; fast-growing species showed higher mortality with conspecific neighbors but tended to allocate more nitrogen to leaves rather than roots. Our study revealed interspecific variation in nutrient allocation among leaves and roots as well as their relationship with functional traits and the plant life history strategy.
Keywords: Conspecific density dependence, functional traits, intraspecific variation, life history strategy, nutrient allocation
Received: 22 Dec 2024; Accepted: 19 Feb 2025.
Copyright: © 2025 Cheng and Yu. This is an open-access article distributed under the terms of the Creative Commons Attribution License (CC BY). The use, distribution or reproduction in other forums is permitted, provided the original author(s) or licensor are credited and that the original publication in this journal is cited, in accordance with accepted academic practice. No use, distribution or reproduction is permitted which does not comply with these terms.
* Correspondence:
Shixiao Yu, Sun Yat-sen University, Guangzhou, China
Disclaimer: All claims expressed in this article are solely those of the authors and do not necessarily represent those of their affiliated organizations, or those of the publisher, the editors and the reviewers. Any product that may be evaluated in this article or claim that may be made by its manufacturer is not guaranteed or endorsed by the publisher.
Research integrity at Frontiers
Learn more about the work of our research integrity team to safeguard the quality of each article we publish.