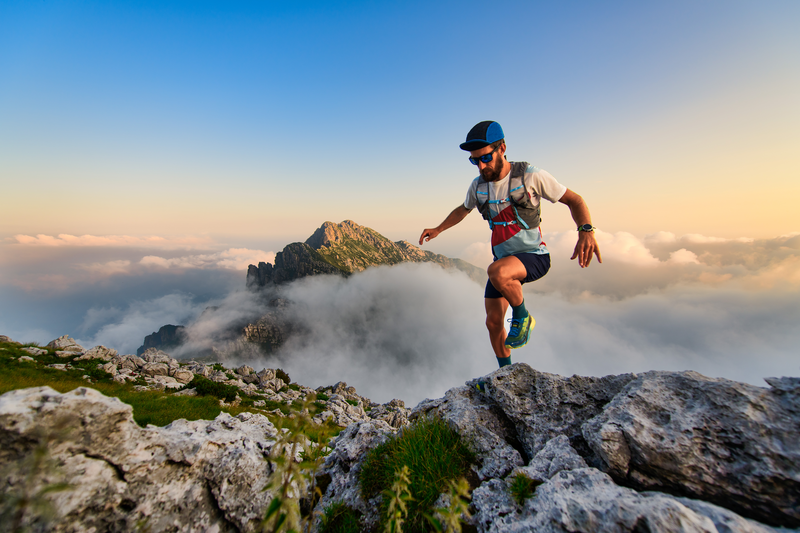
94% of researchers rate our articles as excellent or good
Learn more about the work of our research integrity team to safeguard the quality of each article we publish.
Find out more
ORIGINAL RESEARCH article
Front. Plant Sci.
Sec. Crop and Product Physiology
Volume 16 - 2025 | doi: 10.3389/fpls.2025.1541523
This article is part of the Research Topic New Rootstocks for Fruit Crops: Breeding Programs, Current Use, Future Potential, Challenges and Alternative Strategies, Volume II View all 6 articles
The final, formatted version of the article will be published soon.
You have multiple emails registered with Frontiers:
Please enter your email address:
If you already have an account, please login
You don't have a Frontiers account ? You can register here
The intensification of drought conditions due to climate change poses a major challenge to sustainable grape production. Rootstocks are essential in supporting grapevine water uptake and drought resilience; however, their physiological responses to water stress are not fully understood. Under the hypothesis that root morphology and anatomy may be key traits in grapevine tolerance to water deficit, this study aimed to investigate these traits across diverse rootstocks under progressive water deficit and recovery phases. Thirteen genotypes, including commercial rootstocks and recently bred RG-series and RM2, were evaluated over two seasons in controlled pot-based conditions. Plants were subjected to five distinct watering stages, from well-watered to severe drought. Root traits, such as length, density, and xylem anatomical features, were analysed alongside stem water potential (Ψstem) to gauge plant water status. Results showed significant genotype-specific differences in root morphology and anatomy, impacting drought tolerance and recovery. Rootstocks with higher root length density (RLD) and a larger proportion of fine roots maintained Ψstem more effectively under severe drought. Additionally, smaller xylem vessel diameters and reduced xylem area relative to root cross-sectional area correlated with improved water transport efficiency and faster recovery post-drought. A trade-off emerged wherein increased root density enhanced water uptake capacity but came at the cost of reduced transport efficiency. Notably, rootstocks 420A, 41B, RM2, and Fercal displayed superior drought resilience, while the RG-series did not outperform established genotypes like 13-5 Evex, 110 Richter, and 140 Ruggeri. These results underscore the role of root morphology and anatomy in grapevine drought tolerance, suggesting that these traits could be incorporated as criteria for future rootstocks breeding programs. Nevertheless, field-testing under non-limiting soil conditions is essential to validate these findings.
Keywords: Climate Change, drought, hydraulic conductivity, plant water status, root biomass, root length density, Vitis spp., xylem diameter
Received: 07 Dec 2024; Accepted: 25 Feb 2025.
Copyright: © 2025 Alonso Forn, Buesa, Flor, Sabater, Medrano and Escalona. This is an open-access article distributed under the terms of the Creative Commons Attribution License (CC BY). The use, distribution or reproduction in other forums is permitted, provided the original author(s) or licensor are credited and that the original publication in this journal is cited, in accordance with accepted academic practice. No use, distribution or reproduction is permitted which does not comply with these terms.
* Correspondence:
Ignacio Buesa, University of the Balearic Islands, Palma de Mallorca, Spain
Disclaimer: All claims expressed in this article are solely those of the authors and do not necessarily represent those of their affiliated organizations, or those of the publisher, the editors and the reviewers. Any product that may be evaluated in this article or claim that may be made by its manufacturer is not guaranteed or endorsed by the publisher.
Research integrity at Frontiers
Learn more about the work of our research integrity team to safeguard the quality of each article we publish.