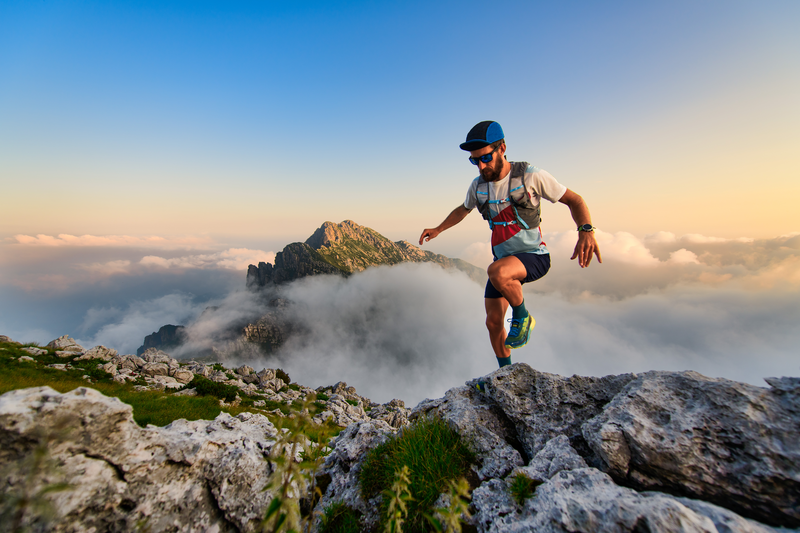
94% of researchers rate our articles as excellent or good
Learn more about the work of our research integrity team to safeguard the quality of each article we publish.
Find out more
ORIGINAL RESEARCH article
Front. Plant Sci.
Sec. Plant Abiotic Stress
Volume 16 - 2025 | doi: 10.3389/fpls.2025.1544879
This article is part of the Research Topic Advanced Breeding for Abiotic Stress Tolerance in Crops, Volume II View all 14 articles
The final, formatted version of the article will be published soon.
You have multiple emails registered with Frontiers:
Please enter your email address:
If you already have an account, please login
You don't have a Frontiers account ? You can register here
N6-methyladenosine (m6A) is the most prevalent posttranscriptional modification in eukaryotic mRNAs. AlkB homologs (ALKBHs) are involved in plant responses to stress by modulating m6A methylation. However, homologous genes in wheat remain largely uncharacterized. In this study, 30 ALKBH genes were identified in wheat, and analyzed their physicochemical properties. The phylogenetic analysis allowed the classification of these genes into seven distinct subfamilies. Additionally, their conserved domains, motif compositions, gene structures, chromosomal localization, and synteny, and the predicted cis-acting elements within their promoters were examined. Expression analysis revealed that TaALKBH9B-5 exhibited the highest expression and its demethylase activity was investigated. Furthermore, TaALKBH9B-5 was significantly upregulated in response to abscisic acid treatment and cold stress, indicating a positive regulatory trend. In conclusion, this study provides a comprehensive genomic assessment of the TaALKBH gene family and offers a theoretical framework for understanding the role of TaALKBH9B in the response to abiotic stress in wheat.
Keywords: AlkB homologs, wheat, M 6 A RNA methylation, Demethylase, abiotic stress
Received: 13 Dec 2024; Accepted: 28 Feb 2025.
Copyright: © 2025 Wang, Zhang, Wu, Yu, Liao, Jian and Sun. This is an open-access article distributed under the terms of the Creative Commons Attribution License (CC BY). The use, distribution or reproduction in other forums is permitted, provided the original author(s) or licensor are credited and that the original publication in this journal is cited, in accordance with accepted academic practice. No use, distribution or reproduction is permitted which does not comply with these terms.
* Correspondence:
Bingjian Sun, College of Plant Protection, Henan Agricultural University, Zhengzhou, 450002, Henan Province, China
Disclaimer: All claims expressed in this article are solely those of the authors and do not necessarily represent those of their affiliated organizations, or those of the publisher, the editors and the reviewers. Any product that may be evaluated in this article or claim that may be made by its manufacturer is not guaranteed or endorsed by the publisher.
Research integrity at Frontiers
Learn more about the work of our research integrity team to safeguard the quality of each article we publish.