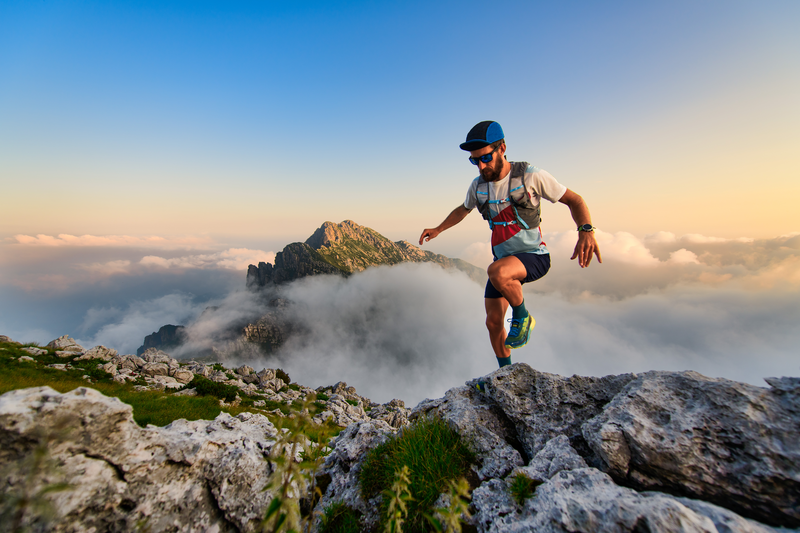
94% of researchers rate our articles as excellent or good
Learn more about the work of our research integrity team to safeguard the quality of each article we publish.
Find out more
ORIGINAL RESEARCH article
Front. Plant Sci.
Sec. Plant Metabolism and Chemodiversity
Volume 16 - 2025 | doi: 10.3389/fpls.2025.1547584
This article is part of the Research Topic In-Depth Interpretation of Critical Genomic Information Related to the Biosynthesis of Key Specialized (Secondary) Metabolism in Medicinal Plants View all 8 articles
The final, formatted version of the article will be published soon.
You have multiple emails registered with Frontiers:
Please enter your email address:
If you already have an account, please login
You don't have a Frontiers account ? You can register here
Aconitum pendulum is a well-known Tibetan medicine that possesses abundant diterpenoid alkaloids (DAs) with high medicinal value. However, due to the complicated structures of DAs and the associated challenges in vitro synthesis presents, plants like Aconitum pendulum remain the primary source for DAs. Given the underutilization of the A. pendulum, a thorough metabolomic and transcriptomic analysis was conducted on its flowers, leaves, and stems to elucidate the regulatory network underlying DA biosynthesis. Metabolomic profiling (utilizing UPLC-QQQ-MS/MS) identified 198 alkaloids, of which 61 were DAs and the relative abundance of DAs was different among different tissues. Without a reference genome, we performed de novo assembly of the transcriptome of A. pendulum. We generated 181,422 unigenes, among which 411 candidate enzyme genes related to the DA synthesis pathway were identified, including 34 DEGs. Through joint analysis of transcriptome and metabolome data, we found a correlation between the detected metabolite levels in various tissues and the expression of related genes. Specifically, it was found that ApCYP1, ApCYP72, and ApCYP256 may be related to turupellin accumulation, while ApBAHD9, ApBAHD10, ApBAHD12 positively associated with the accumulation of aconitine. Furthermore, our study also revealed that genes involved in the diterpene skeleton synthesis pathway tend to be highly expressed in flowers, whereas genes related to DA skeleton synthesis and their subsequent modifications are more likely to be highly expressed in leaf and stem tissues.O-methyltransferases, and 270 CYP450 enzyme genes potentially involved in the biosynthesis of DAs. The co-expression network between metabolites and related genes revealed 116 significant correlations involving 30 DAs and 58 enzyme genes.This study provides valuable resources for in-depth research on the secondary metabolism of A. pendulum, not only deepening our understanding of the regulatory mechanisms of DA biosynthesis but also providing valuable genetic resources for subsequent genetic improvement and metabolic engineering strategies.
Keywords: Aconitum pendulum, Transcriptomic Analysis, Metabolomics, diterpenoid alkaloids biosynthesis, candidate gene
Received: 18 Dec 2024; Accepted: 03 Mar 2025.
Copyright: © 2025 Wang, Xu, Liu, Ding, Wang, Wang, Leng and Zhang. This is an open-access article distributed under the terms of the Creative Commons Attribution License (CC BY). The use, distribution or reproduction in other forums is permitted, provided the original author(s) or licensor are credited and that the original publication in this journal is cited, in accordance with accepted academic practice. No use, distribution or reproduction is permitted which does not comply with these terms.
* Correspondence:
Ting Wang, Chengdu University of Traditional Chinese Medicine, Chengdu, China
Disclaimer: All claims expressed in this article are solely those of the authors and do not necessarily represent those of their affiliated organizations, or those of the publisher, the editors and the reviewers. Any product that may be evaluated in this article or claim that may be made by its manufacturer is not guaranteed or endorsed by the publisher.
Research integrity at Frontiers
Learn more about the work of our research integrity team to safeguard the quality of each article we publish.